Modular synthesis of triazoles from 2-azidoacrylamides having a nucleophilic amino group†
Received
24th January 2022
, Accepted 15th February 2022
First published on 15th February 2022
Abstract
Assembling methods using 2-azidoacrylamides having a nucleophilic amino group are disclosed. Divergent transformations of the amine-type trivalent platform were accomplished with a wide variety of electrophiles to obtain a broad range of 2-azidoacrylamides involving a fluorosulfonyl group-containing trivalent platform. Consecutive click conjugations including triazole formation, thiol–ene-type 1,4-addition, and SuFEx reactions realized the efficient assembly of easily available simple modules.
Introduction
Facile methods to assemble simple modules onto platform molecules having a number of functional groups for click chemistry are of great significance for the preparation of multifunctional compounds in broad research fields such as pharmaceutical science and chemical biology.1–4 Since click reactions have been in the construction of a vast chemical library and in the synthesis of chemical probes bearing reporter groups, various trivalent platform molecules for consecutive click reactions have been developed so far and an efficient consecutive click assembly using compact platforms is awaited (Fig. 1A).5,6
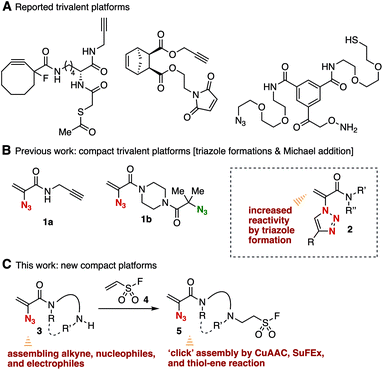 |
| Fig. 1 (A) Trivalent platform molecules. (B) Previous study. (C) This work. | |
We recently succeeded in the synthesis of 2-azidoacrylamides 1 bearing an alkyne or a tertiary azido moiety as compact trivalent platforms (Fig. 1B).6d While 1,4-adducts were not obtained when nucleophiles including thiols and amines were treated with 2-azidoacrylamides 1, 1,4-addition of nucleophiles proceeded efficiently using 2-(1,2,3-triazol-1-yl)acrylamides 2 synthesized from azides 1 by the copper-catalyzed azide–alkyne cycloaddition (CuAAC) reaction with alkynes, clearly showing increased electrophilicity by triazole formation. We herein designed a compact 2-azidoacrylamide platform 5 possessing a sulfonyl fluoride moiety for the sulfur(VI) fluoride exchange (SuFEx) reaction, which is an emerging click reaction7,8 (Fig. 1C). To achieve the synthesis of trivalent platform 5, we conceived a challenging and efficient synthetic route by hydroamination of ethenesulfonyl fluoride (4)9 with biphilic 2-azidoacrylamides 3 having an electrophilic exo-methylene moiety and a nucleophilic free-amino group, paying attention to the inferior electrophilicity of 2-azidoacrylamides 1 compared to that of triazole-substituted acrylamides 2. Since diverse transformations using a free amino group have been established so far, we also expected that amine-type platform 3 can serve as a useful platform to assemble simple modules.
Results and discussion
Synthesis of 2-azidoacrylamides having free amino groups
First, we succeeded in the synthesis of 2-azidoacrylamide 3a having a free secondary amino group (Fig. 2A). Treatment of 2-acrylamide 6a with trifluoroacetic acid in dichloromethane efficiently provided amine 3a after aqueous workup under basic conditions. Although gradual decomposition of amine 3a was observed at room temperature under argon, we fortunately isolated 3a with good purity after the aqueous workup without further purification.10
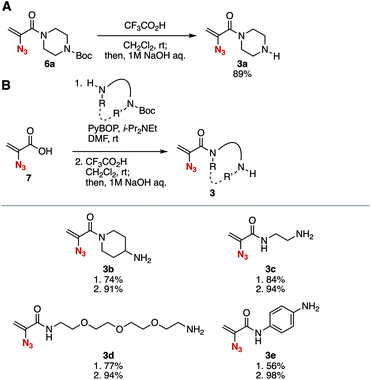 |
| Fig. 2 Synthesis of amines 3. (A) Deprotection of the Boc moiety of 6a. (B) Synthesis of various amines 3 from 2-azidoacrylic acid (7). PyBOP = (benzotriazol-1-yloxy)(trispyrrolidino)phosphonium hexafluorophosphate. See the ESI† for details. | |
A wide range of amines 3b–3e bearing 2-azidoacrylamide moieties were prepared from 2-azidoacrylic acid (7) and amines having tert-butoxycarbonyl (Boc) amide moieties in good yields (Fig. 2B). For example, tertiary amide 3b possessing a primary amino group was synthesized through condensation with 4-(tert-butoxycarbonylamino)piperizine using PyBOP11 followed by the treatment with trifluoroacetic acid in high yield. The synthesis of secondary amides 3c and 3d having a primary amino group was achieved. We found that gradual decomposition of primary amine 3d occurred at room temperature under argon,12 showing similar stability to secondary amine 3a. Also, acrylanilide 3e was synthesized via the condensation between 2-azidoacrylic acid (7) and 4-(tert-butoxycarbonylamino)aniline in moderate yield.
Transformations of 2-azidoacrylamides having free amino groups
Transformations of amine 3a with various electrophiles enabled us to synthesize a broad range of 2-azidoacrylamides 9a–9i in moderate to good yields (Fig. 3). We accomplished the acetylation of amine 3a with acetic anhydride to provide amide 9a in good yield. Synthesis of amide 9b was achieved by the condensation between amine 3a and carboxylic acid 8b using PyBOP without damaging the 2-azidoacrylic amide moiety. Carbamate 9c was successfully prepared from amine 3a and isocyanate 8c under basic conditions. We also succeeded in the guanidination of amine 3a with 1-amidinopyrazole hydrochloride (8d) yielding guanidine 9d quantitatively. Sulfonamide 9e was also prepared from amine 3a using sulfonyl chloride 8e. Triazination of amine 3a with cyanuric chloride (8f) proceeded smoothly to provide triazine 9f in moderate yield. Also, we synthesized amine 9g by the reductive amination of amine 3a with aldehyde 8g using sodium triacetoxyborohydride as a reductant leaving the electrophilic 2-azidoacrylic amide moiety untouched. Arylation of amine 3a with benzyne generated in situ from o-silylphenyl triflate 8h keeping the azido group unreacted, although azides generally react with benzyne to afford benzotriazoles.13 Furthermore, we realized the 1,4-addition of amine 3a with 2-(triazolyl)acrylamide 8i, which was prepared from 1-(2-azidoacryl)-4-(tert-butoxycarbonyl)piperazine (6a) and 4-tolylacetylene by the CuAAC reaction14 in our previous study.6d This result clearly showed that a wide variety of 2-azidoacrylamides can be prepared by sequential transformations using 2-azidoacrylamide-type platforms and alkynes in a modular synthetic manner.
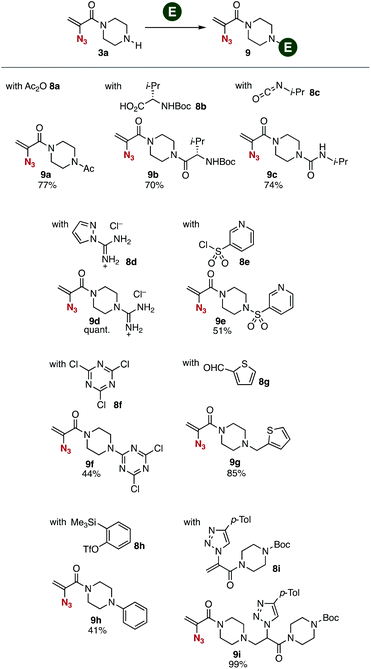 |
| Fig. 3 Synthesis of various 2-azidoacrylamides 9. See the ESI† for details. | |
Subsequent transformations of 2-azidoacrylamides 9 led to the efficient assembly of simple modules (Fig. 4). The CuAAC reaction of azide 9g with alkyne 10a efficiently took place affording 1,2,3-triazole 11 in a quantitative yield. We then achieved the synthesis of amine 13 by the 1,4-addition of 2-(triazolyl)acrylamide 11 with n-butylamine (12) in high yield. These results indicated that diverse 2-(triazolyl)acrylamides can be synthesized from 2-azidoacrylamide 3a, electrophiles, alkynes, and nucleophiles in three steps.
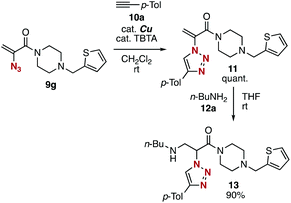 |
| Fig. 4 Synthesis of 2-(triazolyl)amide 13. TBTA = tris((1-benzyl-4-triazolyl)methyl)amine. See the ESI† for details. | |
Synthesis of 2-azidoacrylamides having sulfonyl fluoride moieties
The conjugate addition of amines with ethenesulfonyl fluoride (4) realized the preparation of novel trivalent platforms having an azido, an alkene, and a sulfonyl fluoride group (Fig. 5). Treatment of amine 3a with 4 in the presence of triethylamine provided trivalent platform 5a in an excellent yield leaving three clickable functional groups intact (Fig. 5A). We also accomplished the synthesis of dialkylated amine 5b from primary amine 3d with 4 in moderate yield (Fig. 5B). These results suggested that amine-type trivalent platforms will help in the synthesis of a wide variety of clickable platform molecules with electrophiles bearing functional groups for the click chemistry despite the poor stability of amines 3.
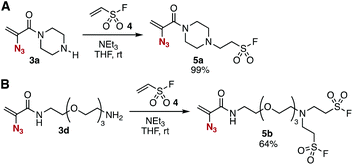 |
| Fig. 5 Synthesis of sulfonyl fluorides 5. (A) Synthesis of 5a. (B) Synthesis of 5b. | |
We succeeded in the synthesis of various 2-azidoacrylamides by the SuFEx reactions of sulfonyl fluoride 5a (Fig. 6).7,8 For example, sulfonyl fluoride 5a efficiently reacted with phenol (14a) under basic conditions to furnish phenyl sulfonate 15a quantitatively without reacting with the 2-azidoacrylamide moiety. Treatment of sulfonyl fluoride 5a with cesium carbonate in 2-propanol (14b) afforded isopropyl sulfonate 15b in high yield. We also achieved the synthesis of phenyl sulfonate 15a by the reaction of silyl ether 16 in the presence of 1,8-diazabicyclo[5.4.0]undec-7-ene (DBU) keeping the electrophilic 2-azidoacrylamide moiety unreacted. Unfortunately, synthesizing sulfonyl amide 15c from sulfonyl fluoride 5a and amine 12b resulted in failure probably due to undesired side reactions such as 1,4-addition.
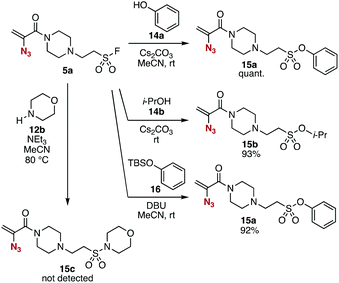 |
| Fig. 6 SuFEx reactions of 5a. See the ESI† for details. | |
Sequential conjugation using trivalent platform 5a
Sequential conjugation of trivalent platform 5a was achieved with alkyne 10a and amine 12a to provide 3-amino-2-triazolyl amide 18 having the sulfonyl fluoride moiety (Fig. 7). Indeed, triazole formation of azide 5a with alkyne 10a catalyzed by tetrakis(acetonitrile)copper and TBTA occurred smoothly to yield 17 in an excellent yield. Then, we successfully prepared amine 18 by efficient conjugate addition using n-butylamine (12a) at room temperature without damaging the amine-susceptive fluorosulfonyl group. These results clearly showed that the 2-azidoacrylamide moiety can react with alkynes and nucleophiles prior to the SuFEx reaction.
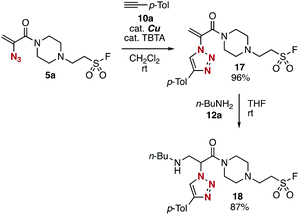 |
| Fig. 7 Modular synthesis of 18. | |
The successful synthesis of 2-triazolylamide 21 by three component assembly indicated the good diversifiable reactivity of trivalent platform 5a (Fig. 8). The CuAAC reaction of phenyl sulfonate 15a followed by the thiol–ene reaction15 with 20 promoted by a base took place efficiently to provide 2-triazolylamide 21 in high yield (Fig. 8A). Moreover, the three component assembly onto platform 5a with phenol (14a), alkyne 10a, and thiol 20 was achieved by the consecutive click reactions in a one-pot fashion (Fig. 8B).
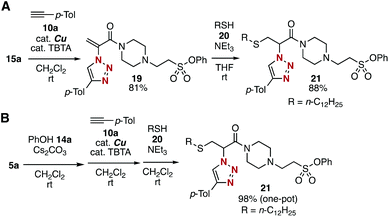 |
| Fig. 8 Modular synthesis of 21. (A) Synthesis of 21via isolated intermediates. (B) One-pot synthesis of 21 from 5a. | |
Synthesis of bis(triazole) 24
To showcase the divergent potential of platforms 3 and 5, we then examined a synthesis of diamide 24 from simple modules (Fig. 9). First, the treatment of amine-type platform 3a with 2-(triazolyl)acrylamide 19 synthesized from trivalent platform 5a, silyl ether 16, and alkyne 10a provided diamide 22 in good yield. Second, CuAAC reaction of diamide 22 proceeded smoothly without damaging amino, triazolyl, and phenoxysulfonyl groups. Third, amine 24 was synthesized efficiently as a mixture of diastereomers by the conjugate addition of n-butylamine (12a) with 2-(triazolyl)acrylamide 23. The good reactivity of 2-(triazolyl)acrylamide 23 as a Michael acceptor will allow us to repeat the conjugation using amine-type platforms 3 to synthesize multi(triazole)s in an iterative manner. Diamide 24 was prepared from trivalent platforms 3a and 5a, alkynes 10a and 10b, amine 12a, and silyl ether 16 in 5 steps. Since alkynes, phenols, silyl ethers, and nucleophiles for 1,4-addition are easily available, the efficient modular synthetic method will be used for synthesizing diverse multi(triazole)s in an iterative manner from simple modules.
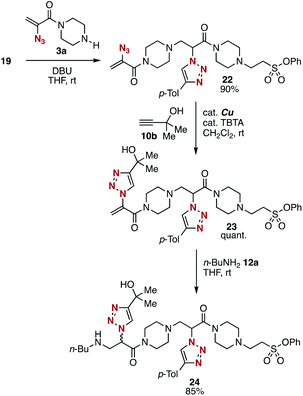 |
| Fig. 9 Modular synthesis of multi(triazole) 24. | |
Conclusions
In summary, we synthesized novel trivalent platforms 5 having azido, alkene, and fluorosulfonyl groups through 2-azidoacrylamides having a free amino group. A wide variety of triazoles were synthesized from trivalent platforms 5 and simple modules by click reactions. Since diverse multi(triazole)s can be prepared in a modular synthetic manner, the combinatorial chemistry using trivalent platforms will be used in constructing a vast chemical library of mid-sized multi(triazole)s. Further studies on the transformations of trivalent platforms 3 and 5 involving the scope and limitations are ongoing in our laboratory.
Conflicts of interest
There are no conflicts to declare.
Acknowledgements
The authors thank Dr Yuki Sakata at Tokyo Medical and Dental University for HRMS analyses. This work was supported by JSPS KAKENHI Grant Number JP19K05451 (C; S. Y.), the Naito Foundation (S. Y.), the Japan Agency for Medical Research and Development (AMED) under Grant Number JP21am0101098 (Platform Project for Supporting Drug Discovery and Life Science Research, BINDS), and the Cooperative Research Project of Research Center for Biomedical Engineering.
Notes and references
-
J. Lahann, Click Chemistry for Biotechnology and Materials Science, John Wiley & Sons, West Sussex, 2009 Search PubMed.
- For reviews of sequential click reactions, see:
(a) A.-C. Knall and C. Slugovc, Chem. Soc. Rev., 2013, 42, 5131 RSC;
(b) Z.-J. Zheng, D. Wang, Z. Xu and L.-W. Xu, Beilstein J. Org. Chem., 2015, 11, 2557 CrossRef CAS PubMed;
(c) S. Yoshida, Org. Biomol. Chem., 2020, 18, 1550 RSC;
(d) D. Sato, Z. Wu, H. Fujita, J. S. Lindsey, D. Sato, Z. Wu, H. Fujita and J. S. Lindsey, Organics, 2021, 2, 161 CrossRef.
- For recent examples of sequential click reactions, see:
(a) I. E. Valverde, A. F. Delmas and V. Aucagne, Tetrahedron, 2009, 65, 7597 CrossRef CAS;
(b) B. C. Sanders, F. Friscourt, P. A. Ledin, N. E. Mbua, S. Arumugam, J. Guo, T. J. Boltje, V. V. Popik and G.-J. Boons, J. Am. Chem. Soc., 2011, 133, 949 CrossRef CAS PubMed;
(c) J. Dommerholt, O. van Rooijen, A. Borrmann, C. F. Guerra, F. M. Bickelhaupt and F. L. van Delft, Nat. Commun., 2014, 5, 5378 CrossRef CAS PubMed;
(d) P. R. Werkhoven, H. van de Langemheen, S. van der Wal, J. A. W. Kruijtzer and R. M. J. Liskamp, J. Pept. Sci., 2014, 20, 235 CrossRef CAS PubMed;
(e) M. Robert, J. Vallée, P. Majkut, D. Krause, M. Gerrits and C. P. R. Hackenberger, Chem. – Eur. J., 2015, 21, 970 CrossRef PubMed;
(f) R. R. Ramsubhag and G. B. Dudley, Org. Biomol. Chem., 2016, 14, 5028 RSC;
(g) D. A. Sutton, S.-H. Yu, R. Steet and V. V. Popik, Chem. Commun., 2016, 52, 553 RSC;
(h) N. Münster, P. Nikodemiak and U. Koert, Org. Lett., 2016, 18, 4296 CrossRef PubMed;
(i) D. Svatunek, N. Houszka, T. A. Hamlin, F. M. Bickelhaupt and H. Mikula, Chem. – Eur. J., 2019, 25, 754 CrossRef CAS PubMed.
- For our recent studies on click chemistry, see:
(a) S. Yoshida, T. Kuribara, H. Ito, T. Meguro, Y. Nishiyama, F. Karaki, Y. Hatakeyama, Y. Koike, I. Kii and T. Hosoya, Chem. Commun., 2019, 55, 3556 RSC;
(b) K. Adachi, T. Meguro, Y. Sakata, K. Igawa, K. Tomooka, T. Hosoya and S. Yoshida, Chem. Commun., 2020, 56, 9823 RSC;
(c) N. Makio, T. Kuribara, K. Adachi, Y. Hatakeyama, T. Meguro, Y. Sakata, K. Igawa, K. Tomooka, T. Hosoya and S. Yoshida, Chem. Commun., 2020, 56, 11449 RSC ; and references therein.
- For recent examples of sequential click reactions using trivalent platforms, see:
(a) V. Vaněk, J. Pícha, B. Fabre, M. Buděšínský, M. Lepšík and J. Jiráček, Eur. J. Org. Chem., 2015, 3689 CrossRef;
(b) B. Fabre, J. Pícha, V. Vaněk, M. Buděšínský and J. Jiráček, Molecules, 2015, 20, 19310 CrossRef CAS PubMed;
(c) B. Fabre, J. Pícha, V. Vaněk, I. Selicharová, M. Chrudinová, M. Collinsová, L. Žáková, M. Buděšínský and J. Jiráček, ACS Comb. Sci., 2016, 18, 710 CrossRef CAS PubMed;
(d) A.-C. Knall, M. Hollauf, R. Saf and C. Slugovc, Org. Biomol. Chem., 2016, 14, 10576 RSC;
(e) T. Yokoi, H. Tanimoto, T. Ueda, T. Morimoto and K. Kakiuchi, J. Org. Chem., 2018, 83, 12103 CrossRef CAS PubMed;
(f) T. Yokoi, T. Ueda, H. Tanimoto, T. Morimoto and K. Kakiuchi, Chem. Commun., 2019, 55, 1891 RSC;
(g) K. Maegawa, H. Tanimoto, S. Onishi, T. Tomohiro, T. Morimoto and K. Kakiuchi, Org. Chem. Front., 2021, 8, 5793 RSC.
- For our recent studies on sequential click reactions using tri- or tetravalent platforms, see:
(a) T. Meguro, Y. Sakata, T. Morita, T. Hosoya and S. Yoshida, Chem. Commun., 2020, 56, 4720 RSC;
(b) N. Terashima, Y. Sakata, T. Meguro, T. Hosoya and S. Yoshida, Chem. Commun., 2020, 56, 14003 RSC;
(c) H. Takemura, S. Goto, T. Hosoya and S. Yoshida, Chem. Commun., 2020, 56, 15541 RSC;
(d) S. Yoshida, Y. Sakata, Y. Misawa, T. Morita, T. Kuribara, H. Ito, Y. Koike, I. Kii and T. Hosoya, Chem. Commun., 2021, 57, 899 RSC ; and references therein.
- For reviews of the SuFEx chemistry, see:
(a) J. Dong, L. Krasnova, M. G. Finn and K. B. Sharpless, Angew. Chem., Int. Ed., 2014, 53, 9430 CrossRef CAS PubMed;
(b) A. S. Barrow, C. J. Smedley, Q. Zheng, S. Li, J. Dongd and J. E. Moses, Chem. Soc. Rev., 2019, 48, 4731 RSC;
(c) Y.-P. Meng, S.-M. Wang, W.-Y. Fang, Z.-Z. Xie, J. Leng, H. Alsulami and H.-L. Qin, Synthesis, 2020, 673 CAS.
- For recent examples of the SuFEx chemistry, see:
(a) R. Zelli, S. Tommasone, P. Dumy, A. Marra and A. Dondoni, Eur. J. Org. Chem., 2016, 5102 CrossRef CAS;
(b) Q. Chen, P. Mayer and H. Mayr, Angew. Chem., Int. Ed., 2016, 55, 12664 CrossRef CAS PubMed;
(c) H.-L. Qin, Q. Zheng, G. A. L. Bare, P. Wu and K. B. Sharpless, Angew. Chem., Int. Ed., 2016, 55, 14155 CrossRef CAS PubMed;
(d) Q. Zheng, J. Dong and K. B. Sharpless, J. Org. Chem., 2016, 81, 11360 CrossRef CAS PubMed;
(e) X. Chen, G.-F. Zha, G. A. L. Bare, J. Leng, S.-M. Wang and H.-L. Qin, Adv. Synth. Catal., 2017, 359, 3254 CrossRef CAS;
(f) H. Wang, F. Zhou, G. Ren, Q. Zheng, H. Chen, B. Gao, L. Klivansky, Y. Liu, B. Wu, Q. Xu, J. Lu, K. B. Sharpless and P. Wu, Angew. Chem., Int. Ed., 2017, 56, 11203 CrossRef CAS PubMed;
(g) J. Leng and H.-L. Qin, Chem. Commun., 2018, 54, 4477 RSC;
(h) C. J. Smedley, M.-C. Giel, A. Molino, A. S. Barrow, D. J. D. Wilson and J. E. Moses, Chem. Commun., 2018, 54, 6020 RSC;
(i) D. Gahtory, R. Sen, S. Pujari, S. Li, Q. Zheng, J. E. Moses, K. B. Sharpless and H. Zuilhof, Chem. – Eur. J., 2018, 24, 10550 CrossRef CAS PubMed;
(j) J. Thomas and V. V. Fokin, Org. Lett., 2018, 20, 3749 CrossRef CAS PubMed;
(k) X. Zhang, B. Moku, J. Leng, K. P. Rakesh and H.-L. Qin, Eur. J. Org. Chem., 2019, 1763 CrossRef CAS;
(l) C. Li, Y. Zheng, K. P. Rakesh and H.-L. Qin, Chem. Commun., 2020, 56, 8075 RSC;
(m) M.-C. Giel, C. J. Smedley, E. R. R. Mackie, T. Guo, J. Dong, T. P. Soares da Costa and J. E. Moses, Angew. Chem., Int. Ed., 2020, 59, 1181 CrossRef CAS PubMed;
(n) S. Li, G. Li, B. Gao, S. P. Pujari, X. Chen, H. Kim, F. Zhou, L. M. Klivansky, Y. Liu, H. Driss, D.-D. Liang, J. Lu, P. Wu, H. Zuilhof, J. Moses and K. B. Sharpless, Nat. Chem., 2021, 13, 858 CrossRef CAS PubMed.
-
(a) B. M. Gladshtein, E. I. Polyanskaya and L. Z. Soborovskii, Zh. Obshch. Khim., 1961, 31, 855 CAS;
(b) J. A. Hyatt and J. J. Krutak, J. Org. Chem., 1977, 42, 169 CrossRef CAS.
- When 3a was placed at room temperature under argon for 3 days, we observed that 65% of 3a was decomposed.
- J. Coste, D. Le-Nguyen and B. Castro, Tetrahedron Lett., 1990, 31, 205 CrossRef CAS.
- When 3d was placed at room temperature under argon for 3 days, we observed that 66% of 3d was decomposed.
- F. Shi, J. P. Waldo, Y. Chen and R. C. Larock, Org. Lett., 2008, 10, 2409 CrossRef CAS PubMed.
-
(a) C. W. Tornøe, C. Christensen and M. Meldal, J. Org. Chem., 2002, 67, 3057 CrossRef PubMed;
(b) V. V. Rostovtsev, L. G. Green, V. V. Fokin and K. B. Sharpless, Angew. Chem., Int. Ed., 2002, 41, 2596 CrossRef CAS;
(c) T. R. Chan, R. Hilgraf, K. B. Sharpless and V. V. Fokin, Org. Lett., 2004, 6, 2853 CrossRef CAS PubMed.
- A. B. Lowe, Polym. Chem., 2014, 5, 4820 RSC.
Footnote |
† Electronic supplementary information (ESI) available: Experimental procedures, characterization for new compounds including NMR spectra. See DOI: 10.1039/d2ob00151a |
|
This journal is © The Royal Society of Chemistry 2022 |
Click here to see how this site uses Cookies. View our privacy policy here.