DOI:
10.1039/D2FO01161A
(Paper)
Food Funct., 2022,
13, 8829-8849
The different effects of psyllium husk and orlistat on weight control, the amelioration of hypercholesterolemia and non-alcohol fatty liver disease in obese mice induced by a high-fat diet†
Received
29th April 2022
, Accepted 17th July 2022
First published on 18th July 2022
Abstract
Obesity is a widespread medical problem, for which many drugs have been developed, each with its own limitations. Orlistat, a lipase inhibitor, functions as a fat absorption blocker and is a widely used over-the-counter drug in China. Psyllium husk, in contrast, is a food source rich in dietary fibre and is beneficial for weight loss because it reduces appetite. Here, it was investigated how psyllium husk treatments affect mice with a high-fat diet (HFD)-induced obesity, using obesity-related indices, metabolism indices, and gut microbiota, compared to orlistat treatments. Orlistat had a greater effect on weight loss, whereas psyllium husk had a greater effect at reducing serum and liver cholesterol and triglyceride levels. Treatments had similar effects on controlling the body fat rate, the expression level of farnesoid X receptor, sterol 27-hydroxylase and oxysterol 7-hydroxylase (CYP7B1) in the liver, and the regulation of major bile acids such as cholic acid, chenodeoxycholic acid, deoxycholic acid, and lithocholic acid in faecal content. However, the expression of CYP7A1 in the liver and the structures of faecal bile acids were different between the two drugs. Furthermore, although they also had similar effects on the gut microbiota at the phylum level, there were differences at the genus level for Roseburia, Bacteroides, Faecalibacterium, Coprobacillus, and Akkernansia, which led to the difference in the serum lipopolysaccharide (LPS) level. Orlistat increased the food intake of the obese mice that were fed a HFD, which led to an increase in water intake, serum triglyceride levels, and lower glucose tolerance. Although orlistat is considered a suitable drug for weight loss, psyllium husk is a comparatively more cost-effective choice for ameliorating hypercholesterolemia and non-alcoholic fatty liver disease caused by a HFD.
Introduction
Obesity is a metabolic disease that results from the excessive accumulation of body fat. When the intake of calories by a body is greater than its internal consumption, excess calories are stored as fat, and this leads to obesity if it exceeds normal physiological requirements. The prevalence of obesity has doubled since 1980.1 Obesity is closely related to diseases of the respiratory, cardiovascular, and immune systems.2–4 Popular drugs used to treat obesity fall into three categories: appetite inhibitors, metabolic stimulants, and nutrition absorption inhibitors. Examples include phentermine, an appetite suppressant, and topiramate, an antiepileptic, which have both been approved to treat obesity by the US Food and Drug Administration (FDA);5,6 however, they can have significant neurorelated side effects.7 Phenylpropanolamine, a metabolic stimulant, is used to treat obesity in the US; however, it is shown to increase the risk of haemorrhagic stroke.8 Orlistat, a nutrition absorption inhibitor, is available worldwide as an obesity treatment. However, it can have adverse effects such as oily stools and abdominal pain.9 Despite this, orlistat is the only over-the-counter drug approved by the National Medical Products Administration in China to treat obesity and was therefore used as a positive control drug in this study.
Psyllium husk (Plantago ovata) is rich in soluble dietary fibre and believed to effectively ameliorate obesity.10 Research showed that psyllium husk reduced serum total cholesterol11 and low-density lipoprotein cholesterol (LDL-C) levels;12 however, the mechanism by which psyllium husk ameliorates serum cholesterol remains unclear. The gut microbiota is closely related to obesity, as it is often accompanied by gut dysbiosis.13 Rebalancing of the intestinal microbiota can help to treat obesity.14 Dietary fibre is proved to be beneficial for those with obesity, as it has prebiotic characteristics that affect the gut microbiota.15 Our preliminary findings showed that both psyllium husk and orlistat aided in weight loss when combined with diet control and increased exercise. However, many obese patients had difficulty controlling their diet and did not want to exercise. The preferred option of the patients was to lose weight by taking medication alone.
Therefore, in this study, our aim was to investigate similarities and differences in weight control between psyllium husk and orlistat while not controlling eating or exercise patterns and maintaining a high-fat diet (HFD). We also aimed to observe the effects of the two drugs on NAFLD and serum obesity-related indicators in high-fat diet-induced obese mice. Furthermore, we explored the mechanism of ameliorating serum and liver cholesterol by the two drugs and observed the effects of psyllium husk and orlistat on the gut microbiota, as well as bile acid metabolomics, from the perspective of the gut microbiota in obese mice.
Our study provides an experimental basis to treat obesity, hypercholesterolemia, and NAFLD.
Experimental
Drugs and reagents
Psyllium husk [origin: India; execution standard: Q/MBBL 0006S; Leesmum (Shanghai) Industrial Development Co., Ltd, Shanghai, China] was obtained for this investigation and its nutritional information is listed in Table 1. Psyllium husk was ground into a powder using a grinder (Wiltal® type: MG-300). Approximately 200 g psyllium husk powder samples were kept in a cool (25 °C) dry room at the Science and Technology Innovation Center, Guangzhou University of Chinese Medicine, Guangzhou, China. The powder was dissolved in distilled water at a concentration of 20 mg mL−1 before it was given to the mice by gavage. Orlistat (0.12 g per capsule; execution standard: YBH01682014; Lunan New Time Bio-Tech Co., Ltd, Linyi China) was dissolved in distilled water at a concentration of 12 mg mL−1. High-fat diet (HFD) material (Research Diets® type: D12492; Research Diets, Inc.) and low-fat diet (LFD) material (Research Diets® type: D12450J; Research Diets, Inc.) were also obtained for this investigation.
Table 1 Nutritional information
|
Per 100 g |
Energy |
795 kJ |
Protein |
2.2 g |
Total fat |
0.9 g |
Carbohydrate |
3.7 g |
Dietary fiber |
82.7 g |
Sodium |
83 mg |
Animal experiments
The experiments were approved by the Guangzhou University of Traditional Chinese Medicine Animal Care and Use Committee (no. 20190621003) and all experiments were in accordance with the ARRIVE guidelines.16 Six-week-old male specific pathogen-free (SPF) C57BL/6J mice were purchased from the Guangzhou University of Traditional Chinese Medicine Animal Experiment Center (Guangzhou, China).
Mice were kept in a 12 h light/dark cycle at 26 °C. Six mice were kept in a cage (cage dimensions: 380 mm × 160 mm × 180 mm). The experiments were conducted in a SPF environment. A total of 36 mice were randomly divided into two groups, a normal control group (NC, n = 6) that received a LFD, and the obesity modelling group (OM, n = 30), which was fed a HFD. The modelling phase lasted 16 weeks. In the OM group, mice whose body weight was less than 120% of the average body weight in the NC group were classified as failed models and excluded from the trial. Eighteen mice in the OM group were successfully modelled and randomly divided into three treatment groups: model control group (MC, n = 6), HFD + psyllium husk group (HFD + PH, n = 6), and HFD + orlistat group (HFD + orlistat, n = 6). During the intervention phase, the NC group received a LFD, whereas obese mice in the MC group received a HFD, obese mice in the HFD + PH group received a HFD and were treated with psyllium husk, and obese mice in the HFD + orlistat group received a HFD and were treated with orlistat. The six mice in each group were kept in a cage (cage dimensions: 380 mm × 160 mm × 180 mm), but during weeks 16 and 25, each mouse from each group was moved to an individual cage (cage dimensions: 200 mm × 200 mm × 170 mm) to record their food and water intake. Psyllium husk has beneficial effects when administered in higher doses with sufficient water.17 However, the husk can expand when mixed with water, and at high concentration, would block the gavage needle. Therefore, in this experiment, a dose of 0.14 g kg−1 psyllium husk was provided three times a day (approximately the lowest recommended daily fibre supplement is 20 g per 50 kg for an adult)17 for obese mice. According to Choi et al.,18 obese mice were administered 20 mg kg−1 d−1 orlistat. All groups were treated in the intervention phase, as shown in Table 2.
Table 2 Times when the corresponding drugs were administered by gavage
Group |
Time |
9:00–9:30 |
13:00–13:30 |
17:00–17:30 |
NC |
Normal saline (10 mL kg−1) |
Normal saline (10 mL kg−1) |
Normal saline (10 mL kg−1) |
MC |
Normal saline (10 mL kg−1) |
Normal saline (10 mL kg−1) |
Normal saline (10 mL kg−1) |
HFD + PH |
Psyllium husk (0.14 g kg−1) |
Psyllium husk (0.14 g kg−1) |
Psyllium husk (0.14 g kg−1) |
HFD + orlistat |
Orlistat (20 mg kg−1) |
Normal saline (10 mL kg−1) |
Normal saline (10 mL kg−1) |
Detection of Lee's index and body fat rate
Lee's index was used to assess the level of obesity, which included body length and weight in the calculation.19 A mouse was placed inside a 500 mL beaker then placed on an electronic scale to record the weight. The length of each mouse was determined using a transparent ruler on the ventral surface to record the distance from the tip of the nose to the anus. Body weight and length were measured three times. Then, Lee's index was used to assess obesity levels as follows: Lee's index = 1000 × [body weight (g)](1/3)/body length (cm).19 During experimental studies on obese mice, the weight of white adipose tissue near the epididymis was used to reflect visceral fat weight.20 We used the weight of white fat on the left side of the epididymis to reflect the weight of visceral fat throughout the body. Body fat rate = [(white fat on the left side of the epididymis) (g)/body weight (g)] × 100%.
Faeces sample collection for 16S rDNA examination and bile acid-targeted metabolomics detection
Mice faeces were collected on the last two days of week 26 of treatment. Briefly, we massaged the abdomen and pulled the tail gently to stimulate defecation. The faeces were then collected in a 2 mL cryopreservation tube and stored at −80 °C.
Assaying a sample for 16S rDNA and sequencing
A QIAamp DNA Stool Mini Kit was used to process the faecal samples. Amplification of the V4 region of the bacterial 16S rDNA was conducted by APTBIO Co., Ltd (Shanghai, China) for sequencing with the Illumina Hiseq 2500 platform.
Determination of bile acids in the faeces
The faecal samples were sent to APTBIO Co., Ltd, Shanghai, China, where standard targeted metabolomics analysis operations (ESI 1†) were performed to determine the bile acids in the faeces.
Glucose tolerance test (GTT)
During the experimental period, we measured the fasting blood glucose levels of the mice during week 28 using an Omron glucometer [type: isens 631-A, execution standard: ISO 15197:2013, OMRON Healthcare (China) Co., Ltd] and Omron glucose strips. According to the methods of Andrikopoulos et al.,21 we obtained approximately 0.5 μL of blood from the tail vein after 6 h of fasting, after which the mice were intraperitoneally administered 2 g kg−1 glucose [kinvita, execution standard: GB/T20880, Kinvita (Fujian) Food Co., Ltd, China)]. Blood glucose levels were measured 30, 60, 90, and 120 min after intraperitoneal glucose injection.
Blood sample collection
On day 196 (day 84 of the treatment), the mice were fasted for 16 h without restricting their access to water overnight. The mice were then anaesthetised by intraperitoneal injection of sodium pentobarbital (50 mg kg−1) and after 30 min, blood was obtained from the eyeball of each mouse. Blood was centrifuged at 3000g for 15 min and the serum was obtained.
Blood sample assay
The TC, triglyceride (TG), high-density lipoprotein cholesterol (HDL-C), low-density lipoprotein cholesterol (LDL-C), lipopolysaccharide (LPS), and alanine aminotransferase (ALT) concentrations in the serum were estimated using commercially available kits (Nanjing Jiancheng Bioengineering Institute, China) according to the manufacturer's instructions, using a microplate reader [Multiskan Mk3, Thermo Fisher Instrument Co., Ltd, Shanghai].
Liver sample assay
The TC and TG concentrations in the liver were estimated using commercially available kits (Nanjing Jiancheng Bioengineering Institute, China), similar to that used in the blood assay samples. The liver samples were sent to APTBIO Co., Ltd, Shanghai, China. Two samples from the same group were randomly selected and mixed. Standard tandem mass tag analysis operations (ESI 2†) were conducted to determine the proteins in the liver. The protein levels of FXR, CYP7A1, CYP7B1, sterol 12-hydroxylase (CYP8B1) and CYP27A1, which were closely associated with liver bile acid metabolism, were selected from the results for further analysis.
Detection of fat mass and body fat rate
After blood sample collection, the mice were placed abdomen up and fixed on the operating table and we disinfected their abdomens with 75% alcohol. After lifting the central skin of the abdomen with tweezers, scissors were used to cut the skin and muscle layers from the top down to expose the internal organs. A large amount of white paraepididymal adipose tissue was seen in the deep groin of the mice. We held the left paraepididymal adipose tissue with tweezers and then separated the other tissue attached to the left paraepididymal adipose tissue with scissors. The left paraepididymal adipose tissue was weighed to represent the visceral fat content of the entire body. Body fat rate = 100% × weight of left paraepididymal adipose tissue (g)/body weight (g).
Histological analysis
To collect liver tissue from the mice, each liver was laid flat on a piece of tinfoil and placed in a specimen box, tinfoil side down. The samples were stored at −80 °C until further use. The liver tissue was then placed on a cryomicrotome (CM3050 S, Leica, Germany) and sliced at a thickness of 10–20 μm. The liver slices were washed with distilled water before being treated with hematoxylin for 1 min. The liver slices were then washed with tap water before the cells were differentiated with 0.5% hydrochloric acid in ethanol solution until the nuclei turned blue. Liver slices were immersed in Sudan III staining solution for 30 min and then differentiated with 70% ethanol for several seconds. Liver slices were immersed in distilled water, washed gently, and placed on a glass slide with a curved hook. Finally, the liver slices were sealed with glycerine gelatine after drying. Pathological sections were randomly numbered and sent to the Pathology Teaching and Research Department of the Guangzhou University of Chinese Medicine. Images were also taken of each pathological section from random angles, and the optical density (OD) value of each image was assessed using the pathological image analysis software Image Pro Plus (version 6.0).
Statistical analysis
The results were analysed using SPSS 23 software and data were expressed as mean ± standard error of the mean (s.e.m.). When data sets were normally distributed with a satisfactory homogeneity of variance, the P values were determined using one-way analysis of variance (ANOVA) with Fisher's least significant difference analysis. Otherwise, the P values were determined using the Kruskal–Wallis test. Differences were considered statistically significant at P < 0.05.
Results
The psyllium husk did not result in significant weight gain, whereas orlistat reduced body weight
During the experimental period, all mice gained weight and their weekly body weight measurements are shown in Fig. 1a. There was a significant difference (P = 0.002) between the NC group and the other three groups after week 3; whereas in the interfering phase, there was a significant difference between the MC group and the two intervention groups from week 19 (Fig. 1a). The body weight of the mice in the NC group increased significantly with natural weight gain during the interference phase (1.09-fold; P = 0.002, Fig. 1b), as did the body weight of those in the MC group when fed a HFD (1.16-fold; P < 0.001, Fig. 1b). Although the body weight of the mice in the HFD + PH group did not show a significant change (0.98-fold; P = 0.329, Fig. 1b), those in the HFD + orlistat group decreased significantly (0.90-fold; P = 0.039, Fig. 1b).
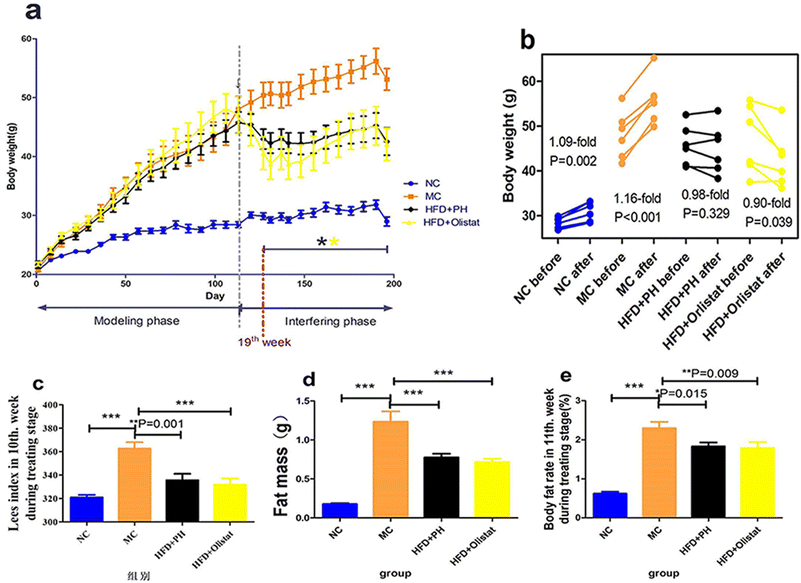 |
| Fig. 1 HFD increased body weight and related indexes and this was ameliorated by psyllium husk and orlistat therapies. (a) Weight changes during the experiment. (b) Body weight before and after therapy. (c) Lee's index at week 10 during the treatment phase. (d) Left paraepididymal fat weight (fat mass) in week 11 during the treatment stage. (e) Body fat rate at week 11 during the treatment stage. The P values were determined by paired-sample T test in (b). The P values in the other panels were determined by one-way analysis of variance (ANOVA) with Fisher's least significant difference analysis. Data are presented as mean ± s.e.m. n = 6 for each group. *: P < 0.05 vs. the MC group. **: P < 0.01 vs. the MC group. ***: P < 0.001 vs. the MC group. | |
Psyllium husk and orlistat had similar effects on fat mass, body fat rate, and Lee's index
The left paraepididymal fat weight (evaluating the fat mass), body fat rate, and Lee's index of the obese mice were significantly higher than in the NC group (NC and MC group, P < 0.001, Fig. 1c; P < 0.001, Fig. 1d; P < 0.001, Fig. 1e). Compared to the MC group, psyllium husk and orlistat significantly ameliorated fat mass, body fat rate, and Lee's index [(HFD + PH and MC groups, P < 0.001, Fig. 1c; P = 0.015, Fig.1d; P = 0.001, Fig. 1e) (HFD + orlistat and MC group, P < 0.001, Fig. 1c; P = 0.009, Fig. 1d; P < 0.001, Fig. 1e)].
Psyllium husk and orlistat had similar initial effects on food intake, but differed during intervention
The weekly food intake of each group during the intervention phase is shown in Fig. 2a. We observed the weekly food intake of each mouse in the final week of the modelling phase and in weeks 1 and 9 of the intervention phase (Fig. 2b). Weekly food intake did not differ significantly among the four groups during the final week of the modelling phase. In the first week of the intervention phase, compared to the MC group, the weekly food intake of the mice in the HFD + PH and HFD + orlistat groups was significantly reduced [(MC and HFD + PH group, P < 0.001, Fig. 2b); (MC and HFD + orlistat group, P < 0.001, Fig. 2b)]. In week 9 of the intervention phase, compared with the MC group, the weekly food intake of the mice in the HFD + PH group was significantly reduced (MC and HFD + PH group, P = 0.049, Fig. 2b), whereas that in the HFD + orlistat group was significantly increased (MC and HFD + orlistat group, P < 0.001, Fig. 2b). Changes in food intake before and after treatment are shown in Fig. 2c. As the body weight of the mice in the NC group increased naturally, so did their food intake (1.07-fold; P = 0.042, Fig. 2c). The weekly food intake of the mice in the MC group did not change significantly after the treatment phase (1.02-fold; P = 0.607, Fig. 2c), whereas that of the mice in the HFD + PH group was significantly reduced (0.96-fold; P = 0.034, Fig. 2c), and the weekly total energy intake (energy offered by the HFD was counted in week 16 of the modelling phase; energy offered by the HFD and psyllium husk was counted in week 9 of the interfering phase) reduced after treatment with the psyllium husk (0.97-fold; P = 0.055, Fig. 2d). The weekly food intake of the mice in the HFD + orlistat group increased significantly after orlistat treatment (1.27-fold; P = 0.001; Fig. 2c).
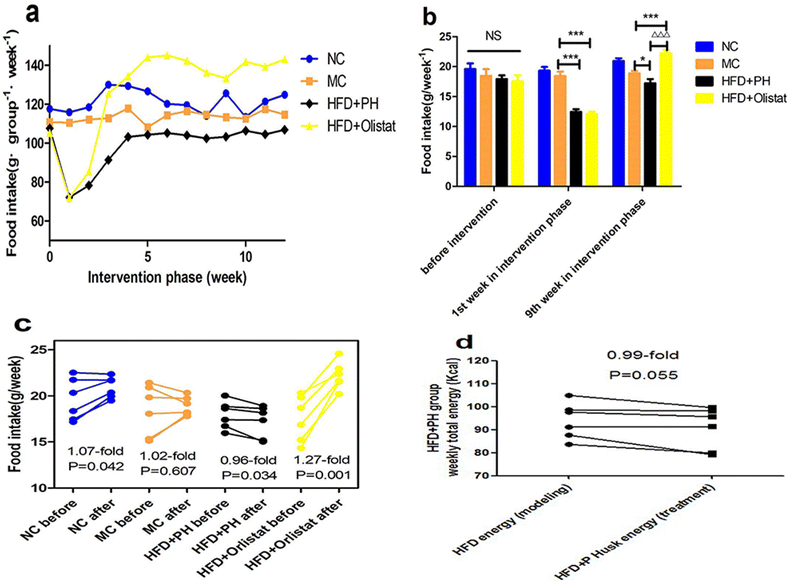 |
| Fig. 2 Weekly food intake decreased with psyllium husk and increased with orlistat. (a) Weekly total food intake for each group during the experiment (n = 6 in each group). (b) Weekly food intake before intervention and in weeks 1 and 9 of the intervention phase. (c) Food intake before and after treatment for each group. (d) Weekly total energy intake for the HFD + PH group before and after treatment. For (a), data are presented as the total number, and n = 6 for each group. For (c), the P values were determined using a paired-sample T test. For (b) and (d), the P values were determined by one-way analysis of variance (ANOVA) with Fisher's least significant difference analysis. For (b), (c), and (d), data are presented as mean ± s.e.m. n = 6 for each group. NS: no significant difference. *: P < 0.05 vs. MC group. Δ: P < 0.05 vs. HFD + orlistat group ΔΔΔ: P < 0.001 vs. HFD + orlistat group. | |
Psyllium husk and orlistat had similar effects on autonomous water intake initially, but differed in the intervention phase
The weekly autonomous water intake of each group during the intervention phase is shown in Fig. 3a. In the final week of the modelling phase and weeks 1 and 9 of the intervention phase, the autonomous water intake for each mouse was recorded (Fig. 3b). The weekly autonomous water intake was not significantly different among the four groups in the last week of the modelling phase or in the first week of the intervention phase. In the last week of the intervention phase, compared with the other three groups, it was significantly increased in the HFD + orlistat group [(NC and HFD + orlistat group, P < 0.001, Fig. 3b), (MC and HFD + orlistat group, P < 0.001, Fig. 3b), (HFD + PH and HFD + orlistat group, P < 0.001, Fig. 3b)]. The changes in autonomous water intake before and after intervention are shown in Fig. 3c. In the NC, MC, and HFD + PH groups, the amount of weekly autonomous water intake showed no significant differences before and after intervention [(NC group, 0.96-fold; P = 0.276, Fig. 3c); (MC group, 0.95-fold; P = 0.148, Fig. 3c); (HFD + PH group, 0.99-fold; P = 0.391, Fig. 3c)]. The weekly autonomous water intake for mice in the HFD + orlistat group increased significantly (1.24-fold; P = 0.002, Fig. 3c) after orlistat treatment.
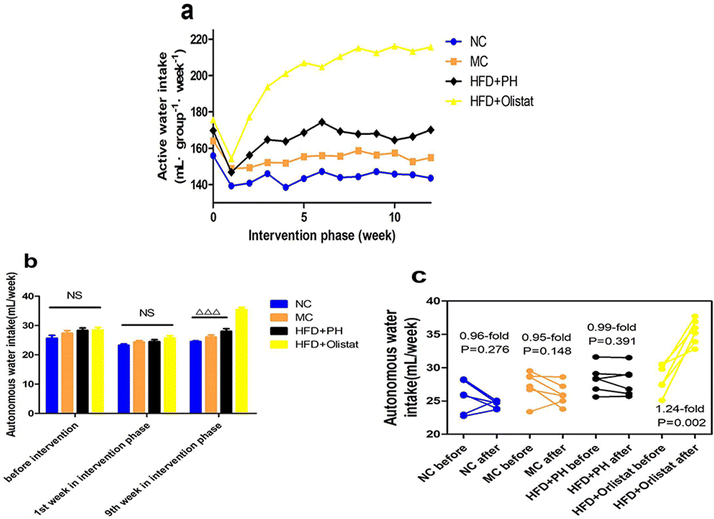 |
| Fig. 3 Orlistat increased water intake. (a) Total weekly water intake for each group during the experiment (n = 6 in each group). (b) Weekly water intake before intervention and at weeks 1 and 9 during the intervention phase. (c) Water intake before and after treatment for each group. For (a), data are presented as the total number. n = 6 for each group. For (b), the P values were determined by one-way analysis of variance (ANOVA) with Fisher's least significant difference analysis. For (c), the P values were determined by the paired-sample T test. For (b) and (c), data are presented as mean ± s.e.m. n = 6 for each group. NS: no significant difference. *: P < 0.05 vs. MC group. ΔΔΔ: P < 0.001 vs. HFD + orlistat group. | |
Psyllium husk reduced the level of TC and TG in the liver
We estimated the severity of NAFLD by comparing the liver cholesterol, liver triglyceride, and liver Sudan III staining OD values of the MC group with the NC and HFD + PH groups, and found them to be significantly lower [(NC and MC groups, P < 0.001, Fig. 4a; P < 0.001, Fig. 4b; P < 0.001, Fig. 4c), (HFD + PH and MC group, P < 0.001, Fig. 4a; P = 0.015, Fig. 4b; P = 0.006, Fig. 4c)]. The HFD + orlistat group were inclined to lower liver cholesterol and liver Sudan III staining OD values (HFD + orlistat and MC group, P = 0.058, Fig. 4a; P = 0.097, Fig. 4c). Compared to orlistat, psyllium husk had a better effect on the reduction of liver cholesterol (HFD + PH and HFD + orlistat group, P < 0.001, Fig. 4a) and on the reduction of liver triglyceride and liver Sudan III staining OD values (HFD + PH and HFD + orlistat group, P = 0.117, Fig. 4b; P = 0.097, Fig. 4c). The relative results of Sudan III liver staining are shown in Fig. 4d.
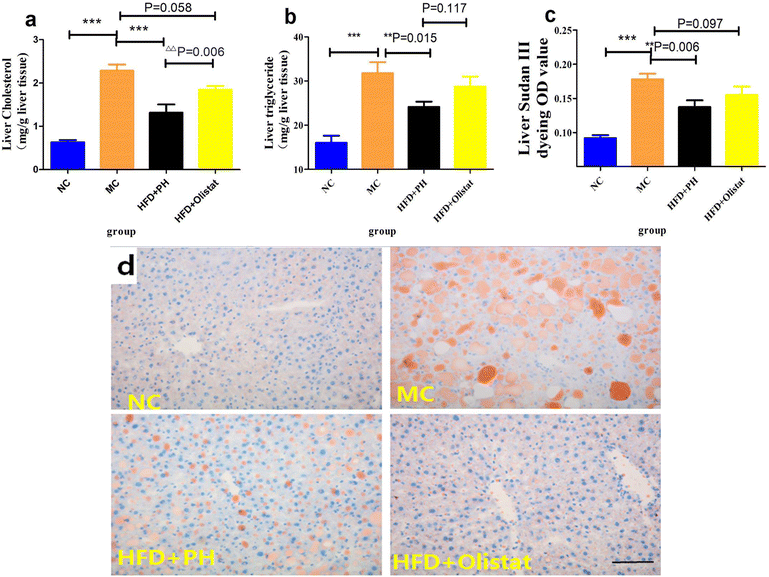 |
| Fig. 4 Psyllium husk reduced the levels of liver cholesterol and triglyceride. (a) Liver cholesterol and (b) liver triglyceride levels. (c) The OD values of liver sections stained with Sudan III. (d) Representative images of liver sections stained with Sudan III. The total magnification is 200×; scale bar = 100 μm. P values were determined by one-way analysis of variance (ANOVA) with Fisher's least significant difference analysis. Data are presented as mean ± s.e.m. n = 6 for each group. **: P < 0.01 vs. the MC group. ***: P < 0.001 vs. the MC group. ΔΔ: P < 0.01 vs. HFD + orlistat group. | |
Psyllium husk and orlistat had similar effects on serum ALT levels and LDL/HDL ratio, but their LPS, TC, and TG levels differed
Obese mice had higher serum ALT, LPS, and TC levels as well as the LDL/HDL ratio than mice in the NC group (NC and MC groups, P < 0.001, Fig. 5a; P < 0.001, Fig. 5b; P < 0.001, Fig. 5d; P < 0.001, Fig. 5c). Compared to the MC group, psyllium husk and orlistat significantly ameliorated serum ALT, LPS, and TC levels as well as the LDL/HDL ratio [(HFD + PH and MC groups, P < 0.001, Fig. 5a; P < 0.001, Fig. 5b; P < 0.001, Fig. 5d; P < 0.001, Fig. 5c) (HFD + orlistat and MC group, P < 0.001, Fig. 5a; P < 0.001, Fig. 5b; P = 0.005, Fig. 5d; P < 0.001, Fig. 5c)]. Mice fed a HFD were inclined to have a higher serum TG level (NC and MC group, P = 0.065, Fig. 5e); however, when obese mice were treated with orlistat, serum TG levels increased significantly [(NC and HFD + orlistat group, P = 0.003, Fig. 5e); (HFD + PH and HFD + orlistat group, P = 0.015, Fig. 5e)]. Psyllium husk was more effective at decreasing serum LPS and serum TC than orlistat (HFD + PH and HFD + orlistat group, P = 0.015, Fig. 5b; P = 0.070, Fig. 5d).
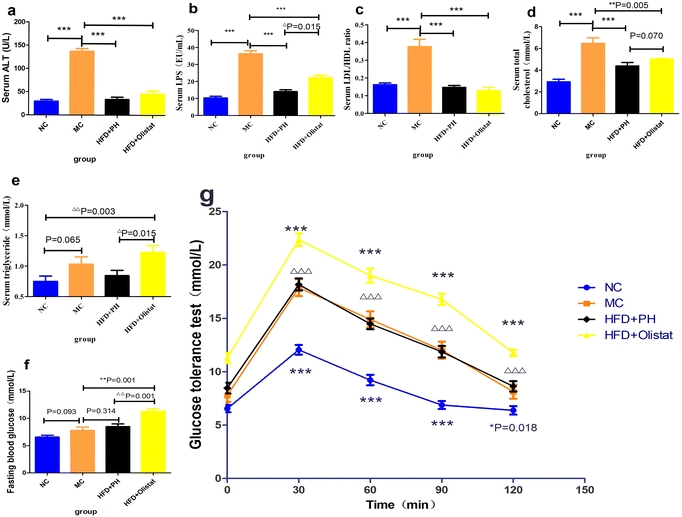 |
| Fig. 5 Psyllium husk and orlistat reduced the levels of serum ALT, TC, and LDL/HDL, but had different effects on serum TG and GTT. The serum (a) ALT, (b) LPS, (c) LDL/HDL, (d) cholesterol, and (e) triglyceride levels. (f) The level of fasting blood glucose and the (g) glucose tolerance test (GTT) results. P values were determined by one-way analysis of variance (ANOVA) with Fisher's least significant difference analysis. Data are presented as mean ± s.e.m. n = 6 for each group. **: P < 0.01 vs. MC group. ***: P < 0.001 vs. MC group. Δ: P < 0.05 vs. HFD + orlistat group ΔΔ: P < 0.01 vs. HFD + orlistat group ΔΔΔ: P < 0.001 vs. HFD + orlistat group. | |
Psyllium husk or orlistat could not ameliorate glucose tolerance
Fasting blood glucose levels in HFD-induced mice increased (NC and MC groups, P = 0.093, Fig. 5f). Psyllium husk did not significantly affect fasting blood glucose levels (HFD + PH and MC groups, P = 0.341, Fig. 5f); however, orlistat significantly increased fasting blood glucose levels (HFD + PH and MC groups, P < 0.001, Fig. 5f). The results of the GTT for the mice are shown in Fig. 5g. Similar to the fasting blood glucose, the MC group had a higher blood glucose 30, 60, 90, and 120 min after being given glucose than the NC group (NC and MC group, P < 0.05, Fig. 5g). Compared to the MC group, psyllium husk did not significantly influence blood glucose at 30, 60, 90, and 120 min after glucose administration (HFD + PH and MC group, P > 0.05, Fig. 5g). Orlistat significantly increased blood glucose levels at 30, 60, 90, and 120 min after glucose administration (HFD + orlistat and MC group, P < 0.05, Fig. 5g), and was significantly higher than the HFD + PH group (HFD + PH and HFD + orlistat group, P < 0.05, Fig. 5g).
Psyllium husk and orlistat had similar effects on the expression levels of FXR, CYP8B1, CYP27A1, and CYP7B1 in the liver, but their CYP7A1 levels differed
Obese mice have lower CYP27A1 and CYP7B1 expression levels than those in the NC group (NC and MC groups, P = 0.109, Fig. 6c; P = 0.093, Fig. 6d). Psyllium husk and orlistat significantly upregulated CYP27A1 and CYP7B1 expression levels [(HFD + PH and MC groups, P = 0.003, Fig. 6c; P = 0.018, Fig. 6d) (HFD + orlistat and MC group, P = 0.027, Fig. 6c; P = 0.021, Fig. 6d)]. Psyllium husk and orlistat downregulated FXR expression levels [(HFD + PH and MC groups, P = 0.014, Fig. 6e), (HFD + orlistat and MC group, P = 0.069, Fig. 6e)]. Mice in the HFD + orlistat group had a lower level of CYP7A1 expression than those in the HFD + PH group (HFD + orlistat and HFD + PH group, P = 0.044, Fig. 6a).
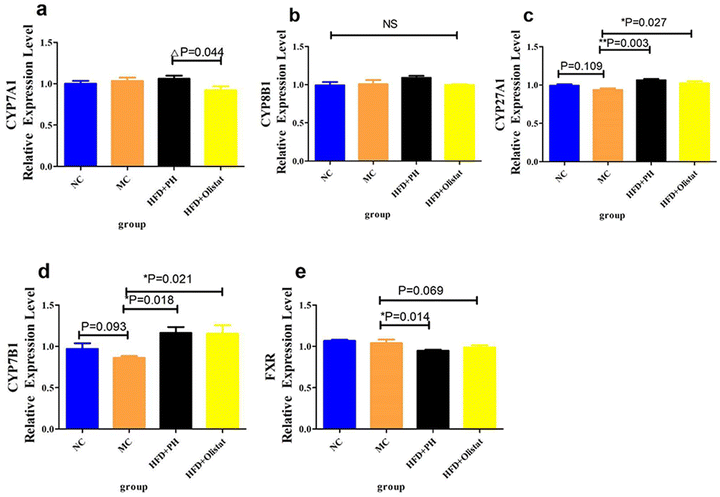 |
| Fig. 6 Psyllium husk and orlistat regulated the synthesis of bile acid. (a) CYP7A1, (b) CYP8B1, (c) CYP27A1, (d) CYP7B1, (e) and FXR tandem mass tag results. P values were determined via one-way ANOVA with Fisher's least significant difference analysis. Data are presented as mean ± s.e.m.; n = 3 for each group. NS: no significant difference. *: P < 0.05 vs. MC group. **: P < 0.01 vs. MC group. ***: P < 0.001 vs. MC group. Δ: P < 0.05 vs. HFD + orlistat group. | |
Psyllium husk and orlistat had different effects on alpha diversity in the faecal microbiota
We evaluated the effect of psyllium husk and orlistat on the gut microbiota of mice using alpha diversity analysis, and Shannon index analysis at the operational taxonomic unit (OTU) level was conducted to assess community diversity. The Shannon index showed that community diversity was lower in the HFD + orlistat group than in the other three groups [(NC and HFD + orlistat group, P = 0.057, Fig. 7a), (MC and HFD + orlistat group, P = 0.003, Fig. 7a), and (HFD + PH and HFD + orlistat group, P = 0.076, Fig. 7a)].
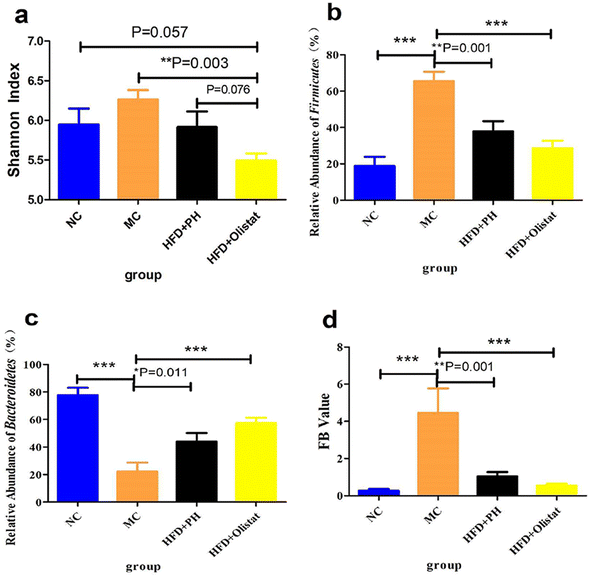 |
| Fig. 7 Psyllium husk and orlistat had different effects on the alpha diversity of the faecal microbiota and microbiota composition at the phylum level. (a) Shannon index to evaluate community diversity. The relative abundance of (b) Firmicutes and (c) Bacteroidetes. (d) The ratio of F/B. P values were determined by one-way ANOVA with Fisher's least significant difference analysis. Data presented as mean ± s.e.m. n = 6 for each group. *: P < 0.05 vs. MC group. **: P < 0.01 vs. MC group. ***: P < 0.001 vs. MC group. | |
Psyllium husk and orlistat had similar effects at the phylum level, but differed at the genera and species levels of the faecal microbiota
At the phylum level, after obesity was induced, the relative abundance of Firmicutes in the MC group increased significantly (NC and MC groups, P < 0.001, Fig. 7b), whereas the relative abundance of Bacteroidetes decreased significantly (NC and MC groups, P < 0.001, Fig. 7c). Therefore, the ratio of Firmicutes/Bacteroidetes (F/B) increased significantly in the MC group (NC and MC groups, P < 0.001, Fig. 7d). Psyllium husk and orlistat increased the relative abundance of Firmicutes [(HFD + PH and MC group, P = 0.001, Fig. 7b) and (HFD + orlistat and MC group, P < 0.001, Fig. 7b)] and Bacteroidetes [(HFD + PH and MC group, P = 0.011, Fig. 7c) and (HFD + orlistat and MC group, P < 0.001, Fig. 7c)], but decreased the F/B ratio [(HFD + PH and MC group, P = 0.001, Fig. 7d) and (HFD + orlistat and MC groups, P < 0.001, Fig. 7d)]. The relative abundances of Roseburia, Bacteroides, Faecalibacterium, Sutterella, Coprobacillus, and Akkermansia did not appear to be significantly different between the NC and MC groups. Psyllium husk and orlistat significantly increased the relative abundance of Sutterella [(HFD + PH and MC group, P = 0.008, Fig. 8a) (HFD + orlistat and MC group, P < 0.001, Fig. 8a)]. While Bilophila increased significantly in obese mice (NC and MC groups, P = 0.005, Fig. 8b), psyllium husk tended to downregulate the relative abundance of Bilophila (HFD + PH and MC group, P = 0.066, Fig. 8b), which differed from orlistat (HFD + PH and HFD + orlistat group, P = 0.066, Fig. 8b). After treatment with psyllium husk, the relative abundance of Roseburia, Bacteroides, Faecalibacterium, and Coprobacillus increased (HFD + PH and MC group, P = 0.096, Fig. 8c; P = 0.054, Fig. 8d; P = 0.033, Fig. 8e; P = 0.003, Fig. 8f). Unlike psyllium husk, orlistat significantly increased Akkermansia abundance (HFD + orlistat and MC group, P = 0.001, Fig. 8g). At the species level, we detected Parabacteroides distasonis, which is closely associated with obesity. Psyllium husk significantly elevated the relative abundance of Parabacteroides distasonis (HFD + PH and MC groups, P < 0.001, Fig. 8h) whereas there was no significant difference among the NC, MC, and HFD + orlistat groups.
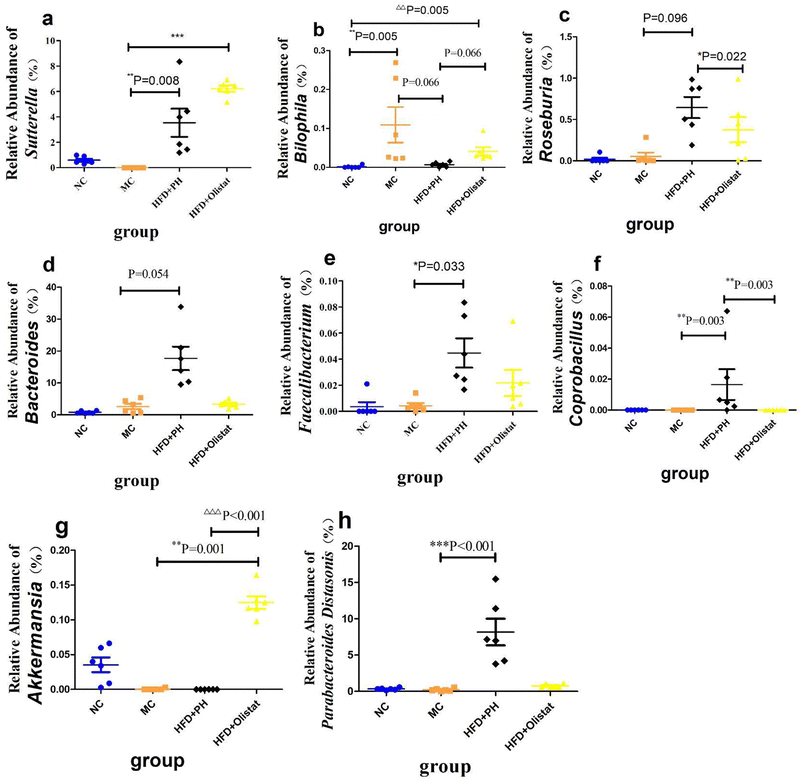 |
| Fig. 8 Psyllium husk and orlistat had different effects on obesity-related bacteria at the genus and species levels. Relative abundance of (a) Sutterella, (b) Bilophila, (c) Roseburia, (d) Bacteroides, (e) Faecalibacterium, (f) Coprobacillus, (g) Akkermansia, and (h) Parabacteroides distasonis. The P values were determined using the Kruskal–Wallis test. Each point represents an individual mouse, n = 6 for each group. *: P < 0.05 vs. MC group. **: P < 0.01 vs. MC group. ***: P < 0.001 vs. MC group. Δ: P < 0.05 vs. HFD + orlistat group. ΔΔ: P < 0.01 vs. HFD + orlistat group. ΔΔΔ: P < 0.001 vs. HFD + orlistat group. | |
Psyllium husk and orlistat had different effects on faecal bile acid composition, which was closely related to the gut microbiota and host metabolism parameters
The total faecal bile acid levels of HFD-induced obese mice increased significantly (MC and NC groups, 1.65-fold, P < 0.001, Fig. 9f). Orlistat significantly reduced total faecal bile acid levels (HFD + orlistat and MC group, 0.79-fold, P = 0.022, Fig. 9f), and psyllium husk further reduced total faecal bile acid levels [(HFD + PH and MC group, 0.56-fold, P < 0.001, Fig. 9f), (HFD + PH and HFD + orlistat group, 0.70-fold, P = 0.022, Fig. 9f)]. There was a significant difference between the NC and MC groups in the main types of bile acid detected (NC and MC groups, P < 0.05, Fig. 9a–e). After treatment with psyllium husk, CA, CDCA, DCA, LCA, and muricholic acid (MCA) levels decreased significantly in the faeces (HFD + PH and MC groups, P < 0.05, Fig. 9a–e). Orlistat significantly decreased the levels of CDCA and LCA (HFD + orlistat and MC group, P < 0.05, Fig. 9c and d). The CA, CDCA, DCA, and MCA levels in the faecal content of mice treated with psyllium husk were significantly lower than those of mice treated with orlistat (HFD + PH and HFD + orlistat group, P < 0.05, Fig. 9). The ratio of (CA + DCA)/(CDCA + LCA + MCA + HDCA + UDCA) increased significantly in the MC group compared with the NC group (MC and NC groups, P < 0.001, Fig. 9g). Psyllium husk significantly reduced the ratio (HFD + PH and MC group, P = 0.018, Fig. 9g), which differed significantly from orlistat (HFD + PH and HFD + orlistat group, P = 0.002, Fig. 9g). The results of Spearman's correlation analysis between obese related indexes, faecal bile acid (BA), and gut microbiota are shown in Fig. 10.
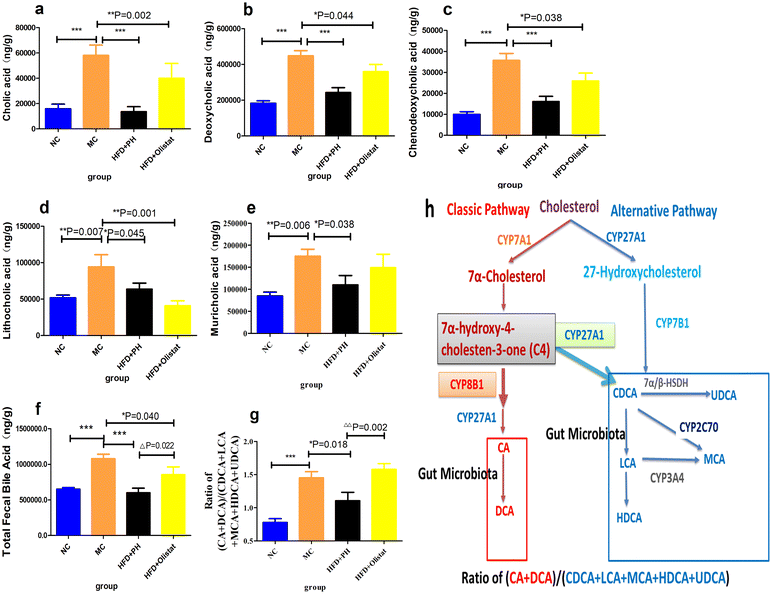 |
| Fig. 9 Psyllium husk and orlistat had different effects on the structure of faecal BA. (a) CA, (b) DCA, (c) CDCA, (d) LCA, (e) MCA, (f) faecal total BA, and (g) (CA + DCA)/(CDCA + LCA + MCA + HDCA + UDCA). P values were determined via one-way ANOVA with Fisher's least significant difference analysis. Data are presented as mean ± s.e.m. n = 6 for each group. *: P < 0.05 vs. MC group. ***: P < 0.001 vs. MC group. Δ: P < 0.05 vs. HFD + orlistat group. ΔΔ: P < 0.01 vs. HFD + orlistat group. (i) Schematic illustration of BA synthesis in the liver. The classical pathway is initiated by CYP7A1 and converts cholesterol into C4. CYP8B1 competes with CYP27A1 for C4. When the CYP8B1 level is increased or the CPY27A1 level is decreased, more C4 will react with CYP8B1 first and produce more CA. While when the CPY27A1 level is increased or the CYP8B1 level is decreased, more C4 will react with CPY27A1 first and produce more CDCA. The alternative synthesis pathway is initiated by CPY27A1 and converts cholesterol into 27-hydroxycholesterol. 27-Hydroxycholesterol reacts with CYP7B1 and produces CDCA. CDCA is converted into UDCA by 7α/β-HSDH, and MCA by CYP2C70 in the liver. LCA can be hydroxylated to HDCA and MCA in the liver. In the intestine, the gut microbiota converts CA into DCA, similar to CDCA to LCA. Therefore, the faecal ratio of (CA + DCA)/(CDCA + LCA + MCA + HDCA + UDCA) may reflect the expression of CYP8B1, CYP27A1 and CYP7B1 in the liver. | |
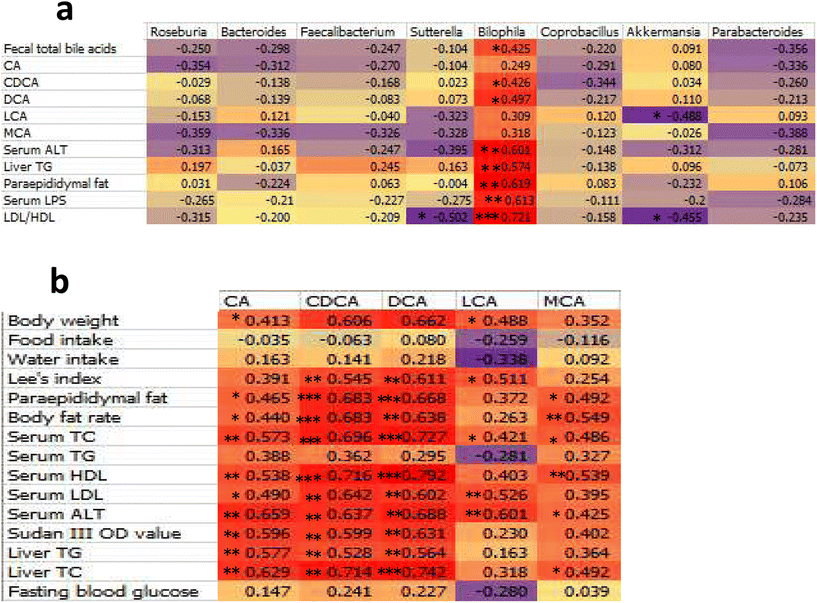 |
| Fig. 10 Heat map of the correlation analysis. (a) Correlations between obesity-related bacteria and faecal bile acid (BA), liver TG, paraepididymal fat, serum LDL/HDL, serum LPS, and serum ALT. (b) Correlations between BA and obesity-related metabolic parameters. Data represent the degree of correlation. The colour intensity indicates the degree of correlation (purple represents a negative correlation, whereas red represents a positive correlation). *: P < 0.05. **: P < 0.01. | |
Discussion
This study compared the effect of psyllium husk and orlistat on obese mice induced by a HFD. Orlistat had a greater effect on weight loss, whereas psyllium husk treatment resulted in a larger reduction in liver triglyceride and cholesterol levels. Orlistat impaired glucose tolerance in obese mice induced by a HFD, whereas psyllium husk did not. Both downregulated the expression of FXR and upregulated the expression of CYP27A1 and CYP7B1 in the liver; however, they had different effects on the expression of CYP7A1, which affected the metabolism of BA and led to differences in lipid absorption. The two drugs bound the BAs through different mechanisms, thus reducing BA reabsorption, which probably led to the change in the composition of faecal BAs and the difference in the gut microbiota, which led to the difference in serum LPS levels that had an influence on NAFLD.
We investigated the functional mechanisms of psyllium husk and compared them with orlistat as a means of weight control without diet restrictions or excessive exercise in mice. Currently, in obese mice models, ob gene modification or a HFD are predominately used.22,23 There are two main research strategies used with HFD models: (1) to supply a HFD while administering drug intervention; or (2) to feed a HFD for a period, then select animals that meet the criteria for an obesity model, followed by drug intervention. This study compared the effects of psyllium husk and orlistat on diet-induced obesity. Obese mice fed a HFD can survive to middle and old age (36–65 weeks of age) with relatively few deaths.24,25 However, ob/ob mice were found to have a significantly shorter life span and deaths occurred frequently over a six-month period;26 consequently ob/ob mice were not selected as the animal model for this study.
Using drug intervention as soon as mice are fed a HFD can increase uncertainty, as there may be obesity-resistant mice in each group; therefore, drug intervention was applied after the obese mouse model induced by a HFD was established.
Although psyllium husk did not significantly reduce the weight of HFD-fed mice, it maintained a comparatively stable weight in the mice, whereas orlistat significantly reduced the body weight of HFD-induced obese mice. From the perspective of weight loss, the effect of orlistat was superior to that of psyllium husk (Fig. 1a and b). HFD-induced obese mice had a Lee's index higher than the LFD-fed mice. Those treated with psyllium husk or orlistat had a lower Lee's index (P < 0.05, Fig. 1c). Compared to the MC group, mice treated with psyllium husk or orlistat had less white fat and a lower body fat ratio (P < 0.05, Fig. 1d and e). These results showed that psyllium husk and orlistat ameliorated HFD-induced obesity, but that orlistat was more effective for weight loss. Obese mice treated with psyllium husk did not show significant changes in water intake (0.99-fold, P = 0.391, Fig. 3c), whereas the food intake of the mice was significantly reduced (0.96-fold, P = 0.034, Fig. 2c). We considered whether the reduction in energy intake due to the reduction in the consumption of a HFD was like the reduction in energy provided by the intragastric administration of psyllium husk. After interference by psyllium husk, the weekly total energy intake (energy offered by psyllium husk and the HFD was counted) of the mice decreased (P = 0.055, Fig. 2d). Therefore, it was suspected that psyllium husk reduced the intake of the HFD via other mechanisms, such as expansion through interaction with water and satiety, rather than providing sufficient energy to replace the energy offered by the HFD. Furthermore, research and analysis are required to identify and confirm the probable mechanisms. Interestingly, in our study, orlistat significantly increased food intake (P = 0.001, Fig. 2c) and water intake (P = 0.002, Fig. 3c), indicating that mice treated with orlistat consumed more food to obtain enough energy. As the HFD contained lard, protein, and sucrose, we suggest that the mice absorbed increased levels of protein and sucrose to compensate for fat malabsorption. Excess sucrose is hydrolysed by the gut, transformed into monosaccharides, and finally absorbed by the small intestine. The increase in monosaccharide concentrations in plasma increases crystalloid osmotic pressure, which stimulates the osmotic pressure receptor of the extracellular fluid,27 and the signal is transmitted to the thirst centre.28 In addition, due to fat diarrhoea induced by orlistat (Fig. S1†), mice consumed high levels of food to replenish their electrolytes and increased their water intake to hydrate their bodies.
We examined serum glycolipid metabolism-related markers and found that obese mice fed a HFD had higher fasting blood glucose levels than mice fed a LFD. The psyllium husk did not reduce fasting blood glucose levels when compared to the MC group. It remains unknown whether psyllium husk ameliorates type II diabetes. Our results support the hypothesis that psyllium husk does not significantly affect fasting blood glucose. The substance–disease relationship between psyllium husk and type II diabetes remains controversial.29 Interestingly, the HFD + orlistat group had the highest fasting blood glucose levels among the four groups (P < 0.05, Fig. 5f). Similar to fasting blood glucose, mice in the MC and HFD + PH groups had a lower glucose tolerance than those in the NC group. The HFD + orlistat group had the lowest glucose tolerance among the four groups (P < 0.05, Fig. 5g). As mentioned previously, orlistat made it difficult for fat in the HFD to be absorbed, producing an effect similar to that of a high-sucrose diet. Other studies previously found that rats fed a high-sucrose diet had a lower glucose tolerance than those fed a standard chow diet.30 Moreover, a high-sucrose diet does not induce obesity.31 Although the food intake of mice in the orlistat + HFD group increased (P = 0.001, Fig. 2c), the body weight of mice in the orlistat group decreased (P = 0.039, Fig. 1b). This was partly reflected by orlistat reducing the amount of fat absorbed from the HFD, which caused mice to consume more sugar to maintain their energy balance. As expected, mice in the MC group had higher serum TC and TG levels than those in the NC group (P < 0.05, Fig. 5d and e). Psyllium husk and orlistat significantly reduced TC levels (P < 0.05, Fig. 5d). Mice in the MC group had a higher Vander's human physiology level than those in the NC group (P = 0.065, Fig. 5e). Psyllium husk did not significantly change the serum TG level. Compared to the NC and HFD + PH group, the TG of mice in the HFD + orlistat group increased significantly (P < 0.05, Fig. 5e), indicating glucose and lipid metabolism disorders in mice. We referred to related research and found this situation was like that of mice fed a high-sucrose diet,30 which supported our suggestion that a HFD along with orlistat produced an effect similar to that of a high-sucrose diet. Elevated LDL-C or low HDL-C levels indicate dyslipidemia.32 The study showed that the LDL/HDL cholesterol ratio could be used to evaluate NAFLD better than a single lipoprotein.33 The LDL/HDL ratio was elevated in the MC group, and intervention with psyllium husk and orlistat decreased the ratio of LDL/HDL (P < 0.001, Fig. 5c), and the ratio was positively correlated with the severity of NAFLD (R = 0.493, P = 0.014).
The results showed that the liver cholesterol, liver triglyceride, and OD values of the liver Sudan III-stained sections of the MC group were significantly higher than those of the NC group (P < 0.001, Fig. 4a–c). Compared to the MC group, psyllium husk significantly reduced the OD value (P = 0.006, Fig. 4c) whereas with orlistat, the OD value only was only inclined towards a reduction (P = 0.097, Fig. 4c). Psyllium husk appeared to alleviate hepatocyte steatosis better than orlistat. The reason for this was that HFD + orlistat produced an effect similar to that of a high-sucrose diet. Although a high-sucrose diet does not induce obesity,31 it promotes fatty liver disease.34 ALT is an indicator of hepatocyte injury.35 Compared to the NC group, serum ALT levels increased significantly in the MC group (P < 0.001, Fig. 5a), suggesting hepatocyte damage in the MC group due to lipid toxicity.36 Psyllium husk and orlistat decreased serum ALT levels (P < 0.001, Fig. 5a), indicating that psyllium husk and orlistat alleviated hepatic steatosis. Cholesterol is a major lipotoxic molecule among hepatic lipid species in the progression of NAFLD.37 Lowering cholesterol levels in the liver improves NAFLD. The liver is the main organ for cholesterol metabolism. CYP7A1 and CYP27A1 are the rate-limiting enzymes in BA synthesis.38 CYP7A1 is an enzyme required for the transformation of cholesterol into primary BAs such as CA and CDCA through the classical pathway.39 FXR plays an important role in BA metabolism and it mainly inhibits CYP8B1,40 which is required for the production of CA through the classical pathway in the liver. CYP27A1 and CYP7B1 are required for cholesterol conversion to CDCA through alternative pathways.41 CYP7A1 and CYP27A1 can regulate the rate of BA production and play a key role in maintaining the balance of cholesterol and BA.42 The CYP7A1 and CYP27A1 expression levels are correlated with the body's ability to convert cholesterol into BA. In this study, the expression levels of FXR, CYP7A1, CYP8B1, CYP27A1, and CYP7B1 in the liver were detected. Compared to the MC group, the expression levels of CYP27A1 and CYP7B1 in the HFD + PH and HFD + orlistat groups were significantly upregulated (P < 0.05, Fig. 6c and d), suggesting that psyllium husk and orlistat reduced the cholesterol content in the liver tissue and serum by affecting the classical pathway and the alternative pathway of cholesterol conversion to CDCA. The expression level of CYP7A1 in the HFD + PH group was significantly higher than in the HFD + orlistat group, suggesting that psyllium husk had a better effect at enhancing the ability to convert cholesterol into BA than orlistat did, which should be one reason why psyllium husk had a better effect at reducing the cholesterol level than orlistat. The type and concentration of BA can affect the expression of FXR. CA has a better effect at upregulating the expression of FXR than CDCA does.43 In our research, psyllium husk (P = 0.014) and orlistat (P = 0.069) downregulated the expression of FXR (Fig. 6e). This was mainly because both drugs upregulated the expression of CYP27A1 and CYP7B1, which increased the production of CDCA and decreased the production of CA. A decrease in the number of total receptors after long-term stimulation by homologous or heterologous chemical messengers at high extracellular concentrations is called receptor downregulation.44 For FXR, CDCA is the most potent endogenous agonist, whereas CA is weak comparatively.45 This explains why both drugs downregulated FXR in terms of receptor theory. FXR mainly inhibits CYP8B1.40 Theoretically speaking, a decrease in the FXR level leads to an increase in the CYP8B1 level. However, both drugs did not significantly affect the CYP8B1 level. This was because they increased the production of CDCA, which efficiently stimulated FXR, counteracting the effect of FXR down-regulation.
The Shannon index was used to assess α diversity in mouse faecal samples. The Shannon index is based on information entropy, which reflects unknown factors of the species in the samples. The larger the index, the greater the uncertainty, which indicates a higher diversity. There is a strong correlation between faecal concentration and α diversity in the faecal flora. The richness decreases with decreasing faecal concentration, and the Shannon index reaches its lowest value in individuals with diarrhoea.46 There were no significant differences in the Shannon index among the NC, MC, and HFD + PH groups; however, the Shannon index of the HFD + orlistat group decreased (P < 0.1, Fig. 7a). The main reason that the Shannon index of the HFD + orlistat group was lower than the other three groups was that orlistat caused a fat absorption disorder in mice, resulting in fat diarrhoea.47 Consequently, faecal concentrations were lower than those in the other three groups. The four dominant phyla of intestinal bacteria in mice are Bacteroidetes, Firmicutes, Proteobacteria, and Actinobacteria.48 Among them, Bacteroidetes and Firmicutes are also the two main phyla in the intestines of mammals and play important roles in regulating the metabolism of carbohydrates, lipids, and BA in the host.49 It was reported that the characteristics of the faecal flora of HFD-induced obese mice were mainly shown as the relative abundance of Firmicutes increased, the relative abundance of Bacteroidetes decreased, and the relative abundance ratio of Firmicutes/Bacteroidetes (F/B value) increased; this is a sign of intestinal dysbiosis.50 Similar results were also obtained at the phylum level in this study (Fig. 7). Compared to the NC group, the relative abundance of Firmicutes increased, whereas the relative abundance of Bacteroidetes decreased, and the F/B value increased significantly in the MC group (P < 0.001, Fig. 7b–d). Both psyllium husk and orlistat could downregulate the relative abundance of Firmicutes and the F/B value and upregulate the relative abundance of Bacteroidetes in faeces (P < 0.05, Fig. 7b–d), which was a sign that intestinal dysbiosis had been ameliorated. At the genus level, we mainly illustrated those with a close relationship with obesity. As Bilophila was positively correlated with several obesity-related indexes (Fig. 10a), we start this section with Bilophila. Meat diets disturb the balance of the gut microbiota by increasing the relative abundance of Bilophila, a bile acid-tolerant bacteria, and decreasing the relative abundance of fibre-dependent bacteria, such as Roseburia.51 Similar results were observed in this study. Compared to the NC group, the relative abundance of Bilophila in the MC group was significantly elevated (P = 0.005, Fig. 8b). Interestingly, that of the HFD + orlistat group also increased significantly (P = 0.005, Fig. 8b). The reason for this phenomenon was that orlistat interfered with HFD fat absorption in the intestine, which created an oily and high BA level environment in the gut cavity suitable for the proliferation of Bilophila. Compared to the MC and orlistat groups, psyllium husk downregulated the relative abundance of Bilophila (P = 0.066, Fig. 8b). We theorised that this was because psyllium husk was rich in dietary fibre, which elevated the relative abundance of fibre-dependent bacteria such as Roseburia (P = 0.096, Fig. 8c), which compete with Bilophila for colonisation space in the gut. Furthermore, an increase in dietary fibre content in the intestinal tract was not conducive to the growth of bile acid-resistant bacteria. Furthermore, the relative abundance of faecal Bilophila was significantly positively correlated with the faecal total BA content (R = 0.425, P = 0.038, Fig. 10a). When psyllium husk decreased cholesterol levels, faecal BA levels also decreased, which created an environment that was not conducive to Bilophila. Studies found that Bilophila, combined with a HFD, affected liver function.52,53 Because Bilophila is a Gram-negative genus,54 it produces LPSs that impair the intestinal barrier, and aggravate NAFLD.55,56 We further measured the LPS level in the serum and found that obese mice had a higher LPS level (Fig. 5b). Interestingly, although orlistat could not significantly affect the relative abundance of Bilophila, it reduced the level of LPS in the serum (P < 0.001, Fig. 5b). We considered that orlistat increased the relative abundance of Akkermansia (Fig. 8g), which improved the damaged intestinal barrier;57 therefore, it prevented LPSs from entering the circulatory system. Psyllium husk had a better effect at reducing the serum LPS level than orlistat (HFD + PH and HFD + orlistat, 0.74-fold, P = 0.015, Fig. 5b). There are two reasons for this phenomenon. First, psyllium husk reduced the relative abundance of Bilophila that produced LPSs, whereas orlistat could not. Second, psyllium husk increased the relative abundance of Faecalibacterium, Sutterella, and Coprobacillus (P < 0.1, Fig. 8), the short-chain fatty acid (SCFA) producers,58–60 and SCFA promoted protection and repair of intestinal epithelial barrier function, preventing LPSs from entering the circulatory system. Psyllium husk and orlistat helped to ameliorate liver impairment by reducing the LPS level, which should be one reason for the reduction of ALT in the serum. Furthermore, the SCFA producers Faecalibacterium, Sutterella, and Coprobacillus also have other benefits for treating obesity such as stimulating energy consumption,13 accelerating fat oxidation, and activating the thermogenesis of brown adipose tissue by producing butyric acid.59–61 Finally, psyllium husk increased the relative abundance of Bacteroides and Parabacteroides distasonis, which improved energy consumption and played a beneficial regulatory role in lipid metabolism, which should be helpful for weight control.62,63 BA metabolism is closely associated with the occurrence of a fatty liver and obesity. BAs are markers of sterol content and energy storage in the body.64 BA synthesis is the main pathway for a daily output of approximately 90% of the cholesterol in the body.65 Under physiological conditions, 95% of BAs entering the intestine of the duodenal papilla are reabsorbed in the intestine, whereas the remaining 5% are excreted in faeces.65 To some extent, the level of BAs in faeces reflects the metabolism of BAs in the body. The BA content in the faeces is affected by the following factors: (1) source: BAs are mainly produced by liver cells. After entering the gallbladder, BAs enter the duodenum of the large papilla with bile excretion. (2) Intestinal flora: the intestinal flora react with BAs, for example, by converting primary BAs into secondary BAs.66 (3) The amount of BAs that is reabsorbed by the intestine.67 CA and CDCA are two major BAs produced by cholesterol metabolism in the liver. These two bile acids are converted into DCA, LCA, MCA, UDCA, and HDCA as previously summarised.68,69 In this study, a HFD significantly increased faecal total BA levels, whereas psyllium husk and orlistat reduced total faecal BA levels (P < 0.05, Fig. 9f), which showed a consistent trend of liver and serum cholesterol (Fig. 4a and 5d). Related research mentioned that the level of total BA in the faeces of HFD-induced obese mice increased significantly,70 and was consistent with the results obtained in this study. The reason that the faecal BA concentration of obese mice was significantly higher than in the NC group was that cholesterol increased significantly in the liver and serum (P < 0.05, Fig. 4a and 5d). Possibly due to the increased BA synthesis in obese mice, most types of BA concentrations in faeces were significantly higher in obese mice than in the NC group (P < 0.05, Fig. 9). After weight loss, BA levels in the faeces decrease accordingly.71 Interestingly, although orlistat had a better effect on weight control than psyllium husk did, orlistat
had a significantly higher level of faecal BA (P = 0.022, Fig. 9f), probably because orlistat had a higher level of serum and liver cholesterol (P = 0.006, Fig. 4a; P = 0.070, Fig. 5d). Furthermore, decreased BA levels mean less intestinal lipid absorption,72 which indicates that psyllium husk reduced lipid absorption by decreasing BA levels. Psyllium husk had a better effect at reducing the level of faecal BAs than orlistat did (Fig. 9f), which should be a reason why psyllium husk had a better effect at ameliorating serum and liver triglyceride than orlistat (P < 0.05, Fig. 5e and 4b). With specific BAs, we analysed CA, a 12α-hydroxylated BA. CA is synthesised by CYP7A1, CYP8B1, and CYP27A1 through the classical pathway of cholesterol metabolism. CA was reported to play an important role in promoting intestinal cholesterol absorption,73 and 12α-hydroxylated BA is associated with insulin resistance and high triglyceride levels.74 It was also found that 13 weeks of a diet supplemented with CA could increase serum triglyceride and cholesterol levels and induce a fatty liver in rats.75 Therefore, the level of CA in food is closely related to fatty liver disease. After CA enters the digestive tract, a portion is reabsorbed by the intestine and enters the liver via the portal vein, whereas the remainder reacts with the gut flora or is excreted. We found that the level of CA in faeces was significantly positively correlated with the level of hepatic TG (R = 0.577, P = 0.003, Fig. 10b). A HFD significantly increased the faecal CA content (P < 0.001, Fig. 9a), since obese mice had a higher cholesterol level. Mice treated with psyllium husk and orlistat had lower levels of faecal CA (P < 0.05, Fig. 9a), because of the reduction in the cholesterol level. Meanwhile, mice in the HFD + PH and HFD + orlistat groups had higher expressions of CYP27A1 and CYP7B1, which metabolised more cholesterol into CDCA rather than CA. Therefore, mice in the MC group had the highest faecal CA among all groups. DCA exhibited strong hepatotoxicity.76 We found a significant positive correlation between faecal DCA concentration and ALT concentration in serum (R = 0.688, P = 0.003, Fig. 10b), suggesting that the faecal DCA concentration could reflect liver injury in bodies with a fatty liver. Furthermore, Lee et al. found that the faecal DCA was more likely to induce a fatty liver than CA in the diet.75 In this study, the faecal DCA content was significantly positively correlated with the level of liver TG (r = 0.564, P = 0.004, Fig. 10b). Psyllium husk and orlistat significantly decreased the faecal DCA content, whereas both drugs reduced the serum ALT concentration (P < 0.001, Fig. 5a) and improved the severity of the fatty liver (Fig. 4). As mentioned previously, a HFD significantly elevated faecal CA, whereas psyllium husk and orlistat reduced faecal CA. Moreover, DCA is a free secondary bile metabolised by bacteria. Therefore, we speculated that psyllium husk and orlistat decreased the level of faecal DCA by downregulating CA production. CDCA is a free primary BA metabolised from cholesterol through the classical or alternative pathway. CDCA can be synthesised through the classical pathway by CYP7A1 and CYP27A1, but also through the alternative pathway by CYP27A1 and CYP7B1. Psyllium husk upregulated the expression of CYP27A1 and CYP7B1 in the liver and increased cholesterol metabolism to produce CDCA through alternative pathways. Meanwhile, in the classical pathway, increasing CYP27A1 expression made more 7α-hydroxy-4-cholesten-3-one (C4) react with CYP27A1 first rather than CYP8B1 and promoted the synthesis of CDCA. By inference, the CDCA level in the faeces of the PH + HFD group should be higher than the MC group. However, in this study, the concentration of faecal CDCA of the MC group was significantly higher than other groups (P < 0.05, Fig. 9c), because the levels of total BA and major BAs in faeces of obese mice induced by a HFD were significantly increased (P < 0.05, Fig. 9f). Specifically, in obese mice, the absolute concentration of CDCA, a major component of total BA, in faeces also increased correspondingly. The levels of faecal BA decreased after weight loss due to decreased serum and liver cholesterol levels,71 and the levels of major BAs were also downregulated. After treatment with psyllium husk, the body weight of mice in the HFD + PH group was significantly lower than obese mice (P < 0.05, Fig. 1a. Therefore, the concentration of total BA in the faeces of mice in the HFD + PH group was lower than that in the MC group and CDCA, one of the main components of total BA, decreased correspondingly. Therefore, the faecal CDCA concentration in the HFD + PH group was significantly lower than in the NC group. After entering the intestinal tract, CDCA can be converted into LCA, a type of free secondary BA, through bacterial metabolism; LCA is hepatotoxic.77 In our study, there was a significant positive correlation between the faecal LCA content and the concentration of serum ALT, a marker of liver injury (r = 0.601, P = 0.002, Fig. 10b). The faecal LCA concentration was increased by a HFD and downregulated by psyllium husk and orlistat (P < 0.05, Fig. 9d). The faecal LCA content may reflect liver injury induced by the fatty liver. Furthermore, LCA can be hydroxylated to hyodeoxycholic acid (HDCA) by CYP3A4.69 In the mice's livers, CDCA can be converted into MCA, the main BA in mice, by CYP2C70 or CYP3A4.69 CDCA can also be converted into ursodeoxycholic acid (UDCA) by 7α/β-HSDH.69 We further analysed the relationship between faecal BA composition, the intervention of psyllium husk or orlistat, and the expression levels of enzymes related to BA synthesis in the liver. The cholesterol level in the MC group was significantly higher than in the other three groups (P < 0.05, Fig. 4a and 5d), and the absolute value of the contents of various major BAs in faeces was significantly higher than those of the other three groups (P < 0.05, Fig. 9a–e), so the differences in the expressions of CYP27A1 and CYP7B1 in liver among all groups cannot be reflected merely by the absolute concentration of certain BAs in faeces. Therefore, we designed a relatively reasonable intergroup comparison method, which was based on the differences in enzymes required by cholesterol metabolism to generate CA, as well as its downstream products DCA and CDCA, as well as its downstream products LCA, MCA, HDCA, and UDCA. CDCA, LCA, MCA, HDCA, and UDCA can be produced by classical or alternative pathways, but are independent of CYP8B1 synthesis. Therefore, the expression of CYP27A1 and CYP7B1 in the liver can be reflected in the ratio of the concentration of CYP8B1-dependent BA and that of non-CYP8B1-dependent BA in faeces, namely, (CA + DCA)/(CDCA + LCA + MCA + HDCA + UDCA) (abbreviated to CA/CDCA) (Fig. 9h). There were no significant differences in the expression levels of CYP8B1 among the MC, HFD + PH and HFD + orlistat groups (P > 0.05, Fig. 6b), suggesting there were no significant differences in the ability of the three groups to metabolise C4 into CA. The CA/CDCA value in the HFD + PH group was significantly lower than in the MC group (P = 0.018, Fig. 9g). From the perspective of the composition of faecal BA, it was supposed that psyllium husk could make more cholesterol metabolise into CDCA by upregulating liver CYP27A1 through classical or alternative pathways. Psyllium husk could facilitate the excretion of CDCA and its downstream products from the body in faeces. CA and CDCA are converted into T/GCA and T/GCDCA, the conjugated BAs of CA and CDCA, in the liver before being excreted into the intestine.69 Dietary fibre binds to BAs, reducing BA reabsorption and increasing the concentration of BAs excreted from faeces, thus reducing the cholesterol level. Compared to T/GCA, T/GCDCA is more likely to be bound to dietary fibre.78 Psyllium husk is rich in dietary fibre, and it is more likely to bind to T/GCDCA rather than T/GCA after BAs enter the intestine from the major duodenal papilla. Therefore, during intestinal BA reabsorption, compared with T/GCDCA, more T/GCA is reabsorbed by the intestine. Correspondingly, more T/GCDCA is retained and further metabolised to produce products such as LCA. This is another possible reason why psyllium husk significantly decreased the faecal CA/CDCA value. Interestingly, although orlistat could also upregulate the expression of CYP27A1 and CYP7B1 in the liver, unlike
psyllium husk, orlistat did not significantly change the CA/CDCA value (Fig. 9g). Unlike psyllium husk, orlistat achieves its cholesterol-lowering effect through another mechanism that affects BA reabsorption. Orlistat inhibits lipase activity and may reduce the solubility of cholesterol by reducing the amounts of monoglycerides and fatty acids present in the intestine, leading to cholesterol sequestration within a more persistent oil phase in the gut.79 It is likely that BAs as cholesterol metabolites can also be sequestered by the persistent oil phase in the gut. CA is more hydrophilic than CDCA,69 less soluble in oils, and more likely to be sequestered by the oil phase. Therefore, for the HFD + orlistat group, compared to CA, more CDCA was reabsorbed in the intestine. Consequently, more CA is retained and further metabolised to produce products such as DCA. This may explain why orlistat cannot significantly decrease the faecal CA/CDCA value. Although psyllium husk and orlistat reduced the reabsorption of bile acids in different ways, the reduction of the reabsorption of bile acid will decrease the liver BA level, and increase the expression of liver CYP27A1 and CYP7B1 through a feedback mechanism, which promotes the transformation of cholesterol into bile acid in the liver and discharge from the body, forming a virtuous cycle, and ameliorating NAFLD and hypercholesterolemia.
Our study has limitations. As psyllium husk is a type of food with a high safety level, most previous studies utilising it were conducted through clinical studies, and animal studies mainly focused on its specific extracts. Therefore, we could not obtain an appropriate concentration of psyllium husk for gastric gavage from literature studies. Psyllium husk expands as it absorbs water and clogs the gavage needle if the concentration is too high; therefore, in this experiment, we used a concentration that passed more easily through the gavage needle, and the amount of daily gavage used was approximately equivalent to the lowest recommended daily fibre supplement for an adult. Due to the high cost of a HFD, the long experimental period, and the uncertain weight control effect of psyllium husk, only one concentration was used in this study, and the dose–effect relationship of the weight loss effect of psyllium husk was not studied. In future studies, we intend to examine several more concentration gradients in the next stage of the experiment to study the dose–effect relationship of psyllium husk in the treatment of HFD-induced obese mice. As it is costly to raise each mouse in an individual cage during the experimental phase, we only kept one mouse per cage for the final week of each phase to observe the food and water intake of each mouse. Therefore, we provided data on the total amount of food and water intake for the whole group in one cage during the other weeks (Fig. 2a and 3a). During the sampling process, only fresh faeces from the anus of mice were collected, whereas faeces from the small intestine and cecum were not collected. Therefore, we did not observe how faecal flora and BA interacted with the intestinal mucosa of the small intestine, cecum, and anus. We considered using rats as experimental animals, but rats consume large amounts of a HFD, which is costly. Consequently, for this investigation, mice were used as experimental animals after thorough consideration of various factors. However, the amount of liver tissue and serum that could be extracted from the mice did not allow us to perform targeted detection of BA metabolomics in liver tissues and serum; therefore, the hepatoenteric circulation state of the BAs was not completely determined. Takeda G-protein-coupled receptor 5 (TGR5) is also an imperative receptor for BA metabolism; however, the relative content of TGR5 in the liver could not be detected by the TMT technique due to technical limitations and there was no sample left for us to test through other methods such as western blotting and parallel reaction monitoring. In future experiments, obese rats will be used as experimental animals to observe the effects of psyllium husk on BA hepatoenteric circulation and receptors related to bile acid metabolism. Because orlistat and psyllium husk control body weight, ameliorate NAFLD and affect BA metabolism through different mechanisms, we will observe whether the combination of both drugs can produce better results in the subsequent experiment.
Conclusions
This study compared the effects of psyllium husk and orlistat on HFD-induced obese mice using metabolic indices in the serum, liver pathology, gut microbiota, and BA metabolism. Orlistat had a greater effect on weight loss, while psyllium husk treatment resulted in a larger reduction in cholesterol and triglyceride levels. The two therapies similarly controlled the body fat rate, elevated the expression of CYP27A1 and CYP7B1 in the liver, and regulated BAs, such as CA, CDCA, DCA, and LCA, in the faecal content. The structures of faecal BAs differed as the two drugs reduced the reabsorption of different bile acids through different mechanisms, which helped cholesterol be metabolised into BAs that were excreted. Both treatments had similar effects on the gut microbiota at the phylum level; however, there were differences at the genus level for Bilophila, Roseburia, Bacteroides, Faecalibacterium, Coprobacillus, and Akkermansia, which probably meant that the two drugs had different effects on the LPS level in serum that affected NAFLD. Furthermore, orlistat increased the food intake of obese mice fed a HFD, which might increase water intake, serum triglyceride levels, and lower glucose tolerance. Although orlistat is a useful drug for weight loss, psyllium husk offers a safer choice for weight control as it is classified as a food and is better than orlistat at ameliorating hypercholesterolemia and HFD-induced NAFLD.
Author contributions
Zhitong Deng: conceptualisation, methodology, animal experiments; Cong Meng: writing – original draft; Haodong Huang: writing – review & editing; Siyu Song: drawing figures; Linchun Fu: data curation; Zhuotao Fu: supervision. All authors have read and agreed to the published version of the manuscript.
Conflicts of interest
There are no conflicts to declare.
Acknowledgements
This study was funded by the Traditional Chinese Medicine Bureau of Guangdong Province (Grant No. 20212055).
This study was funded by a project of the Guangzhou University of Chinese Medicine (No. 2021-XK-75).
We also thank the Lingnan Medical Research Center for providing us with experimental facilities, and would like to thank Dr Siobhan Roisin from Editage for English language editing.
References
- A. Afshin, M. H. Forouzanfar, M. B. Reitsma, P. Sur, K. Estep, A. Lee, L. Marczak, A. H. Mokdad, M. Moradi-Lakeh, M. Naghavi, J. S. Salama, T. Vos, K. H. Abate, C. Abbafati, M. B. Ahmed, Z. Al-Aly, A. Alkerwi, R. Al-Raddadi, A. T. Amare, A. Amberbir, A. K. Amegah, E. Amini, S. M. Amrock, R. M. Anjana, J. Arnlov, H. Asayesh, A. Banerjee, A. Barac, E. Baye, D. A. Bennett, A. S. Beyene, S. Biadgilign, S. Biryukov, E. Bjertness, D. J. Boneya, I. Campos-Nonato, J. J. Carrero, P. Cecilio, K. Cercy, L. G. Ciobanu, L. Cornaby, S. A. Damtew, L. Dandona, R. Dandona, S. D. Dharmaratne, B. B. Duncan, B. Eshrati, A. Esteghamati, V. L. Feigin, J. C. Fernandes, T. Furst, T. T. Gebrehiwot, A. Gold, P. N. Gona, A. Goto, T. D. Habtewold, K. T. Hadush, N. Hafezi-Nejad, S. I. Hay, M. Horino, F. Islami, R. Kamal, A. Kasaeian, S. V. Katikireddi, A. P. Kengne, C. N. Kesavachandran, Y. S. Khader, Y. H. Khang, J. Khubchandani, D. Kim, Y. J. Kim, Y. Kinfu, S. Kosen, T. Ku, B. K. Defo, G. A. Kumar, H. J. Larson, M. Leinsalu, X. Liang, S. S. Lim, P. Liu, A. D. Lopez, R. Lozano, A. Majeed, R. Malekzadeh, D. C. Malta, M. Mazidi, C. McAlinden, S. T. McGarvey, D. T. Mengistu, G. A. Mensah, G. B. M. Mensink, H. B. Mezgebe, E. M. Mirrakhimov, U. O. Mueller, J. J. Noubiap, C. M. Obermeyer, F. A. Ogbo, M. O. Owolabi, G. C. Patton, F. Pourmalek, M. Qorbani, A. Rafay, R. K. Rai, C. L. Ranabhat, N. Reinig, S. Safiri, J. A. Salomon, J. R. Sanabria, I. S. Santos, B. Sartorius, M. Sawhney, J. Schmidhuber, A. E. Schutte, M. I. Schmidt, S. G. Sepanlou, M. Shamsizadeh, S. Sheikhbahaei, M. J. Shin, R. Shiri, I. Shiue, H. S. Roba, D. A. S. Silva, J. I. Silverberg, J. A. Singh, S. Stranges, S. Swaminathan, R. Tabares-Seisdedos, F. Tadese, B. A. Tedla, B. S. Tegegne, A. S. Terkawi, J. S. Thakur, M. Tonelli, R. Topor-Madry, S. Tyrovolas, K. N. Ukwaja, O. A. Uthman, M. Vaezghasemi, T. Vasankari, V. V. Vlassov, S. E. Vollset, E. Weiderpass, A. Werdecker, J. Wesana, R. Westerman, Y. Yano, N. Yonemoto, G. Yonga, Z. Zaidi, Z. M. Zenebe, B. Zipkin and C. J. L. Murray, Health Effects of Overweight and Obesity in 195 Countries over 25 Years, N. Engl. J. Med., 2017, 377, 13–27 CrossRef PubMed.
- F. Petermann-Rocha, S. Yang, S. R. Gray, J. P. Pell, C. Celis-Morales and F. K. Ho, Sarcopenic obesity and its association with respiratory disease incidence and mortality, Clin. Nutr., 2020, 39, 3461–3466 CrossRef PubMed.
- T. Kawada, Metabolically healthy obesity and cardiovascular events: A risk of obesity, Diabetes, Obes. Metab., 2022, 24, 763, DOI:10.1111/dom.14628.
- F. P. de Heredia, S. Gómez-Martínez and A. Marcos, Obesity, inflammation and the immune system, Proc. Nutr. Soc., 2012, 71, 332–338 CrossRef CAS PubMed.
- E. Colman, Anorectics on trial: a half century of federal regulation of prescription appetite suppressants, Ann. Intern. Med., 2005, 143, 380–385 CrossRef PubMed.
- A. Beydoun, Monotherapy trials of new antiepileptic drugs, Epilepsia, 1997, 38(Suppl 9), S21–S31 CrossRef CAS PubMed.
- X. G. Lei, J. Q. Ruan, C. Lai, Z. Sun and X. Yang, Efficacy and Safety of Phentermine/Topiramate in Adults with Overweight or Obesity: A Systematic Review and Meta-Analysis, Obesity, 2021, 29, 985–994 CrossRef CAS PubMed.
- W. N. Kernan, C. M. Viscoli, L. M. Brass, J. P. Broderick, T. Brott, E. Feldmann, L. B. Morgenstern, J. L. Wilterdink and R. I. Horwitz, Phenylpropanolamine and the risk of hemorrhagic stroke, N. Engl. J. Med., 2000, 343, 1826–1832 CrossRef CAS PubMed.
- T. D. Filippatos, C. S. Derdemezis, I. F. Gazi, E. S. Nakou, D. P. Mikhailidis and M. S. Elisaf, Orlistat-associated adverse effects and drug interactions: a critical review, Drug Saf., 2008, 31, 53–65 CrossRef CAS PubMed.
- A. C. Frati-Munari, J. A. Fernández-Harp, M. Becerril, A. Chávez-Negrete and M. Bañales-Ham, Decrease in serum lipids, glycemia and body weight by Plantago psyllium in obese and diabetic patients, Arch. Invest. Med., 1983, 14, 259–268 CAS.
- R. F. Irvine, S. M. Lloyd-Burton, J. C. Yu, A. J. Letcher and M. J. Schell, The regulation and function of inositol 1,4,5-trisphosphate 3-kinases, Adv. Enzyme Regul., 2006, 46, 314–323 CrossRef CAS PubMed.
- D. L. Sprecher, B. V. Harris, A. C. Goldberg, E. C. Anderson, L. M. Bayuk, B. S. Russell, D. S. Crone, C. Quinn, J. Bateman, B. R. Kuzmak and L. D. Allgood, Efficacy of psyllium in reducing serum cholesterol levels in hypercholesterolemic patients on high- or low-fat diets, Ann. Intern. Med., 1993, 119, 545–554 CrossRef CAS PubMed.
- B. Shirouchi, K. Nagao, M. Umegatani, A. Shiraishi, Y. Morita, S. Kai, T. Yanagita, A. Ogawa, Y. Kadooka and M. Sato, Probiotic Lactobacillus gasseri SBT2055 improves glucose tolerance and reduces body weight gain in rats by stimulating energy expenditure, Br. J. Nutr., 2016, 116, 451–458 CrossRef CAS PubMed.
- S. Z. Li, S. L. Zeng and E. H. Liu, Anti-obesity natural products and gut microbiota, Food Res. Int., 2022, 151, 110819 CrossRef CAS PubMed.
- J. L. Sonnenburg and F. Bäckhed, Diet-microbiota interactions as moderators of human metabolism, Nature, 2016, 535, 56–64 CrossRef CAS PubMed.
- J. C. McGrath and E. Lilley, Implementing guidelines on reporting research using animals (ARRIVE etc.): new requirements for publication in BJP, Br. J. Pharmacol., 2015, 172, 3189–3193 CrossRef CAS PubMed.
- P. Garg, Psyllium Husk Should Be Taken at Higher Dose with Sufficient Water to Maximize Its Efficacy, J. Acad. Nutr. Diet., 2017, 117, 681 CrossRef PubMed.
- J. Choi, K. J. Kim, E. J. Koh and B. Y. Lee, Gelidium elegans Regulates the AMPK-PRDM16-UCP-1 Pathway and Has a Synergistic Effect with Orlistat on Obesity-Associated Features in Mice Fed a High-Fat Diet, Nutrients, 2017, 9, 342 CrossRef PubMed.
- N. Hariri and L. Thibault, High-fat diet-induced obesity in animal models, High-fat diet-induced obesity in animal models, Nutr. Res. Rev., 2010, 23, 270–299 CrossRef CAS PubMed.
- G. Chen, H. Li, Y. Zhao, H. Zhu, E. Cai, Y. Gao, S. Liu, H. Yang and L. Zhang, Saponins from stems and leaves of Panax ginseng prevent obesity via regulating thermogenesis, lipogenesis and lipolysis in high-fat diet-induced obese C57BL/6 mice, Food Chem. Toxicol., 2017, 106, 393–403 CrossRef CAS PubMed.
- S. Andrikopoulos, A. R. Blair, N. Deluca, B. C. Fam and J. Proietto, Evaluating the glucose tolerance test in mice, Am. J. Physiol. Endocrinol. Metab., 2008, 295, E1323–E1332 CrossRef CAS PubMed.
- F. Suriano, S. Vieira-Silva, G. Falony, M. Roumain, A. Paquot, R. Pelicaen, M. Regnier, N.M. Delzenne, J. Raes, G. G. Muccioli, M. Van Hul and P. D. Cani, Novel insights into the genetically obese (ob/ob) and diabetic (db/db) mice: two sides of the same coin, Microbiome, 2021, 9, 147 CrossRef CAS PubMed.
- M. C. Hamilton, M. M. Heintz, M. Pfohl, E. Marques, L. Ford, A. L. Slitt and W. S. Baldwin, Increased toxicity and retention of perflourooctane sulfonate (PFOS) in humanized CYP2B6-Transgenic mice compared to Cyp2b-null mice is relieved by a high-fat diet (HFD), Food Chem. Toxicol., 2021, 152, 112175 CrossRef CAS PubMed.
-
G. Zhang, The Effects of Feeding High Caloric Diet to Mice Different Developmental Stage on Several Physiological Indexes, Shandong Agricultural University, 2008 Search PubMed.
- F. Capel, M. H. Delmotte, M. Brun, M. Lonchampt, B. De Fanti, L. Xuereb, L. Baschet, G. Rolland, J. P. Galizzi, B. Lockhart, A. Ktorza and C. Dacquet, Aging and obesity induce distinct gene expression adaptation in the liver of C57BL/6J mice, J. Nutrigenet. Nutrigenomics, 2011, 4, 154–164 CAS.
- B. Fromenty, N. Vadrot, J. Massart, B. Turlin, N. Barri-Ova, P. Lettéron, A. Fautrel and M. A. Robin, Chronic ethanol consumption lessens the gain of body weight, liver triglycerides, and diabetes in obese ob/ob mice, J. Pharmacol. Exp. Ther., 2009, 331, 23–34 CrossRef CAS PubMed.
- R. Sharif-Naeini, S. Ciura, Z. Zhang and C. W. Bourque, Contribution of TRPV channels to osmosensory transduction, thirst, and vasopressin release, Kidney Int., 2008, 73, 811–815 CrossRef CAS PubMed.
- J. K. Walters and G. I. Hatton, Supraoptic neuronal activity in rats during five days of water deprivation, Physiol. Behav., 1974, 13, 661–667 CrossRef CAS PubMed.
- C. R. Rivers and M. A. Kantor, Psyllium husk intake and risk of type 2 diabetes: an evidence-based scientific and regulatory review of a qualified health claim conducted by the US Food and Drug Administration, Nutr. Rev., 2020, 78, 787–797 CrossRef PubMed.
- V. Olvera-Hernández, J. L. Ble-Castillo, D. Betancur-Ancona, J. J. Acevedo-Fernández, A. Castellanos-Ruelas and L. Chel-Guerrero, Effects of modified banana (Musa cavendish)starch on glycemic control and blood pressure in rats with high sucrose diet, Nutr. Hosp., 2018, 35, 588–595 Search PubMed.
- O. L. Patkar, A. Z. Mohamed, A. Narayanan, K. Mardon, G. Cowin, R. Bhalla, D. H. R. Stimson, M. Kassiou, K. Beecher, A. Belmer, I. Alvarez Cooper, M. Morgan, D. A. Hume, K. M. Irvine, S. E. Bartlett, F. Nasrallah and P. Cumming, A binge high sucrose diet provokes systemic and cerebral inflammation in rats without inducing obesity, Sci Rep., 2021, 11, 11252 CrossRef CAS PubMed.
- S. Liangpunsakul and N. Chalasani, Unexplained elevations in alanine aminotransferase in individuals with the metabolic syndrome: results from the third National Health and Nutrition Survey (NHANES III), Am. J. Med. Sci., 2005, 329, 111–116 CrossRef PubMed.
- Y. Zou, L. Zhong, C. Hu, M. Zhong, N. Peng and G. Sheng, LDL/HDL cholesterol ratio is associated with new-onset NAFLD in Chinese non-obese people with normal lipids: a 5-year longitudinal cohort study, Lipids Health Dis., 2021, 20, 28 CrossRef CAS PubMed.
- S. Sun, Y. Araki, F. Hanzawa, M. Umeki, T. Kojima, N. Nishimura, S. Ikeda, S. Mochizukiand and H. Oda, High sucrose diet-induced dysbiosis of gut microbiota promotes fatty liver and hyperlipidemia in rats, J. Nutr. Biochem., 2021, 93, 108621 CrossRef CAS PubMed.
- Y. Lu, Q. Wang, L. Yu, X. Yin, H. Yang, X. Xu, Y. Xia, Y. Luo, Y. Peng, Q. Yu, Z. Chen, J. Yu, M. Lai, N. Wu, X. B. Pan and X. Zheng, Revision of serum ALT upper limits of normal facilitates assessment of mild liver injury in obese children with non-alcoholic fatty liver disease, J. Clin. Lab. Anal., 2020, 34, e23285 CAS.
- M. J. Armstrong, D. Hull, K. Guo, D. Barton, J. M. Hazlehurst, L. L. Gathercole, M. Nasiri, J. Yu, S. C. Gough, P. N. Newsome and J. W. Tomlinson, Glucagon-like peptide 1 decreases lipotoxicity in non-alcoholic steatohepatitis, J. Hepatol., 2016, 64, 399–408 CrossRef CAS PubMed.
- G. N. Ioannou, The Role of Cholesterol in the Pathogenesis of NASH, Trends Endocrinol. Metab., 2016, 27, 84–95 CrossRef CAS PubMed.
- H. Jones, G. Alpini and H. Francis, Bile acid signaling and biliary functions, Acta Pharm. Sin. B, 2015, 5, 123–128 CrossRef PubMed.
- D. Rizzolo, K. Buckley, B. Kong, L. Zhan, J. Shen, M. Stofan, A. Brinker, M. Goedken, B. Buckley and G. L. Guo, Bile Acid Homeostasis in a Cholesterol 7alpha-Hydroxylase and Sterol 27-Hydroxylase Double Knockout Mouse Model, Hepatology, 2019, 70, 389–402 CAS.
- B. Kong, L. Wang, J. Y. Chiang, Y. Zhang, C. D. Klaassen and G. L. Guo, Mechanism of tissue-specific farnesoid X receptor in suppressing the expression of genes in bile-acid synthesis in mice, Hepatology, 2012, 56, 1034–1043 CrossRef CAS PubMed.
- G. Lorbek, M. Lewinska and D. Rozman, Cytochrome P450s in the synthesis of cholesterol and bile acids–from mouse models to human diseases, FEBS J., 2012, 279, 1516–1533 CrossRef CAS PubMed.
- F. M. Vaz and S. Ferdinandusse, Bile acid analysis in human disorders of bile acid biosynthesis, Mol. Aspects Med., 2017, 56, 10–24 CrossRef CAS PubMed.
- J. Dai, H. Wang, Y. Shi, Y. Dong, Y. Zhang and J. Wang, Impact of bile acids on the growth of human cholangiocarcinoma via FXR, J. Hematol. Oncol., 2011, 4, 41 CrossRef CAS PubMed.
-
E. P. Widmaier and A. J. Vander, Vander's Human Physiology: the Mechanisms of Body Function, 15th edn, McGraw-Hill Education, New York, 2019 Search PubMed.
- H. Wang, J. Chen, K. Hollister, L. C. Sowers and B. M. Forman, Endogenous bile acids are ligands for the nuclear receptor FXR/BAR, Mol. Cell, 1999, 3, 543–553 CrossRef CAS PubMed.
- D. Vandeputte, G. Falony, S. Vieira-Silva, R. Y. Tito, M. Joossens and J. Raes, Stool consistency is strongly associated with gut microbiota richness and composition, enterotypes and bacterial growth rates, Gut, 2016, 65, 57–62 CrossRef CAS PubMed.
- M. J. Perrio, L. V. Wilton and S. A. Shakir, The safety profiles of orlistat and sibutramine: results of prescription-event monitoring studies in England, Obesity, 2007, 15, 2712–2722 CrossRef PubMed.
- R. Nagpal, S. Wang, L. C. Solberg Woods, O. Seshie, S. T. Chung, C. A. Shively, T. C. Register, S. Craft, D. A. McClain and H. Yadav, Comparative
Microbiome Signatures and Short-Chain Fatty Acids in Mouse, Rat, Non-human Primate, and Human Feces, Front. Microbiol., 2018, 9, 2897 CrossRef PubMed.
- V. Tremaroli and F. Bäckhed, Functional interactions between the gut microbiota and host metabolism, Nature, 2012, 489, 242–249 CrossRef CAS PubMed.
- M. C. Dao and K. Clément, Gut microbiota and obesity: Concepts relevant to clinical care, Eur. J. Intern. Med., 2018, 48, 18–24 CrossRef PubMed.
- L. A. David, C. F. Maurice, R. N. Carmody, D. B. Gootenberg, J. E. Button, B. E. Wolfe, A. V. Ling, A. S. Devlin, Y. Varma, M. A. Fischbach, S. B. Biddinger, R. J. Dutton and P. J. Turnbaugh, Diet rapidly and reproducibly alters the human gut microbiome, Nature, 2014, 505, 559–563 CrossRef CAS PubMed.
- J. M. Natividad, B. Lamas, H. P. Pham, M. L. Michel, D. Rainteau, C. Bridonneau, G. da Costa, J. van Hylckama Vlieg, B. Sovran, C. Chamignon, J. Planchais, M. L. Richard, P. Langella, P. Veiga and H. Sokol, Bilophila wadsworthia aggravates high fat diet induced metabolic dysfunctions in mice, Nat. Commun., 2018, 9, 2802 CrossRef PubMed.
- J. M. Natividad, A. Agus, J. Planchais, B. Lamas, A. C. Jarry, R. Martin, M. L. Michel, C. Chong-Nguyen, R. Roussel, M. Straube, S. Jegou, C. McQuitty, M. Le Gall, G. da Costa, E. Lecornet, C. Michaudel, M. Modoux, J. Glodt, C. Bridonneau, B. Sovran, L. Dupraz, A. Bado, M. L. Richard, P. Langella, B. Hansel, J. M. Launay, R. J. Xavier, H. Duboc and H. Sokol, Impaired Aryl Hydrocarbon Receptor Ligand Production by the Gut Microbiota Is a Key Factor in Metabolic Syndrome, Cell Metab., 2018, 28(5), 737–749.e4 CrossRef CAS PubMed.
- M. J. Kasten, J. E. Rosenblatt and D. R. Gustafson, Bilophila wadsworthia bacteremia in two patients with hepatic abscesses, J. Clin. Microbiol., 1992, 30, 2502–2503 CrossRef CAS PubMed.
- H. Cetuk, A. Anishkin, A. J. Scott, S. B. Rempe, R. K. Ernst and S. Sukharev, Partitioning of Seven Different Classes of Antibiotics into LPS Monolayers Supports Three Different Permeation Mechanisms through the Outer Bacterial Membrane, Langmuir, 2021, 37, 1372–1385 CrossRef CAS PubMed.
- G. Carpino, M. Del Ben, D. Pastori, R. Carnevale, F. Baratta, D. Overi, H. Francis, V. Cardinale, P. Onori, S. Safarikia, V. Cammisotto, D. Alvaro, G. Svegliati-Baroni, F. Angelico, E. Gaudio and F. Violi, Increased Liver Localization of Lipopolysaccharides in Human and Experimental NAFLD, Hepatology, 2020, 72, 470–485 CrossRef CAS PubMed.
- M. Shi, Y. Yue, C. Ma, L. Dong and F. Chen, Pasteurized Akkermansia muciniphila Ameliorate the LPS-Induced Intestinal Barrier Dysfunction via Modulating AMPK and NF-kappaB through TLR2 in Caco-2 Cells, Nutrients, 2022, 14, 764 CrossRef CAS PubMed.
- J. Lee, J. d'Aigle, L. Atadja, V. Quaicoe, P. Honarpisheh, B. P. Ganesh, A. Hassan, J. Graf, J. Petrosino, N. Putluri, L. Zhu, D. J. Durgan, R. M. Bryan Jr., L. D. McCullough and V. R. Venna, Gut Microbiota-Derived Short-Chain Fatty Acids Promote Poststroke Recovery in Aged Mice, Circ. Res., 2020, 127, 453–465 CrossRef CAS PubMed.
- A. Detman, D. Laubitz, A. Chojnacka, P. R. Kiela, A. Salamon, A. Barberán, Y. Chen, F. Yang, M. K. Błaszczyk and A. Sikora, Dynamics of dark fermentation microbial communities in the light of lactate and butyrate production, Microbiome, 2021, 9, 158 CrossRef CAS PubMed.
- K. Muthuramalingam, V. Singh, C. Choi, S. I. Choi, Y. M. Kim, T. Unno and M. Cho, Dietary intervention using (1,3)/(1,6)-beta-glucan, a fungus-derived soluble prebiotic ameliorates high-fat diet-induced metabolic distress and alters beneficially the gut microbiota in mice model, Eur. J. Nutr., 2020, 59, 2617–2629 CrossRef CAS PubMed.
- Z. Li, C. X. Yi, S. Katiraei, S. Kooijman, E. Zhou, C. K. Chung, Y. Gao, J. K. van den Heuvel, O. C. Meijer, J. F. P. Berbée, M. Heijink, M. Giera, K. Willems van Dijk, A. K. Groen, P. C. N. Rensen and Y. Wang, Butyrate reduces appetite and activates brown adipose tissue via the gut-brain neural circuit, Gut, 2018, 67, 1269–1279 CrossRef CAS PubMed.
- P. Pathak, C. Xie, R. G. Nichols, J. M. Ferrell, S. Boehme, K. W. Krausz, A. D. Patterson, F. J. Gonzalez and J. Y. L. Chiang, Intestine farnesoid X receptor agonist and the gut microbiota activate G-protein bile acid receptor-1 signaling to improve metabolism, Hepatology, 2018, 68, 1574–1588 CrossRef CAS PubMed.
- K. Wang, M. Liao, N. Zhou, L. Bao, K. Ma, Z. Zheng, Y. Wang, C. Liu, W. Wang, J. Wang, S. J. Liu and H. Liu, Parabacteroides distasonis Alleviates Obesity and Metabolic Dysfunctions via Production of Succinate and Secondary Bile Acids, Cell Rep., 2019, 26, 222–235.e5 CrossRef CAS PubMed.
- R. Yoshitsugu, K. Kikuchi, H. Iwaya, N. Fujii, S. Hori, D. G. Lee and S. Ishizuka, Alteration of Bile Acid Metabolism by a High-Fat Diet Is Associated with Plasma Transaminase Activities and Glucose Intolerance in Rats, J. Nutr. Sci. Vitaminol., 2019, 65, 45–51 CrossRef CAS PubMed.
- D. W. Russell, The enzymes, regulation, and genetics of bile acid synthesis, Annu. Rev. Biochem., 2003, 72, 137–174 CrossRef CAS PubMed.
- P. Puri, K. Daita, A. Joyce, F. Mirshahi, P. K. Santhekadur, S. Cazanave, V. A. Luketic, M. S. Siddiqui, S. Boyett, H. K. Min, D. P. Kumar, R. Kohli, H. Zhou, P. B. Hylemon, M. J. Contos, M. Idowu and A. J. Sanyal, The presence and severity of nonalcoholic steatohepatitis is associated with specific changes in circulating bile acids, Hepatology, 2018, 67, 534–548 CrossRef CAS PubMed.
- H. Lastuvkova, F. A. Faradonbeh, J. Schreiberova, M. Hroch, J. Mokry, H. Faistova, Z. Nova, R. Hyspler, I. C. Igreja Sa, P. Nachtigal, A. Stefela, P. Pavek and S. Micuda, Atorvastatin Modulates Bile Acid Homeostasis in Mice with Diet-Induced Nonalcoholic Steatohepatitis, Int. J. Mol. Sci., 2021, 22, 6468 CrossRef CAS PubMed.
- E. Kwong, Y. Li, P. B. Hylemon and H. Zhou, Bile acids and sphingosine-1-phosphate receptor 2 in hepatic lipid metabolism, Acta Pharm. Sin. B, 2015, 5, 151–157 CrossRef PubMed.
- J. Y. L. Chiang and J. M. Ferrell, Bile acid receptors FXR and TGR5 signaling in fatty liver diseases and therapy, Am. J. Physiol.: Gastrointest. Liver Physiol., 2020, 318, G554–G573 CrossRef PubMed.
- H. Lin, Y. An, H. Tang and Y. Wang, Alterations of Bile Acids and Gut Microbiota in Obesity Induced by High Fat Diet in Rat Model, J. Agric. Food Chem., 2019, 67, 3624–3632 CrossRef CAS PubMed.
- J. O. Alemán, N. A. Bokulich, J. R. Swann, J. M. Walker, J. C. De Rosa, T. Battaglia, A. Costabile, A. Pechlivanis, Y. Liang, J. L. Breslow, M. J. Blaser and P. R. Holt, Fecal microbiota and bile acid interactions with systemic and adipose tissue metabolism in diet-induced weight loss of obese postmenopausal women, J. Transl. Med., 2018, 16, 244 CrossRef PubMed.
- B. L. Clifford, L. R. Sedgeman, K. J. Williams, P. Morand, A. Cheng, K. E. Jarrett, A. P. Chan, M. C. Brearley-Sholto, A. Wahlström, J. W. Ashby, W. Barshop, J. Wohlschlegel, A. C. Calkin, Y. Liu, A. Thorell, P. J. Meikle, B. G. Drew, J. J. Mack, H. U. Marschall, E. J. Tarling, P. A. Edwards and T. Q. de Aguiar Vallim, FXR activation protects against NAFLD via bile-acid-dependent reductions in lipid absorption, Cell Metab., 2021, 33, 1671–1684.e4 CrossRef CAS PubMed.
- C. Murphy, P. Parini, J. Wang, I. Björkhem, G. Eggertsen and M. Gåfvels, Cholic acid as key regulator of cholesterol synthesis, intestinal absorption and hepatic storage in mice, Biochim. Biophys. Acta, 2005, 1735, 167–175 CrossRef CAS PubMed.
- R. A. Haeusler, B. Astiarraga, S. Camastra, D. Accili and E. Ferrannini, Human insulin resistance is associated with increased plasma levels of 12alpha-hydroxylated bile acids, Diabetes, 2013, 62, 4184–4191 CrossRef CAS PubMed.
- J. Y. Lee, H. Shimizu, M. Hagio, S. Fukiya, M. Watanabe, Y. Tanaka, G. H. Joe, H. Iwaya, R. Yoshitsugu, K. Kikuchi, M. Tsuji, N. Baba, T. Nose, K. Tada, T. Hanai, S. Hori, A. Takeuchi, Y. Furukawa, B. Shirouchi, M. Sato, T. Ooka, Y. Ogura, T. Hayashi, A. Yokota and S. Ishizuka, 12alpha-Hydroxylated bile acid induces hepatic steatosis with dysbiosis in rats, Biochim. Biophys. Acta, Mol. Cell Biol. Lipids, 2020, 1865, 158811 CrossRef CAS PubMed.
- P. Song, Y. Zhang and C. D. Klaassen, Dose-response of five bile acids on serum and liver bile Acid concentrations and hepatotoxicty in mice, Toxicol. Sci., 2011, 123, 359–367 CrossRef CAS PubMed.
- B. L. Woolbright, F. Li, Y. Xie, A. Farhood, P. Fickert, M. Trauner and H. Jaeschke, Lithocholic acid feeding results in direct hepato-toxicity independent of neutrophil function in mice, Toxicol. Lett., 2014, 228, 56–66 CrossRef CAS PubMed.
- S. Naumann, U. Schweiggert-Weisz, J. Eglmeier, D. Haller and P. Eisner, In Vitro Interactions of Dietary Fibre Enriched Food Ingredients with Primary and Secondary Bile Acids, Nutrients, 2019, 11, 1424 CrossRef CAS PubMed.
- E. Muls, J. Kolanowski, A. Scheen, L. Van Gaal and ObelHyx Study Group, The effects of orlistat on weight and on serum lipids in obese patients with hypercholesterolemia: a randomized, double-blind, placebo-controlled, multicentre study, Int. J. Obes. Relat. Metab. Disord., 2001, 25, 1713–1721 CrossRef CAS PubMed.
|
This journal is © The Royal Society of Chemistry 2022 |