DOI:
10.1039/D2MD00190J
(Research Article)
RSC Med. Chem., 2022,
13, 1239-1245
Integrating a covalent probe with ubiquicidin fragment enables effective bacterial infection imaging†
Received
23rd June 2022
, Accepted 25th July 2022
First published on 1st August 2022
Abstract
Developing potent and novel bacterial imaging agents remains formidable due to the rapid development of bacterial resistance. Ubiquicidin and its derivatives are the most studied antimicrobial peptides that bind to anionic membranes of a broad range of bacterial pathogens. Studies reveal that UBI (29-41) labeled with 99mTc and 68Ga could distinguish sterile inflammation from infection. A significant challenge that remains for cationic peptides is their poor salt tolerance. The present study deliberates the increment of UBI (29-41) peptide interaction with the bacterial membrane by incorporating 2-acetylphenylboronic acid (2-APBA) as a covalent probe and developing infection imaging probes with improved retention at the target. Given that both 99mTc-UBI (29-41) and 99mTc-UBI (29-41)-2-APBA peptide complexes are stable in serum over 16 h, 99mTc-UBI (29-41)-2-APBA shows enhanced uptake in S. aureus cells as compared to 99mTc-UBI (29-41). SPECT imaging in a mouse model of infection exhibited a higher target to non-target ratio after 2 h in the case of 99mTc-UBI (29-41)-2-APBA. The present study reveals a synergistic mechanism of target binding through covalent conjugation and non-covalent interaction, which could be a potential strategy for improving bacterial infection imaging. As a proof of concept, 99mTc-UBI (29-41)-2-APBA elicits our hypothesis by in vivo imaging of bacterial infection.
Introduction
Bacterial infections are one of the significant causes of mortality and morbidity worldwide. It was reported that in 2017 alone, 20% (11.0 million) of global deaths were due to bacterial sepsis.1 Approximately 5 million deaths were attributed to bacterial antimicrobial resistance (AMR) worldwide in 2019.2 The evolution of drug-resistant pathogens demands focused research on designing new antimicrobials to overcome the crisis. Moreover, the development of fast and non-invasive methods for early detection of infection might play a pivotal role in managing different types of focal infections such as fever of unknown origin (FUO), osteomyelitis, and soft tissue infections (STI).
Direct imaging of bacteria using functional imaging modalities i.e., single photon emission computed tomography (SPECT) and positron emission tomography (PET), are being envisaged for non-invasive and efficient detection of focal infections. Strategies based on a variety of molecules, i.e., bacteria-specific metabolites (sorbitol, maltotriose), antibiotics (ciprofloxacin, rifampicin), antibodies against specific proteins present on microbial surfaces (1D9), and antimicrobial peptides (AMPs) such as ubiquicidin (UBI) and, human lactoferrin (hLF) have been employed to achieve this objective.3,4 AMPs are attractive candidates for developing infection imaging radiopharmaceuticals due to their versatility and desirable pharmacokinetics. AMP derived peptide UBI (29-41) has shown promise as PET as well as SPECT based infection imaging probe.5–9 We have previously demonstrated that ubiquicidin derived peptides selectively interact with the bacterial membrane through electrostatic interaction.10 We hypothesize that detection sensitivity could be improved by modifying the peptide to avoid solely electrostatic dependent interaction with bacterial membrane. In this regard, the stapling of antimicrobial peptides, covalent probe installation, and non-natural amino acid incorporation are being pursued to develop a new generation of antibacterials and imaging agents for clinical translation.11–13
A covalent probe 2-acetyl phenylboronic acid (2-APBA) attached to AMP interacts with the primary amine groups of bacterial phospholipids, Lys-PG (phosphatidylglycerol) and PE (phosphatidylethanolamine) via iminoboronate formation.14 2-APBA probe incorporated AMPs have been established to improve binding to bacterial membrane due to the synergistic action of 2-APBA and the peptides.15,16 Lysylation of PG is a well-known strategy employed by S. aureus to evade the binding of cationic antibiotics.17 But covalent amine recognition strategy implies that lysyl-PG based detection can even help diagnose certain antimicrobial resistant strains.18
The present work aims to modify UBI (29-41) with a 2-APBA probe for bacterial phospholipid specific interaction, eventually improving UBI's binding efficacy. This synergistic interaction (Fig. 1a) leads to the development of 99mTc labeled UBI (29-41)-2-APBA as an infection imaging probe with improved sensitivity. The 99mTc-UBI (29-41)-2-APBA was evaluated for radiochemical purity, in vitro stability and bacterial uptake. Specificity and retention of 99mTc-UBI (29-41)-2-APBA complex was then assessed in infection bearing mice to confirm its potential for in situ imaging of infection.
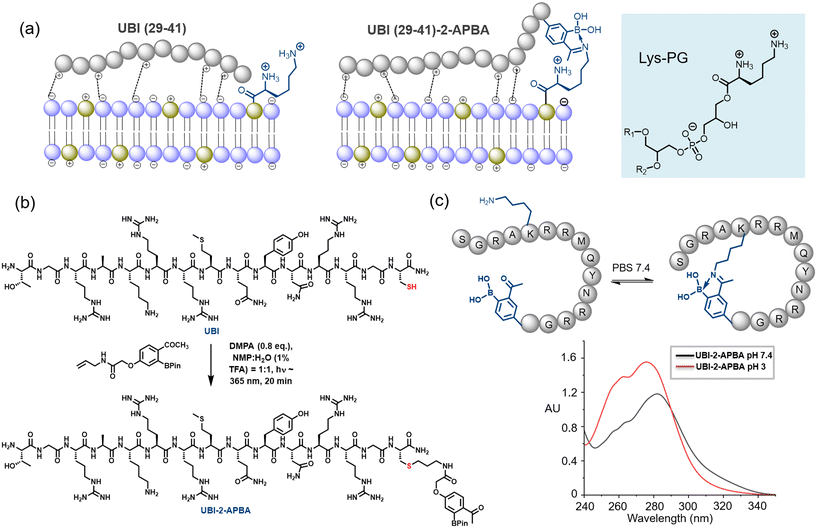 |
| Fig. 1 a) A cartoon representation of synergistic interaction. Iminoboronate formation with Lysyl-PG is shown. b) Installation of 2-APBA probe on UBI (29-41) by thiol–ene click reaction. c) UBI cyclization at physiological pH through iminoboronate is demonstrated by UV-vis spectra. | |
Results and discussion
Synthesis of UBI (29-41)-2-APBA
The synthesis of the peptide was carried out using the conventional Fmoc-based solid-phase peptide synthesis. Further, the 2-APBA moiety was installed on a programmed cysteine moiety at C-terminus via thiol-ene click reaction (Fig. 1b, see ESI UBI-2-APBA synthesis). An alkene handle was positioned with 2-APBA moiety as per the reported procedure.19 A glycine residue has been placed in between UBI (29-41) sequence and 2-APBA moiety installed on the cysteine residue. We hypothesize that the glycine spacer would allow both functional parts to interact on the bacterial plasma membrane without any interference. The UBI (29-41)-2-APBA was purified through HPLC, confirmed its identity via ESI-HRMS (ESI† Fig. S1), and further subjected to studies. As the UBI (29-41)-2-APBA peptide contains a lysine residue, it is expected to form a cyclic structure through intramolecular spontaneous iminoboronate formation, which was confirmed by the UV-vis experiment (Fig. 1c and ESI† Fig. S2). A clear UV-vis red shift from λmax ∼275 nm to ∼282 nm was observed, previously reported by Bandyopadhyay et al.20 This cyclic structure may restrict unwanted modification with lysine side chains of protein during in vivo imaging.
Radiolabeling and in vitro stability studies
Labeling of peptides with 99mTc was carried out at room temperature, pH ∼10 using direct labeling method.21 Both 99mTc-UBI (29-41) and 99mTc-UBI (29-41)-2-APBA complexes were formed with high (>96%) radiochemical purity (RCP). Retention time (Rt) were also comparable for these complexes i.e., 14.89 ± 0.12 min (n = 3) for 99mTc-UBI (29-41) and 15.04 ± 0.07 min (n = 3) for 99mTc-UBI (29-41)-2-APBA complex. Although, % RCP values of 99mTc-UBI (29-41)-2-APBA complex in human serum as well as in PBS at 16 h were higher as compared to 99mTc-UBI (29-41) but statistically significant difference in the stability of the complexes was not observed (Fig. 2).
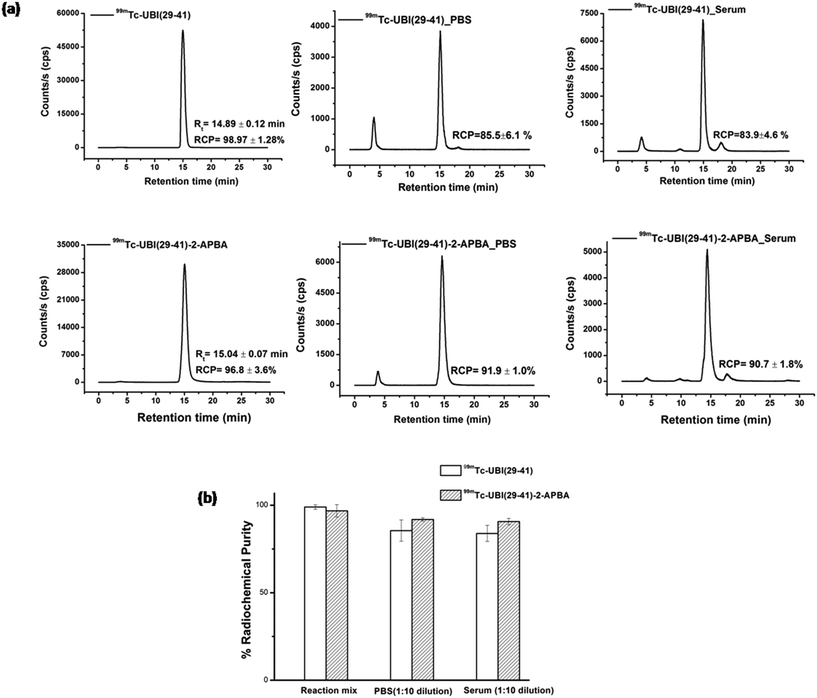 |
| Fig. 2 a) HPLC radio-chromatograms showing in vitro stability of 99mTc-UBI (29-41) (upper panels) and 99mTc-UBI (29-41)-2-APBA (lower panels) in PBS and serum at 16 h. b) Graph depicts % RCP of 99mTc-UBI complexes under specified conditions. | |
Log
P values of −2.39 ± 0.05 (n = 3) and −1.72 ± 0.09 (n = 3) were recorded for 99mTc-UBI (29-41) and 99mTc-UBI (29-41)-2-APBA complexes respectively. These values significantly differed from each other (p < 0.0005). Therefore, the introduction of the 2-APBA group to UBI (29-41) made the peptide more lipophilic. Using molecular mechanics and quantum-mechanical calculations, it was previously reported that the amine groups of Arg (ref. 7) and Lys are the most probable sites for the complex formation with 99mTc.22 We expect 99mTc-UBI (29-41) and 99mTc-UBI (29-41)-2-APBA complexes to be structurally similar (Fig. 3).
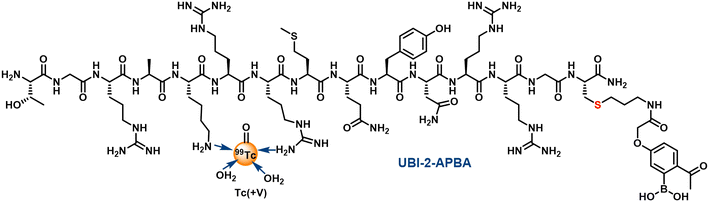 |
| Fig. 3 Probable structure of 99mTc complex of UBI (29-41)-2-APBA. | |
Stability of these complexes were also assessed in L-cysteine and L-histidine (1 mM). The % RCP values for 99mTc-UBI (29-41) and 99mTc-UBI (29-41)-2-APBA (after 16 h of incubation) was found to decrease by ∼15% and ∼6% respectively. On the other hand, the effect of cysteine was more drastic on the RCP of complexes as the % RCP decreased by ∼53% and ∼43% for 99mTc-UBI (29-41) and 99mTc-UBI (29-41)-2-APBA respectively after 16 h (Fig. 4). However, the trans chelation by cysteine was not as severe at 3 h, which may be more relevant from the point of view of imaging with 99mTc (see ESI†).
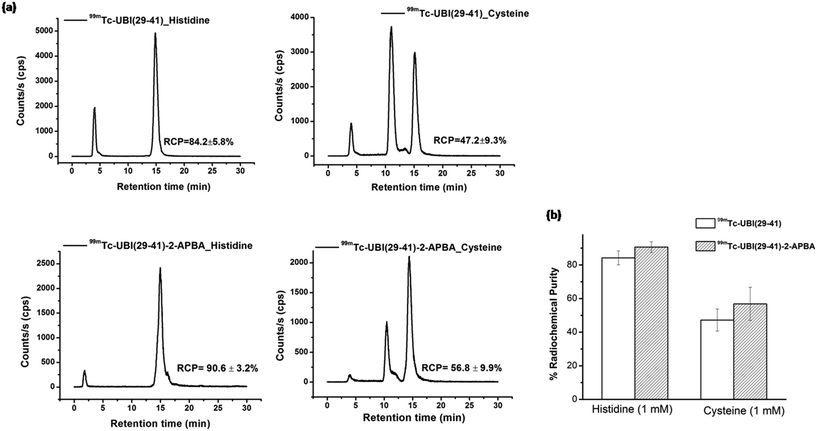 |
| Fig. 4 a) HPLC radio-chromatograms showing in vitro stability of 99mTc-UBI (29-41) (upper panels) and 99mTc-UBI (29-41)-2-APBA (lower panels) in the presence of 1 mM histidine (left) or 1 mM cysteine (right) at 16 h. b) Graph depicts comparison of % RCP of 99mTc-UBI complexes under specified conditions. | |
In vitro uptake assay
Both 99mTc-UBI (29-41) and 99mTc-UBI (29-41)-2-APBA showed good uptake in S. aureus. The % association of 99mTc-UBI (29-41)-2-APBA was significantly higher as compared to 99mTc-UBI (29-41) (Fig. 5). Both the complexes showed a decrease in % uptake with increasing concentration of salt (NaCl), revealing the electrostatic nature of the membrane-peptide interaction. 99mTc-UBI (29-41)-2-APBA showed significantly higher % uptake in bacteria even at elevated NaCl concentrations. This can be explained by the stronger covalent interaction (iminoboronate linkage) between the 2-APBA group with bacterial phospholipid Lysyl-PG and comparatively weaker electrostatic interaction between positively charged amino acids and bacterial phospholipids. It is worth mentioning that Lysyl-PG constitutes up to 10% of all membrane lipids in S. aureus. Therefore, the improved binding of 2-APBA conjugated UBI (29-41) could be explained by synergy between electrostatic interaction (positively charged amino acids and negatively charged lipids) and covalent interaction (2-APBA group and lysyl-PG). Both complexes showed inhibition of uptake in the presence of excess unlabeled peptides confirming the specificity of the peptides for S. aureus membrane.
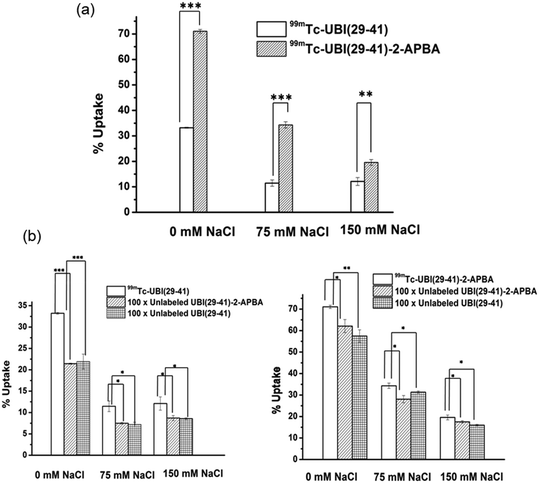 |
| Fig. 5
In vitro studies a) uptake of 99mTc-UBI (29-41)-2-APBA and 99mTc-UBI (29-41) in S. aureus with varying concentrations of NaCl. b) Graphs depicting % uptake of 99mTc-UBI (29-41)-2-APBA and 99mTc-UBI (29-41) in S. aureus in presence of excess conc. of either UBI (29-41)-2-APBA or UBI (29-41). * indicates p < 0.05, ** for p < 0.005 and *** is for p < 0.0005. | |
In situ imaging of bacteria in mice model of infection using SPECT
To test the potential of 99mTc-UBI complexes to detect S. aureus cells in vivo, SPECT imaging was carried out in mice model of infection (right thigh muscle) and sterile inflammation (left thigh muscle). The uptake of UBI complexes was observed at the site of infection (right thigh) (Fig. 6). The target to non-target ratio or T/NT (ratio of tracer uptake in infected right thigh vs. inflamed left thigh) is depicted in Fig. 6a. It was observed that the value of T/NT for 99mTc-UBI (29-41) was maximum (T/NT: 5.2 ± 0.9) at 1 h but declined at 2 h (T/NT: 3.7 ± 0.6), indicating faster clearance from infection foci. On the other hand, the accumulation of 99mTc-UBI (29-41)-2-APBA at the target site continued to improve for 2 h (T/NT: 6.2 ± 1). These observations can be attributed to the more efficient membrane–peptide interaction achieved by introducing the 2-APBA group to UBI (29-41), corroborating the data from in vitro uptake assays. Apart from the target site, the complexes were taken up by the liver, intestine, kidney and bladder. The % uptake in these organs were compared for both complexes, and it was found that the distribution of radiotracers in the liver was not significantly different at either time point. This was surprising as the introduction of 2-APBA to UBI (29-41) was observed to dramatically increase the lipophilicity of the complex, as evident from the log
P values. This observation could perhaps be explained by the intramolecular iminoboronate linkage formed by 2-APBA with the lysine present in UBI (29-41) at physiological pH (Fig. 1c). Due to this “stapling” of the UBI (29-41)-2-APBA conjugate, the newly introduced 2-APBA and lysine residue present in the peptide are not available for interaction with blood proteins. This is further bolstered by the peptide conjugate's fast renal clearance, which was significantly better than the 99mTc-UBI (29-41) (Fig. 6b and c). All animal procedures were performed in accordance with the national guidelines for care and use of laboratory animals and approved by the institutional animal ethics committee of Bhabha Atomic Research Centre, India.
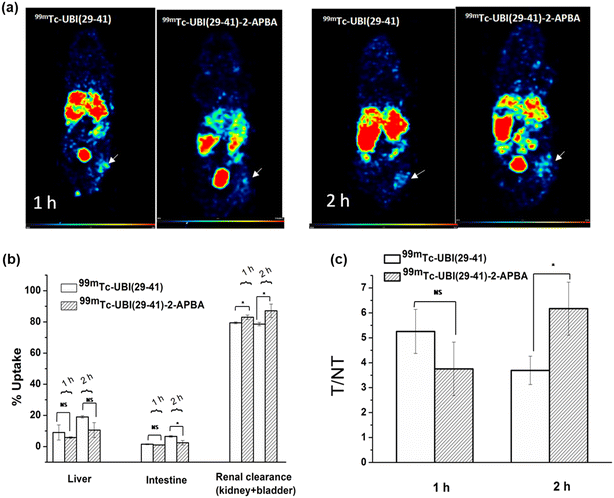 |
| Fig. 6
In vivo studies a) SPECT images of mice model (Balb/c) of infection at various time points showing uptake of 99mTc-UBI (29-41) and 99mTc-UBI (29-41)-2-APBA in infected (right thigh, arrow) vs. inflamed regions (left thigh). b) % uptake in vital organs and renal clearance c) T/NT (target to non-target) ratio at 1 h and 2 h p.i * indicates p < 0.05. | |
Radiolabeling of UBI (29-41) and UBI (29-41)-2-APBA with 99mTc, resulted in a complex with high radiochemical purity. 99mTc-UBI (29-41)-2-APBA showed higher stability in PBS and human serum than 99mTc-UBI (29-41). 99mTc-UBI (29-41)-2-APBA also showed better uptake in S. aureus at elevated salt concentrations. The complex could also differentiate between infection and sterile inflammation in the mice model of infection. Although at an initial time point of 1 h, uptake of 99mTc-UBI (29-41) was higher, at a later time point of 2 h, 99mTc-UBI (29-41)-2-APBA showed higher uptake at the site of infection, which is advantageous in case of 99mTc based tracers. Further studies planned at 3 h and 4 h time points, might lead to new insights on the specific uptake and clearance pattern of the complexes.
Conclusion
We have shown a facile synthesis of 2-APBA installed UBI (29-41) peptide in high yield. The 2-APBA probe did not alter radiolabeling of conjugate with 99mTc, which was reproducible in our studies. In addition, the 99mTc-UBI (29-41)-2-APBA complex was found to be stable in saline and serum. We examined 99mTc-UBI (29-41)-2-APBA, which shows improved in vitro uptake in S. aureus cells as well as at infection foci in mice model of infection by SPECT imaging and compared with 99mTc-UBI (29-41). Interestingly, studies reveal the potential of 99mTc-UBI (29-41)-2-APBA through improved uptake at infection foci at a later time point of 2 h. The synergistic approach of non-covalent interaction along with a covalent bond formation to the target site to improve the affinity of the peptide holds tremendous potential to mitigate the current limitations associated with ubiquicidin derivatives for targeting infections.
Ethics approval and consent to participate
The animal studies reported in the present article were approved by the Institutional Animal Ethics Committee (IAEC) of BARC and all animal experiments were carried out in strict compliance with the institutional guidelines following the relevant national laws related to the conduct of animal experimentation.
Consent for publication
All authors have given their consent for publication of the manuscript.
Author contributions
JB, SC and AK: data curation, investigation, methodology and writing – original draft. AB and AM: conceptualization, formal analysis, funding acquisition, project administration supervision and writing – review & editing.
Conflicts of interest
The authors have no relevant financial or non-financial interests to disclose.
Acknowledgements
Research at the Bhabha Atomic Research Centre (BARC) is part of the ongoing activities of the Department of Atomic Energy, India, and is fully supported by government funding. We appreciatively acknowledge the financial support provided by SERB (SRG/2019/000546) and IIT Ropar through the ISIRD grant. SC acknowledges IIT Ropar for a doctoral fellowship. The authors express their sincere thanks to staff members of BRIT facility for providing 99mTc from 99Mo/99mTc generator and the IIT Ropar central research facility for Mass and NMR. The authors thank staff of animal house facility at BARC for providing support while conducting animal experiments.
References
- K. E. Rudd, S. C. Johnson, K. M. Agesa, K. A. Shackelford, D. Tsoi and D. R. Kievlan,
et al., Global, regional, and national sepsis incidence and mortality, 1990-2017: analysis for the Global Burden of Disease Study, Lancet, 2020, 395(10219), 200–211 CrossRef.
- Antimicrobial Resistance Collaborators, Global burden of bacterial antimicrobial resistance in 2019: a systematic analysis, Lancet, 2022, 399(10325), 629–655 CrossRef.
- I. Polvoy, R. R. Flavell, O. S. Rosenberg, M. A. Ohliger and D. M. Wilson, Nuclear Imaging of Bacterial Infection: The State of the Art and Future Directions, J. Nucl. Med., 2020, 61(12), 1708–1716 CrossRef CAS PubMed.
- A. Signore, V. Artiko, M. Conserva, G. Ferro-Flores, M. M. Welling, S. K. Jain, S. Hess and M. Sathekge, Imaging Bacteria with Radiolabelled Probes: Is It Feasible?, J. Nucl. Med., 2020, 9(8), 2372 CAS.
- E. Vallejo, I. Martinez, A. Tejero, S. Hernandez, L. Jimenez, D. Bialostozky, G. Sanchez, H. Ilarraza and G. Ferro-Flores, Clinical utility of 99mTc-labeled ubiquicidin 29-41 antimicrobial peptide for the scintigraphic detection of mediastinitis after cardiac surgery, Arch. Med. Res., 2008, 39, 768–774 CrossRef CAS PubMed.
- T. Ebenhan, M. M. Sathekge, T. Lengana, M. Koole, O. Gheysens, T. Govender and J. R. Zeevaart, 68Ga-NOTA-Functionalized Ubiquicidin: Cytotoxicity, Biodistribution, Radiation Dosimetry, and First-in-Human PET/CT Imaging of Infections, J. Nucl. Med., 2018, 59(2), 334–339 CrossRef CAS PubMed.
- C. Arteaga de Murphy, F. Gemmel and J. Balter, Clinical trial of specific imaging of infections, Nucl. Med. Commun., 2010, 31(8), 726–733 CrossRef PubMed.
- A. S. Shinto, A. Mukherjee, K. K. Karuppusamy, J. Joseph, J. Bhatt, A. Korde, I. Upadhya, C. Arjun, G. Samuel and A. Dash, Clinical utility of 99mTc-ubiquicidin (29-41) as an adjunct to bone scan in differentiating infected versus noninfected loosening of prosthesis before revision surgery, Nucl. Med. Commun., 2017, 38(4), 285–290 CrossRef CAS PubMed.
- M. Sathekge, O. Garcia-Perez, D. Paez, N. El-Haj, T. Kain-Godoy, I. Lawal and E. Estrada-Lobato, Molecular imaging in musculoskeletal infections with 99mTc-UBI 29-41 SPECT/CT, Ann. Nucl. Med., 2018, 32(1), 54–59 CrossRef CAS PubMed.
- J. Bhatt Mitra, V. K. Sharma, A. Mukherjee, V. Garcia Sakai, A. Dash and M. Kumar, Ubiquicidin-Derived Peptides Selectively Interact with the Anionic Phospholipid Membrane, Langmuir, 2020, 36(1), 397–408 CrossRef CAS PubMed.
- D. Migoń, D. Neubauer and W. Kamysz, Hydrocarbon Stapled Antimicrobial Peptides, Protein J., 2018, 37(1), 2–12 CrossRef.
- A. Bandyopadhyay and J. Gao, Targeting biomolecules with reversible covalent chemistry, Curr. Opin. Chem. Biol., 2016, 34, 110–116 CrossRef CAS PubMed.
- N. Molchanova, P. R. Hansen and H. Franzyk, Advances in Development of Antimicrobial Peptidomimetics as Potential Drugs, Molecules, 2017, 22(9), 1430 CrossRef PubMed.
- H. U. Koch, R. Haas and W. Fischer, The role of lipoteichoic acid biosynthesis in membrane lipid metabolism of growing Staphylococcus aureus, Eur. J. Biochem., 1984, 138(2), 357–363 CrossRef CAS PubMed.
- T. A. Aweda, Z. F. B. Muftuler, A. V. F. Massicano, D. Gadhia, K. A. McCarthy, S. Queern, A. Bandyopadhyay, J. Gao and S. E. Lapi, Radiolabeled Cationic Peptides for Targeted Imaging of Infection, Contrast Media Mol. Imaging, 2019, 2019, 3149249 Search PubMed.
- A. Bandyopadhyay, K. A. McCarthy, M. A. Kelly and J. Gao, Targeting bacteria via iminoboronate chemistry of amine-presenting lipids, Nat. Commun., 2015, 6, 6561 CrossRef CAS PubMed.
- H. Roy, Tuning the properties of the bacterial membrane with aminoacylated phosphatidylglycerol, IUBMB Life, 2009, 61(10), 940–953, DOI:10.1002/iub.240.
- C. J. Slavetinsky, A. Peschel and C. M. Ernst, Alanyl-phosphatidylglycerol and lysyl-phosphatidylglycerol are translocated by the same MprF flippases and have similar capacities to protect against the antibiotic daptomycin in Staphylococcus aureus, Antimicrob. Agents Chemother., 2012, 56(7), 3492–3497 CrossRef CAS PubMed.
- S. Chatterjee, A. Chowdhury, S. Saproo, N. Mani, S. Naidu and A. Bandyopadhyay, ChemRxiv, 2022 DOI:10.26434/chemrxiv-2022-83xg5.
- A. Bandyopadhyay and J. Gao, Iminoboronate-Based Peptide Cyclization That Responds to pH, Oxidation, and Small Molecule Modulators, J. Am. Chem. Soc., 2016, 138(7), 2098–2101 CrossRef CAS PubMed.
- C. Arjun, A. Mukherjee, J. Bhatt, P. Chaudhari, K. M. Repaka, M. Venkatesh and G. Samuel, Studies on batch formulation of a kit for the preparation of the 99mTc-Ubiquicidin (29-41): An infection imaging agent, Appl. Radiat. Isot., 2016, 107, 8–12 CrossRef CAS PubMed.
- L. Meléndez-Alafort, M. Ramírez Fde and G. Ferro-Flores, Arteaga de Murphy C, Pedraza-López M, Hnatowich DJ. Lys and Arg in UBI: a specific site for a stable Tc-99m complex?, Nucl. Med. Biol., 2003, 30(6), 605–615 CrossRef.
|
This journal is © The Royal Society of Chemistry 2022 |