DOI:
10.1039/D2GC00855F
(Paper)
Green Chem., 2022,
24, 3993-4003
Visible-light photocatalytic metal-free multicomponent Ugi-like chemistry†
Received
4th March 2022
, Accepted 19th April 2022
First published on 20th April 2022
Abstract
The possibility of harnessing the photoactivity of isocyanides in the development of metal-free Ugi-like visible light photo-triggered multicomponent transformations has been reported herein. More in detail, Ugi-3CR, Ugi-Tetrazole-3CR, and Ugi–Joullié-3CR afforded imide peptidomimetic derivatives in good yields and with a wide substrate scope, involving the late-stage editing of complex bioactive scaffolds. Furthermore, a 2-step-one-pot sequence affording linear secondary imides, and a 3-step-one-pot protocol leading to densely functionalized bis-amide derivatives have also been developed to highlight the huge potential of these mild metal-free reaction conditions to afford complex and diverse compounds while being in accordance with the green chemistry principles.
Introduction
According to the twelve principles theorized by P. Anastas and J. Warner in 1998, green chemistry could be defined as a science of reduction.1 The reduction or elimination of waste, and, in particular, of hazardous and pollutant substances, as well as the saving of time, resources, and energy consumption, has been set as a must for the development of new sustainable processes for drug manufacturing. In this context multicomponent reactions, defined as one-pot reactions of three or more starting materials, can be considered as “ideal syntheses”. Their hallmarks indeed involve mild reaction conditions, wide scope, convergence, atom economy (thanks to the ability to incorporate all or almost all the atoms of the starting materials), and access to molecular diversity and complexity in high-yields and in shorter times (compared to multistep synthesis). The observed chemoselectivity, accounting for the high yields, is allowed by the domino nature of these transformations and by the fact that most of the potential side reactions are reversible, while the main reaction is not.2 Moreover, the reliability and the possibility of scaling-up have been demonstrated as key features since the first application of the Ugi reaction to the synthesis of anesthetics xylocaine and related analogues in 1959.3 In the realm of multicomponent reactions, those involving isocyanides represent the widest and most flourishing class with the Passerini three-component reaction (P-3CR, 1921)4 followed by the Ugi four-component reaction (U-4CR, 1959)5 (Fig. 1) being considered the stepping stones for the widespread applications of such chemistry, from chemical biology to process chemistry, as observed in the last few decades.6 The robustness and versatility of the U-4CR, along with the possibility to apply “diversification strategies” in terms of both post-Ugi modifications and identification of new multicomponent reactions (e.g., by changing one of the starting materials of the classic U-4CR or by the union of known MCRs, to cite a few) led to the development of Ugi-like chemistry based on the exploration of the unique reactivity of the isocyano-functional group.7
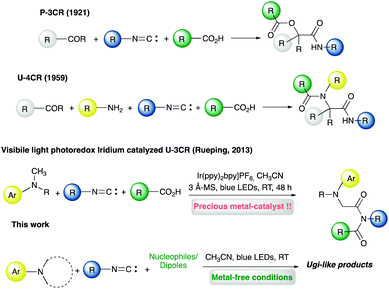 |
| Fig. 1 Original P-3CR and U-4CR, and the visible light photoredox metal-catalyzed Ugi-like 3CR. | |
Recently, we showed that aromatic isocyanides are able to absorb visible light and reach an electronically excited state to promote the oxidation of aromatic tertiary amines.8 This finding provided a route to the exploitation of aromatic isocyanides as visible-light photocatalysts in different transformations such as Mannich, Strecker, aza-Henry, Michael addition, and phosphonylation reactions. On the other hand, we also observed that aliphatic isocyanides could induce the oxidation of tertiary aromatic amines as well via the formation of an electron donor–acceptor (EDA) complex. Intrigued by the possibility to harness this photocatalytic activity in visible-light triggered Ugi-like multicomponent reactions herein we report a small collection of different transformations leading to multifunctional molecular architectures, not always accessible via classic MCRs and under unprecedented metal-free reaction conditions (for a comparison with a literature reported metal-catalyzed photochemical Ugi-like reaction, see Fig. 1).9 While the origins of visible-light photoredox catalysis are undeniably tied to iridium- and ruthenium polypyridyl complexes, endowed with long half-lives (ns to ms), the need for avoiding precious metals has been pushing the field toward the identification of more sustainable organic photocatalysts.10 In this regard, the possibility to harness aromatic isocyanides as self-catalyzing agents in visible-light photocatalytic multicomponent reactions combines multiple green chemistry features to afford new and efficient synthetic methodologies for drug-like scaffolds.11
Results and discussion
Based on our recent findings8 about the photocatalytic properties of both aromatic (direct photoexcitation) and aliphatic isocyanides (EDA complex with aromatic tertiary amines) we wondered if such (photo)reactivity could not only be preserved in the presence of different nucleophiles but also match with their ability to enable multicomponent processes (Fig. 2a).
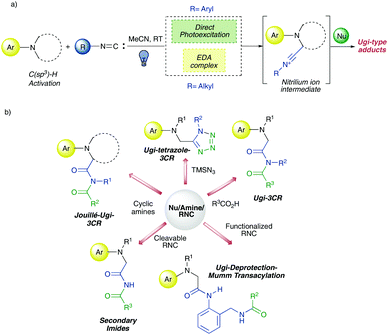 |
| Fig. 2 (a) Mechanistic basis of photo-triggered metal-free multicomponent reactions; (b) transformations investigated herein. | |
Depending on either the selected nucleophiles or the use of functionalized starting amines as well as isocyanides, a range of photo-triggered metal-free multicomponent reactions were investigated (Fig. 2b). More in detail, they involved transformations such as (1) Ugi-3CR (Nu: both aliphatic and aromatic carboxylic acids), (2) Ugi-tetrazole 3CR12 (Nu: TMSN3), (3) Jouillè-Ugi-3CR13 (cyclic tertiary aromatic amines as starting materials), (4) synthesis of secondary imides by using 2,4-dimethoxybenzyl isocyanide as a cleavable one (to our knowledge, reported herein for the first time), and (5) a one-pot domino sequence of Ugi-3CR/deprotection/Mumm transacylation to afford multi-functionalized Ugi-like diamides (Fig. 2b).
Ugi-3CR
In order to verify the feasibility of an Ugi-like 3CR, N,N-dimethylaniline 1 (2 equiv.), cyclohexyl isocyanide 2 (1 equiv.), and m-toluic acid 3 (2 equiv.) were dissolved in acetonitrile and reacted overnight at room temperature under blue LED light irradiation to give the imide 4 in 99% yield after 72 h (Table 1, entry 1). Decreasing the equivalents of the acid component to 1.5 and 1 gave 99 and 82% yields, respectively (Table 1, entries 2 and 3), while shorter reaction times (Table 1, entry 4) as well as the absence of either molecular sieves or light proved to be detrimental with respect to entries 1 and 2 (Table 1, entries 5 and 6).
Table 1 Optimization of the reaction conditions for the Ugi-3CRa

|
Entry |
Equiv. of 1 |
Equiv. of 2 |
Equiv. of 3 |
Time |
Yield |
MeCN 0.1 M, 30 W blue LEDs, 3 Å MS, RT, open to air.
NMR yield.
No MS.
In the dark.
|
1 |
2 |
1 |
2 |
72 h |
99% |
2 |
2 |
1 |
1.5 |
72 h |
99% |
3 |
2 |
1 |
1 |
72 h |
82%b |
4 |
2 |
1 |
1.5 |
48 h |
83%b |
5c |
2 |
1 |
1.5 |
72 h |
83%b |
6d |
2 |
1 |
1.5 |
72 h |
Traces |
With the aim of investigating the substrate scope and the robustness of the metal-free visible light triggered Ugi-like 3CR, the optimized reaction conditions (entry 2, Table 1) were applied to different both aliphatic and aromatic isocyanides (4–7, Fig. 3). Similarly, both aromatic and aliphatic carboxylic acids showed to be competent starting materials, including complex molecular architectures such as ibuprofen, biotin, indomethacin, and cholic acid (8–11, Fig. 3). These results clearly show how the mildness of the developed transformation proved to be suitable for the late-stage editing of drugs and natural compounds. Interestingly, the use of difunctionalized starting materials, such as the terephthalic acid, led to dimeric derivative 12 in a good 73% yield (Fig. 3). A detailed reaction mechanism is proposed in Scheme 1.
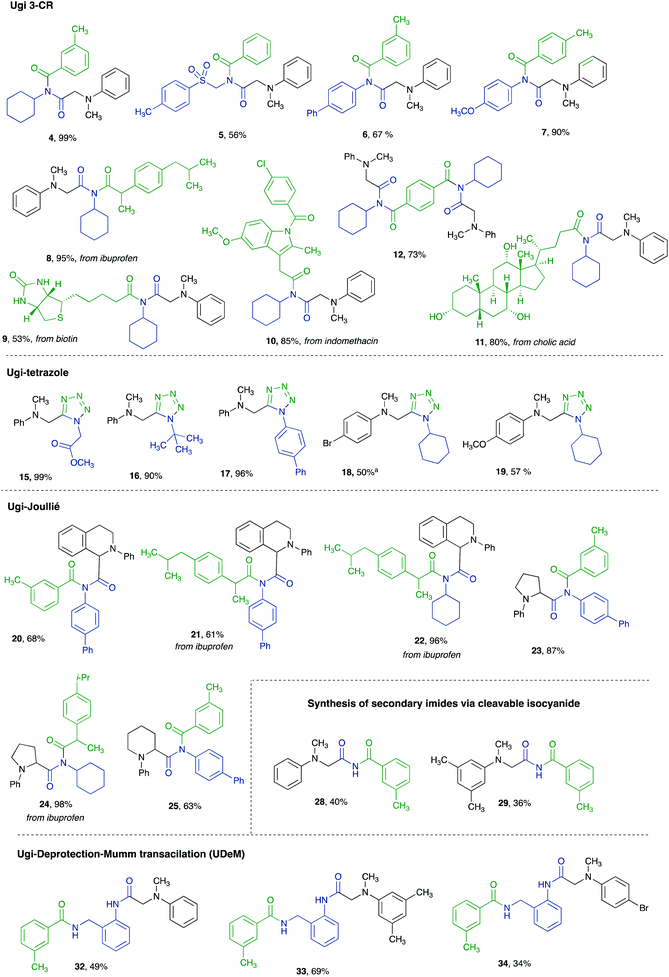 |
| Fig. 3 Synthesized compounds via photocatalytic metal-free multicomponent reactions (a: 3 equiv. of both 2 and TMSN3 used). | |
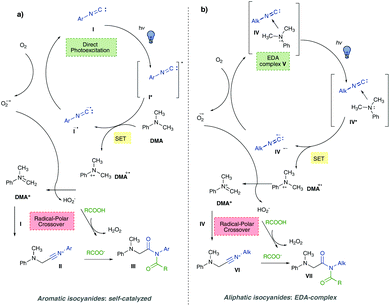 |
| Scheme 1 Detailed reaction mechanism. | |
Aromatic isocyanides I upon light absorption, are able to reach an electronically excited state, thus acting as photocatalysts: a SET from DMA to I* led to the formation of the imidoyl radical anion I˙− and the radical cation of dimethylaniline DMA˙+. Molecular oxygen was then able to regenerate isocyanides I, while forming a superoxide radical anion O2˙−, which abstracted a hydrogen atom from DMA˙+, thus leading to the formation of the iminium ion DMA+. The latter was then intercepted by the ground-state isocyanides I to afford the nitrilium ion II and eventually, after the addition of a carboxylate anion, generated upon deprotonation of the carboxylic acid mediated by HO2−, the imide III (Scheme 1a).
In the case of aliphatic isocyanides IV, visible-light excitation of the EDA complex V triggered a SET from DMA (the donor) to the isocyanide IV (the acceptor), thus forming the radical cation DMA˙+ and the isocyanide radical anion IV˙−. The latter was oxidized back to IV by molecular oxygen furnishing a superoxide radical anion O2˙−, which was responsible for hydrogen atom abstraction from DMA˙+, eventually affording the iminium ion DMA+. As previously, the addition of the ground-state isocyanide IV, and then of the carboxylate anion afforded the imide products VII. In both cases the formation of imides proceeded via a radical/polar crossover pathway.
Ugi-tetrazole 3-CR
Prompted by the good yields and the excellent substrate scope of the Ugi-3CR, we wondered if the presence of a different nucleophile such as azidotrimethylsilane (TMSN3), able to react with the in situ formed nitrilium ion as a 1,3-dipole in a [3 + 2] cycloaddition reaction, could be tolerated under the required photocatalytic conditions and could afford 1,5-disubstituted tetrazole derivatives.12 Accordingly, N,N-dimethylaniline 1 (2 equiv.), cyclohexyl isocyanide 2 (1 equiv.), and TMSN313 (1.5 equiv.) were reacted under standard conditions as for the Ugi-3CR (Table 2, entry 1) to give the desired tetrazole 14 in 60% yield. Increasing the equivalents of isocyanide 2 and TMSN3 to 3, while decreasing the aniline (Table 1, entry 2), led to 86% yield. To verify the possibility of either decreasing or eliminating the amount of organic solvent, the reaction was also tested in a MeCN/water 1
:
1 mixture and in a 2 wt%. TPGS-750 M micellar solution (Table 2, entries 3 and 4).
Table 2 Optimization of the reaction conditions for the Ugi-tetrazole-3CRa
Unfortunately, these reaction conditions led to either a modest 30% yield or traces of the product. A further test reaction with 2 equivalents of both the isocyanide 2 and TMSN3 in acetonitrile revealed that this stoichiometry was already sufficient to afford an excellent 92% yield (Table 2, entry 5). As for the previous transformation, the Ugi-tetrazole-3CR needed light irradiation to be promoted (Table 2, entry 6). These reaction conditions proved to be optimum with both primary, secondary, and tertiary aliphatic isocyanides (15, 16, 18, and 19, Fig. 3) as well as with aromatic ones (17, Fig. 3). Similarly, both electron-poor and electron-rich aromatic tertiary amines afforded the corresponding tetrazole derivatives, albeit in moderate yields (18 and 19, Fig. 3).
Ugi–Joullié 3-CR
The three-component Ugi–Joullié reaction (UJ-3CR), reported for the first time in 1982, involves a cyclic imine, an isocyanide, and a carboxylic acid to give an acyl derivative of a secondary cyclic amine (Fig. 4a).13
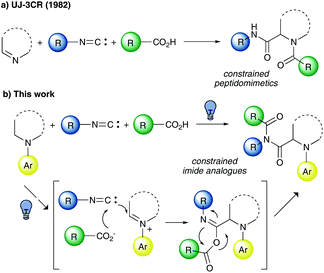 |
| Fig. 4 (a) Ugi–Joullié three-component reaction; (b) visible light photocatalytic Ugi–Joullié three-component reaction developed herein. | |
Over the past years, it has been exploited as a very useful synthetic methodology to access conformationally constrained peptidomimetics and antibacterial depsipeptides.14 Furthermore, it has been investigated, using an α-siloxy five-membered cyclic imine, as a solvent-dependent diastereodivergent reaction.15 Given the relevance of such multicomponent transformation, we wondered if the use of a tertiary cyclic aromatic amine, oxidized in situ to an iminium ion via the photocatalytic activity of an isocyanide and reacted with a carboxylic acid, could afford imide derivatives (Fig. 4b).
By applying standard conditions as for the Ugi-3CR (Table 1, entry 2) a small library of imide derivatives (20–25, Fig. 3) was obtained. The reaction proved to be suitable for different cyclic tertiary aromatic amines, such as N-phenyl-tetrahydroisoquinoline (20–22Fig. 3), N-phenylpyrrolidine (23 and 24, Fig. 3), and N-phenylpiperidine (25, Fig. 3). Interestingly, both aromatic and aliphatic isocyanides were able to afford the desired constrained imide derivatives, thus proving the feasibility of a catalytic activity of isocyanides via either a direct photoexcitation (in the case of aromatic substrates) or the formation of an EDA complex (in the case of aliphatic substrates) also in the UJ-3CR.
Ugi-3CR followed by one-pot post-condensation modifications
To further highlight the advantages of the metal-free visible light photocatalytic mild reaction conditions developed herein, we carried out different post-condensation modifications. This approach was very useful to enable two-step one-pot multicomponent reactions: after performing the photocatalytic Ugi-3CR, the reaction conditions were changed without any intermediate purification, to trigger a new transformation.16 More in detail, the synthesis of secondary imides via the use of a cleavable isocyanide and an Ugi-3CR/deprotection/Mumm transacylation sequence were chosen as representative multi-step one-pot processes.
Accordingly, the Ugi-3CR with 2,4-dimethoxybenzyl isocyanide 26 afforded tertiary imides with a general structure 27 (Scheme 2), which were converted in situ into secondary linear imides 28–29 (Scheme 2 and Fig. 3) by stirring the crude reaction mixture in a 1
:
1 DCM/TFA mixture at 50 °C for 4 hours. It is worth noting that to our knowledge, isocyanide 26 was reported as a cleavable one for the first time. The latter was demonstrated to be superior to the well-known Walborsky reagent,6b as when 1,1,3,3-tetramethylbutyl isocyanide was reacted with N,N-dimethylaniline 1 and m-toluic acid 3 to give the corresponding Ugi-3CR adduct, and then stirred in TFA to attempt the removal of the isocyanide alkyl moiety, the desired secondary imide was obtained only in traces.
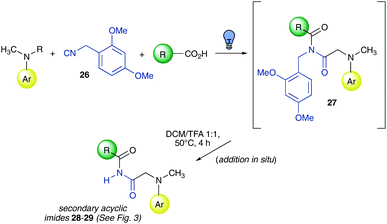 |
| Scheme 2 Synthesis of secondary imides via the use of 26 as a cleavable isocyanide. | |
The second post-condensation modification, investigated herein under photocatalytic conditions, relied on a one-pot three-step Ugi-3CR/Deprotection/Mumm transacylation sequence. This transformation, reported herein for the first time, would be reminiscent of both the PADAM protocol17 reported by Prof. L. Banfi in 2000 (Fig. 5a) for the synthesis of peptide-like structures and the split-Ugi reaction18 reported by Prof. G. B. Giovenzana and Prof. G. C. Tron in 2006 where bis-secondary diamines were shown to mimic primary amines in the Ugi-4CR (Fig. 5b).
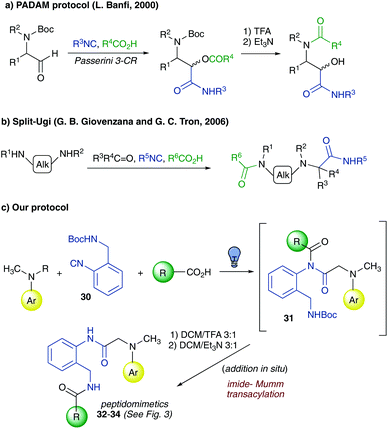 |
| Fig. 5 One-pot Ugi-3CR/deprotection/Mumm transacylation sequence. | |
In analogy with these two interesting transformations, we speculated that the use of isocyanide 30 endowed with a Boc-protected amino group (Fig. 5c), tethered in the ortho-position, would afford a tertiary imide 31 (Fig. 5c), which could undergo a base-promoted Mumm transacylation step after the acidic cleavage of the Boc-protecting group (32–34, Fig. 5c and Fig. 3). The reaction, albeit requiring, as in the PADAM protocol, the deprotection of the nucleophilic primary amine, relied, like the split-Ugi reaction, on the transacylation of a nitrogen atom. It is worth noting that while the latter transformation provided access to tertiary amide bonds and the original Ugi-4CR afforded both a tertiary and a secondary amide bond, the protocol herein developed allowed the formation of peptidomimetics endowed with two secondary amide bonds. Furthermore, this reaction also revealed how tertiary imides could be good acylating agents in the presence of a suitable nucleophile (i.e., the primary amine). To our knowledge this behaviour has never been reported in the literature and sheds light on the versatility of these Ugi-like products as useful synthetic intermediates.19 According to Fig. 3, electron-donor substituents on the amine aromatic ring seem to be able to afford better yields (33, Fig. 3) with respect to electron-withdrawing ones (34, Fig. 3).
Conclusions
Starting from the key finding relying on the visible light photoactivity of isocyanides, different multicomponent processes such as the Ugi-3CR, the Ugi–Tetrazole-3CR, and the Ugi–Joullié-3CR have been developed herein, for the first time, under metal-free visible light photocatalytic reaction conditions. The reported MCRs enabled the access to peptidomimetic imide derivatives in good yields (U-3CR, UT-3CR, UJ-3CR, respectively, Fig. 6) and with a wide substrate scope. The exploitation of such mild conditions was also successful with complex molecular architectures, as demonstrated in the late-stage editing of both drugs and biorelevant compounds (e.g., biotin and cholic acid). It is worth noting that the possibility to harness the photocatalytic activity of isocyanides under irradiation with visible light enabled to avoid the use of additional oxidants such as benzoyl peroxide20 and DEAD21 as well as the use of photocatalysts such as either ruthenium- or iridium-based polypyridyl complexes22 or organic dyes.23 Furthermore, a two-step-one-pot Ugi-like 3CR (UC-3CR-2S), using 2,4-dimethoxybenzyl isocyanide as a cleavable one, afforded linear non-cyclic secondary imides. To our knowledge, such isocyanide was reported herein as a cleavable one for the first time and proved to be superior to other well-known isocyanides that are able to release a C- or a CN-unit. It should also be considered that the moderate 38% medium yield corresponds to a 62% theoretical yield for each step (i.e., the MCR and TFA-promoted cleavage of the 2,4-dimethoxybenzyl moiety, Fig. 6).
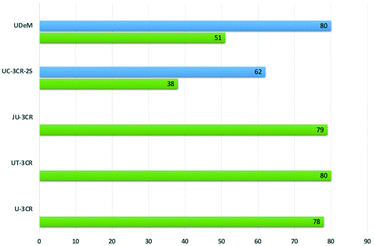 |
| Fig. 6 Medium yields of the photocatalyzed MCRs developed herein (in blue, theoretical yield per single step). | |
Finally, to further highlight the potential of such developed metal-free visible light photocatalytic methodologies in the exploration of a wide chemical space, we established a one-pot three-step multicomponent protocol (UDeM) involving a U-3CR, a Boc-deprotection step, and a base-promoted transacylation of the imide intermediate affording densely functionalized peptidomimetics. As for the previous transformation, the medium 51% yield of the three-step protocol stands for a medium 80% theoretical yield for each step (UDeM, Fig. 6), while keeping all the advantages of avoiding multiple purifications, longer times, the use of protecting groups and coupling agents, otherwise required for multistep syntheses. In conclusion, it has been shown herein how the combination of visible light photochemistry and isocyanide-based multicomponent chemistry could boost the development of green synthetic protocols useful to efficiently provide diversity and complexity, key to medicinal chemistry research and discovery campaigns, in short times while saving energies and resources, so precious, yet highly demanded and still too quickly depleting.
Experimental
General methods
Commercially available reagents and solvents were used without further purification. Photochemical reactions were carried out using a PhotoRedOx Box (EvoluChem™) with 30 W blue LEDs (EvoluChem™, model: HCK1012-01-008, wavelength 450 nm, LEDs: CREE XPE. A holder suitable for 4 ml scintillation vials (45 × 14.7 mm) has been fitted within the box: this allows a fixed sample placement distance from the light source). All NMR spectra were recorded with a Bruker Avance NEO 400 or 700 MHz instrument. Experiments for structural elucidation were performed in CDCl3 at 25 °C with an RT-DR-BF/1H-5 mm-OZ SmartProbe. High-resolution ESI-MS spectra were recorded using a Thermo LTQ Orbitrap XL mass spectrometer. The spectra were recorded by infusion into the ESI source using MeOH as the solvent. Chemical shifts (δ) are reported in parts per million (ppm) relative to the residual solvent peak. Column chromatography was performed on silica gel (70–230 mesh ASTM) using the reported eluents. Thin layer chromatography (TLC) was carried out on 5 × 20 cm plates with a layer thickness of 0.25 mm (silica gel 60 F254) to monitor the reaction by using UV as the revelation method.
General procedure for the synthesis of compounds 4–8, 10 and 20–25
To a 4 mL colourless screw-cap glass vial equipped with a magnetic stir bar, isocyanide (0.08 mmol), carboxylic acid (0.12 mmol, 1.5 equiv.), the aniline derivative (0.16 mmol, 2 equiv.) and 800 μL of dry MeCN (0.1 M) were added; 80 mg of 3 Å activated molecular sieves were then added to the resulting mixture, which was stirred open flask in a PhotoRedOx Box (EvoluChem™), under 30 W blue LED irradiation, at room temperature, until the completion of the reaction, as monitored by TLC (specific reaction times are available for each compound). Then the solvent was removed under vacuum and the crude mixture was purified by silica gel chromatography.
N-Cyclohexyl-3-methyl-N-(N-methyl-N-phenylglycyl)benzamide (4).
The crude material (reaction time: 72 h) was purified by column chromatography (n-hexane/ethyl acetate 99
:
1) to give the product as a pale yellow amorphous solid (28.9 mg, 99% yield also when performed on a 0.8 mmol scale). 1H NMR (400 MHz, CDCl3) δ 7.33 (d, J = 7.6 Hz, 1H), 7.28 (d, J = 7.6 Hz, 1H), 7.22–7.19 (m, 2H), 7.16–7.12 (m, 2H), 6.77 (t, J = 7.3 Hz, 1H), 6.42 (d, J = 8.1 Hz, 2H), 4.03–3.95 (m, 1H), 3.86 (s, 2H), 2.62 (s, 3H), 2.22 (s, 3H), 1.95–1.86 (m, 2H), 1.76–1.72 (m, 4H), 1.58–1.55 (m, 1H), 1.23–1.11 (m, 3H); 13C NMR (101 MHz, CDCl3) δ 174.1, 173.4, 148.3, 138.5, 134.8, 133.6, 129.9, 128.9, 128.3, 126.2, 118.6, 113.2, 58.6, 58.2, 39.9, 30.0, 26.23, 25.2, 21.2; HRMS (ESI) m/z: calcd [M + H]+ for C23H29N2O2+ 365.2224; found [M + H]+ 365.2225.
N-([1,1′-Biphenyl]-4-yl)-3-methyl-N-(N-methyl-N-phenylglycyl)benzamide (6).
The crude material (reaction time: 72 h) was purified by column chromatography (n-hexane/ethyl acetate 98
:
2) to give the product as a reddish sticky solid (23.4 mg, 67% yield). 1H NMR (700 MHz, CDCl3) δ 7.51–7.49 (m, 4H), 7.40 (t, J = 7.7 Hz, 2H), 7.33 (t, J = 7.4 Hz, 1H), 7.30–7.28 (m, 2H), 7.25 (d, J = 8.8 Hz, 1H), 7.18–7.17 (m, 2H), 7.14 (d, J = 8.4 Hz, 2H), 7.09–7.07 (m, 1H), 6.85 (t, J = 7.3 Hz, 1H), 6.70 (d, J = 8.2 Hz, 2H), 4.44 (s, 2H), 2.90 (s, 3H), 2.16 (s, 3H); 13C NMR (176 MHz, CDCl3) δ 176.0, 172.1 148.6, 140.4, 140.0, 138.0, 137.6, 133.4, 133.0, 130.7, 129.3, 128.8, 128.1, 128.0, 127.6, 127.5, 127.2, 127.1, 118.1, 112.7, 58.4, 40.6, 21.2; HRMS (ESI) m/z: calcd [M + H]+ for C29H27N2O2+ 435.2067; found [M + H]+ 435.2053.
N-(4-Methoxyphenyl)-4-methyl-N-(N-methyl-N-phenylglycyl)benzamide (7).
The crude material (reaction time: 72 h) was purified by column chromatography (n-hexane/ethyl acetate 98
:
2) to give the product as a reddish sticky solid (27.9 mg, 90% yield). 1H NMR (700 MHz, CDCl3) δ 7.25 (d, J = 8.2 Hz, 2H), 7.23–7.20 (m, 2H), 6.94–6.92 (m, 4H), 6.77 (t, J = 7.3 Hz, 1H), 6.75 (d, J = 8.9 Hz, 2H), 6.64 (d, J = 8.2 Hz, 2H), 4.37 (s, 2H), 3.68 (s, 3H), 2.87 (s, 3H), 2.24 (s, 3H); 13C NMR (176 MHz, CDCl3) δ 176.0, 172.1, 158.7, 148.7, 143.2, 131.2, 130.6, 130.0, 129.2, 128.9, 128.6, 117.8, 114.7, 112.6, 58.4, 55.4, 40.5, 21.6; HRMS (ESI) m/z: calcd [M + H]+ for C24H25N2O3+ 389.1860; found [M + H]+ 389.1852.
N-Cyclohexyl-2-(4-isobutylphenyl)-N-(N-methyl-N-phenylglycyl)propanamide (8).
The crude material (reaction time: 48 h) was purified by column chromatography (n-hexane/ethyl acetate 99.5
:
0.5) to give the product as a pale-yellow sticky solid (33.0 mg, 95% yield). 1H NMR (700 MHz, CDCl3) δ 7.17 (d, J = 8.0 Hz, 2H), 7.15–7.12 (m, 4H), 6.67 (t, J = 7.3 Hz, 1H), 6.53 (d, J = 8.2 Hz, 2H), 4.38 (d, J = 18.0 Hz, 1H), 4.30 (d, J = 18.0 Hz, 1H), 4.01 (q, J = 6.8 Hz, 1H), 3.70–3.66 (m, 1H), 3.00 (s, 3H), 2.46 (d, J = 7.2 Hz, 2H), 2.21–2.15 (m, 1H), 1.85–1.79 (m, 2H), 1.77–1.75 (m, 1H), 1.63–1.61 (m, 1H), 1.49–1.48 (m, 5H), 1.18–1.12 (m, 1H), 1.03–0.97 (m, 1H), 0.87 (d, J = 6.7 Hz, 6H), 0.85–0.79 (m, 1H), 0.74–0.73 (m, 1H); 13C NMR (176 MHz, CDCl3) δ 178.8, 175.9, 148.9, 141.0, 137.7, 129.9, 129.1, 127.2, 116.9, 112.0, 59.2, 58.7, 46.9, 45.0, 39.8, 30.6, 30.3, 29.0, 26.8, 26.4, 25.0, 22.3, 22.2, 20.3; HRMS (ESI) m/z: calcd [M + H]+ for C28H39N2O2+ 435.3007; found [M + H]+ 435.3020.
2-(1-(4-Chlorobenzoyl)-5-methoxy-2-methyl-1H-indol-3-yl)-N-cyclohexyl-N-(N-methyl-N-phenylglycyl)acetamide (10).
The crude material (reaction time: 72 h) was purified by column chromatography (n-hexane/ethyl acetate 96
:
4) to give the product as a yellowish solid (40.0 mg, 85% yield). 1H NMR (700 MHz, CDCl3) δ 7.65 (d, J = 8.4 Hz, 2H), 7.47 (d, J = 8.4 Hz, 2H), 7.17–7.15 (m, 2H), 6.93 (d, J = 2.4 Hz, 1H), 6.82 (d, J = 9.0 Hz, 1H), 6.71 (t, J = 7.3 Hz, 1H), 6.68 (dd, Ja = 9.0, Jb = 2.5 Hz, 1H), 6.59 (d, J = 8.3 Hz, 2H), 4.39 (s, 2H), 3.96 (s, 2H), 3.79 (s, 3H), 3.72–3.69 (m, 1H), 3.02 (s, 3H), 2.41 (s, 3H), 2.21–2.16 (m, 2H), 1.77–1.75 (m, 2H), 1.62–1.50 (m, 3H), 1.15–1.08 (m, 3H); 13C NMR (176 MHz, CDCl3) δ 175.5, 175.0, 168.2, 156.1, 148.8, 139.5, 135.9, 133.7, 131.2, 130.8, 130.4, 129.2 (4C), 117.3, 115.1, 112.4, 112.1, 111.7, 101.2, 59.3, 59.1, 55.7, 39.7, 34.6, 30.1, 26.6, 24.9, 13.5; HRMS (ESI) m/z: calcd [M + H]+ for C34H37ClN3O4+ 586.2467; found [M + H]+ 586.2487; m.p. 80–81 °C.
N-([1,1′-Biphenyl]-4-yl)-N-(3-methylbenzoyl)-2-phenyl-1,2,3,4-tetrahydroisoquinoline-1-carboxamide (20).
The crude material (reaction time: 48 h) was purified by column chromatography (n-hexane/ethyl acetate 98.5
:
1.5) to give the product as a yellowish amorphous solid (28.4 mg, 68% yield). 1H NMR (700 MHz, CDCl3) δ 7.49–7.48 (m, 3H), 7.45 (d, J = 8.5 Hz, 2H), 7.39 (t, J = 7.7 Hz, 2H), 7.31 (t, J = 7.4 Hz, 1H), 7.30 (s, 1H), 7.28–7.27 (m, 1H), 7.25–7.22 (m, 2H), 7.21–7.18 (m, 4H), 7.12 (t, J = 7.6 Hz, 1H), 6.98 (d, J = 8.5 Hz, 2H), 6.85 (t, J = 7.3 Hz, 1H), 6.79 (d, J = 8.1 Hz, 2H), 6.13 (s, 1H), 3.75–3.71 (m, 1H), 3.60–3.57 (m, 1H), 2.95–2.89 (m, 2H), 2.24 (s, 3H); 13C NMR (176 MHz, CDCl3) δ 176.2, 171.9, 148.1, 140.2, 140.0, 138.3, 138.0, 135.4, 133.8, 133.1, 132.1, 130.5, 129.1, 128.8, 128.7, 128.6 (2C), 127.9, 127.8, 127.7, 127.6, 127.0, 126.8, 126.2, 119.8, 116.2, 61.5, 44.6, 26.9, 21.2; HRMS (ESI) m/z: calcd [M + H]+ for C36H31N2O2+ 523.2381; found [M + H]+ 523.2374.
N-([1,1′-Biphenyl]-4-yl)-N-(2-(4-isobutylphenyl)propanoyl)-2-phenyl-1,2,3,4-tetrahydroisoquinoline-1-carboxamide (21).
The crude material (reaction time: 72 h) was purified by column chromatography (n-hexane/ethyl acetate 99
:
1) to give the product as an off-white solid (29.1 mg, 61% yield; 1
:
0.6 diastereoisomeric mixture). 1H NMR (700 MHz, CDCl3; major diastereomer) δ 7.52–7.50 (m, 2H), 7.45–7.40 (m, 4H), 7.38–7.35 (m, 1H), 7.33–7.31 (m, 2H), 7.28–7.27 (m, 1H), 7.15 (d, J = 7.6 Hz, 2H), 7.01 (d, J = 8.0 Hz, 4H), 6.97–6.94 (m, 1H), 6.89 (d, J = 8.0 Hz, 2H), 6.87 (t, J = 7.3 Hz, 1H), 6.79–6.74 (m, 1H), 6.66–6.63 (m, 1H), 6.53 (m, 1H), 3.80–3.73 (m, 2H), 3.57–3.51 (m, 1H), 3.03–2.99 (m, 1H), 2.95–2.86 (m, 2H), 2.47 (d, J = 7.2 Hz, 2H), 1.92–1.84 (m, 1H), 1.50 (d, J = 6.9 Hz, 3H), 0.92 (d, J = 6.6 Hz, 6H); 13C NMR (176 MHz, CDCl3; major diastereomer) δ 177.5, 177.4; 149.1, 141.7, 140.8, 140.0, 137.4, 137.1, 136.0, 132.5, 129.4, 129.3, 129.2, 128.9, 128.2, 127.9, 127.9, 127.8, 127.7, 127.6, 127.2, 126.3, 118.6, 114.9, 62.4, 45.7, 45.1, 43.5, 30.3, 27.9, 22.4, 19.9; HRMS (ESI) m/z: calcd [M + H]+ for C41H41N2O2+ 593.3163; found [M + H]+ 593.3153; m.p. 150–151 °C.
N-Cyclohexyl-N-(2-(4-isobutylphenyl)propanoyl)-2-phenyl-1,2,3,4-tetrahydroisoquinoline-1-carboxamide (22).
The crude material (reaction time: 72 h) was purified by column chromatography (n-hexane/ethyl acetate 99.5
:
0.5) to give the product as an off-white amorphous solid (40.2 mg, 96% yield; 1
:
1 diastereoisomeric mixture) 1H NMR (700 MHz, CDCl3) δ 7.35–7.34 (m, 1H), 7.31–7.28 (m, 3H), 7.27–7.22 (m, 4H), 7.21–7.18 (m, 1H), 7.16–7.13 (m, 4H), 7.10–7.07 (m, 3H), 7.03–7.02 (m, 4H), 6.99–6.96 (m, 4H), 6.86 (t, J = 7.2 Hz, 1H), 6.81 (t, J = 7.2 Hz, 1H), 6.24–6.23 (m, 2H), 3.86 (q, J = 6.7 Hz, 1H), 3.82 (q, J = 6.8 Hz, 1H), 3.75–3.71 (m, 1H), 3.70–3.67 (m, 1H), 3.64–3.50 (m, 4H), 3.04–3.00 (m, 1H), 2.97–2.85 (m, 3H), 2.45–2.43 (m, 4H), 1.93–1.87 (m, 1H), 1.86–1.80 (m, 2H), 1.75–1.68 (m, 2H), 1.63–1.61 (m, 2H), 1.51 (d, J = 6.8 Hz, 3H), 1.41–1.36 (m, 8H), 1.05–0.99 (m, 2H), 0.95–0.89 (m, 4H), 0.88–0.86 (m, 12H), 0.76–0.74 (m, 2H), 0.64–0.58 (m, 1H), 0.53–0.51 (m, 1H); 13C NMR (176 MHz, CDCl3) δ 178.8, 178.0, 177.7, 149.2, 148.7, 140.7, 140.7, 137.8, 137.6, 135.7, 135.4, 132.9, 132.3, 129.6, 129.5, 129.3, 129.3, 128.4, 128.4, 127.9, 127.8, 127.8, 127.6, 127.4, 127.3, 126.3, 126.3, 119.2, 118.8, 115.7, 115.1, 63.5, 63.5, 58.9, 58.5, 46.7, 46.6, 45.0, 45.0, 30.9, 30.4, 30.2, 29.7, 29.4, 27.8, 27.2, 26.6, 26.6, 26.3, 26.3, 25.0, 22.3, 22.3, 20.5, 20.2; HRMS (ESI) m/z: calcd [M + H]+ for C35H43N2O2+ 523.3320; found [M + H]+ 523.3312.
N-([1,1′-Biphenyl]-4-yl)-N-(3-methylbenzoyl)-1-phenylpyrrolidine-2-carboxamide (23).
The crude material (reaction time: 72 h) was purified by column chromatography (n-hexane/ethyl acetate 98.5
:
1.5) to give the product as an orange amorphous solid (31.9 mg, 87% yield). 1H NMR (700 MHz, CDCl3) δ 7.53–7.51 (m, 4H), 7.42–7.37 (m, 4H), 7.33 (t, J = 7.4 Hz, 1H), 7.24 (t, J = 7.9 Hz, 2H), 7.20–7.19 (m, 3H), 7.12 (t, J = 7.6 Hz, 1H), 6.75 (t, J = 7.3 Hz, 1H), 6.64 (d, J = 8.0 Hz, 2H), 4.86 (dd, J = 8.5, 3.7 Hz, 1H), 3.55–3.52 (m, 1H), 3.41–3.38 (m, 1H), 2.55–2.51 (m, 1H), 2.47–2.41 (m, 1H), 2.27–2.25 (m, 1H), 2.23 (s, 3H), 2.07–2.02 (m, 1H); 13C NMR (176 MHz, CDCl3) δ 178.8, 172.4, 146.8, 140.69, 139.9, 138.2, 137.8, 133.7, 133.3, 130.4, 129.2, 128.8, 128.2, 128.1, 128.0, 127.7, 127.1, 126.8, 117.1, 112.4, 62.7, 49.1, 31.3, 23.7, 21.2; HRMS (ESI) m/z: calcd [M + H]+ for C31H29N2O2+ 461.2224; found [M + H]+ 461.2214.
N-Cyclohexyl-N-(2-(4-isobutylphenyl)propanoyl)-1-phenylpyrrolidine-2-carboxamide (24).
The crude material (reaction time: 72 h) was purified by column chromatography (n-hexane/ethyl acetate 98
:
2) to give the product as a colourless sticky solid (36.1 mg, 98% yield, 1
:
1 diastereoisomeric mixture). 1H NMR (700 MHz, CDCl3) δ 7.28 (d, J = 8.0 Hz, 2H), 7.21–7.18 (m, 6H), 7.13 (d, J = 8.0 Hz, 2H), 7.01 (t, J = 7.9 Hz, 2H), 6.67 (t, J = 7.3 Hz, 1H), 6.60 (t, J = 7.3 Hz, 1H), 6.55 (d, J = 8.0 Hz, 2H), 6.29 (d, J = 8.0 Hz, 2H), 4.90 (m, 1H), 4.61 (dd, J = 8.7, 2.0 Hz, 1H), 4.16 (q, J = 6.7 Hz, 1H), 4.05 (q, J = 6.8 Hz, 1H), 3.82–3.78 (m, 1H), 3.76–3.72 (m, 1H), 3.59–3.54 (m, 2H), 3.43–3.37 (m, 2H), 2.52–2.50 (m, 2H), 2.47 (d, J = 7.2 Hz, 2H), 2.44–2.38 (m, 1H), 2.31–2.13 (m, 6H), 2.05–1.83 (m, 8H), 1.81–1.76 (m, 2H), 1.70–1.66 (m, 3H), 1.54–1.52 (m, 9H), 1.23–1.14 (m, 2H), 1.07–0.99 (m, 2H), 0.97–0.90 (m, 9H), 0.88 (d, J = 6.6 Hz, 6H); 13C NMR (176 MHz, CDCl3) δ 180.3, 179.2, 179.1, 179.0, 146.6, 146.3, 141.2, 140.9, 137.8, 137.7, 130.0, 129.7, 129.1, 129.0, 127.5, 127.3, 116.3, 116.1, 112.0, 111.8, 63.2, 62.9, 58.8, 58.6, 48.8, 48.4, 47.2, 46.9, 45.1, 45.0, 31.8, 31.3, 31.0, 30.5, 30.3, 30.2, 29.4, 28.4, 26.9, 26.8, 26.5, 26.5, 25.0, 23.2, 23.1, 22.4, 22.2, 20.5, 20.1; HRMS (ESI) m/z: calcd [M + H]+ for C30H41N2O2+ 461.3163; found [M + H]+ 461.3153.
N-([1,1′-Biphenyl]-4-yl)-N-(3-methylbenzoyl)-1-phenylpiperidine-2-carboxamide (25).
The crude material (reaction time: 48 h) was purified by column chromatography (n-hexane/ethyl acetate 98
:
2) to give the product as a yellowish amorphous solid (24.0 mg, 63% yield). 1H NMR (700 MHz, CDCl3) δ 7.51–7.50 (m, 2H), 7.49–7.48 (m, 2H), 7.40 (t, J = 7.7 Hz, 2H), 7.33 (t, J = 7.4 Hz, 1H), 7.28 (s, 1H), 7.24–7.21 (m, 3H), 7.18 (d, J = 7.5 Hz, 1H), 7.11 (t, J = 7.6 Hz, 1H), 6.98–6.96 (m, 2H), 6.88 (t, J = 7.3 Hz, 1H), 6.85 (d, J = 8.2 Hz, 2H), 5.10–5.09 (m, 1H), 3.46–3.42 (m, 1H), 3.37–3.35 (m, 1H), 2.37–2.35 (m, 1H), 2.23 (s, 3H), 1.99–1.94 (m, 1H), 1.87–1.82 (m, 1H), 1.80–1.78 (m, 1H), 1.75–1.72 (m, 1H), 1.64–1.57 (m, 1H); 13C NMR (176 MHz, CDCl3) δ 176.8, 172.0, 149.6, 140.5, 140.0, 138.2, 138.1, 134.0, 133.0, 130.2, 129.1, 128.8, 128.0, 128.0, 127.9, 127.6, 127.0, 126.6, 119.9, 116.7, 58.2, 45.8, 25.8, 24.6, 21.2, 19.9; HRMS (ESI) m/z: calcd [M + H]+ for C32H31N2O2+ 475.2381; found [M + H]+ 475.2396.
General procedure for the synthesis of compounds 9 and 11
To a 4 mL colourless screw-cap glass vial equipped with a magnetic stir bar isocyanide (0.08 mmol), carboxylic acid (0.16 mmol, 2 equiv.), the aniline derivative (0.16 mmol, 2 equiv.) and 800 μL of a 9
:
1 mixture of MeCN and H2O (0.1 M) were added. The resulting mixture was stirred open flask in a PhotoRedOx Box (EvoluChem™), under 30 W blue LED irradiation, at room temperature, for 72 h. After the completion of the reaction, as monitored by TLC, the solvent was removed under vacuum and the crude mixture was purified by silica gel chromatography.
N-Cyclohexyl-N-(N-methyl-N-phenylglycyl)-5-((3aS,4S,6aR)-2-oxohexahydro-1H-thieno[3,4-d]imidazol-4-yl)pentanamide (9).
The crude material was purified by column chromatography (dichloromethane/methanol 98
:
2) to give the product as an off-white amorphous solid (20.0 mg, 53% yield). 1H NMR (700 MHz, CDCl3) δ 7.21 (t, J = 7.9 Hz, 2H), 6.72 (t, J = 7.2 Hz, 1H), 6.64 (d, J = 8.2 Hz, 2H), 5.88 (br s, –NH), 5.37 (br s, –NH), 4.49–4.47 (m, 1H), 4.35 (s, 2H), 4.29–4.28 (m, 1H), 3.68–3.65 (m, 1H), 3.16–3.13 (m, 1H), 3.01 (s, 3H), 2.89 (dd, J = 12.8, 5.0 Hz, 1H), 2.72 (d, J = 12.8 Hz, 1H), 2.54 (t, J = 7.4 Hz, 2H), 2.18–2.12 (m, 2H), 1.82–1.80 (m, 2H), 1.76–1.65 (m, 4H), 1.64–1.60 (m, 3H), 1.51–1.42 (m, 2H), 1.22–1.20 (m, 2H), 1.16–1.10 (m, 1H); 13C NMR (176 MHz, CDCl3) δ 177.3, 175.4, 163.7, 148.9, 129.2, 117.2, 112.2, 62.0, 60.1, 59.3, 58.7, 55.4, 40.6, 39.8, 37.3, 30.2, 28.5, 28.4, 26.6, 25.0, 24.9; HRMS (ESI) m/z: calcd [M + H]+ for C25H37N4O3S+ 473.2581; found [M + H]+ 473.2584.
(R)-N-Cyclohexyl-N-(N-methyl-N-phenylglycyl)-4-((3R,5S,7R,8R,9S,10S,12S,13R,14S,17R)-3,7,12-trihydroxy-10,13-dimethylhexadecahydro-1H-cyclopenta[a]phenanthren-17-yl)pentanamide (11).
The crude material was purified by column chromatography (dichloromethane/methanol 97
:
3) to give the product as an off-white amorphous solid (41.0 mg, 80% yield). 1H NMR (700 MHz, CDCl3) δ 7.20 (t, J = 7.9 Hz, 2H), 6.71 (t, J = 7.2 Hz, 1H), 6.63 (d, J = 8.3 Hz, 2H), 4.35 (s, 2H), 3.96 (s, 1H), 3.85 (s, 1H), 3.68–3.65 (m, 1H), 3.48–3.43 (m, 1H), 3.01 (s, 3H), 2.59–2.55 (m, 2H), 2.46–2.41 (m, 1H), 2.27–2.13 (m, 4H), 1.95–1.88 (m, 3H), 1.83–1.78 (m, 5H), 1.76–1.66 (m, 4H), 1.64–1.60 (m, 4H), 1.56–1.50 (m, 3H), 1.45–1.39 (m, 4H), 1.33–1.27 (m, 2H), 1.23–1.20 (m, 2H), 1.16–1.09 (m, 2H), 1.01 (d, J = 6.1 Hz, 3H), 1.00–0.95 (m, 1H), 0.89 (s, 3H), 0.68 (s, 3H); 13C NMR (176 MHz, CDCl3) δ 178.2, 175.5, 148.9, 129.1, 117.1, 112.1, 73.1, 71.9, 68.5, 59.4, 58.8, 47.1, 46.5, 41.7, 41.5, 39.8, 39.6, 39.5, 35.4, 35.3, 34.8, 34.7, 34.7, 31.2, 30.4, 30.2, 30.2, 29.7, 28.2, 27.6, 26.7, 26.4, 25.1, 23.3, 22.5, 17.6, 12.5; HRMS (ESI) m/z: calcd [M + H]+ for C39H61N2O5+ 637.4575; found [M + H]+ 637.4570.
N
1,N4-Dicyclohexyl-N1,N4-bis(N-methyl-N-phenylglycyl)terephthalamide (12).
To a 4 mL colourless screw-cap glass vial equipped with a magnetic stir bar, terephthalic acid (13.3 mg, 0.08 mmol), cyclohexyl isocyanide (19.9 μL, 0.16 mmol, 2 equiv.), N,N-dimethylaniline (30.4 μL, 0.24 mmol, 3 equiv.) and 800 μL of dry MeCN (0.1 M) were added; 80 mg of 3 Å activated molecular sieves were then added to the resulting mixture, which was stirred open flask in a PhotoRedOx Box (EvoluChem™), under 30 W blue LED irradiation, at room temperature, for 72 h. After the completion of the reaction, as monitored by TLC, the solvent was removed under vacuum and the crude mixture was purified by silica gel chromatography (n-hexane/ethyl acetate 97
:
3), to give the product as a yellow amorphous solid (36.4 mg, 73% yield). 1H NMR (700 MHz, CDCl3) δ 7.32 (s, 4H), 7.12 (t, J = 7.9 Hz, 4H), 6.80 (t, J = 7.2 Hz, 2H), 6.40 (d, J = 8.2 Hz, 4H), 3.96–3.93 (m, 2H), 3.81 (s, 4H), 2.55 (s, 6H), 1.90–1.84 (m, 4H), 1.78–1.76 (m, 4H), 1.70–1.68 (m, 4H), 1.60–1.58 (m, 2H), 1.24–1.19 (m, 4H), 1.13–1.08 (m, 2H); 13C NMR (176 MHz, CDCl3) δ 173.7, 171.6, 148.0, 137.9, 129.0, 128.6, 119.4, 113.6, 58.3, 58.3, 40.0, 30.0, 26.3, 25.2; HRMS (ESI) m/z: calcd [M + H]+ for C38H47N4O4+ 623.3592; found [M + H]+ 623.3578.
General procedure for the preparation of compounds 14–19
To a 4 mL colourless screw-cap glass vial equipped with a magnetic stir bar the aniline derivative (0.3 mmol), isocyanide (0.6 mmol, 2 equiv.), azidotrimethylsilane (0.6 mmol, 2 equiv.), and 2.0 mL of dry MeCN (0.15 M) were added. The resulting mixture was stirred open flask in a PhotoRedOx Box (EvoluChem™), under 30 W blue LED irradiation, at room temperature, for 48 h. After the completion of the reaction, as monitored by TLC, the solvent was removed under vacuum and the crude mixture was purified by silica gel chromatography.
N-((1-Cyclohexyl-1H-tetrazol-5-yl)methyl)-N-methylaniline (14).
The crude material was purified by column chromatography (n-hexane/ethyl acetate 92
:
8) to give the product as a beige solid (70.2 mg, 86% yield). 1H NMR (700 MHz, CDCl3) δ 7.29–7.27 (m, 2H), 6.89–6.86 (m, 3H), 4.75 (s, 2H), 4.20–4.16 (m, 1H), 2.88 (s, 3H), 1.95–1.90 (m, 2H), 1.86–1.81 (m, 4H), 1.68–1.66 (m, 1H), 1.28–1.15 (m, 3H); 13C NMR (176 MHz, CDCl3) δ 151.3, 149.2, 129.5, 119.6, 114.8, 58.3, 46.6, 38.9, 32.9, 25.3, 24.7; HRMS (ESI) m/z: calcd [M + H]+ for C15H22N5+ 272.1870; found [M + H]+ 272.1866; m.p. 120–121 °C.
N-((1-(tert-Butyl)-1H-tetrazol-5-yl)methyl)-N-methylaniline (16).
The crude material was purified by column chromatography (n-hexane/ethyl acetate 95
:
5) to give the product as an off-white solid (66.0 mg, 90% yield). 1H NMR (700 MHz, CDCl3) δ 7.29–7.27 (m, 2H), 6.87–6.85 (m, 3H), 4.76 (s, 2H), 2.92 (s, 3H), 1.74 (s, 9H); 13C NMR (176 MHz, CDCl3) δ 151.7, 149.4, 129.4, 119.3, 114.7, 61.5, 47.7, 39.4, 29.4; HRMS (ESI) m/z: calcd [M + H]+ for C13H20N5+ 246.1714; found [M + H]+ 247.1724; m.p. 116–117 °C.
N-((1-([1,1′-Biphenyl]-4-yl)-1H-tetrazol-5-yl)methyl)-N-methylaniline (17).
The crude material was purified by column chromatography (n-hexane/ethyl acetate 96
:
4) to give the product as a brownish solid (26.2 mg, 96% yield; reaction performed on a 0.08 mmol scale). 1H NMR (700 MHz, CDCl3) δ 7.71 (d, J = 8.4 Hz, 2H), 7.61 (d, J = 7.2 Hz, 2H), 7.51 (t, J = 7.7 Hz, 2H), 7.45–7.43 (m, 3H), 7.16–7.14 (m, 2H), 6.78 (t, J = 7.3 Hz, 1H), 6.63 (d, J = 8.3 Hz, 2H), 4.79 (s, 2H), 2.86 (s, 3H); 13C NMR (176 MHz, CDCl3) δ 153.0, 148.4, 143.7, 139.2, 132.7, 129.3, 129.1, 128.4, 128.3, 127.3, 125.6, 118.9, 114.1, 46.6, 39.1; HRMS (ESI) m/z: calcd [M + H]+ for C21H20N5+ 342.1714; found [M + H]+ 342.1706; m.p. 147–148 °C.
4-Bromo-N-((1-cyclohexyl-1H-tetrazol-5-yl)methyl)-N-methylaniline (18).
The crude material was purified by column chromatography (n-hexane/ethyl acetate 9
:
1) to give the product as a white solid (52.5 mg, 50% yield; reaction performed with 3 equiv. of cyclohexyl isocyanide and azidotrimethylsilane). 1H NMR (700 MHz, CDCl3) δ 7.37–7.35 (m, 2H), 6.76–6.74 (m, 2H), 4.72 (s, 2H), 4.16–4.12 (m, 1H), 2.90 (s, 3H), 1.99–1.93 (m, 2H), 1.90–1.88 (m, 2H), 1.85–1.83 (m, 2H), 1.72–1.70 (m, 1H), 1.31–1.19 (m, 3H); 13C NMR (176 MHz, CDCl3) δ 151.0, 148.1, 132.2, 116.1, 111.6, 58.4, 46.5, 39.1, 32.9, 25.3, 24.7; HRMS (ESI) m/z: calcd [M + H]+ for C15H21BrN5+ 350.0975; found [M + H]+ 350.0987; m.p. 134–135 °C.
N-((1-Cyclohexyl-1H-tetrazol-5-yl)methyl)-4-methoxy-N-methylaniline (19).
The crude material was purified by column chromatography (n-hexane/ethyl acetate 85
:
15) to give the product as a brownish solid (51.2 mg, 57% yield). 1H NMR (700 MHz, CDCl3) δ 6.89 (d, J = 8.7 Hz, 2H), 6.85 (d, J = 8.8 Hz, 2H), 4.59 (s, 2H), 4.25–4.22 (m, 1H), 3.77 (s, 3H), 2.78 (s, 3H), 1.97–1.92 (m, 2H), 1.89–1.87 (m, 2H), 1.84–1.82 (m, 2H), 1.69 (m, 1H), 0.89–0.87 (m, 3H); 13C NMR (176 MHz, CDCl3) δ 154.2, 151.1, 143.9, 118.3, 114.8, 58.4, 55.6, 48.2, 40.4, 32.9, 25.4, 24.8; HRMS (ESI) m/z: calcd [M + H]+ for C16H24N5O+ 302.1975; found [M + H]+ 30 2.1984; m.p. 125–126 °C.
General procedure for the preparation of compounds 28 and 29
To a 4 mL colourless screw-cap glass vial equipped with a magnetic stir bar, 2,4-dimethoxybenzyl isocyanide (14.2 mg, 0.08 mmol), carboxylic acid (0.12 mmol, 1.5 equiv.), the aniline derivative (0.16 mmol, 2 equiv.) and 800 μL of dry MeCN (0.1 M) were added; 80 mg of 3 Å activated molecular sieves were then added to the resulting mixture, which was stirred open flask in a PhotoRedOx Box (EvoluChem™), under 30 W blue LED irradiation, at room temperature, for 48 h. After the completion of the reaction, as monitored by TLC, the crude mixture was transferred into a 10 ml round bottom flask and the solvent was removed under vacuum; a 1
:
1 DCM/TFA mixture (0.1 M, 400 μL of dry DCM and 400 μL of TFA) was then added at 0 °C, and the resulting mixture was stirred at 50° C for 4 h. After the completion of the reaction, as monitored by TLC, the solvent was removed under vacuum, by adding n-hexane several times in order to remove the residual TFA, and the crude mixture was purified by silica gel chromatography.
3-Methyl-N-(N-methyl-N-phenylglycyl)benzamide (28).
The crude material was purified by column chromatography (n-hexane/ethyl acetate 96
:
4) to give the product as a colourless sticky solid (9.1 mg, 40% yield). 1H NMR (700 MHz, CDCl3) δ 9.14 (br s, –NH), 7.54 (s, 1H), 7.51 (d, J = 7.7 Hz, 1H), 7.39 (d, J = 7.6 Hz, 1H), 7.33 (t, J = 7.6 Hz, 1H), 7.30–7.27 (m, 2H), 6.83 (t, J = 7.3 Hz, 1H), 6.78 (d, J = 8.1 Hz, 2H), 4.47 (s, 2H), 3.11 (s, 3H), 2.38 (s, 3H); 13C NMR (176 MHz, CDCl3) δ 171.8, 165.4, 148.8, 139.1, 134.1, 132.5, 129.5, 128.9, 128.4, 124.7, 118.4, 112.9, 58.7, 39.8, 21.3; HRMS (ESI) m/z: calcd [M + H]+ for C17H19N2O2+ 283.1442; found [M + H]+ 283.1434.
N-(N-(3,5-Dimethylphenyl)-N-methylglycyl)-3-methylbenzamide (29).
The crude material was purified by column chromatography (n-hexane/ethyl acetate 94
:
6) to give the product as a pinkish solid (9.0 mg, 36% yield). 1H NMR (700 MHz, CDCl3) δ 9.26 (br s, –NH), 7.51 (s, 1H), 7.49 (d, J = 7.8 Hz, 1H), 7.39 (d, J = 7.5 Hz, 1H), 7.32 (t, J = 7.6 Hz, 1H), 6.51 (s, 1H), 6.42 (s, 2H), 4.37 (s, 2H), 3.07 (s, 3H), 2.37 (s, 3H), 2.28 (s, 6H); 13C NMR (176 MHz, CDCl3) δ 171.5, 165.3, 148.9, 139.2, 139.0, 134.0, 132.6, 128.9, 128.4, 124.7, 120.7, 111.1, 58.9, 40.00, 21.8, 21.3; HRMS (ESI) m/z: calcd [M + H]+ for C19H23N2O2+ 311.1754; found [M + H]+ 311.1746; m.p. 113–114 °C.
General procedure for the preparation of compounds 32–34
To a 4 mL colourless screw-cap glass vial equipped with a magnetic stir bar, tert-butyl (2-isocyanobenzyl)carbamate (18.7 mg, 0.08 mmol), carboxylic acid (0.12 mmol, 1.5 equiv.), the aniline derivative (0.16 mmol, 2 equiv.) and 800 μL of dry MeCN (0.1 M) were added; 80 mg of 3 Å activated molecular sieves were then added to the resulting mixture, which was stirred open flask in a PhotoRedOx Box (EvoluChem™), under 30 W blue LED irradiation, at room temperature, for 48–72 h. After the completion of the reaction, as monitored by TLC, the crude mixture was transferred into a 10 ml round bottom flask and the solvent was removed under vacuum; a 3
:
1 DCM/TFA mixture (0.1 M, 600 μL of dry DCM and 200 μL of TFA) was then added at 0° C, and the resulting mixture was stirred at room temperature for 1 h until the completion of the reaction, as monitored by TLC. After evaporating TFA under a nitrogen positive flow, a 3
:
1 DCM/Et3N mixture (0.1 M, 600 μL of dry DCM and 200 μL of Et3N) was added dropwise at 0 °C, and the resulting mixture was stirred at room temperature for 1 h until the completion of the reaction, as monitored by TLC. Then the solvent was removed under vacuum and the crude mixture was purified by silica gel chromatography.
3-Methyl-N-(2-(2-(methyl(phenyl)amino)acetamido)benzyl)benzamide (32).
The crude material was purified by column chromatography (n-hexane/ethyl acetate 75
:
25) to give the product as a pale-yellow amorphous solid (15.3 mg, 49% yield; 1
:
0.9 mixture of rotamers). 1H NMR (700 MHz, CDCl3; major rotamer) δ 9.55 (br s, –NH), 7.79 (d, J = 8.0 Hz, 1H), 7.50 (s, 1H), 7.46 (d, J = 7.4 Hz, 1H), 7.32–7.26 (m, 1H), 7.24–7.20 (m, 4H), 7.16–7.10 (m, 2H), 6.78–6.77 (m, 3H), 6.73 (br t, –NH), 4.34 (d, J = 5.9 Hz, 2H), 4.09 (s, 2H), 3.18 (s, 3H), 2.36 (s, 3H); 13C NMR (176 MHz, CDCl3; major rotamer) δ 171.8, 166.6, 149.1, 138.4, 136.5, 134.7, 132.5, 130.4, 129.6, 129.5, 128.9, 128.7, 128.5, 125.5, 124.6, 124.0, 118.3, 113.3, 58.9, 40.7, 39.9, 21.5; HRMS (ESI) m/z: calcd [M + H]+ for C24H26N3O2+ 388.2020; found [M + H]+ 388.2012.
N-(2-(2-((3,5-Dimethylphenyl)(methyl)amino)acetamido)benzyl)-3-methylbenzamide (33).
The crude material was purified by column chromatography (n-hexane/ethyl acetate 87
:
13) to give the product as a beige solid (22.9 mg, 69% yield; 1
:
0.6 mixture of rotamers). 1H NMR (700 MHz, CDCl3; major rotamer) δ 9.35 (br s, –NH), 7.73 (d, J = 8.0 Hz, 1H), 7.51 (s, 1H), 7.45 (d, J = 7.4 Hz, 1H), 7.37–7.31 (m, 4H), 7.17–7.15 (m, 1H), 6.77 (br t, –NH), 6.45 (s, 1H), 6.41 (s, 2H), 4.40 (d, J = 5.7 Hz, 2H), 4.04 (s, 2H), 3.13 (s, 3H), 2.36 (s, 3H), 2.23 (s, 6H); 13C NMR (176 MHz, CDCl3; major rotamer) δ 170.5, 167.7, 149.3, 138.9, 138.4, 135.6, 133.7, 132.4, 130.8, 130.7, 128.9, 128.4, 127.7, 126.0, 124.7, 124.0, 120.6, 111.2, 59.2, 40.8, 40.4, 21.7, 21.3; HRMS (ESI) m/z: calcd [M + H]+ for C26H30N3O2+ 416.2333; found [M + H]+ 416.2325; m.p. 64–65 °C.
N-(2-(2-((4-Bromophenyl)(methyl)amino)acetamido)benzyl)-3-methylbenzamide (34).
The crude material was purified by column chromatography (n-hexane/ethyl acetate 80
:
20) to give the product as an off-white amorphous solid (12.8 mg, 34% yield; 1
:
0.6 mixture of rotamers). 1H NMR (700 MHz, CDCl3; major rotamer) δ 9.86 (br s, –NH), 7.46 (s, 1H), 7.44 (d, J = 7.6 Hz, 1H), 7.37 (d, J = 7.2 Hz, 1H), 7.36–7.65 (m, 4H), 7.24–7.22 (m, 2H), 7.14–7.11 (m, 1H), 6.66 (br t, J = 5.6 Hz, –NH), 6.61–6.60 (m, 2H), 4.33 (d, J = 6.1 Hz, 2H), 4.10 (s, 2H), 3.18 (s, 3H), 2.37 (s, 3H); 13C NMR (176 MHz, CDCl3) δ 169.5, 167.9, 148.1, 138.5, 135.9, 133.2, 132.7, 131.8, 130.7, 129.2, 129.0, 128.5, 127.7, 125.4, 124.5, 124.0, 114.5, 111.0, 58.6, 40.8, 40.4, 21.4; HRMS (ESI) m/z: calcd [M + H]+ for C24H25BrN3O2+ 466.1125; found [M + H]+ 466.1122.
Conflicts of interest
There are no conflicts to declare.
Acknowledgements
Financial support from Università degli Studi di Napoli “Federico II” and Università del Piemonte Orientale, Novara, Italy, is acknowledged. R. C. acknowledges MIUR-Ministero dell'Istruzione, dell'Università e della Ricerca (Italian Ministry of Education, University and Research), PON R&I 2014-2020-AIM (Attraction and International Mobility), project AIM1873131 – Num. Attività 2 – Linea 2.1.
References
-
P. T. Anastas and J. C. Warner, Green Chemistry: Theory and Practice, Oxford University Press Inc, 2000 Search PubMed.
-
I. Ugi, Isonitrile Chemistry, Academic Press, New York-London, 1971 Search PubMed.
-
I. Ugi and C. Steinbrueckner, 1103337B, p. 1959.
-
(a) M. Passerini, Gazz. Chim. Ital., 1920, 50, 340–344 CAS;
(b) M. Passerini, Gazz. Chim. Ital., 1921, 51, 126–129 CAS.
- A. Dömling and I. Ugi, Multicomponent Reactions with Isocyanides, Angew. Chem., Int. Ed., 2000, 39, 3168–3210 CrossRef.
-
(a) A. Dömling, W. Wang and K. Wang, Chem. Rev., 2012, 112, 3083–3135 CrossRef PubMed;
(b) M. Giustiniano, A. Basso, V. Mercalli, A. Massarotti, E. Novellino, G. C. Tron and J. Zhu, Chem. Soc. Rev., 2017, 46, 1295–1357 RSC.
-
(a) U. K. Sharma, N. Sharma, D. D. Vachhani and E. V. Van Der Eycken, Chem. Soc. Rev., 2015, 44, 1836–1860 RSC;
(b) M. Giustiniano, L. Moni, L. Sangaletti, S. Pelliccia, A. Basso, E. Novellino and G. C. Tron, Synthesis, 2018, 3549–3570 CrossRef CAS.
- C. Russo, J. Amato, G. C. Tron and M. Giustiniano, J. Org. Chem., 2021, 86, 18117–18127 CrossRef CAS.
-
(a) M. Rueping and C. Vila, Org. Lett., 2013, 15, 2092–2095 CrossRef CAS PubMed;
(b) C. Vila and M. Rueping, Green Chem., 2013, 15, 2056–2059 RSC;
(c) M. Rueping, C. Vila and T. Bootwicha, ACS Catal., 2013, 3, 1676–1680 CrossRef CAS.
-
(a) M. H. Shaw, J. Twilton and D. W. C. MacMillan, J. Org. Chem., 2016, 81, 6898–6926 CrossRef CAS PubMed;
(b) N. A. Romero and D. A. Nicewicz, Chem. Rev., 2016, 116, 10075–10166 CrossRef CAS PubMed.
-
(a) M. Zhang, Q.-Y. Fu, G. Gao, H.-Y. He, Y. Zhang, Y.-S. Wu and Z.-H. Zhang, ACS Sustainable Chem. Eng., 2017, 5, 6175–6182 CrossRef CAS;
(b) M. Zhang, M.-N. Chen and Z.-H. Zhang, Adv. Synth. Catal., 2019, 361, 5182–5190 CrossRef CAS;
(c) G. A. Coppola, S. Pillitteri, E. V. Van der Eycken, S.-L. You and U. K. Sharma, Chem. Soc. Rev., 2022, 51, 2313–2382 RSC.
-
(a) C. G. Neochoritis, T. Zhao and A. Dömling, Chem. Rev., 2019, 119, 1970–2042 CrossRef CAS;
(b) E. W. Badder, Moderne Ergebnisse Der Silikose Forschung, Angew. Chem., 1959, 71, 373–388 Search PubMed.
-
(a) R. F. Nutt and M. M. Joullié, J. Am. Chem. Soc., 1982, 104, 5852–5853 CrossRef CAS;
(b) D. M. Flanagan and M. M. Joullié, Synth. Commun., 1989, 19, 1–12 CrossRef CAS;
(c) G. C. Tron, Eur. J. Org. Chem., 2013, 1849–1859 CrossRef CAS;
(d) R. Mossetti, T. Pirali, D. Saggiorato and G. C. Tron, Chem. Commun., 2011, 47, 6966–6968 RSC.
- S. Gazzotti, G. Rainoldi and A. Silvani, Expert Opin. Drug Discovery, 2019, 14, 639–652 CrossRef CAS PubMed.
-
(a) X. Tian, C. Cassani, Y. Liu, A. Moran, A. Urakawa, P. Galzerano, E. Arceo and P. Melchiorre, J. Am. Chem. Soc., 2011, 133, 17934–17941 CrossRef CAS;
(b) X. Li, M. Lu, Y. Dong, W. Wu, Q. Qian, J. Ye and D. J. Dixon, Nat. Commun., 2014, 5, 4479–4487 CrossRef CAS PubMed;
(c) C. Verrier and P. Melchiorre, Chem. Sci., 2015, 6, 4242–4246 RSC;
(d) A. Katsuyama, A. Matsuda and S. Ichikawa, Org. Lett., 2016, 18, 2552–2555 CrossRef CAS.
- L. Moni, F. De Moliner, S. Garbarino, J. Saupe, C. Mang and A. Basso, Front. Chem., 2018, 6, 369–382 CrossRef PubMed.
- L. Banfi, G. Guanti and R. Riva, Chem. Commun., 2000, 985–986 RSC.
- G. B. Giovenzana, G. C. Tron, S. Di Paola, I. G. Menegotto and T. Pirali, Angew. Chem., Int. Ed., 2006, 45, 1099–1102 CrossRef CAS PubMed.
- C. Sivaraj and T. Gandhi, Chem. – Asian J., 2021, 16, 2773–2794 CrossRef CAS PubMed.
- T. Brandhofer, A. Gini, S. Stockerl, D. G. Piekarski and O. García Mancheño, J. Org. Chem., 2019, 84, 12992–13002 CrossRef CAS.
- J. Wang, Y. Sun, G. Wang and L. Zhen, Eur. J. Org. Chem., 2017, 6338–6348 CrossRef CAS.
- Y. Chena and G. Feng, Org. Biomol. Chem., 2015, 13, 4260–4265 RSC.
- L. A. Ho, C. L. Raston and K. A. Stubbs, Chem. – Eur. J., 2018, 24, 8869–8874 CrossRef CAS.
|
This journal is © The Royal Society of Chemistry 2022 |