DOI:
10.1039/D2FO01358D
(Paper)
Food Funct., 2022,
13, 8399-8410
The influence of a tomato food matrix on the bioavailability and plasma kinetics of oral gamma-aminobutyric acid (GABA) and its precursor glutamate in healthy men†
Received
17th May 2022
, Accepted 3rd July 2022
First published on 4th July 2022
Abstract
Gamma-aminobutyric acid (GABA) and its precursor glutamate play signaling roles in a range of tissues. Both function as neurotransmitters in the central nervous system, but they also modulate pancreatic and immune functioning, for example. Besides endogenous production, both compounds are found in food products, reaching relatively high levels in tomatoes. Recent studies in rodents suggest beneficial effects of oral GABA on glucose homeostasis and blood pressure. However, the bioavailability from food remains unknown. We studied the bioavailability of GABA and glutamate from tomatoes relative to a solution in water. After a fasting blood sample was taken, eleven healthy men randomly received 1 liter of 4 different drinks in a cross-over design with a one-week interval. The drinks were a solution of 888 mg L−1 GABA, a solution of 3673 mg L−1 glutamate, pureed fresh tomatoes and plain water as the control. Following intake, 18 blood samples were taken at intervals for 24 hours. Plasma GABA and glutamate concentrations were determined by ultra-pressure liquid chromatography coupled with tandem mass spectrometry (UPLC-MS/MS). Fasting plasma GABA and glutamate concentrations were found to be 16.71 (SD 2.18) ng mL−1 and 4626 (SD 1666) ng mL−1, respectively. Fasting GABA levels were constant (5.8 CV%) between individuals, while fasting glutamate levels varied considerably (23.5 CV%). GABA from pureed tomatoes showed similar bioavailability to that of a solution in water. For glutamate, the absorption from pureed tomatoes occurred more slowly as seen from a longer tmax (0.98 ± 0.14 h vs. 0.41 ± 0.04 h, P = 0.003) and lower Cmax (7815 ± 627 ng mL−1vs. 16
420 ± 2778 ng mL−1, P = 0.006). These data suggest that GABA is bioavailable from tomatoes, and that food products containing GABA could potentially induce health effects similar to those claimed for GABA supplements. The results merit further studies on the bioavailability of GABA from other food products and the health effects of GABA-rich diets. The clinical trial registry number is NCT04086108 (https://clinicaltrials.gov/ct2/show/NCT04303468).
1. Introduction
The amino acid gamma-aminobutyric acid (GABA) and its precursor glutamate are well known as primary inhibitory and excitatory neurotransmitters in the central nervous system, respectively.1 In addition to effects on the central nervous system, GABA receptors have been identified on peripheral cells of the immune system and the pancreas.2–5 Therefore, it has been hypothesized that GABA may also have a role in, for example, the metabolic regulation of glucose homeostasis.6 This has sparked interest in the potential beneficial effects of GABA supplements, and several rodent studies have indeed shown promising health effects of oral GABA intake. For example, GABA supplementation was found to improve diabetic symptoms (e.g. lower blood glucose levels and improved insulin sensitivity) in animal models for both type 1 and type 2 diabetes, and to lower blood pressure.7–11 Clinical trials studying oral supplementation of GABA in humans are still scarce and focus mainly on the effects on mental processes.12,13
GABA is primarily known as a neurotransmitter that is endogenously formed. Endogenous GABA is synthesized from glutamate via the enzymatic activity of glutamic acid decarboxylase in the brain, pancreas, liver, and it is produced by the gut microbiota.14 The GABA-precursor glutamate is produced from glutamine, a major dietary amino acid, via the action of glutaminase in the liver, brain and kidneys.15 In addition to the endogenous synthesis route, GABA and glutamate are also commonly present in the diet. In particular, fermented foods and several fruits and vegetables are rich sources of GABA.16 Tomatoes can be considered to be a relevant source since they are rich in GABA, and consumption rates are high in many cultures.17 Tomatoes are not only rich in GABA but they also contain high amounts of glutamate, the natural precursor for GABA. Previous studies have demonstrated that oral GABA supplementation leads to transient peaks in circulating GABA concentrations, suggesting that oral GABA can be absorbed by the GI tract.18 However, whether GABA from a food matrix is also absorbed and can increase plasma concentrations has not been investigated. To date, it is also unknown whether the GABA-precursor glutamate, which is also present in high concentrations in tomatoes, can affect circulating GABA concentrations.
The potential health benefits of GABA in combination with its abundance in food sources have given rise to the speculation that GABA-rich food products like tomatoes may improve metabolic health.19 To design intervention studies to investigate this will require an insight into the absorption of GABA from a food matrix compared to its absorption from supplements. In view of this, a four-way crossover study was undertaken to investigate the relative oral bioavailability of GABA and its precursor glutamate from tomatoes compared to that from a solution in water in healthy volunteers.
2. Materials and methods
2.1 Sample size and participant characteristics
The study was carried out according to the guidelines laid out in the Declaration of Helsinki and approved by the medical ethics committee of Wageningen University (METC-WU, 19/13). The study is registered at clinicaltrials.gov (NCT04086108). All participants signed a written informed consent before participating in the study. This is the first study to assess the relative bioavailability of GABA and glutamate from a food matrix; therefore, sample size calculation was based on a published plasma kinetics study18 and justified by the well-controlled nature of the current study. Sample size was fixed to be 10 participants, and two additional participants were included to account for possible dropouts. We included a total of 12 healthy male participants. The exclusion criteria included suffering from any disease, any gastrointestinal disorder within 3 months prior to the intervention, recent medication or supplement use, recent substantial change in weight, adherence to a specific diet (e.g., vegetarian), using recreational drugs more than once a month, smoking and excessive alcohol consumption (>10 standardized glasses a week). Whether the participants met these criteria was assessed using a self-reported questionnaire. In addition, whether hemoglobin levels were lower than 8.5 mmol L−1 was measured by a finger prick (HemoCue Hb 201, Ängelholm, Sweden) during screening in the month before the study at the Human Nutrition Research Unit of Wageningen University and Research. A total of 11 participants completed all 4 test days. One of the participants withdrew from participation for reasons that were not related to the study after the first 2 test days, and therefore his data were excluded from further analysis. More details are shown in a CONSORT flow diagram (ESI 1†). Participants had a mean age of 23.6 (SD 2.4) years and mean BMI of 22.5 (SD 1.8) kg m−2.
2.2 Study design
We conducted a randomized four-way crossover study from November to December 2019 at the Human Nutrition Research Unit of Wageningen University and Research. We assessed the relative bioavailability of GABA and glutamate from pureed tomatoes compared to that from solutions prepared in water. The study consisted of four test days for each participant with at least a one-week washout in between. In a random order, participants received one of the four test products on each test day. These test products were a GABA solution in water, a glutamate solution in water, pureed tomatoes or water as a control (section 2.3).
The day before each test day, participants consumed standardized meals that did not include products with a high GABA content. In addition, they did not eat or drink anything other than water from 22:00 onwards. On the test days, participants arrived in a fasted state at the research facility. After a venous catheter was placed and a baseline blood sample drawn, they received the test product. Participants were instructed to drink the allocated test product in exactly 15 minutes. After that, blood samples were taken at t = 15, 30 and 45 minutes and 1, 1.5, 2, 2.5, 3, 3.5, 4, 4.5, 5, 6, 7, 8, 9, 10, and 24 hours after the ingestion was complete (see Fig. 1). The venous catheter was used until t = 10 hours and was kept open by slowly infusing a sterile saline solution. Samples at t = 24 hours were taken by venepuncture.
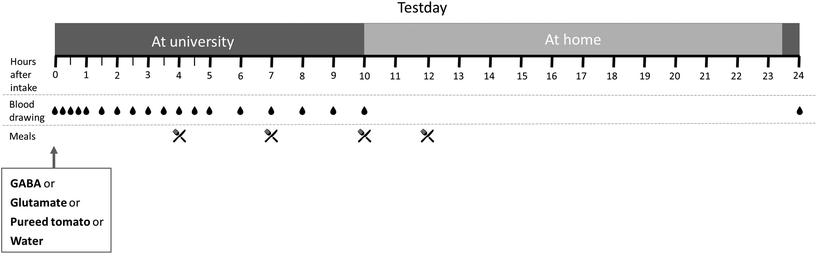 |
| Fig. 1 Study design of a single test day showing the hours after intake of the test products and the different actions of the research participants on each day. In this crossover design, every research participant underwent the test day four times. Each time a different test product was given. | |
During the blood sampling period, participants were provided standardized meals or snacks, supplied by the university, at t = 4, 7, 10, and 12 hours. The meals were consumed after the blood sample was taken. They were low in protein to minimize glutamate intake and did not include any products with a high GABA content. The composition of these meals and the food products is provided in ESI 2.† Between two hours before and until 2 hours after ingestion of the test products, the participants were not allowed to drink water. Water was provided ad libitum during the remainder of the test day. Between t = 10 hours and t = 24 hours, the participants went home but were not allowed to eat or drink anything except the provided snack at t = 12 hours and water. They were also asked not to take part in intensive exercise. This was monitored by questioning the day after.
2.3 Preparation of test products
The test products were 888 mg of GABA powder (99% purity, as acid, food grade, BulkSupplements, lot: 1902403) dissolved in 1 liter of water; 3673 mg of glutamate powder (99% purity, L-glutamic acid, food grade, Sigma Aldrich, W328512) dissolved in 1 liter of water; 1 liter of pureed fresh tomatoes; and 1 liter of water as the control. Tomatoes (cv. Madara, a GABA-rich variety) were provided by the vegetable breeding company Nunhems Netherlands BV (Nunhem, NL). After arrival at Wageningen University, the fresh whole (unpeeled) tomatoes were homogenized in a blender (HBH650, Hamilton Beach) for 20 seconds at the maximum speed, which resulted in a homogeneous juice containing 100% tomatoes. The pureed tomato was stored in batches of 1.5 liters in plastic bags at −20 °C. A first harvest batch (∼1 kilo, a week before the study start date) was processed and three samples were analyzed before the start of the study to determine the GABA and glutamate content of the tomatoes (see section 2.4). The amount of GABA and glutamate powder dissolved in water was adjusted to the amounts present in this first batch of pureed tomatoes. These pureed tomatoes contained 888 (SD 34.8) mg L−1 GABA and 3673 (SD 486) mg L−1 glutamate. The day before each test day, the required amount of pureed tomato was taken out of the freezer and left to thaw at room temperature. GABA and glutamate were dissolved in a liter of water in plastic bottles the day before each test day. It was specifically chosen to dissolve the powders in water, instead of administering as capsules, to obtain a similar concentration as was found in the freshly pureed tomatoes. The pureed tomato samples were taken on each test day for retrospective determination of the actual GABA and glutamate contents.
2.4 Analysis of GABA and glutamate content in tomatoes
The concentrations of GABA and glutamate present in the pureed tomato were quantified using gas chromatography coupled to mass spectrometry (GCMS). The extraction procedure was according to the protocol described by Carreno-Quintero et al. 2012.20 Briefly, 200 mg of pureed tomato fruit was extracted with 1400 μl of 90% (v
:
v) methanol/water solution containing 15
000 ng mL−1 GABA-d6 and 50
000 ng mL−1L-glutamic acid-d5 (both from Merck-Sigma). After sonication and centrifugation (20
000g) each for 10 minutes, 500 μL of the clear supernatant was taken and mixed with 375 μL of chloroform and 750 μL of water. After centrifugation (20
000g) again, 50 μL aliquots of the upper (polar) phase were dried overnight (16 h) by vacuum centrifugation (Savant®, SPD121P, Thermo Scientific) at room temperature, and the vials were subsequently closed under an argon atmosphere with magnetic crimp caps. Prior to their analysis, dried extracts were derivatized online using a TriPlusRSH autosampling/injection robot (Thermo Scientific), which was programmed to firstly add 12.5 μL of O-methylhydroxylamine hydrochloride (20 mg mL−1 pyridine), then incubate for 30 min at 40 °C with agitation, and finally derivatize with 17.5 μL of N-methyl-N-trimethylsilyltrifluoroacetamide (MSTFA) for 60 min.
The derivatized compounds were analyzed by a GCMS system (Thermo Scientific) consisting of a Trace 1300 GC with a programmable temperature vaporizing injector coupled to a TSQ8000 DUO-series triple quadrupole MS. One microliter of each sample was introduced into the injector at 70 °C using a split flow of 19 mL min−1. Chromatographic separation was performed using a VF-5 ms capillary column (Varian, Palo Alto, CA, USA; 30 m × 0.25 mm × 0.25 mm) including a 10 m guard column, with helium as the carrier gas at a column flow rate of 1 mL min−1. The column effluent was ionized by electron impact at 70 eV and mass spectra of the eluting compounds were acquired in a combined selective reaction monitoring (SRM) and full scan mode with a m/z range of 50 to 600 at an ion source temperature of 290 °C. The absolute levels of GABA and glutamate were calculated using calibration curves based on 6 different concentrations of GABA (from 1000 up to 100
000 ng mL−1) and glutamate (from 5000 up to 500
000 ng mL−1) while taking into account the recovery of their deuterated internal standards. Macronutrient composition of the pureed tomato was analyzed in an external laboratory (Nutrilab B.V., Giessen, the Netherlands) using validated methods.
2.5 Blood collection
Blood was drawn via a catheter or via venepuncture (t = 24 h samples) from a forearm vein and collected in 3 mL K2EDTA blood collection tubes (BD Vacutainer; Becton, Dickinson and Company). Next, the tubes were centrifuged at 3000g for 10 minutes at 4 °C (Sigma 4-16K; Sigma-zentrifugen). Plasma samples were immediately put on dry ice for rapid freezing. At the end of each test day, the plasma samples were stored at −80 °C until further analysis.
2.6 Plasma sample extraction and UPLC-MS/MS analysis
Plasma GABA and glutamate concentrations were quantified according to a validated UPLC-MS/MS method. The full extraction and analysis protocol including the method validation, are described elsewhere in full detail.21 Briefly, the analytes were extracted from plasma as follows. To 100 μL of plasma, 400 μL of acetonitrile (ACN, Biosolve, Valkenswaard, the Netherlands) containing 0.1% v/v formic acid (FA, Biosolve, Valkenswaard, the Netherlands), 200 ng mL−1 GABA-d2 (Sigma Aldrich, Munich, Germany, 617458) and 2000 ng mL−1 glutamic acid-d5 (Sigma Aldrich, Munich, Germany, 631973) were added. Samples were mixed at 1400 rpm (Eppendorf thermomixer, Eppendorf) for 5 minutes and centrifuged at 15
000g for 15 minutes. The supernatant was diluted to 95% ACN with 1500 μL of ACN. The extract was purified by solid phase extraction (SPE) using BondElut C8 200 mg SPE cartridges (Agilent, Santa Clara, United States). To this end, the samples were loaded onto the cartridges, previously conditioned with 1 mL of methanol (Biosolve, Valkenswaard, the Netherlands) and 1 mL of 50% methanol. Cartridges were washed with 2 mL of 95% ACN and the analytes were eluted with 2 mL of 80% acetonitrile. Samples were evaporated to dryness in a vacuum concentrator (Turbovap, Biotage, Uppsala, Sweden) for 40 minutes at 30 °C and dissolved in 100 μL of MQ water with 0.1% v/v FA. The extracts were stored at −80 °C until analysis.
Extracts were analyzed for determining GABA and glutamate concentrations using an Acquity I-class UPLC coupled to a Xevo TQ-S triple quadrupole mass spectrometer (Waters Corp, Etten-Leur, the Netherlands). Chromatographic separation was performed on an Acquity UHPLC HSS reversed phase C18 column (2.1 × 150 mm with 1.8 μm particle size, Waters Corp, Etten-Leur, the Netherlands). Eluent A consisted of MQ water with 0.1% v/v FA, and eluent B consisted of 100% methanol. The following gradient was applied: 98% A; 0.0–3.0 min, 98%–50% A; 3.0–6.0 min, 50% A; 6.0–8.0 min, 98% A; 8.0–10.0 min. Total runtime was 10 minutes and the eluent flowed at a rate of 0.15 mL min−1. Positive electrospray ionization was used for all analytes. The MS was run in the SRM mode. The following mass to charge ratios in the precursor to product ion transitions were used: for GABA 104.1 > 87, for GABA-d2 106.1 > 89.1, for glutamate 148 > 130 and for glutamate-d5 153 > 135.
Calibration ranges were 3.4–1500 ng mL−1 for GABA and 30.9–22
500 ng mL−1 for glutamate. Quantification was performed against a linear, 1/x weighted, regression curve based on the duplicate injection of calibration standards. Within- and between-day deviation of accuracy and precision of the method were <10% at the quality control concentrations of 15 ng mL−1 (low), 150 ng mL−1 (medium), and 1500 ng mL−1 (high) for GABA, and 1000 ng mL−1 (low), 5000 ng mL−1 (medium) and 15
000 ng mL−1 (high) for glutamate. Similar accuracy and precision characteristics were achieved during this analysis. Sample extraction and analysis were performed after finishing the trial, taking all samples of the same participant together in a single run. For every 10 study samples, a quality control sample was analyzed.
2.7 Data analysis
Statistical analyses were performed using R 3.6.1.22 Figures were produced using the R package ggplot2.23 The 0–10 h area under the curve (AUC), the maximum concentration (Cmax) and the time to peak (tmax) were calculated using GraphPad Prism 5 (GraphPad Software). For the calculation of the area under the curve, the trapezoidal rule was used. Area under the curve was baseline adjusted by subtracting the area under the curve of the control (1 liter of tap water) condition. Normality was visually assessed using normal qqplots. For both the Cmax of the GABA and glutamate concentrations as well as for the AUC of GABA, there was an outlier. Outliers were included in the analysis since the data are valid and outliers could be representative of the variability in the population. Furthermore, excluding these outliers did not affect the test outcome, and the mean, standard error and test result without the outliers are shown in the ESI 3.† The differences between the AUC, Cmax and tmax between the supplement and the tomato conditions were assessed using a paired-samples t-test. Differences in fasting plasma concentrations were assessed using ANOVA. Differences were considered to be significant if P < 0.05. Correlation between fasting plasma GABA and glutamate concentrations was assessed using a Pearson's correlation. To determine the differences between individual timepoints, a two-way repeated measures ANOVA was carried out in IBM SPSS Statistics 25 with the Bonferroni adjustment. For this analysis the data were log-transformed, and one extreme outlier was removed.
3. Results
3.1 Participants and test products
Participant characteristics are described in the Materials and Methods section. A total of 11 participants completed all four test days. GABA and glutamate contents of the first harvest batch of Madara tomatoes were analyzed just before this study, and the concentrations in the GABA and glutamate solutions were based on these analyses. The macronutrient composition of the pureed Madara tomatoes is shown in Table 1. As a check to assess the actual administered dose, a sample from the pureed tomatoes was taken on each study day, just before it was consumed. These pureed samples were analyzed after the completion of the study for their GABA and glutamate contents. In the pureed tomato samples (n = 8), the GABA content was on average 1044 (SD 98.5, i.e., 9.4 CV%) mg L−1 which is 118% of the initially measured 888 mg L−1, and the glutamate content was on average 3574 (SD 297, i.e., 8.3 CV%) mg L−1 which is 97% of the initial 3673 mg L−1. These differences between the actual and target values, which were <20%, were deemed acceptable in light of the 20% tolerance limits that are allowed for declaring nutrient content.24
Table 1 Macronutrient composition of the pureed Madara tomatoes
Macronutrients |
g per 100g pureed tomatoa |
A litre of pureed tomatoes weighs 1.03 kilograms.
|
Water |
91.9 |
Protein |
1.1 |
Fat |
<0.5 |
Carbohydrate |
3.7 |
Fibre |
1.7 |
3.2 Plasma kinetics of GABA and glutamate
The mean plasma GABA concentration–time profiles following the consumption of each test product is shown in Fig. 2A. The descriptive kinetic parameters are summarized in Table 2 and individual data are presented in Fig. 3. Following the consumption of GABA solution in water and pureed tomatoes, the GABA concentrations peaked rapidly after 0.5 and 0.36 hours, respectively. Participants tended to have a shorter mean GABA tmax after consumption of pureed tomatoes (P = 0.052). Although the mean AUC and Cmax of GABA were higher following the ingestion of pureed tomatoes, they were not significantly different. The (non-significant) difference in the mean AUC and Cmax between the GABA solution and pureed tomatoes can mostly be explained by a single outlier; one participant had a more than 3-fold higher AUC and 5-fold higher Cmax of GABA in response to tomato consumption as compared to the GABA solution in water (Fig. 3A and B).
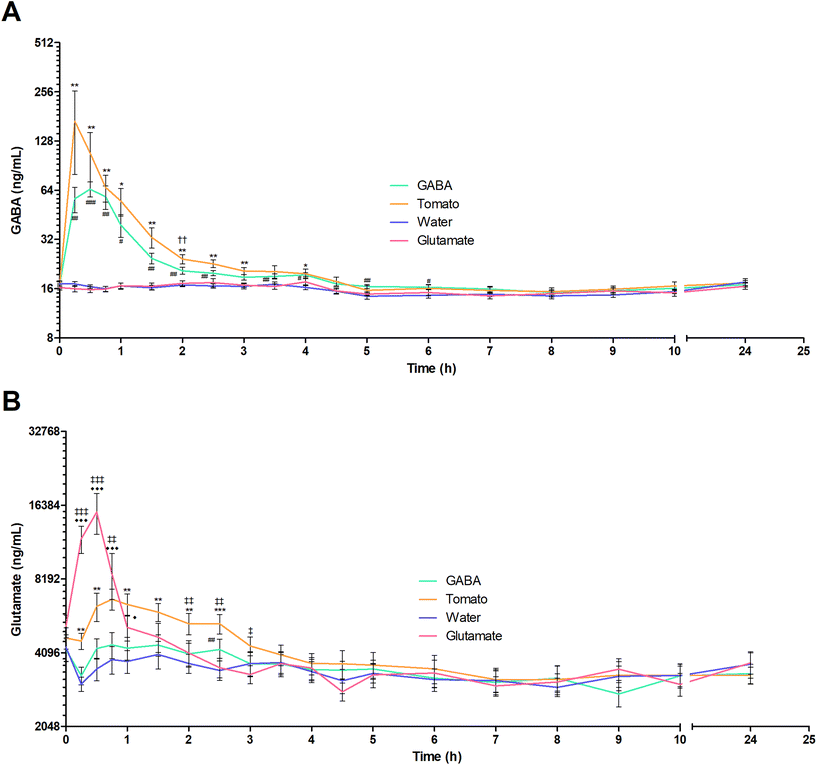 |
| Fig. 2 Average plasma GABA and glutamate concentration–time profiles for 11 volunteers. (A) Average plasma GABA concentration over time is shown after ingestion of GABA solution (888 mg in 1 liter), pureed tomatoes (1 liter, 1044 mg GABA and 3574 mg glutamate), water (1 liter) or glutamate (3673 mg in 1 liter). (B) Average plasma glutamate concentration over time is shown after ingestion of GABA (888 mg in 1 liter), tomato (1 liter), water (1 liter) or glutamate (3673 mg in 1 liter). Data is presented as mean ± SEM on a logarithmic scale. The following symbols represent significant differences in GABA/glutamate plasma concentration for the test products relative to that after ingestion of water. For GABA, #: P < 0.05; ##: P < 0.01; ###: P < 0.001. For tomato, *: P < 0.05; **: P < 0.01; ***: P < 0.001. For glutamate, ◆: P < 0.05; ◆◆: P < 0.01; ◆◆◆: P < 0.001. Significant differences between the GABA solution and the pureed tomato are represented by: ††: P < 0.01. Significant differences between the glutamate solution and the pureed tomato are represented by: ‡: P < 0.05; ‡‡: P < 0.01; ‡‡‡: P < 0.001. | |
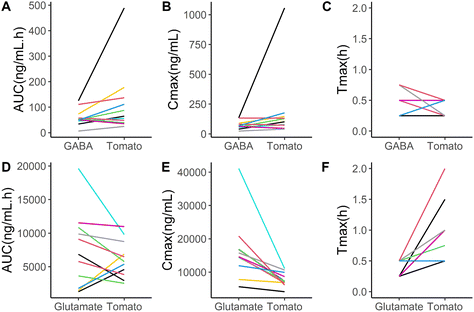 |
| Fig. 3 Line graphs showing kinetic parameters per individual, comparing tomato to the GABA and glutamate solutions. The individual's GABA kinetic parameters 10-hour AUC, Cmax and tmax are depicted in respectively Figure A, B and C. The individual's glutamate kinetic parameters 10-hour AUC, Cmax and tmax are depicted in respectively Figure D, E and F. Colors represent the research subjects. | |
Table 2 Plasma kinetic parameters of GABA and glutamate. Parameters are calculated from plasma kinetics after ingestion of the GABA and glutamate solutions and pureed tomatoes. The 10 hour area under the curve shown is corrected for the area under the curve after taking the placebo
Test product |
|
GABA solution |
Pureed tomato |
|
GABA |
Mean |
SEM |
Mean |
SEM |
P-value |
C
max (ng mL−1) |
74.7 |
10.5 |
184.0 |
88.4 |
0.213 |
t
max (h) |
0.50 |
0.05 |
0.36 |
0.04 |
0.052 |
AUC (ng mL−1 h−1) |
59.7 |
10.1 |
115.7 |
40.0 |
0.117 |
|
Glutamate solution |
Pureed tomato |
|
Glutamate |
Mean |
SEM |
Mean |
SEM |
P-value |
C
max (ng mL−1) |
16420 |
2779 |
7815 |
628 |
0.006 |
t
max (h) |
0.41 |
0.04 |
0.98 |
0.14 |
0.003 |
AUC (ng mL−1 h−1) |
7579 |
2272 |
7362 |
1873 |
0.929 |
The differences in mean concentration at the individual timepoints were assessed by a two-way repeated measures ANOVA. When comparing the plasma GABA concentration following the consumption of the GABA solution to that after pureed tomatoes, a significant difference was only seen at 2 hours after intake (19.74 ± 0.65 ng mL−1vs. 23.08 ± 1.19 ng mL−1, P = 0.003) (Fig. 2A). When comparing with water, significant differences in the GABA plasma concentrations were found until 4 hours after consumption of both pureed tomatoes and the GABA solution (t = 4 hours: 19.06 ± 1.24 ng mL−1 and 19.23 ± 0.59 ng mL−1vs. 16.11 ± 0.57 ng mL−1, P = 0.015 and P = 0.010 respectively). After ingestion of the GABA solution, the GABA plasma concentration was elevated compared to that after water at two later timepoints as well: after 5 hours (16.19 ± 0.41 vs. 14.07 ± 0.61 ng mL−1, P = 0.003) and 6 hours (16.04 ± 0.54 vs. 14.26 ± 0.48 ng mL−1, P = 0.003). Although some differences in plasma kinetics were observed, on average, the food matrix did not markedly affect GABA bioavailability.
The mean plasma glutamate concentration–time profiles after ingestion of each test product are shown in Fig. 2B. Following consumption of the glutamate solution, glutamate plasma levels peaked rapidly. However, the mean glutamate Cmax was significantly lower after the consumption of pureed tomatoes compared to the glutamate solution (7815 ± 627 ng mL−1vs. 16
420 ± 2778 ng mL−1, P = 0.006) (Table 2). In addition, the glutamate curve shows a significantly longer tmax, after the consumption of pureed tomatoes compared to the solution in water (0.41 ± 0.04 h vs. 0.98 ± 0.14 h, P = 0.003). Yet, when comparing with the glutamate solution, the average AUC of glutamate after ingestion of pureed tomatoes was not significantly different.
When comparing the glutamate plasma concentration at individual timepoints following the consumption of the glutamate solution and pureed tomatoes, significant differences were seen at 15 minutes (11
933 ± 1508 vs. 4571 ± 346, P = < 0.0001), 30 minutes (15
379 ± 2925 vs. 6330 ± 838, P = <0.0001), 45 minutes (8579 ± 1818 vs. 6776 ± 669, P = 0.001), 2 hours (4082
± 502 vs. 5379 ± 541, P = 0.003), 2.5 hours (3563 ± 323 vs. 5378 ± 488, P = 0.001) and 3 hours (3339 ± 284 vs. 4365 ± 387, P = 0.022) (Fig. 2B). When comparing with water, significant differences were found until 1 hour (t = 1 hour: 5195 ± 619 vs. 3775 ± 400, P = 0.030) after the consumption of the glutamate solution and for 2.5 hours (t = 2.5 hours: 5378 ± 487 vs. 3470 ± 314, P = <0.0001) after the consumption of pureed tomatoes. In summary, these results show a lower and later peak glutamate plasma concentration and a slower time-dependent decline after the ingestion of pureed tomatoes as compared to the glutamate solution.
Fig. 2 also shows that glutamate intake does not seem to influence GABA plasma concentrations. While this is mostly also true the other way around, a significantly elevated glutamate concentration as compared to that from water is observed 2.5 hours after intake of the GABA solution (4047 ± 433 vs. 3705 ± 332, P = 0.010).
3.3 Interindividual differences in kinetic parameters
The interindividual differences are illustrated using line graphs, comparing the GABA and glutamate kinetic variables of the pureed tomato and the solutions (Fig. 3). For both GABA and glutamate concentrations, on average no significant differences in AUC were found (Table 2), while interindividual differences are seen in these line graphs. Eight out of the eleven participants showed a higher AUC for GABA after taking the pureed tomatoes than after taking the GABA solution (Fig. 3A). The tmax and Cmax of GABA also show interindividual differences (Fig. 3B and C). In the case of glutamate, eight out of the eleven participants showed a lower AUC after taking the pureed tomatoes than after taking the glutamate solution (Fig. 3D). The direction of the differences in the tmax and Cmax of glutamate shows more consistency between the participants (Fig. 3E and F).
3.4 Variation in fasting GABA and glutamate concentrations
Fasting GABA and glutamate levels were measured 4 times in each individual over the course of 5 weeks, each time in the early morning. Fig. 4 shows the 4 GABA and glutamate plasma concentrations per individual. We found no correlation between fasting GABA and glutamate plasma concentrations, r = 0.137. Average fasting GABA was found to be 16.71 (SD 2.18) ng mL−1 in a range from 14.2 to 20.3 ng mL−1 (Fig. 4A). Average fasting glutamate was found to be 4626 (SD 1666) ng mL−1 in a range from 3021 to 6723 ng mL−1 (Fig. 4B).
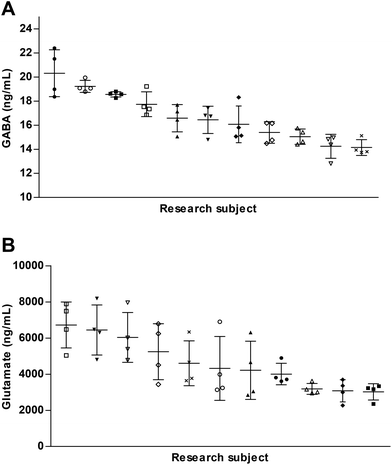 |
| Fig. 4 Scatter dot plots of fasting GABA and glutamate concentrations from 11 volunteers. Showing (a) GABA and (b) glutamate fasting plasma concentrations in ng mL−1 per individual measured at 4 different days with at least a week in between. Concentrations are ordered from highest on the left to lowest on the right. In both graphs A and B, the different symbols indicate the same participant. Expressed as individual values and mean ± SD. | |
The average within person coefficient of variation (CV) for GABA was 5.8% (SD 2.58%). The average within person CV for glutamate was 23.5% (SD 9.76%). This shows that fasting GABA concentrations are fairly stable over a period of 5 weeks, while this is less the case for glutamate. With an ANOVA it was assessed whether the observed differences between individuals in fasting plasma concentrations were not due to chance. Fasting plasma GABA (F(10, 33) = 14.41, p = <0.001) and glutamate (F(10, 33) = 4.84, p = <0.001) concentrations were found to be significantly different between participants.
4. Discussion
In view of their pleiotropic effects, including but not limited to their role as neurotransmitters, more insight into the uptake and potential effects of GABA and glutamate obtained through the diet is of relevance. However, thus far limited information is available about the oral bioavailability of GABA and glutamate. To our knowledge we are the first to show that GABA is bioavailable from tomatoes and that its bioavailability is not markedly hampered by the food matrix, although some differences in kinetics are observed. On the other hand, we show that glutamate kinetics are significantly influenced by the tomato matrix.
The plasma kinetics of oral GABA have not extensively been investigated since it is primarily known for its endogenous properties as a neurotransmitter and not as a nutritional component. We show that after oral GABA intake, the plasma GABA concentration peaks rapidly after 0.5 hours, reaching values of ∼4.5 times that at the baseline. A similar study was done by Li et al. (2015), who described the plasma kinetics of a GABA supplement.18 Our findings are not entirely in line with their results. The authors also observe a rapid increase in GABA levels after intake. However, they measured a time to peak concentration of 1.5 hours at ∼45 times the baseline values. Remarkably, the participants received a single dose of 2 grams of GABA, which is only 2.25 times the dose that was given in the present study. Formulation in tablets could have been responsible for a longer time to peak by requiring solubilization of GABA, as was observed in the study by Li et al. (2015). In addition, compared to administration in a relatively large volume of water, higher local concentrations are likely when taking a tablet. Combined with the higher dose administered, this may at least partly explain the difference found in the maximum achieved concentration.
In both our results and the paper from Li et al. (2015), a clear transient elevation in plasma GABA concentration is observed after the ingestion of GABA. However, measurements in blood only reflect the current circulating concentrations, which are the balance of various processes that may work in opposing directions. These processes such as the absorption, distribution, metabolism and excretion of GABA are not yet fully understood. Studies in mice suggest that intravenously administered GABA is distributed extremely rapidly (within 3 minutes after injection), mainly to the liver and kidneys, and metabolized to other components, like succinate.25–27 In the liver, GABA-transaminase activity is high and it is therefore mainly responsible for the catabolism of GABA.28 The high uptake of GABA from the portal vein into the liver would keep the plasma GABA concentration in systemic circulation relatively low. Studies with, for example, isotopically labeled GABA would therefore be necessary to further elucidate the route that GABA takes after oral ingestion.
The main goal of the present study was to determine the relative bioavailability of GABA from tomatoes. We show that GABA uptake and bioavailability from tomatoes is not significantly hampered by the food matrix. Therefore, it can be concluded that the food matrix does not markedly affect the absorption of GABA, although small differences in kinetics are observed. Interindividual variation in the GABA concentration after ingestion of tomatoes is larger than the variations after GABA intake in a solution. In some individuals, the matrix seems to enhance the GABA bioavailability, even when considering the somewhat higher amount of GABA in the pureed tomatoes (118%) compared to the GABA solution. For further research a larger sample size would be needed to study the incidence of these differences. In the current study, the tomatoes were pureed to minimize interindividual variation. This standardizes the mode of ingestion and avoids batch effects. This pre-processing may have influenced the bioaccessibility of GABA. Ingestion of whole tomatoes would require additional steps in the digestive process which could possibly delay or inhibit GABA absorption. Therefore, it is not unlikely that GABA bioavailability from whole tomatoes is slightly lower.
Other nutrients or non-nutritive components present in the pureed tomatoes do not seem to influence GABA bioavailability. GABA is hydrophilic in nature and hence its absorption is unlikely to be affected by the presence of fats which is for example the case for the carotenoids in tomatoes.29 Data from other studies have shown that GABA uptake from the intestinal lumen requires active transport processes, such as through PAT1 (SLC36A1) which is a low affinity and high capacity transporter (in the mM range).30 PAT1 is also responsible for the transport of other small amino acids like proline, alanine and glycine, and of pharmacological GABA analogues like vigabatrin.31 The literature regarding these GABA analogues may be relevant in interpreting our findings. The kinetic properties of vigabatrin have been more extensively investigated than those of GABA itself.32 Nøhr et al. (2014) looked at the interactions with food with regard to the uptake of vigabatrin.32 They found that the presence of amino acids that are substrates of PAT1 (proline, tryptophan and sarcosine) inhibits the uptake of vigabatrin. Further research could investigate whether this is also the case for GABA uptake. In tomatoes, these amino acids are also present but in much lower amounts compared to GABA.33 Therefore, the results of this study do not rule out potential effects of food components, not present in tomatoes, on GABA bioavailability. Studies have been performed with functional foods that aimed for high GABA concentrations, like fermented milk products.34,35 Additional specific assessment of GABA bioavailability in these types of food products would be relevant.
Notwithstanding the consistency of our findings when considering these at a group level, it remains of interest to pay attention to specifically the interindividual variation in GABA kinetics. Just like Li et al. (2015) we find a large interindividual variation in GABA plasma kinetics (AUC CV of 81% (Li et al. (2015)) and 56% (present study)). Many factors influence plasma kinetics, like for example the gastric emptying rate which has an interindividual variation of 16%–39% (AUC CV) even under highly standardized conditions.36 However, genetic polymorphisms in transport processes can also play a role here. Genetic variation in genes encoding for proteins involved in GABA transport are known to exist. Single nucleotide polymorphisms (rs357629, rs357618) in the PAT1 transporter have been found to affect its function for instance.37 Similarly, a single nucleotide polymorphism (rs555044) was found to influence the function of GAT2 (SLC6A13), a transporter protein in the liver, responsible for GABA transport.38
Besides GABA, tomatoes are also very rich in glutamate, one of the other main neurotransmitters and the precursor of GABA. For this reason, we also investigated the relative oral bioavailability of glutamate from tomatoes and any interaction with GABA levels. Although we do not see an increase in GABA concentration after the ingestion of glutamate, we do observe a slight increase in glutamate concentration after the intake of GABA. This observation needs independent replication before any speculation on the mechanism is made. Glutamate is well known as a nutrient and food additive; therefore more research about its oral bioavailability is already available. We found that glutamate appears rapidly in the bloodstream, peaking after about 25 minutes at ∼3.6 times the baseline concentration. Multiple studies have been conducted that investigated the plasma kinetics of monosodium glutamate (MSG).39,40 In those studies, a 3 times higher dose was used compared to the present study. Both in our study as in those studies, the glutamate was given as a solution in water, although in a lower volume (∼300 mL). Considering this higher dose, their results are comparable to the glutamate concentration time curve that we have measured. They report a similarly rapid increase in glutamate plasma concentrations with a peak after about 30 minutes at ∼7.6 and ∼14.6 times the baseline concentration. Glutamate normally has a low bioavailability, with 95% of the glutamate ingested being used as a substrate by the enterocytes or metabolized into other amino acids like alanine, aspartate and GABA.41,42 Stegink et al. (1983) showed that the bioavailability of glutamate is even further reduced by the co-ingestion of carbohydrates.39 In their study, a high oral dose of glutamate together with 1.1 gram of carbohydrate led to a blunted peak of the glutamate concentration. The authors suggest that the carbohydrates serve as a source of pyruvate which would facilitate the catabolism of glutamate into alanine and therefore reduces the amount of glutamate that reaches the circulation. Also for other amino acids it is known that co-ingestion with carbohydrates reduces their bioavailability.43 This would be in line with our findings. The amount (1 kg) of pureed tomatoes that the participants consumed contained 37 grams of carbohydrates. It is therefore likely that also in our study the carbohydrates reduced the maximum concentration of glutamate in the circulation.
On the other hand, as opposed to the findings by Stegink et al. (1983), we show that the relative oral bioavailability, as indicated by the AUC, did not differ between the glutamate solution and the pureed tomatoes. However, these two conditions cannot directly be compared since tomatoes also contain protein-bound glutamate, while the dose of the glutamate solution was based solely on the amount of free glutamate in the tomatoes. The amount of pureed Madara tomato consumed contained 11 grams of protein, and on average 7–13% of plant protein consists of glutamate.44,45 This would mean that upon ingestion of the pureed tomatoes the participants received an estimated maximum of 1430 mg protein-bound glutamate in addition to the measured 3574 mg of free glutamate. Glutamate plasma kinetics following tomato consumption has not been investigated previously, but presumably the proteins that contain glutamate need to be (fully) hydrolyzed before absorption. The plasma kinetics of glutamate from tomatoes show a similar pattern as is commonly seen with amino acids that are incorporated into proteins, like phenylalanine and leucine.46 This leads to a prolonged absorption phase, as shown by elevated plasma concentrations until 2.5 hours after ingestion of pureed tomatoes as opposed to just 1 hour after ingestion of the glutamate solution. We therefore speculate that the glutamate AUC would have been lower after ingestion of pureed tomatoes if no glutamate-containing protein was present. This strongly suggests that the relative oral bioavailability of free glutamate is lower after the ingestion of pureed tomatoes as compared to that after the ingestion of glutamate solution in water, most likely due to the presence of carbohydrates in tomatoes.
The crossover design also gave us the opportunity to measure fasting GABA and glutamate. This could provide us with useful information since several studies suggest that their concentrations in the circulation are associated with a spectrum of central nervous system related outcomes. For example, abnormal GABA and glutamate concentrations in blood have been associated with depression, autism, schizophrenia and bipolar disorder.47–58 For each individual, we collected 4 samples at least one week apart. Their analyses show the individual variation of GABA and glutamate plasma concentrations over time. The GABA plasma concentration appears to be very stable. Both within each individual on different days and within the day, there are significant differences between individuals. Petty et al., (1983) related GABA plasma concentration to the occurrence of depression. They found a similar range of GABA plasma concentrations of up to 20 ng mL−1 as we found here. Interestingly, GABA concentrations below 10 ng mL−1 were only found in depressed individuals. As of yet, it is unclear how genetic variability, differences in microbiota composition,59–61 or long-term dietary intake of GABA, influence the plasma concentrations of individuals. As compared to GABA, fasting glutamate plasma concentrations between the different days were found to be far more variable. It is unknown how differences in behavior and food intake in between the test days might have influenced glutamate concentrations. However, the participants were asked to eat the same meals the day before each test day. These findings should be considered when interpreting research that investigates the potential use of glutamate as a biomarker.
We show that a food matrix has a different impact on GABA and glutamate bioavailability, and the practical implications of these results are therefore different for both molecules. Since the glutamate kinetics profile is influenced by the food matrix it seems that physiological effects of glutamate supplementation cannot be immediately generalized to glutamate that is ingested with food. On the other hand, food products, or at least tomatoes, are a viable natural source of GABA. Bioavailability does not seem to be reduced by the food matrix. In light of these findings, it is tempting to speculate that the presumed positive health effects ascribed to GABA, would also be applicable to GABA-containing food products. While long term health effects of a high GABA intake have not yet been determined in humans, the results of this study merit further investigation of the potential health effects of GABA-rich foods and diets.
Author contributions
Tessa H. de Bie: conceptualization, methodology, formal analysis, investigation, writing–original draft, visualization, project administration; Michiel G. J. Balvers: conceptualization, methodology, writing–review & editing, supervision. Ric C. H. de Vos: methodology, formal analysis, writing–review & editing. Renger F. Witkamp: conceptualization, writing–review & editing, supervision, funding acquisition. Maarten A. Jongsma: conceptualization, methodology, writing–review & editing, supervision, funding acquisition.
Sources of support
This work was supported by a consortium of companies (Agrico Research, Nunhems Netherlands, AVEBE) taking part in a collaborative public-partnership under the “Topsector Agri-Food” programme organised by the Dutch Ministry of Economic Affairs [grant number AF17048].
Conflicts of interest
No conflicts of interest.
Acknowledgements
We thank Sanne Gjaltema for her help in laboratory work and conducting the study. We also thank the staff of the Human Nutrition Research Unit (Henriette Fick-Brinkhof and Ineke Klöpping-Ketelaars) for their help in conducting the study. We thank Henriëtte van Eekelen and Roland Mumm, both from WPR-Bioscience, for their technical assistance in analysing the GABA and glutamate content of the tomatoes.
References
- S. E. Hyman, Neurotransmitters, Curr. Biol., 2005, 15(5), R154–R158, DOI:10.1016/J.CUB.2005.02.037.
- A. Barragan, J. M. Weidner, Z. Jin, E. R. Korpi and B. Birnir, GABAergic signalling in the immune system, Acta Physiol., 2015, 213(4), 819–827, DOI:10.1111/apha.12467.
- E. Xu, M. Kumar and Y. Zhang,
et al., Intra-islet insulin suppresses glucagon release via GABA-GABAA receptor system, Cell. Metab., 2006, 3(1), 47–58, DOI:10.1016/J.CMET.2005.11.015.
- N. Ben-Othman, A. Vieira and M. Courtney,
et al., Long-Term GABA Administration Induces Alpha Cell-Mediated Beta-like Cell Neogenesis, Cell, 2017, 168(1–2), 73–85.e11, DOI:10.1016/J.CELL.2016.11.002.
- D. Menegaz, D. W. Hagan and J. Almaça,
et al., Mechanism and effects of pulsatile GABA secretion from cytosolic pools in the human beta cell, Nat. Metab., 2019, 1(11), 1110–1126, DOI:10.1038/s42255-019-0135-7.
- G. M. Noguchi and M. O. Huising, Integrating the inputs that shape pancreatic islet hormone release, Nat. Metab., 2019, 1(12), 1189–1201, DOI:10.1038/s42255-019-0148-2.
- J. Tian, H. Dang and Z. Chen,
et al., γ-aminobutyric acid regulates both the survival and replication of human β-cells, Diabetes, 2013, 62(11), 3760–3765, DOI:10.2337/db13-0931.
- K. Hayakawa, M. Kimura and K. Kamata, Mechanism underlying γ-aminobutyric acid-induced antihypertensive effect in spontaneously hypertensive rats, Eur. J. Pharmacol., 2002, 438(1–2), 107–113, DOI:10.1016/S0014-2999(02)01294-3.
- S. Sohrabipour, M. R. Sharifi, A. Talebi, M. Sharifi and N. Soltani, GABA dramatically improves glucose tolerance in streptozotocin-induced diabetic rats fed with high-fat diet, Eur. J. Pharmacol., 2018, 826, 75–84, DOI:10.1016/J.EJPHAR.2018.01.047.
- N. Soltani, H. Qiu and M. Aleksic,
et al., GABA exerts protective and regenerative effects on islet beta cells and reverses diabetes, Proc. Natl. Acad. Sci. U. S. A., 2011, 108(28), 11692–11697, DOI:10.1073/pnas.1102715108.
- N. Rathwa, N. Parmar and S. P. Palit,
et al., Calorie restriction potentiates the therapeutic potential of GABA in managing type 2 diabetes in a mouse model, Life Sci., 2022, 295, 120382, DOI:10.1016/J.LFS.2022.120382.
- A. M. Abdou, S. Higashiguchi, K. Horie, M. Kim, H. Hatta and H. Yokogoshi, Relaxation and immunity enhancement effects of gamma-aminobutyric acid (GABA) administration in humans, Biofactors, 2006, 26(3), 201–208 CrossRef CAS PubMed.
- A. Yoto, S. Murao and M. Motoki,
et al., Oral intake of γ-aminobutyric acid affects mood and activities of central nervous system during stressed condition induced by mental tasks, Amino Acids, 2012, 43(3), 1331–1337, DOI:10.1007/s00726-011-1206-6.
- S. B. Sarasa, R. Mahendran, G. Muthusamy, B. Thankappan, D. R. F. Selta and J. Angayarkanni, A Brief Review on the Non-protein Amino Acid, Gamma-amino Butyric Acid (GABA): Its Production and Role in Microbes, Curr. Microbiol., 2020, 77(4), 534–544, DOI:10.1007/S00284-019-01839-W/FIGURES/5.
- J. M. Matés, J. A. Segura and J. A. Campos-Sandoval,
et al., Glutamine homeostasis and mitochondrial dynamics, Int. J. Biochem. Cell Biol., 2009, 41(10), 2051–2061, DOI:10.1016/J.BIOCEL.2009.03.003.
- B. Redruello, A. Szwengiel, V. Ladero, B. del Rio and M. A. Alvarez, Identification of technological/metabolic/environmental profiles of cheeses with high GABA contents, LWT, 2020, 109603, DOI:10.1016/J.LWT.2020.109603.
- B. Burton-Freeman and K. Reimers, Tomato Consumption and Health: Emerging Benefits, Am. J. Lifestyle Med., 2011, 5(2), 182–191, DOI:10.1177/1559827610387488.
- J. Li, Z. Zhang and X. Liu,
et al., Study of GABA in Healthy Volunteers: Pharmacokinetics and Pharmacodynamics, Front. Pharmacol., 2015, 6, 260, DOI:10.3389/fphar.2015.00260.
- D. H. Ngo and T. S. Vo, An Updated Review on Pharmaceutical Properties of Gamma-Aminobutyric Acid, Molecules, 2019, 24(15), 2678, DOI:10.3390/MOLECULES24152678.
- N. Carreno-Quintero, A. Acharjee and C. Maliepaard,
et al., Untargeted metabolic quantitative trait loci analyses reveal a relationship between primary metabolism and potato tuber quality, Plant Physiol., 2012, 158(3), 1306–1318, DOI:10.1104/pp.111.188441.
- T. H. de Bie, R. F. Witkamp, M. A. Jongsma and M. G. J. Balvers, Development and validation of a UPLC-MS/MS method for the simultaneous determination of gamma-aminobutyric acid and glutamic acid in human plasma, J. Chromatogr. B Anal. Technol. Biomed. Life Sci., 2021, 1164, 122519, DOI:10.1016/j.jchromb.2020.122519.
- R Core Team, R: A Language and Environment for Statistical Computing, 2017, https://www.r-project.org/ Search PubMed.
-
H. Wickham, Ggplot2: Elegant Graphics for Data Analysis, Springer New York, New York, NY, 2009. DOI:10.1007/978-0-387-98141-3.
- European Commission, Guidance Document for Competent Authorities for the Control of Compliance with EU Legislation on Regulation (EU) no. 1169/2011, Council Directive 90/496/EEC and Directive 2002/46/EC with Regard to the Setting of Tolerances for Nutrient Values Declared on a Label, 2012 Search PubMed.
- E. Kuroda, M. Watanabe, T. Tamayama and M. Shimada, Autoradiographic distribution of radioactivity from 14C-GABA in the mouse, Microsc. Res. Tech., 2000, 48(2), 116–126, DOI:10.1002/(SICI)1097-0029(20000115)48:2<116::AID-JEMT7>3.0.CO;2-P.
- D. Ravasz, G. Kacso, V. Fodor, K. Horvath, V. Adam-Vizi and C. Chinopoulos, Catabolism of GABA, succinic semialdehyde or gamma-hydroxybutyrate through the GABA shunt impair mitochondrial substrate-level phosphorylation, Neurochem. Int., 2017, 109, 41–53, DOI:10.1016/j.neuint.2017.03.008.
- A. Mori and M. Kosaka, Incorporation γ–aminobutyric acid (GABA) in the brain and internal organs of mice, Psychiatry Clin. Neurosci., 1961, 15(2), 92–97, DOI:10.1111/j.1440-1819.1961.tb00637.x.
- P. Ferenci, T. Stehle, J. Ebner, R. Schmid and D. Häussinger, Uptake and catabolism of γ-aminobutyric acid by the isolated perfused rat liver, Gastroenterology, 1988, 95(2), 402–407, DOI:10.1016/0016-5085(88)90497-0.
- P. Riso, A. Brusamolino, L. Scalfi and M. Porrini, Bioavailability of carotenoids from spinach and tomatoes, Nutr., Metab. Cardiovasc. Dis., 2004, 14(3), 150–156, DOI:10.1016/s0939-4753(04)80035-8.
- M. Boll, M. Foltz and C. M. H. Anderson,
et al., Substrate recognition by the mammalian proton-dependent amino acid transporter PAT1, Mol. Membr. Biol., 2003, 20(3), 261–269, DOI:10.1080/0968768031000100759.
- L. Metzner, K. Neubert and M. Brandsch, Substrate specificity of the amino acid transporter PAT1, Amino Acids, 2006, 31(2), 111–117, DOI:10.1007/s00726-005-0314-6.
- M. K. Nøhr, S. Frølund, R. Holm and C. U. Nielsen, Pharmacokinetic aspects of the anti-epileptic drug substance vigabatrin: focus on transporter interactions, Ther. Deliv., 2014, 5(8), 927–942, DOI:10.4155/tde.14.55.
- D. Mallamace, C. Corsaro and A. Salvo,
et al., A multivariate statistical analysis coming from the NMR metabolic profile of cherry tomatoes (The Sicilian Pachino case), Phys. A, 2014, 401, 112–117, DOI:10.1016/J.PHYSA.2013.12.054.
- D. M. Linares, T. F. O'Callaghan, P. M. O'Connor, R. P. Ross and C. Stanton, Streptococcus thermophilus APC151 Strain Is Suitable for the Manufacture of Naturally GABA-Enriched Bioactive Yogurt, Front. Microbiol., 2016, 7, 1876, DOI:10.3389/fmicb.2016.01876.
- K. Pouliot-Mathieu, C. Gardner-Fortier, S. Lemieux, D. St-Gelais, C. P. Champagne and J.-C. Vuillemard, Effect of cheese containing gamma-aminobutyric acid-producing lactic acid bacteria on blood pressure in men, PharmaNutrition, 2013, 1(4), 141–148, DOI:10.1016/J.PHANU.2013.06.003.
- M. Grimm, M. Koziolek, J. P. Kühn and W. Weitschies, Interindividual and intraindividual variability of fasted state gastric fluid volume and gastric emptying of water, Eur. J. Pharm. Biopharm., 2018, 127, 309–317, DOI:10.1016/j.ejpb.2018.03.002.
- P. Crocco, E. Hoxha and S. Dato,
et al., Physical decline and survival in the elderly are affected by the genetic variability of amino acid transporter genes, Aging, 2018, 10(4), 658–673, DOI:10.18632/aging.101420.
- B. Yu, Y. Zheng, D. Alexander, A. C. Morrison, J. Coresh and E. Boerwinkle, Genetic Determinants Influencing Human Serum Metabolome among African Americans, PLoS Genet., 2014, 10(3), e1004212, DOI:10.1371/journal.pgen.1004212.
- L. D. Stegink, L. J. Filer and G. L. Baker, Effect of carbohydrate on plasma and erythrocyte glutamate levels in humans ingesting large doses of monosodium L-glutamate in water, Am. J. Clin. Nutr., 1983, 37(6), 961–968, DOI:10.1093/ajcn/37.6.961.
- T. E. Graham, V. Sgro, D. Friars and M. J. Gibala, Glutamate ingestion: the plasma and muscle free amino acid pools of resting humans, Am. J. Physiol. Endocrinol. Metab., 2000, 278(1), E83–E89, DOI:10.1152/ajpendo.2000.278.1.E83.
- P. J. Reeds, D. G. Burrin, B. Stoll and F. Jahoor, Intestinal Glutamate Metabolism, J. Nutr., 2000, 130(4), 978S–982S, DOI:10.1093/jn/130.4.978S.
- S. P. Haÿs, J. M. Ordonez, D. G. Burrin and A. L. Sunehag, Dietary Glutamate Is Almost Entirely Removed in Its First Pass Through the Splanchnic Bed in Premature Infants, Pediatr. Res., 2007, 62(3), 353–356, DOI:10.1203/PDR.0b013e318123f719.
- S. H. M. Gorissen, N. A. Burd, H. M. Hamer, A. P. Gijsen, B. B. Groen and L. J. C. van Loon, Carbohydrate Coingestion Delays Dietary Protein Digestion and Absorption but Does Not Modulate Postprandial Muscle Protein Accretion, J. Clin. Endocrinol. Metab., 2014, 99(6), 2250–2258, DOI:10.1210/jc.2013-3970.
- S. H. Choi, D. S. Kim and N. Kozukue,
et al., Protein, free amino acid, phenolic, β-carotene, and lycopene content, and antioxidative and cancer cell inhibitory effects of 12 greenhouse-grown commercial cherry tomato varieties, J. Food Compos. Anal., 2014, 34(2), 115–127, DOI:10.1016/j.jfca.2014.03.005.
- S. H. M. Gorissen, J. J. R. Crombag and J. M. G. Senden,
et al., Protein content and amino acid composition of commercially available plant-based protein isolates, Amino Acids, 2018, 50(12), 1685–1695, DOI:10.1007/s00726-018-2640-5.
- R. Koopman, S. Walrand and M. Beelen,
et al., Dietary Protein Digestion and Absorption Rates and the Subsequent Postprandial Muscle Protein Synthetic Response Do Not Differ between Young and Elderly Men, J. Nutr., 2009, 139(9), 1707–1713, DOI:10.3945/jn.109.109173.
- H.-L. Cai, R.-H. Zhu and H.-D. Li,
et al., Elevated plasma γ-aminobutyrate/glutamate ratio and responses to risperidone antipsychotic treatment in schizophrenia, Prog. Neuropsychopharmacol. Biol. Psychiatry, 2010, 34(7), 1273–1278, DOI:10.1016/J.PNPBP.2010.07.006.
- A. Arrúe, R. Dávila and M. Zumárraga,
et al., GABA and Homovanillic Acid in the Plasma of Schizophrenic and Bipolar I Patients, Neurochem. Res., 2010, 35(2), 247–253, DOI:10.1007/s11064-009-0048-z.
- Z. Zheng, T. Zhu, Y. Qu and D. Mu, Blood Glutamate Levels in Autism Spectrum Disorder: A Systematic Review and Meta-Analysis, PLoS One, 2016, 11(7), e0158688, DOI:10.1371/journal.pone.0158688.
- A. Palomino, A. González-Pinto and A. Aldama,
et al., Decreased levels of plasma glutamate in patients with first-episode schizophrenia and bipolar disorder, Schizophr. Res., 2007, 95(1–3), 174–178, DOI:10.1016/J.SCHRES.2007.06.012.
- F. Petty, G. L. Kramer, M. Fulton, F. G. Moeller and A. J. Rush, Low Plasma GABA Is a Trait-Like Marker for Bipolar Illness, Neuropsychopharmacology, 1993, 9(2), 125–132, DOI:10.1038/npp.1993.51.
- E. Küçükibrahimoğlu, M. Z. Saygın, M. Çalışkan, O. K. Kaplan, C. Ünsal and M. Z. Gören, The change in plasma GABA, glutamine and glutamate levels in fluoxetine- or S-citalopram-treated female patients with major depression, Eur. J. Clin. Pharmacol., 2009, 65(6), 571–577, DOI:10.1007/s00228-009-0650-7.
- F. Petty, G. L. Kramer, M. Fulton, L. Davis and A. J. Rush, Stability of plasma GABA at four-year follow-up in patients with primary unipolar depression, Biol. Psychiatry, 1995, 37(11), 806–810, DOI:10.1016/0006-3223(94)00226-S.
- D. Dhossche, H. Applegate and A. Abraham,
et al., Elevated plasma gamma-aminobutyric acid (GABA) levels in autistic youngsters: stimulus for a GABA hypothesis of autism, Med. Sci. Monit., 2002, 8(8), PR1–PR6 CAS.
- A. J. Russo, Correlation between Hepatocyte Growth Factor (HGF) and Gamma-Aminobutyric Acid (GABA) Plasma Levels in Autistic Children, Biomark. Insights, 2013, 8, BMI.S11448, DOI:10.4137/BMI.S11448.
- J. S. Kim, W. Schmid-Burgk, D. Claus and H. H. Kornhuber, Increased serum glutamate in depressed patients, Arch. Psychiatr. Nervenkr., 1982, 232(4), 299–304, DOI:10.1007/BF00345492.
- C. Shimmura, S. Suda and K. J. Tsuchiya,
et al., Alteration of Plasma Glutamate and Glutamine Levels in Children with High-Functioning Autism, PLoS One, 2011, 6(10), e25340, DOI:10.1371/journal.pone.0025340.
- H. Mitani, Y. Shirayama, T. Yamada, K. Maeda, C. R. Ashby and R. Kawahara, Correlation between plasma levels of glutamate, alanine and serine with severity of depression, Prog. Neuropsychopharmacol. Biol. Psychiatry, 2006, 30(6), 1155–1158, DOI:10.1016/J.PNPBP.2006.03.036.
- M. Matsumoto, T. Ooga, R. Kibe, Y. Aiba, Y. Koga and Y. Benno, Colonic Absorption of Low-Molecular-Weight Metabolites Influenced by the Intestinal Microbiome: A Pilot Study, PLoS One, 2017, 12(1), e0169207, DOI:10.1371/journal.pone.0169207.
- E. Barrett, R. P. Ross, P. W. O'Toole, G. F. Fitzgerald and C. Stanton, γ-Aminobutyric acid production by culturable bacteria from the human intestine, J. Appl. Microbiol., 2012, 113(2), 411–417, DOI:10.1111/j.1365-2672.2012.05344.x.
- P. Strandwitz, K. H. Kim and D. Terekhova,
et al., GABA-modulating bacteria of the human gut microbiota, Nat. Microbiol., 2018, 4(3), 396, DOI:10.1038/s41564-018-0307-3.
|
This journal is © The Royal Society of Chemistry 2022 |