DOI:
10.1039/D2FO00672C
(Paper)
Food Funct., 2022,
13, 7614-7628
Bioavailability and biotransformation of linolenic acid from basil seed oil as a novel source of omega-3 fatty acids tested on a rat experimental model
Received
8th March 2022
, Accepted 6th June 2022
First published on 7th June 2022
Abstract
Basil is an aromatic herb with a high concentration of bioactive compounds. The oil extracted from its seeds is a good source of α-linolenic acid (ALA) and also provides substantial amounts of linoleic acid (LA). This study aimed to test the bioavailability of the oil derived from basil seeds and its effects on different physiological parameters using 7–15% dietary inclusion levels. Furthermore, the assimilation of LA and ALA and their transformation in long-chain polyunsaturated fatty acids (LC-PUFAs) have been studied. Digestive utilization of total fat from basil seed oil (BSO) was high and similar to that of olive oil used as a control. Consumption of BSO resulted in increased LA and ALA levels of the plasma, liver, and erythrocyte membrane. In addition, the transformation of LA to arachidonic acid (ARA) was decreased by the high dietary intake of ALA which redirected the pathway of the Δ-6 desaturase enzyme towards the transformation of ALA into eicosapentaenoic acid (EPA). No alterations of hematological and plasma biochemical parameters were found for the 7 and 10% dietary inclusion levels of BSO, whereas a decrease in the platelet count and an increase in total- and HDL-cholesterol as well as plasma alkaline phosphatase (ALP) were found for a 15% BSO dose. In conclusion, BSO is a good source of ALA to be transformed into EPA and decrease the precursor of the pro-inflammatory molecule ARA. This effect on the levels of EPA in different tissues offers potential for its use as a dietary supplement, novel functional food, or a constituent of nutraceutical formulations to treat different pathologies.
1. Introduction
Ocimum basilicum L. (Basil) is an aromatic herb belonging to the Labiatae family and features among the most economically important species within the Ocimum genus. Basil seeds are interesting sources of bioactive compounds such as flavonoids and polyphenols, with rosmarinic acid being among the most important.1 Furthermore, they are used for biotechnological purposes due to the presence of gum, which has been isolated and widely studied for its emulsifying, gelling, and foaming properties.2 Concerning the nutritional composition, less attention has been paid to basil seeds. Nevertheless, the fat content of these seeds, which ranges from 13 to 15% of the total weight, exhibits a very interesting fatty acid profile from a nutritional and functional point of view since α-linolenic acid (ALA) is the main component of the lipid fraction that ranges from 60 to 80% of the total fatty acid content depending on the geographical and soil conditions as well as on the genotype and cultivar.3 In addition, basil seeds contain considerable amounts of linoleic acid (LA) that can exceed 20% of the total fatty acid composition. ALA (C18:3n-3) and LA (C18:2n-6) are essential fatty acids for humans4 since they cannot form double bonds in polyunsaturated fatty acids (PUFAs) between the methyl end and the middle of the molecule (Δ-9 desaturase activity).5 Therefore, these fatty acids must be provided by the diet to carry out their functions in the human body. LA is an energy source that can form phospholipids to maintain the fluidity of the phospholipid membrane and has functions in cell signaling.6 Moreover, LA is involved in growth, reproduction, and skin function and is the precursor of arachidonic acid (ARA). The best-known functions of ALA are its involvement in carbon recycling for de novo lipogenesis, its incorporation as part of the skin or fur, and its preferred metabolic pathway as the substrate for β-oxidation.5 Besides, LA and ALA are precursors of the longer chain n-3 polyunsaturated fatty acids (PUFA) eicosapentaenoic, docosapentaenoic and docosahexaenoic acids (EPA, DPA and DHA, respectively).
In recent years, the protective role of ALA against several diseases has been extensively studied. Its neuroprotective properties are worth mentioning,7,8 as well as its ability to promote osteoblastic function9 and capacity to improve endothelial dysfunction and ameliorate hypertension.10 In addition, higher circulating levels of n-3 fatty acids have been associated with a lower risk of type 2 diabetes.11 Omega-3 fatty acids in general, and, specifically, linolenic acid, have received increased attention for their anti-inflammatory properties.12 The inclusion of ALA-rich oil in a high-fat diet inhibited foam cell formation in atherosclerosis,13 leukotriene B4 (LTB4) release in some respiratory diseases,14 and decreased expression of NFκB-p65 and SREBP-1c in subclinical mastitis.15 These effects could be mediated by EPA, DHA and ARA acid metabolites which are precursors for the synthesis of specialized proresolving mediators (SPMs) as resolvins, protectins, maresins and lipoxins,16 as well as, SPMs derived from n-3 DPA with an important role in the resolution of inflammation.17
The optimal dietary ratio of LA/ALA to promote an effective transformation of ALA to EPA and DHA is controversial but it has been suggested by several studies that the 3–4
:
1 ratio prevents the development of different pathologies.18 In the past decades, LA intake in the western diet experienced a huge growth due to the consumption of soybean and corn oil. This has raised the LA/ALA ratio to 10–20
:
1, increasing the risk of cancer, cardiovascular and inflammatory diseases.19 A good strategy for reverting this situation is to encourage omega-3 intake from different dietary sources, although it must be taken into account that LA has important functions in the body and its consumption should be decreased but not ceased.
Nowadays, the main approach to increase the consumption of omega-3 fatty acids is based on marine products such as fish and krill oil in food,20 but sometimes their organoleptic properties are not well accepted by the general population. Some vegetable sources of omega-6 and omega-3 like linseed, chia, or perilla seed oil have been considered for inclusion as dietary fats.21,22 An essential consideration about new sources of omega-3 fatty acids is knowing the real bioavailability and transformation of these fatty acids when they are consumed. Therefore, this work aimed to obtain a good source of omega-6 and omega-3 fatty acids from basil seed oil, and test its digestive utilization, potential interaction with the nutritive utilization of dietary protein, and lack of significant adverse effects. Besides, in this study, we assessed the biotransformation of fatty acids present in basil seed oil by measuring the occurrence of their by-products in the plasma, liver, and erythrocyte membrane using an in vivo experimental model of Wistar rats.
2. Materials and methods
2.1. Basil seeds and basil seed oil
Ocimum basilicum var. minimum L. seeds were obtained from Agrointec Solutions S.L. (Almería, Spain). The oil from the seed was extracted by CELLBITEC S.L. Briefly, the seeds of Ocimun basilicum were defatted separating the oleaginous part of the mature seed using a cold seed oil extraction press (KOMET series) without exceeding 40 °C. The average working speed of the extraction process was 2–3 kg seed h−1, and the average extraction yield ranged from 16 to 23%. As a result of the former processing conditions, pure basil seed oil was obtained.
2.2. Lipid profiles of olive and basil seed oil
Aliquots of commercial olive oil and basil seed oil (0.05 mL) were methylated according to Lepage & Roy23 for gas chromatography analysis of the fatty acid profile using an Agilent 7890A chromatograph equipped with a CTC Pal combi-xt model sampler and a Waters Quattro micro GC mass spectrometer detector as previously described.24 Individual fatty acid methyl esters (FAMEs) were separated using a 30 m × 0.25 mm ZB Fame capillary column (0.2 μm thickness) (Phenomenex, Torrance, CA, USA). FAMEs were identified using analytical standards (FAME mix, 37 Component, TraceCERT®, and Supelco®) and mass spectral library. Peak areas were measured and used to calculate the percentage of each fatty acid (Food Analysis Unit and Structural Assessment Analysis Unit CIC, University of Granada). In addition, a complete profile of all lipid components in basil oil was obtained. Tocopherol analysis was carried out by high-performance liquid chromatography (HPLC), whereas diterpenoid, aliphatic and triterpene alcohols were analyzed using gas chromatography at the Instituto de la Grasa of the Spanish National Research Council (CSIC, Spain).
2.3. Animals and experimental diets
A total of thirty-two male Wistar rats (Charles Rives, Barcelona) aged 6 weeks with an initial average body weight of 137.0 ± 1.33 g were randomly divided into four experimental groups of eight animals each with the following design: a control group fed olive oil as the only dietary fat source (OLO 7%) and three experimental groups fed basil seed oil at 7, 10, and 15% dietary inclusion levels (BSO 7%, BSO 10% and BSO 15%, respectively). All diets were formulated following the recommendations to meet the nutrient requirements of the growing rat.25 The composition of the different oils and experimental diets is shown in Tables 1–3. The animals were housed in a well-ventilated and thermostatically controlled room (21 ± 2 °C) (Animal Experimental Unit, CIC, University of Granada), they consumed the diet ad libitum, and had free access to type 2 water. The experiments lasted for 55 days, and in the first three days, the animals were allowed to adapt to the experimental diet and housing conditions. Then, six animals from each experimental group were housed individually in metabolic cages to allow a separate collection of feces and urine for 5 days to determine the digestive and metabolic utilization of protein and fat. After the bioavailability assessment period, the animals were allocated in group cages (n = 4) where they were kept until the end of the experiment. Food intake was recorded daily, whereas body weight was determined weekly. All experiments were performed according to Directional Guides Related to Animal Housing and Care,26 and all procedures were approved by the Animal Experimentation Ethics Committee of the University of Granada, Spain (project number 16/07/2019/132). To select the number of rats assigned to each experimental group (n = 8), we implemented the 3Rs principle.27 At the end of the experimental period, the animals were anesthetized with ketamine (75 mg kg−1 body weight) and xylazine (10 mg kg−1 body weight), and the blood was collected by abdominal aorta puncture using heparin as an anticoagulant. An aliquot of 0.25 mL was used to assess blood parameters (KX-21 Automated Hematology Analyzer, Sysmex Corporation), and the rest was centrifuged at 1458g for 15 min to separate plasma that was subsequently frozen in liquid nitrogen and stored at −80 °C until its analysis. The erythrocyte fraction was collected, washed twice with physiological saline solution, and also stored at 80 °C. The kidneys, liver, and heart were removed and weighed to check the lack of adverse effects of the basil seed oil on these organs. A portion of the liver was freeze-dried and processed to determine the amount of total fat and fatty acid profile.
Table 1 Fatty acid profiles of olive (OLO) and basil seed (BSO) oilsa
Fatty acid (%) |
Olive oil |
Basil seed oil |
Data are the results of 4 independent measures.
|
Lauric acid (C12) |
<0.05% |
<0.01% |
Myristic acid (C14) |
<0.05% |
<0.04% |
Palmitic acid (C16) |
7.9 ± 0.63 |
4.1 ± 0.56 |
Palmitoleic acid (C16:1) |
<0.9% |
<0.3% |
Margaric acid (C17) |
<0.05% |
<0.08% |
Margaroleic acid (C17:1) |
<0.09% |
<0.05% |
Stearic acid (C18) |
2.1 ± 0.31 |
1.6 ± 0.40 |
Oleic acid (C18:1n9) |
84.0 ± 2.10 |
7.8 ± 1.46 |
Linoleic acid (C18:2n6) |
3.3 ± 0.21 |
14.8 ± 1.67 |
Arachidic acid (C20) |
<0.05% |
<0.5% |
α-Linolenic acid (C18:3n3) |
— |
71.7 ± 4.89 |
Eicosenoic (C20:1) |
— |
<0.15% |
Behenic acid (C22) |
<0.05% |
<0.04% |
Erucic acid (C22:1) |
<0.05% |
<0.04% |
Lignoceric acid (C24) |
<0.9% |
<0.03% |
Table 2 Profile of lipid components in basil seed oil
Tocopherols (mg kg−1) |
Basil seed oil |
Olive oil |
Data from ref. 67.
Data from ref. 68.
Data from ref. 69.
Data from ref. 70.
Data from ref. 71.
Data from ref. 72.
|
α-Tocopherol (vit. E) |
<2 |
92–208a |
β-Tocopherol |
<2 |
0.75–1.03a |
γ-Tocopherol |
976 |
0.70–2.15a |
Δ-Tocopherol |
12 |
— |
Total tocopherols |
988 |
93–210a |
Diterpenoid alcohols (mg kg
−1
)
|
Phytol |
534 |
25–595f |
Geraniol |
22 |
<50f |
Aliphatic alcohols (mg kg
−1
)
|
C-22-OH |
41 |
35.8b |
C-24-OH |
8 |
39.6b |
C-26-OH |
18 |
32.7b |
C-28-OH |
78 |
2.01b |
Total alcohols (C-22 to C-28) |
145 |
110.1 |
Triterpene alcohols (mg kg
−1
)
|
β-Armyrin |
142 |
10.0c |
Butyrospermol |
91 |
— |
Cycloartenol (CA) |
72 |
128.2c |
24-Methylene-cycloartenol |
44 |
413.5c |
Citrostadienol |
194 |
56.8c |
Sterols (%)
|
Cholesterol |
0.8 |
0.02–0.90d |
Brassicasterol |
<0.1 |
0.01–0.08d |
24-Methylene cholesterol |
0.1 |
0.00–0.45d |
Campesterol |
15.1 |
2.4–5.1d |
Campestanol |
0.4 |
0.05–0.47d |
Stigmasterol |
4.7 |
0.15–1.90d |
Δ-7-Campesterol |
0.9 |
— |
Δ-5,23-Stigmastadienol |
<0.1 |
0.51–0.76e |
Clerosterol |
1.1 |
0.7–1.55d |
β-Sitosterol |
52.4 |
74.2–89.3d |
Sitostanol |
1.1 |
0.00–2.0d |
Δ-5-Avenasterol |
17.5 |
2.6–18.6d |
Δ-5,24-Stigmastadienol |
2 |
0.20–1.46d |
Δ-7-Stigmasterol |
1.2 |
0.10–0.45d |
Δ-7-Avenasterol |
2.7 |
0.20–0.96d |
Apparent β-sitosterol |
74.1 |
88–96d |
Total sterols (ppm) |
2880 |
20.99–2378d |
Table 3 Formulation, proximate composition and fatty acid profile of the four experimental diets
g per 100 g of diet |
OLO 7% |
BSO 7% |
BSO 10% |
BSO 15% |
40% of starch was included as dextrin; OLO: olive oil used as dietary fat; BSO: basil seed oil used as dietary fat at different inclusion percentages.
|
Formulation of experimental diets
|
Casein |
16 |
16 |
16 |
16 |
Methionine |
0.5 |
0.5 |
0.5 |
0.5 |
Sucrose |
10 |
10 |
10 |
10 |
Fat |
7 |
7 |
10 |
15 |
Cellulose |
5 |
5 |
5 |
5 |
Mineral mix |
3.5 |
3.5 |
3.5 |
3.5 |
Vitamin mix |
1 |
1 |
1 |
1 |
Choline |
0.25 |
0.25 |
0.25 |
0.25 |
Starcha |
56.5 |
56.5 |
53.5 |
48.5 |
Nutritional composition
|
Water |
8.79 |
8.85 |
6.65 |
7.86 |
Ash |
2.41 |
2.33 |
2.43 |
2.37 |
Protein |
14.1 |
14.1 |
14.5 |
14.2 |
Fat |
6.84 |
5.75 |
9.51 |
13.77 |
Carbohydrates |
67.9 |
69.0 |
67.1 |
61.8 |
Fatty acid (%)
|
Palmitic (C16) |
9.75 |
8.74 |
6.73 |
7.11 |
Stearic (C18) |
2.34 |
3.50 |
2.58 |
2.38 |
Oleic (Z C18:1n9) |
84.21 |
10.92 |
10.86 |
10.87 |
Linoleic (Z C18:2n6) |
3.69 |
19.46 |
18.51 |
18.56 |
α-Linolenic (C18:3n3) |
— |
57.4 |
61.3 |
61.1 |
SFA |
12.09 |
12.25 |
9.30 |
9.49 |
MUFA |
84.2 |
10.9 |
10.9 |
10.9 |
PUFA |
3.69 |
76.84 |
79.84 |
79.63 |
2.4. Biological indices
The following indices and parameters were determined for each group according to the formulas given below and following the methodology described by Kapravelou et al.:28 protein efficiency ratio (PER: weight gain in grams per day/protein intake in grams per day); food transformation index (FTI: total intake in grams of dry matter per day/increase in body weight in grams per rat per day); apparent digestibility coefficient of protein and total fat (ADC) (1); nitrogen retention (nitrogen balance) (2); and percent nitrogen retention/nitrogen absorption (% R/A) (3). | ADC = [(I − F)/I] × 100 | (1) |
| % R/A = {[I − (F + U)]/(I − F)} × 100 | (3) |
where I = intake, F = fecal excretion, and U = urinary excretion.
2.5. Plasma biochemical parameters
Triglycerides (mg dL−1), total cholesterol, HDL-cholesterol, LDL-cholesterol (mg dL−1), albumin (g dL−1), creatinine (mg dL−1), aspartate aminotransferase (U L−1), alanine aminotransferase (U L−1), gamma-glutamyl transferase (U L−1), alkaline phosphatase (U L−1) and phosphorus (mg dL−1) were analyzed using a Shenzhen Midray BS-200 Chemistry Analyzer (Bio-Medical Electronics) at the Bioanalysis Unit of the Scientific Instrumentation Center (CIC, Centre of Biomedical Research, University of Granada).
2.6. Fatty acid profile of the plasma, liver, and erythrocyte membrane
A portion of the liver was freeze-dried and 0.1 g were extracted and then methylated following the same methodology used for the determination of fatty acid profiles in olive oil and basil seed oil. One mL of erythrocytes was extracted sequentially using 0.05% butyl hydroxytoluene in isopropanol, chloroform, and hexane. After hexane extraction, the samples were centrifuged at 1458g for 10 min. The upper phase was collected, evaporated under a nitrogen stream, and subsequently methylated. Plasma aliquots (100 μL) were directly methylated.
Pearson's test was carried out on the different fatty acid profiles to test the correlation between the percentage of basil seed oil included in the different experimental diets and the percentage of individual fatty acids present in each compartment studied: plasma, liver, and erythrocyte membrane. Where the Pearson's test showed r > 0.6 and p < 0.01, a linear regression model was run to predict the percentage of each fatty acid to the amount of basil seed oil included in the diet. Furthermore, some product to precursor fatty acid ratios were used as indices of desaturase or desaturase–elongase enzyme activities in the liver as described by González-Torres et al.29 using the following formulas:
Delta-6-elongase-desaturase:
(i) Docosahexaenoic acid/linolenic acid (DHA/ALA).
(ii) Arachidonic acid/linoleic acid (ARA/LA).
Stearoyl-coA activity (SCD):
(i) Palmitoleic acid/palmitic acid (PE/PI).
(ii) Oleic acid/stearic acid (OLE/STE).
Delta-5 desaturase activity:
(i) Arachidonic acid/eicosatrienoic acid (ARA/EI).
2.7. Total hepatic lipid content
A liver portion was lyophilized to determine the percentage of water. Hepatic lipids were extracted with hexane from an aliquot of the freeze-dried liver portion using the method described by Folch et al.30 with slight modifications.24 Total liver lipids were measured gravimetrically after solvent extraction under a nitrogen stream.
2.8. Statistical analysis
The influence of dietary fat type and inclusion levels on food intake, body weight, digestive and metabolic utilization of protein and fat, hematological and plasma biochemical parameters, fatty acid profiles of plasma, liver, and erythrocyte membrane, and fatty acid indices was analyzed by a one-way ANOVA. The results are given as mean values and standard error of the mean. Tukey's test was used to detect differences between treatment means. Data on specific fatty acid profiles (oleic, linoleic, linolenic, arachidonic, eicosapentaenoic, and docosahexaenoic acids) in response to the inclusion levels of BSO were adjusted to a lineal regression model in each of the three compartments (plasma, liver, and erythrocytes) studied. The analyses were performed with Statistical Package for Social Sciences (IBM SPSS for Windows®, version 22.0, Armonk, NY), and the level of significance was set at p < 0.05.
3. Results
3.1. Fatty acid profiles of olive and basil seed oils
The fatty acid profiles of olive and basil seed oils are shown in Table 1. Olive oil was mainly composed of oleic acid (nearly 90% of the total) followed by palmitic and linoleic acids, whereas basil seed oil had linolenic acid as its major component (71.7%) followed by 14.8% of linoleic acid. The lipid components of basil seed oil and olive oil are presented in Table 2. Among all the compounds measured in BSO, the presence of γ-tocopherol, phytol, β-armyrin and β-sitosterol that exhibited higher concentrations within each class of alcohol assessed is worth mentioning. Bibliographic data from olive oil show lower contents of γ-tocopherol, β-armyrin, campesterol and stigmasterol compared to BSO, whereas the levels of α-tocopherol, β-sitosterol and apparent β-sitosterol were markedly higher.
3.2. Food intake and body weight
The evolution of food intake and body weight of the four experimental groups is presented in Fig. 1. The inclusion of basil seed oil in the experimental diet did not interfere with food intake, since no differences were observed in this parameter between animals consuming olive oil as a source of dietary fat at 7% and those consuming BSO at the same percentage. The increase in dietary inclusion of BSO led to a decrease in food intake which was more pronounced for the animals that consumed the diet formulated with the highest percentage of BSO (15%). Nevertheless, the caloric intake was adjusted by the animals and no significant differences were found among the four experimental groups.
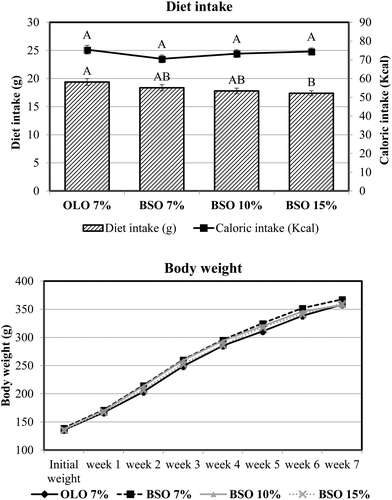 |
| Fig. 1 Food intake, caloric intake, and body weight of rats fed the different experimental diets. OLO: olive oil used as dietary fat; BSO: basil seed oil used as dietary fat. Results are means of 8 rats. A and B mean within the same parameter with different letters indicating significant difference (ANOVA treatment, p < 0.05). | |
3.3. Digestive and metabolic utilization of protein and fat
Food intake, body weight gain, fecal weight, and digestive and metabolic utilization of protein and fat in the control and experimental groups are presented in Table 4. The protein efficiency ratio (PER) of the animals was not affected by the type of oil consumed or the percentage of BSO included in the experimental diet. However, a slight decrease in the food transformation index (FTI) was observed at higher percentages of dietary fat (10 and 15%) due to the lower amount of food ingested.
Table 4 Food intake, weight gain, fecal excretion, digestive and metabolic utilization of protein and fat in the four experimental groups
|
OLO 7% |
BSO 7% |
BSO 10% |
BSO 15% |
OLO: olive oil used as dietary fat, BSO: basil seed oil used as dietary fat, DM: dry matter, PER: protein efficiency ratio, FTI: food transformation index, ADC: apparent digestibility coefficient, R/A: percent nitrogen retention/nitrogen absorption. Results are means of 8 rats. a–d mean within the same line with different letters indicating significant difference (P < 0.05). |
Food intake (g DM d−1) |
19.4 (0.55) a |
19.1 (0.41) a |
18.9 (0.68) a |
17.8 (0.42) a |
Protein intake (g d−1) |
2.72 (0.08) a |
2.69 (0.06) a |
2.74 (0.10) a |
2.54 (0.06) a |
Weight gain (g d−1) |
7.31 (0.28) a |
7.15 (0.14) a |
7.97 (0.61) a |
7.76 (0.26) a |
PER |
2.67 (0.08) a |
2.66 (0.04) a |
2.89 (0.13) a |
3.00 (0.07) a |
FTI |
2.66 (0.08) b |
2.67 (0.05) b |
2.42 (0.10) ab |
2.33 (0.06) a |
Faecal weight (g DM d−1) |
1.45 (0.06) a |
1.45 (0.04) a |
1.46 (0.04) a |
1.38 (0.03) a |
N intake (mg d−1) |
436.1 (12.29) a |
429.9 (9.17) a |
438.4 (15.66) a |
405.8 (9.47) a |
Fecal N (mg d−1) |
20.7 (0.68) a |
20.9 (0.87) a |
20.3 (1.04) a |
19.50 (0.91) a |
Urinary N (mg d−1) |
141.2 (6.76) b |
115.8 (4.09) a |
122.5 (6.13) ab |
180.8 (7.48) a |
Absorbed N (mg d−1) |
415.4 (12.15) a |
409.01 (8.62) a |
417.9 (15.92) a |
386.3 (9.72) a |
N ADC (%) |
95.2 (0.18) a |
95.2 (0.16) a |
95.3 (0.32) a |
95.2 (0.28) a |
Balance (mg d−1) |
274.2 (8.93) a |
293.2 (6.73) a |
295.5 (11.16) a |
277.5 (7.38) a |
N % R/A |
66.0 (1.13) a |
71.7 (0.76) b |
70.7 (0.81) b |
71.9 (1.52) b |
Fat intake (g d−1) |
1325.6 (37.4) b |
1096.5 (23.4) a |
1803.1 (64.4) c |
2454.0 (57.3) d |
Fecal fat (mg d−1) |
24.7 (1.37) ab |
22.7 (1.08) a |
31.1 (1.21) b |
43.3 (3.23) c |
Fat ADC (%) |
98.2 (0.09) a |
97.9 (0.10) a |
98.3 (0.09) a |
98.2 (0.14) a |
In vivo protein and fat bioaccessibility assessed by the apparent digestibility coefficient (ADC) was also unaffected by the inclusion of BSO as the fat source, with values above 95 and 98% for protein and fat digestibility, respectively. On the other hand, metabolic utilization of nitrogen assessed as % R/A was higher in animals fed the basil seed oil (Table 4).
3.4. Hematological and plasma biochemical parameters
The effects of basil seed oil on hematological and plasma biochemical parameters are shown in Table 5. Regarding the hematological parameters, no signs of metabolic alterations were found due to the consumption of BSO as the fat source since no significant differences were found between the animals fed an OLO diet at 7% and the animals that consumed BSO at the same percentage. Nevertheless, increasing the percentage of fat included in the diet negatively affected some parameters related to iron metabolism such as hemoglobin concentration and hematocrit. Increasing the BSO content in the experimental diets up to 15% caused a significant collateral increase in total cholesterol of the plasma of the animals, mostly in the form of HDL-cholesterol. Likewise, this high level of dietary fat intake caused a significant increase in the plasma concentration of hepatic enzymes such as alanine aminotransferase (ALT) and alkaline phosphatase (ALP).
Table 5 Effect of basil seed oil inclusion levels on haematological and plasma biochemical parameters
|
OLO 7% |
BSO 7% |
BSO 10% |
BSO 15% |
WBC, white blood cells, RBC, red blood cells, HGB, hemoglobin, HCT, hematocrit, PLT, platelet, MCV, mean corpuscular volume, MCH, mean corpuscular hemoglobin, MCHC, mean corpuscular hemoglobin content, RDW, red cell distribution width. OLO, olive oil used as dietary fat, BSO, basil seed oil used as dietary fat, TG, triglycerides, T-CHOL, total cholesterol, HDL-CHOL, HDL-cholesterol, LDL-CHOL, LDL-cholesterol, AST, aspartate aminotransferases, ALT, alanine aminotransferase, γ-GT, gamma glutamyl transferase, ALP, alkaline phosphatase, P, phosphorus. Results are means of 8 rats. a and b mean within the same line with different letters indicating significant difference (P < 0.05). |
Haematological parameters
|
WBC (×103 μl−1) |
3.08 (0.29) a |
4.06 (0.33) a |
4.01 (0.39) a |
2.96 (0.19) a |
RBC (×106 μl−1) |
7.14 (0.08) a |
7.38 (0.05) ab |
7.76 (0.14) b |
6.98 (0.34) a |
HGB (g dL−1) |
13.9 (0.17) ab |
14.5 (0.14) b |
14.8 (0.23) b |
12.7 (0.63) a |
HCT (%) |
36.0 (0.57) ab |
36.6 (0.39) ab |
37.6 (0.66) b |
32.8 (1.79) a |
PLT (×103 μl−1) |
609.7 (12.72) b |
530.4 (28.65) ab |
525.0 (15.48) ab |
479.5 (29.64) a |
MCV (fL) |
50.5 (0.58) a |
49.6 (0.56) a |
48.5 (0.45) a |
49.0 (0.37) a |
MCH (pg) |
19.5 (0.26) a |
19.7 (0.21) a |
19.1 (0.18) a |
19.0 (0.18) a |
MCHC (g dL−1) |
38.7 (0.28) a |
39.8 (0.09) b |
39.4 (0.12) ab |
38.8 (0.32) a |
RDW (fL) |
16.1 (0.22) a |
16.2 (0.18) a |
16.6 (0.37) a |
16.1 (0.19) a |
Plasma biochemical parameters
|
TG (mg dL−1) |
73.7 (12.68) a |
62.4 (6.75) a |
72.1 (1.51) a |
85.9 (6.99) a |
T-CHOL (mg dL−1) |
46.8 (3.21) ab |
39.3 (3.58) a |
48.1 (5.61) ab |
56.5 (3.97) b |
HDL-CHOL (mg dL−1) |
30.9 (1.97) ab |
22.4 (1.95) a |
29.4 (2.16) a |
39.3 (2.73) b |
LDL-CHOL (mg dL−1) |
4.40 (0.26) a |
3.62 (0.23) a |
4.90 (0.60) a |
4.99 (0.71) a |
Albumin (g dL−1) |
3.47 (0.05) a |
3.56 (0.03) a |
3.58 (0.03) a |
3.51 (0.02) a |
Creatinine (mg dL−1) |
0.33 (0.02) a |
0.35 (0.05) a |
0.32 (0.01) a |
0.33 (0.04) a |
AST (U L−1) |
67.7 (9.87) a |
72.1 (2.23) a |
64.8 (2.03) a |
67.3 (2.47) a |
ALT (U L−1) |
17.6 (1.60) a |
19.9 (0.85) a |
20.5 (0.86) a |
25.9 (1.18) b |
γ-GT (U L−1) |
4.24 (0.65) a |
3.69 (0.25) a |
4.09 (0.42) a |
3.26 (0.37) a |
ALP (U L−1) |
95.4 (6.23) a |
76.2 (3.59) a |
78.6 (5.81) a |
209.4 (15.05) b |
P (mg dL−1) |
5.6 (0.30) a |
5.6 (0.21) a |
5.5 (0.27) a |
5.8 (0.30) a |
3.5. Liver parameters
The influence of different basil seed oil inclusion levels on liver weight and liver fat content is presented in Fig. 2. Liver weight was affected by the BSO percentage consumed, since the former parameter was significantly increased in the animals fed the diet with BSO 15%, compared to the other two dietary fat concentrations tested (7 and 10%). Nevertheless, the percentage of liver fat did not differ among the four experimental groups although the total liver fat was significantly higher in the BSO 15% group due to its larger liver weight.
 |
| Fig. 2 Liver weight, hepatic fat percentage, and total liver fat of rats fed the different experimental diets. OLO: olive oil used as dietary fat; BSO: basil seed oil used as dietary fat. Results are means of 8 rats. Labels A and B mean within the same parameter with different letters indicating significant difference (ANOVA treatment, p < 0.05). | |
3.6. Fatty acid profile of the plasma, liver, and erythrocyte membrane
The influence of BSO inclusion on the fatty acid profile of the plasma, liver, and erythrocyte membrane is presented in Table 6. The effects of BSO intake were the same in all three compartments studied. Oleic and arachidonic acids were significantly lower in animals that consumed BSO compared to animals that consumed OLO as a fat source. On the other hand, BSO intake significantly increased the percentage of LA in the plasma, liver, and erythrocyte membrane. Furthermore, this increment paralleled the increased percentage of BSO included in the diet. ALA and EPA were not present in the plasma, liver, or erythrocyte membrane of animals that consumed the OLO diet, but BSO intake resulted in the presence of ALA and EPA in these three compartments.
Table 6 Influence of the percentage of basil oil included in the experimental diets on the fatty acid profile (%) of the plasma, liver and erythrocyte membrane
|
OLO 7% |
BSO 7% |
BSO 10% |
BSO 15% |
OLO, olive oil used as dietary fat, BSO, basil seed oil used as dietary fat. Results are means of 8 rats. a–c mean within the same line in each specific tissue component or organ with different letters indicating significant difference (p < 0.05). |
Liver
|
Myristic acid (C14) |
0.31 (0.02) b |
0.35 (0.03) b |
0.28 (0.02) ab |
0.22 (0.01) a |
Pentadecanoic acid (C15) |
— |
— |
— |
0.10 (0.01) |
cis-10-Pentadecenoic acid (C15:1) |
— |
— |
— |
0.07 (0.00) |
Palmitic acid (C16) |
21.0 (0.48) a |
24.5 (0.91) b |
21.2 (0.55) a |
20.3 (0.54) a |
Palmitoleic acid (Z C16:1n9) |
2.13 (0.13) bc |
2.79 (0.31) c |
1.51 (0.19) ab |
0.83 (0.06) a |
Margaric acid (C17) |
— |
— |
0.18 (0.06) a |
0.18 (0.01) a |
Stearic acid (C18) |
17.8 (1.14) a |
16.0 (0.86) a |
17.2 (0.97) a |
15.9 (0.91) a |
Oleic acid (Z C18:1n9) |
28.4 (2.50) b |
12.5 (0.96) a |
9.75 (0.54) a |
8.97 (0.53) a |
Linolelaidic acid (E C18:2n6) |
— |
— |
0.19 (0.02) a |
0.17 (0.01) a |
Linoleic acid (Z C18:2n6) |
5.70 (0.39) a |
17.1 (0.64) b |
19.7 (0.57) c |
21.6 (0.43) c |
Linolenic acid (C18:3n3) |
— |
11.87 (1.11) a |
14.51 (1.28) a |
17.67 (1.25) a |
Arachidic acid (C20) |
— |
— |
0.22 (0.03) a |
0.35 (0.03) b |
cis-11.14-Eicosadienoic acid (C20:2) |
1.22 (0.10) |
— |
— |
— |
cis-8.11.14-Eicosatrienoic acid (C20:3n6) DGLA |
0.46 (0.03) b |
0.30 (0.04) a |
0.38 (0.04) ab |
0.44 (0.02) b |
Arachidonic acid (C20:4n6) AA (W6) |
17.4 (1.17) b |
5.89 (0.61) a |
6.06 (0.32) a |
5.38 (0.29) a |
Eicosapentaenoic acid (C20:5n3) EPA |
— |
4.27 (0.25) a |
4.77 (0.54) a |
4.63 (0.27) a |
Lignoceric acid (C24) |
0.36 (0.02) a |
0.36 (0.03) a |
0.40 (0.04) a |
0.35 (0.02) a |
Docosahexaenoic acid (C22:6n3) DHA |
2.85 (0.20) a |
2.13 (0.21) a |
2.41 (0.21) a |
2.09 (0.36) a |
C18:1n7 acid (W7) |
2.25 (0.08) b |
1.99 (0.05) b |
1.60 (0.07) a |
1.56 (0.07) a |
Plasma
|
Myristic acid (C14) |
0.85 (0.08) |
— |
— |
— |
Palmitic acid (C16) |
32.5 (0.58) ab |
35.1 (1.28) b |
34.1 (1.19) b |
28.8 (1.03) a |
Palmitoleic acid (Z C16:1n9) |
2.13 (0.16) a |
2.15 (0.16) a |
2.28 (0.10) a |
— |
Stearic acid (C18) |
16.8 (1.37) a |
17.8 (2.03) a |
17.8 (1.00) a |
18.2 (0.91) a |
Oleic acid (Z C18:1n9) |
27.3 (1.69) b |
10.0 (0.78) a |
7.9 (0.42) a |
6.8 (0.41) a |
Linoleic acid (Z C18:2n6) |
4.85 (0.15) a |
16.2 (0.69) b |
19.2 (0.71) c |
23.7 (0.56) d |
Linolenic acid (C18:3n3) |
— |
8.61 (1.13) a |
9.56 (0.90) a |
12.6 (1.55) a |
Arachidonic acid (C20:4n6) AA (W6) |
14.2 (0.67) b |
6.07 (0.55) a |
5.82 (0.23) a |
5.80 (0.52) a |
Eicosapentaenoic acid (C20:5n3) EPA |
— |
3.98 (0.33) a |
4.39 (0.64) a |
3.45 (0.26) a |
Docosahexaenoic acid (C22:6n3) DHA |
0.76 (0.05) a |
1.23 (0.18) a |
2.08 (0.49) a |
— |
C18:1n7 acid (W7) |
1.76 (0.04) a |
1.60 (0.12) a |
— |
— |
Erythrocyte membrane
|
Caprylic acid (C8) |
1.06 (0.29) a |
1.25 (0.21) a |
1.31 (0.14) a |
1.25 (0.26) a |
Myristic acid (C14) |
0.36 (0.08) |
— |
— |
— |
Pentadecanoic acid (C15) |
0.11 (0.08) |
— |
— |
— |
cis-10-Pentadecenoic acid (C15:1) |
0.16 (0.11) |
— |
— |
— |
Palmitic acid (C16) |
39.7 (0.72) b |
43.7 (0.93) b |
43.4 (0.70) b |
41.0 (0.61) ab |
Palmitoleic acid (Z C16:1n9) |
0.58 (0.09) a |
0.35 (0.17) a |
— |
— |
Margaric acid (C17) |
0.04 (0.04) |
— |
— |
— |
Stearic acid (C18) |
18.6 (0.50) a |
20.6 (0.69) ab |
21.9 (0.58) bc |
22.9 (1.21) c |
Oleic acid (Z C18:1n9) |
12.3 (0.29) c |
6.40 (0.24) b |
5.60 (0.24) ab |
5.38 (0.17) a |
Linoleic acid (Z C18:2n6) |
3.85 (0.14) a |
8.91 (0.34) b |
10.0 (0.51) b |
12.0 (0.31) c |
Linolenic acid (C18:3n3) |
— |
1.14 (0.07) a |
1.06 (0.09) a |
1.80 (0.14) a |
Arachidic (C20) |
0.04 (0.04) |
— |
— |
— |
cis-11.14-Eicosadienoic acid (C20:2) |
0.70 (0.18) a |
0.46 (0.25) a |
0.37 (0.15) a |
0.12 (0.12) a |
Heneicosanoic acid (C21) |
0.18 (0.12) |
— |
— |
— |
cis-8.11.14-Eicosatrienoic acid (C20:3n6) DGLA |
0.25 (0.08) |
— |
— |
— |
Arachidonic acid (C20:4n6) AA |
15.5 (0.94) b |
8.73 (0.54) a |
8.47 (0.27) a |
8.41 (0.65) a |
Behenic acid (C22) |
0.32 (0.09) |
— |
— |
— |
Eicosapentaenoic acid (C20:5n3) EPA |
— |
3.71 (0.21) a |
3.73 (0.24) a |
2.75 (0.15) a |
Lignoceric acid (C24) |
1.07 (0.17) a |
2.29 (0.10) b |
2.18 (0.25) b |
2.91 (0.22) b |
Nervonic acid (C24:1) |
1.83 (0.09) |
— |
— |
— |
Docosahexaenoic acid (C22:6n3) DHA |
1.00 (0.09) a |
1.27 (0.19) a |
0.81 (0.07) a |
1.24 (0.30) a |
C18:1n7 acid (W7) |
2.37 (0.10) b |
1.77 (0.08) ab |
1.49 (0.05) a |
1.29 (0.07) a |
The saturated (SFA), monounsaturated (MUFA), and polyunsaturated fatty acid (PUFA) indices of the plasma, liver, and erythrocyte membrane are presented in Fig. 3. In the three compartments, the intake of BSO produced changes in the percentage of MUFA which significantly decreased, whereas the percentage of PUFA increased. Furthermore, a slight increase in the percentage of SFA was found in the erythrocyte membrane of animals fed the BSO diets.
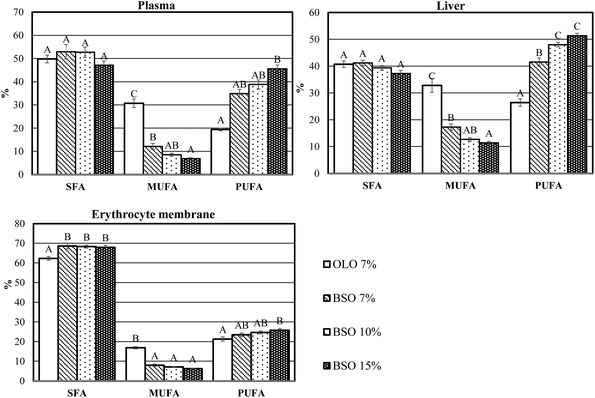 |
| Fig. 3 Saturated (SFA), monounsaturated (MUFA), and polyunsaturated fatty acid (PUFA) indices of the plasma, liver, and erythrocyte membrane. OLO: olive oil used as dietary fat; BSO: basil seed oil used as dietary fat. Results are means of 8 rats. A–C mean within the same index with different letters indicating significant difference (ANOVA treatment, p < 0.05). | |
3.7. Hepatic fatty acid indices and Pearson correlations
The influence of dietary BSO consumption on hepatic fatty acid indices is presented in Table 7. Both desaturase–elongase (ARA/LA) and Scd-1 activities (OLE/STE) were significantly decreased by BSO regardless of the oil inclusion level. The 5-elongase activity (ARA/EI) was also significantly lower when BSO was added to the diet compared to the activity in animals that consumed OLO, and this reduction was analogous to the increase in BSO inclusion in the diet.
Table 7 Influence of the type and dietary inclusion of basil oil on hepatic fatty acid indices
|
ARA/LA |
DHA/ALA |
PE/PI |
OLE/STE |
ARA/EI |
ARA/LA, arachidonic/linoleic acid (desaturase–elongase), DHA/ALA, docosahexaenoic/linolenic acid (desaturase–elongase), PE/PI, palmitoleic/palmitic acid (Scd-1 activity), OLE/STE, oleic/stearic acid (Scd-1 activity), ARA/EI, arachidonic/eicosatrienoic acid (5-elongase). Results are means of 8 rats. a–c mean within the same column with different letters indicating significant difference (P < 0.05). |
OLO 7% |
3.15 (0.28) a |
— |
0.10 (0.00) a |
1.74 (0.33) a |
36.9 (1.48) a |
BSO 7% |
0.35 (0.04) b |
0.21 (0.05) a |
0.11 (0.01) a |
0.82 (0.11) b |
21.2 (0.37) b |
BSO 10% |
0.31 (0.02) b |
0.18 (0.02) a |
0.06 (0.01) b |
0.59 (0.06) b |
15.9 (1.61) c |
BSO 15% |
0.25 (0.01) b |
0.10 (0.03) a |
0.04 (0.00) b |
0.59 (0.06) b |
12.3 (0.47) c |
Regression analysis (upon significant Pearson correlation coefficient) between basil oil percentages included in the experimental diets and the fatty acid profile on each compartment (plasma, liver, and erythrocytes) is presented in Fig. 4. Negative correlations were found between the increasing basil oil inclusion percentages and oleic acid as well as arachidonic acid, whereas positive correlations were found for linoleic, linolenic, and eicosapentaenoic acids in all the compartments studied. Changes in the percentage of fatty acid in response to the BSO inclusion level were fitted to a linear regression model that was satisfied by the equation y = a + b [% BSO inclusion], where y = percentage of fatty acid in the specific compartment studied. As for the regression slopes, they were always greater in the plasma and liver compared to erythrocytes. On the other hand, the fatty acids showing greater net slope values were oleic, linoleic and linolenic acids, followed by arachidonic and eicosapentaenoic acids.
 |
| Fig. 4 Linear regression model estimated between basil oil percentages included in the experimental diets and the fatty acid profile on each compartment: plasma, liver, and erythrocyte membrane. The linear regression model was performed when the Pearson correlation test result was statistically significant (p < 0.01). | |
4. Discussion
Ocimum basilicum, basil, is a common aromatic herb with interesting functional properties and is used in countless countries to prepare different dishes. The oil extracted from the seed has attractive characteristics because of its fatty acid profile and is mostly composed of LA and ALA. However, to our knowledge, no studies have focused on its in vivo bioavailability and biotransformation. This study aimed to assess the bioavailability of BSO used as a fat source, as well as the biotransformation of LA and ALA in growing rats compared to a well-known and standardized fat source such as olive oil. Our results showed a high digestive utilization of basil seed oil and the absence of toxic effects associated with its consumption as well as null interference in the digestive and nutritive utilization of dietary protein. In addition, the presence of ALA from BSO was detected in the plasma, liver, and erythrocyte membrane together with its biotransformation product eicosapentaenoic acid (EPA).
The fatty acid profile of BSO showed a high concentration of PUFAs like ALA (71.7%) and LA (14.8%). Some authors have shown how the levels of these fatty acids are influenced by different factors like the basil population and geographical characteristics of the area where the plant is cultivated.3 Nevertheless, the levels of LA and ALA usually range between 16–25% and 42–62%, respectively.3,31 Under our experimental conditions, the levels of LA were lower than the results reported by other authors, whereas the ALA percentage obtained was higher. Regarding the content of fat-soluble components, the most abundant tocopherol in BSO was γ-tocopherol, exceeding its concentration in olive oil by almost 500 times. γ-Tocopherol has been described as the most abundant tocopherol in other seed oils such as linseed or soybean oil,32 and protects cells from damage associated with inflammation as evidenced by its enhanced presence during the inflammatory response.33 On the other hand, the presence of phytosterols like campesterol, stigmasterol and β-sitosterol has been detected in both basil seed and olive oils. BSO showed a low concentration in β-sitosterol compared to both olive oil and other oils rich in polyunsaturated fatty acids,32 whereas campesterol and stigmasterol levels were higher in BSO. The efficacy of phytosterols such as stigmasterol and β-sitosterol, in the treatment of NAFLD by reducing hepatic cholesterol has been reported.34
To test the true bioavailability of PUFAs from basil seed oil, we carried out an in vivo experiment using BSO as the sole source of fat. Growing rats were fed diets with increasing concentrations of BSO (7, 10, and 15%) to assess potential adverse effects and test possible linear effects of increasing PUFA concentration on their bioaccessibility and biotransformation, whereas a group of animals fed a diet with 7% olive oil (OLO) served as the control, both of dietary fat content and fatty acid profile. It is important to highlight that during all the experimental period the animals did not exhibit any appreciable harm caused by consuming BSO. The data obtained on the food intake only showed a decrease concerning a high-fat dietary level (BSO 15%), thus maintaining the energy intake at the same levels of the rest of the experimental groups. This shows the capacity of the animals to balance the energy intake and energy expenditure, a biological aspect widely studied in diet-induced obesity experimental models.35 This situation was reflected in the bodyweight of the animals, which was similar among all groups throughout the experimental period, thus indicating no negative effects of BSO consumption on this parameter. All this resulted in an adequate protein efficiency ratio (PER). Furthermore, no interaction was found between BSO intake and dietary protein bioavailability, a finding that argues in favor of the possible incorporation of BSO to the usual diet as a commonly used ingredient.
Regarding hematological parameters, BSO did not cause any significant effect except for a lower platelet count found in the BSO 15% group. Our results agree with those of Yamada et al.36 and Takeuchi et al.37 who reported that the platelet count in rats decreased as a result of EPA and DHA supplementation. Lower levels of platelets could in turn lead to a lower risk of platelet aggregation and thrombosis. On the other hand, the lack of substantial effects in other hematological parameters is usually considered a positive sign of low or null toxic action.38
The main effect of ALA intake on plasma biomarkers of cardiovascular risk focuses on its potential to lower LDL-cholesterol levels.39 Under our experimental conditions, this effect was not noticed since olive oil used as a control fat source is well known to exhibit beneficial properties on the cardiovascular system, decreasing total-, HDL-, and LDL-cholesterol levels as well as their oxidation due to its high polyphenol content, thus reducing the risk of atherosclerosis.40 In addition, an overall absence of liver damage caused by BSO intake could be inferred from the low plasma activity values of transaminases. The slight increase in ALT and the 2.2-fold increment in ALP levels for the BSO 15% group could be attributed to the increase in the dietary fat levels24,41,42 and could indicate a potential adverse effect of the higher oil dose assayed in this study, as has been reported for studies involving different dietary oils43 but not for the dose within the range of values that meet the nutrient requirements of the experimental animal used.25
Concerning the fatty acid profile in the plasma, liver, and erythrocyte membrane, it is important to remark the indispensable character of LA and ALA, given that animals cannot convert oleic acid to the former fatty acids that must be acquired from the diet. In the endoplasmic reticulum of liver cells, LA and ALA are transformed in their respective long-chain polyunsaturated fatty acids (LC-PUFA), arachidonic acid (ARA) and eicosapentaenoic (EPA) acid, after two desaturation and two-carbon elongation processes.20 In our study, we observed that the presence of high dietary proportions of LA present in BSO vs. OLO decreased the percentage of ARA in all the target organs and tissues studied. The efficiency of bio-transformation in fatty acid metabolism can be determined using different hepatic fatty acid indices.44 The efficacy of these indices to predict specific aspects of lipid metabolism has been confirmed by several studies that have investigated the use of surrogate plasma fatty acid analysis to provide further insights into the underlying adiposity and development of the metabolic syndrome,45 the lipid ratios representing SCD1, FADS1, and FADS2 activities as candidate biomarkers of early growth and adiposity,46 the use of a panel of free fatty acid ratios to predict the development of metabolic abnormalities in healthy obese individuals,47 or the increased stearoyl-CoA desaturase activity indices in the rat liver to assess omega-3 fatty acid deficiency.48 Our results show a significant decrease of the indices of Scd-1 activity derived from ALA supplementation that are in agreement with those of the above-mentioned authors who pointed out increased liver indices of the former enzyme as a result of omega-3 deficiency. On the other hand, the remarkable decrease in desaturase–elongase (ARA/LA) and Δ-5 elongase (ARA/EI) indices when the animals were fed a BSO diet could be mainly because the affinity of the Δ6 desaturase enzyme (responsible for the first transformation step from LA to ARA) for ALA is higher than that for LA. Thus, if ALA supply is higher than LA supply, the formation of LA derivatives of higher unsaturation (ARA) is hindered in favor of the transformation of ALA into EPA and DHA.49 A negative correlation between the dietary inclusion levels of BSO and the hepatic content of ARA was found. This shunting of desaturase enzyme activity towards EPA formation derived from BSO inclusion in the diet could be an effective strategy to decrease the synthesis of pro-inflammatory molecules as eicosanoids.50,51 Furthermore, EPA has been described to exhibit an inhibitory effect against the production of pro-inflammatory cytokines such as IL-1, 6, or 8, as well as TNFα.52 In addition, it is related to the synthesis of intermediates with known vasodilation and anti-aggregation action. All this can result in significantly improved cardiovascular health.53,54
Although the dietary inclusion levels of BSO showed a positive correlation with hepatic EPA levels, the higher inclusion levels of BSO were not reflected in further increments of EPA and DHA on the plasma, erythrocyte membrane, or liver once a threshold level of 4% was reached in the former tissues. Tu et al.55,56 described that the relationship between dietary ALA and hepatic EPA is linear and dose-dependent when the inclusion level of ALA in the diet ranges from 0.2 to 2.9%. However, the relationship was not linear between dietary ALA and plasma or erythrocyte EPA. Our experimental conditions involved an inclusion level of dietary ALA from 6 to 12% showing a positive correlation with hepatic EPA, although the constant levels of EPA suggest a negative feedback of the former on Δ-6 desaturase, which could in turn act as a limiting step to increase EPA and DHA formation. Moreover, the inhibiting effects of EPA and DHA on the activity and expression of Δ-5 and Δ-6 desaturases have been reported.57 On the other hand, Gibson et al.58 have reported that diets containing ALA above an optimal level result in no further increase in DHA levels in animals and humans. The authors reported that the peak of the plasma phospholipid DHA was obtained supplementing a narrow range of 1–3% of energy for ALA and 1–2% of energy in LA, while higher amounts of dietary fatty acids supplemented suppressed the observed peak to basal levels.
Increasing levels of ALA intake did not seem to influence the amount of DHA that was maintained within the range of 2% in the liver and 1% in the plasma and erythrocyte membrane in the animals consuming both BSO and OLO diets. Studies reporting the effects of ALA intake on DHA levels are not conclusive due to disparity of results,59 and a higher ALA intake did not always translate into a higher DHA content, as observed in our study. Indeed, we did not find any correlation between these two parameters, possibly due to the saturation effect on the Δ-6 desaturase enzyme produced by the high percentage of dietary ALA. Furthermore, the hepatic fatty acid index of DHA/ALA (desaturase–elongase activity) was stable in the three groups consuming different percentages of BSO.
Another controversial factor involved in the conversion of DHA from ALA is the LA
:
ALA ratio. Optimal metabolism benefits are found when dietary LA is decreased and ALA is increased to 4–1
:
1, although some authors have found that the relationship between ALA and hepatic DHA is not linear.56,60 Greupner et al.61 reported that DHA levels in the blood are independent of this ratio, since the use of different LA
:
ALA ratios (0.5
:
1 vs. 20–30
:
1) did not affect the DHA levels in blood. Although in the present study the ratio LA
:
ALA from BSO (1
:
3) did not influence plasma, liver, and erythrocyte membrane DHA levels, this ratio is optimal for enhancing proliferation and differentiation of embryonic neural stem cells. Therefore, BSO could be a therapeutic intervention in neurological disorders.62 However, it appears that the best way to increase DHA levels in the organism is through the dietary intake of DHA from fish or other sources,20 although the use of vegetable oils with high ALA content has beneficial effects such as the reduction of precursors of inflammatory substances.
Recent research has focused on new sources of oil containing high ALA levels. In this regard, several vegetable oils with different ALA levels such as rosa mosqueta, sacha inchi, chia, perilla, and linseed oils among others, have been studied. The dietary inclusion of 10% rosa mosqueta, chia, and sacha inchi oils (ALA levels of 33, 48, and 63%, respectively) resulted in the appearance of ALA and EPA in the liver,63 in a similar way to the results of the present study when BSO was included as the source of fat in the diet. Furthermore, the administration of 5 g kg−1 perilla oil for 21 days increased ALA and EPA levels in the liver.64 In contrast, Rincón-Cervera et al.63 reported an increase in DHA levels after supplementing with rosa mosqueta, chia, and sacha inchi oils. This difference could be attributed to the higher ALA content in BSO or the different LA
:
ALA ratios. As for linseed oil, Murru et al.65 observed that its dietary inclusion at 4% for 10 weeks produced an increase in ALA and EPA levels in the plasma and liver of Wistar rats, but not in DHA.
The potential use of basil seed oil as a dietary supplement to enhance the circulating and storage levels of n-3 fatty acids in humans must be oriented in the context of the requirement and recommended dietary allowances for these nutrients. According to Hibbeln et al.66 a healthy dietary allowance for n-3 long chain fatty acids ranges from 750 mg to 3.5 g d−1 depending on the country and can be affected by the intake of n-6 fatty acids, with the possibility of a marked reduction in n-3 allowance if consumption of n-6 fats is reduced. In this regard, the high percentage of ALA content in BSO and its significant digestibility and incorporation of fatty acid to different tissues where it can be efficiently converted to EPA can give the oil added value as a dietary supplement.
The effectiveness of specific dietary fat sources in changing the fatty acid profiles of different tissues after administration for relatively short periods may be dependent on the fat's specific fatty acid composition and bioaccessibility in the digestive tract. This, in turn, may affect not only the nutritional properties of each fat but also its health-related effects. In this regard, the linear equations that relate the different oil inclusion levels to changes in the relative content of individual fatty acids in the plasma, erythrocyte membrane, and liver can be a useful predictive tool to assess and compare the bioavailability and biotransformation of different dietary fats. Under the experimental conditions of the present study, the incorporation and biotransformation of fatty acids took place at a higher rate in the plasma and liver compared to the erythrocyte membrane, whereas oleic, linoleic, and linolenic acids were those which exhibited a greater degree of change resulting from the dietary inclusion of BSO.
5. Conclusions
Basil seed oil is a good source of ALA and its bioavailability determined by the apparent digestibility coefficient was 95%, comparable to that of olive oil. Furthermore, BSO did not interfere with protein digestibility, food intake, or animal weight, and did not produce any major alteration in the parameters studied or at any of the concentrations included in the animals’ diets. ALA from BSO was assimilated, accumulated, and transformed into EPA found in the liver, plasma, and erythrocyte membrane of the animals although the DHA levels remained constant and unaffected by BSO intake. Basil seed oil could be used not only as a nutrient but also as a functional ingredient capable of increasing EPA formation from ALA and decreasing the pro-inflammatory state found in some pathologies such as the metabolic syndrome, cancer or some neurological diseases, via lowering ARA levels. Finally, the high ALA content of BSO can make it a useful ingredient in nutraceutical formulations aimed to efficiently adjust the dietary level of the former fatty acid.
5.1 Strengths and limitations
The main strength of this article is that it shows in an in vivo model the nutritional potential and lack of adverse effects of a novel source of highly available omega-3 fatty acids, and the biotransformation to omega-3 derivatives like EPA that exhibit interesting anti-inflammatory, vasodilation, and anti-thrombotic activities. In contrast, the study has some limitations such as the imbalance of LA and ALA in olive oil. Nevertheless, the former oil has been widely used in numerous nutritional studies in control diets due to its cardiovascular benefits. Another limitation is the protein content of the 15% BSO diet that was not increased by 10–15% to compensate for the reduced food intake derived from the adaptation process of the animals to the higher energy density of the experimental diet. The entire range of lipid components in the olive oil has been thoroughly studied by numerous research groups all over the world and, particularly in areas where olive oil is the basic fat in the diet. For this reason, the fatty acid profile of olive oil used in this study was assessed by means of gas chromatography using a FAME 105 mix, 37 component, TraceCERT standard, whereas the rest of the lipid components were completed with bibliographic data from relevant researchers in the field.
7% olive oil was used as a control following the recommendations to meet the nutritional requirements of the laboratory rat. It was also considered that due to the low levels of omega-3 fatty acids present in olive oil, this would be a good reference to compare against it the effects of different omega-3 fatty acids provided by the three BSO doses assayed. This is the reason why we did not consider applying the same doses to three olive oil control groups.
Author contributions
Jesús M. Porres, Rosario Martínez, María López-Jurado and Francisco Bermúdez: conceptualization; Rosario Martínez, Cristina Mesas, Ana Guzmán, Milagros Galisteo and Jesús M. Porres: investigation; Francisco Bermúdez, Jesús M. Porres, Consolación Melguizo, and José Carlos Prados: resources; Rosario Martínez: formal analysis; Rosario Martínez and Jesús M. Porres: writing – original draft; and María López-Jurado, Jesús M. Porres, Consolación Melguizo, José Carlos Prados and Francisco Bermúdez: supervision, writing – review & editing, and funding acquisition.
Conflicts of interest
The authors declare no conflict of interest.
Acknowledgements
The authors want to thank Susana Ibáñez from the Analytical Unit of Scientific Instrumentation Centre (CIC, UGR) for its excellent technical assistance and Antonio Murillo Cancho from the University of Almería and CELLBITEC S.L. This research was funded by the Spanish Ministry of Science, Innovation, and Universities as well as the European Union through projects PTQ-17-09172, RTC-2017-6540-1, RTI2018-100934-B-I00, and RTC2019-006870-1, and the FEDER program. In addition, this work was supported by funds from research groups AGR145, CTS164, and CTS-107 (Andalusian Government).
References
- M. Moghaddam and L. Mehdizadeh, Variability of total phenolic, flavonoid and rosmarinic acid content among Iranian basil accessions, LWT – Food Sci. Technol., 2015, 63, 535–540 CrossRef CAS
.
- S. Naji-Tabasi and S. M. A. Razavi, Functional properties and applications of basil seed gum: An overview, Food Hydrocolloids, 2017, 73, 313–325 CrossRef CAS
.
- S. Mostafavi, H. A. Asadi-Gharneh and M. Miransari, The phytochemical variability of fatty acids in basil seeds (Ocimum basilicum L.) affected by genotype and geographical differences, Food Chem., 2019, 276, 700–706 CrossRef CAS PubMed
.
- G. O. Burr and M. M. Burr, A New Deficiency Disease Produced by the Rigid Exclusion of Fat from the Diet, J. Biol. Chem., 1929, 82, 345–367 CrossRef CAS
.
- A. J. Sinclair, N. M. Attar-Bashi and D. Li, What is the role of α-linolenic acid for mammals?, Lipids, 2002, 37, 1113–1123 CrossRef CAS PubMed
.
- J. Whelan and K. Fritsche, Linoleic Acid, Adv. Nutr., 2013, 4, 311–312 CrossRef PubMed
.
- S. E. Desale and S. Chinnathambi, α-Linolenic acid modulates phagocytosis and endosomal pathways of extracellular Tau in microglía, Cell Adhes. Migr., 2021, 15, 84–100 CrossRef CAS PubMed
.
- N. Blondeau, R. H. Lipsky, M. Bourourou, M. W. Duncan, P. B. Gorelick and A. M. Marini, Alpha-linolenic acid: an omega-3 fatty acid with neuroprotective properties—ready for use in the stroke clinic, BioMed. Res. Int., 2015, 2015, 519830 Search PubMed
.
- F. Chen, Y. Wang, H. Wang, Z. Dong, Y. Wang, M. Zhang, J. Li, S. Shao, C. Yu, Z. Huan and J. Xu, Flaxseed oil ameliorated high-fat-diet-induced bone loss in rats by promoting osteoblastic function in rat primary osteoblasts, Nutr. Metab., 2019, 16, 71 CrossRef PubMed
.
- G. Li, X. Wang, H. Yang, P. Zhang, F. Wu, Y. Li, Y. Zhou, X. Zhang, H. Ma, W. Zhang and J. Li, α-Linolenic acid but not linolenic acid protects against hypertension: critical role of SIRT3 and autophagic flux, Cell Death Dis., 2020, 11, 1–13 CrossRef PubMed
.
- F. Qian, A. V. Ardisson Korat, F. Imamura, M. Marklund, N. Tintle, J. K. Virtanen, X. Zhou, J. K. Bassett, H. Lai, Y. Hirakawa, K.-L. Chien, A. C. Wood, M. Lankinen, R. A. Murphy, C. Samieri, K. Pertiwi, V. D. de Mello, W. Guan, N. G. Forouhi, N. Wareham, I. C. F. B. Hu, U. Riserus, L. Lind, W. S. Harris, A. H. Shadyab, J. G. Robinson, L. M. Steffen, A. Hodge, G. G. Giles, T. Ninomiya, M. Uusitupa, J. Tuomilehto, J. Lindström, M. Laakso, D. S. Siscovick, C. Helmer, J. M. Geleijnse, J. H. Y. Wu, A. Fretts, R. N. Lemaitre, R. Micha, D. Mozaffarian and Q. Sun, n-3 fatty acid biomarkers and incident type 2 diabetes: an individual participant-level pooling project of 20 prospective cohort studies, Diabetes Care, 2021, 44, 1133–1142 CrossRef CAS PubMed
.
- P. C. Calder, Omega-3 fatty acids and inflammatory processes: from molecules to man, Biochem. Soc. Trans., 2017, 45, 1105–1115 CrossRef CAS PubMed
.
- T. Nagatake, Y. Shibata, S. Morimoto, E. Node, K. Sawane, S.-I. Hirata, J. Adachi, Y. Abe, J. Isoyama, A. Saika, K. Hosomi, T. Tomonaga and J. Kunisawa, 12-Hydroxyeicosapentaenoic acid inhibits foam cell formation and ameliorates high-fat diet-induced pathology of atherosclerosis in mice, Sci. Rep., 2021, 11, 10426 CrossRef CAS PubMed
.
- H.-X. Zhang, Y.-H. Tian, J. Guan, Q.-M. Xie and Y.-Q. Zhao, The anti-tussive, anti-inflammatory effects and sub-chronic toxicological evaluation of perilla seed oil, J. Sci. Food Agric., 2021, 101, 1419–1427 CrossRef CAS PubMed
.
- R. K. Yadav, M. Singh, S. Roy, S. Gautam, J. K. Rawat, L. Singh, M. N. Ansari, A. S. Saeedan and G. Kaithwas, Short communication: evaluation of α-linolenic acid-based intramammary nanosuspension for treatment of subclinical mastitis, J. Dairy Sci., 2020, 103, 2701–2706 CrossRef CAS PubMed
.
- C. N. Serhan, N. Chiang, J. Dalli and B. D. Levy, Lipid mediators in the resolution of inflammation, Cold Spring Harbor Perspect. Biol., 2015, 7, a016311 CrossRef PubMed
.
- S. Ghasemi Fard, D. Cameron-Smith and A. J. Sinclair, n
-
3 Docosapentaenoic acid: the iceberg n
-
3 fatty acid, Curr. Opin. Clin. Nutr. Metab. Care, 2021, 24, 134–138 CrossRef CAS PubMed
.
- A. P. Simopoulos, Genetic variation, diet, inflammation, and the risk for COVID-19, Lifestyle Genomics, 2021, 14, 37–42 CrossRef CAS PubMed
.
- A. P. Simopoulos, The importance of the omega-6/omega-3 fatty acid ratio in cardiovascular disease and other chronic diseases, Exp. Biol. Med., 2008, 233, 674–688 CrossRef CAS PubMed
.
- R. K. Saini and Y.-S. Keum, Omega-3 and omega-6 polyunsaturated fatty acids: Dietary sources, metabolism, and significance-A review, Life Sci., 2018, 203, 255–267 CrossRef CAS PubMed
.
- H. O. Santos, J. C. Price and A. A. Bueno, Beyond fish oil supplementation: The effects of alternative plant sources of omega-3 polyunsaturated fatty acids upon lipid indexes and cardiometabolic biomarkers-An overview, Nutrients, 2020, 12, 3159 CrossRef CAS PubMed
.
- J. Wang, Y. He, D. Yu, L. Jin, X. Gong and B. Zhang, Perilla oil regulates intestinal microbiota and alleviates insulin resistance through the PI3 K/AKT signaling pathway in type-2 diabetic KKAy mice, Food Chem. Toxicol., 2020, 135, 110965 CrossRef CAS PubMed
.
- G. Lepage and C. C. Roy, Direct transesterification of all classes of lipids in a one-step reaction, J. Lipid Res., 1986, 27(1), 114–120 CrossRef CAS
.
- R. Martínez, L. M. López-Trinidad, G. Kapravelou, F. Arrebola, M. Galisteo, P. Aranda, J. M. Porres and M. López-Jurado, A combined healthy strategy for successful weight loss, weight maintenance and improvement of hepatic lipid metabolism, J. Nutr. Biochem., 2020, 85, 108456 CrossRef PubMed
.
- P. G. Reeves, F. H. Nielsen and G. C. Fahey Jr., AIN-93 purified diets for laboratory rodents: final report of the American Institute of Nutrition ad hoc writing committee on the reformulation of the AIN-76A rodent diet, J. Nutr., 1993, 123, 1939–1951 CrossRef CAS PubMed
.
- Directive 2010/63/EU of the European Parliament and of the Council of 22 September 2010 on the protection of animals used for scientific purposes, Off. J. Eur. Union, 2010, L276, 33–79 Search PubMed
.
- R. C. Hubrecht and E. Carter, The 3Rs and humane experimental technique: implementing change, Animals, 2019, 9(10), 754 CrossRef PubMed
.
- G. Kapravelou, R. Martínez, J. Martino, J. M. Porres and I. Fernández-Fígares, Natural fermentation of cowpea (Vigna unguiculata) flour improves the nutritive utilization of indispensable amino acids and phosphorus by growing rats, Nutrients, 2020, 12, 2186 CrossRef CAS PubMed
.
- L. González-Torres, C. Matos, M. Vázquez-Velasco, J. A. Santos-López, I. Sánchez-Martínez, C. García-Fernández, S. Bastida, J. Benedí and F. J. Sánchez-Muniz, Glucomannan- and glucomannan plus spirulina-enriched pork affect liver fatty acid profile, LDL receptor expression and antioxidant status in Zucker fa/fa rats fed atherogenic diets, Food Nutr. Res., 2016, 61, 1264710 CrossRef PubMed
.
- J. Folch, M. Lees and G. H. Sloane Stanley, A simple method for the isolation and purification of total lipides from animal tissues, J. Biol. Chem., 1957, 226, 497–509 CrossRef CAS PubMed
.
- P. Angers, M. R. Morales and J. E. Simon, Fatty acid variation in seed oil among Ocimum species, J. Am. Oil Chem. Soc., 1996, 73, 393–395 CrossRef CAS
.
- S. Liu, H. Hu, Y. Yu, J. Zhao, L. Liu, S. Zhao, J. Xie, C. Li and M. Shen, Simultaneous determination of tocopherols, phytosterols, and squalene in vegetable oils by high performance liquid chromatography-tandem mass spectrometry, Food Anal. Methods, 2021, 14, 1567–1576 CrossRef
.
- M. D. Thompson and R. V. Cooney, The potential physiological role of γ-tocopherol in human health: a qualitative review, Nutr. Cancer, 2020, 72(5), 808–825 CrossRef CAS PubMed
.
- S. Feng, L. Gan, C. S. Yang, A. B. Liu, W. Lu, P. Shao, Z. Dai, P. Sun and Z. Luo, Effects of stigmasterol and β-sitosterol on nonalcoholic fatty liver disease in a mouse model: a lipidomic analysis, J. Agric. Food Chem., 2018, 66(13), 3417–3425 CrossRef CAS PubMed
.
- M. So, M. P. Gaidhu, B. Maghdoori and R. B. Ceddia, Analysis of time-dependent adaptations in whole-body energy balance in obesity induced by high-fat diet in rats, Lipids Health Dis., 2011, 10, 99 CrossRef PubMed
.
- N. Yamada, Y. Kobatake, S. Ikegami, T. Takita, M. Wada, J. Shimizu, Y. Kanke and S. Innami, Changes in blood coagulation, platelet aggregation, and lipid metabolism in rats given lipids containing docosahexaenoic acid, Biosci. Biotechnol. Biochem., 1997, 61(9), 1454–1458 CrossRef CAS PubMed
.
- H. Takeuchi, T. Nagaosa and K. Muramatsu, Effects of a dietary supplementation of n-3 eicosapentaenoic acid on the platelets and spleen in rats, Agric. Biol. Chem., 1990, 54(9), 2437–2440 CAS
.
- X. Zhang, X. Xu, S. Li, L.-H. Wang, J. Zhang and R. Wang, A systematic evaluation of the biocompatibility of cucurbit[7]uril in mice, Sci. Rep., 2018, 8, 8819 CrossRef PubMed
.
- Y. Kawakami, H. Yamanaka-Okumura, Y. Naniwa-Kuroki, M. Sakuma, Y. Taketani and E. Takeda, Flaxseed oil intake reduces serum small dense low-density lipoprotein concentrations in Japanese men: a randomized, double blind, crossover study, Nutr. J., 2015, 14, 39 CrossRef PubMed
.
- H. Berrougui, S. Ikhlef and A. Khalil, Extra virgin olive oil polyphenols promote cholesterol efflux and improve HDL functionality, J. Evidence-Based Complementary Altern. Med., 2015, 2015, 208062 Search PubMed
.
- L. Álvarez-Amor, A. L. Sierra, A. Cárdenas, L. López-Bermudo, J. López-Beas, E. Andújar, M. Pérez-Alegre, R. Gallego-Durán, L. M. Varela, A. Martin-Montalvo, G. Berná, A. Rojas, M. J. Robles-Frías, A. Hmadcha, M. Romero-Gómez, R. Kleemann and F. Martín, Extra virgin olive oil improved body weight and insulin sensitivity in high fat diet-induced obese LDLr−/−Leiden mice without attenuation of steatohepatitis, Sci. Rep., 2021, 11, 8250 CrossRef PubMed
.
- A. Amirinejad, A. Hekmatdoost, A. Ebrahimi, F. Ranjbaran and F. Shidfar, The effects of hydroalcoholic extract of spinach on prevention and treatment of some metabolic and histologic features in a rat model of nonalcoholic fatty liver disease, J. Sci. Food Agric., 2020, 100, 1787–1796 CrossRef CAS PubMed
.
- J. Ritskes-Hoitinga, P. M. Verschuren, G. W. Meijer, A. Wiersma, A. J. van de Kooij, W. G. Timmer, C. G. Blonk and J. A. Weststrate, The association of increasing dietary concentrations of fish oil with hepatotoxic effects and a higher degree of aorta atherosclerosis in the ad lib-fed rabbit, Food Chem. Toxicol., 1998, 36(8), 663–672 CrossRef CAS PubMed
.
- R. Martínez, G. Kapravelou, A. Donaire, C. Lopez-Chaves, F. Arrebola, M. Galisteo, S. Cantarero, P. Aranda, J. M. Porres and M. López-Jurado, Effects of a combined intervention with a lentil protein hydrolysate and a mixed training protocol on the lipid metabolism and hepatic markers of NAFLD in Zucker rats, Food Funct., 2018, 9, 830–850 RSC
.
- L. M. Jones and M. Legge, Plasma fatty acids as markers for desaturase and elongase activities in spinal cord injured males, J. Spinal Cord Med., 2019, 42(2), 163–170 CrossRef PubMed
.
- L. Olga, J. A. van Diepen, I. Bobeldijk-Pastorova, G. Gross, P. M. Prentice, S. G. Snowden, S. Furs, T. Kooistra, I. A. Hughes, M. H. Schoemaker, E. A. F. van Tol, W. van Duyvenvoorde, P. Y. Wielinga, K. K. Ong, D. B. Dunger, R. Kleemann and A. Koulman, Lipid ratios representing SCD1, FADS1, and FADS2 activities as candidate biomarkers of early growth and adiposity, EBioMedicine, 2021, 63, 103198 CrossRef CAS PubMed
.
- L. Zhao, Y. Ni, X. Ma, A. Zhao, Y. Bao, J. Liu, T. Chen, G. Xie, J. Panee, M. Su, H. Yu, C. Wang, C. Hu and W. Jia, A panel of free fatty acid ratios to predict the development of metabolic abnormalities in healthy obese individuals, Sci. Rep., 2016, 6, 28418 CrossRef CAS PubMed
.
- R. Hofacer, I. J. Magrisso, R. Jandacek, T. Rider, P. Tso, S. C. Benoit and R. K. McNamara, Omega-3 fatty acid deficiency increases stearoyl-CoA desaturase expression and activity indices in rat liver: positive association with non-fasting plasma triglyceride levels, Prostaglandins, Leukotrienes Essent. Fatty Acids, 2012, 86(1–2), 71–77 CrossRef CAS PubMed
.
- A. Valenzuela and R. U. D. Imbarak,
in Tratado de Nutrición Search PubMed
, Funciones y metabolismo de los ácidos grasos esenciales y de sus derivados activos in Tratado de nutrición, (Bases fisiológicas y bioquímicas de la nutrición/Ángel Gil, Luis Fontana Gallego, Fermín Sánchez de Medina Contreras, coord), Editorial Médica Panamericana, 2017, vol. 1, pp. 155–171, ISBN: 978-84-9830123-9.
- A. Czumaj and T. Śledziński, Biological role of unsaturated fatty acid desaturases in health and disease, Nutrients, 2020, 12, 356 CrossRef CAS PubMed
.
- J. K. Innes and P. C. Calder, Omega-6 fatty acids and inflammation, Prostaglandins, Leukotrienes Essent. Fatty Acids, 2018, 132, 41–48 CrossRef CAS PubMed
.
- P. C. Calder, Omega-3 fatty acids and inflammatory processes, Nutrients, 2010, 2(3), 355–374 CrossRef CAS PubMed
.
- R. Limbu, G. S. Cottrell and A. J. McNeish, Characterisation of the vasodilation effects of DHA and EPA, n-3 PUFAs (fish oils), in rat aorta and mesenteric resistance arteries, PLoS One, 2018, 13(2), e0192484 CrossRef PubMed
.
- J. K. Innes and P. C. Calder, Marine omega-3 (n-3) fatty acids for cardiovascular health: an update for 2020, Int. J. Mol. Sci., 2020, 21(4), 1362 CrossRef CAS PubMed
.
- W. C. Tu, R. J. Cook-Johnson, M. J. James, B. S. Mühlhäusler and R. A. Gibson, Omega-3 long chain fatty acid synthesis is regulated more by substrate levels than gene expression, Prostaglandins, Leukotrienes Essent. Fatty Acids, 2010, 83, 61–68 CrossRef CAS PubMed
.
- W. C. Tu, B. S. Mühlhäusler, L. N. Yelland and R. A. Gibson, Correlations between blood and tissue omega-3 LCPUFA status following dietary ALA intervention in rats, Prostaglandins, Leukotrienes Essent. Fatty Acids, 2013, 88, 53–60 CrossRef CAS PubMed
.
- F. Tosi, F. Sartori, P. Guarini, O. Olivieri and N. Martinelli, Delta-5 and delta-6 desaturases: crucial enzymes in polyunsaturated fatty acid-related pathways with pleiotropic influences in health and disease, Adv. Exp. Med. Biol., 2014, 824, 61–81 CrossRef CAS PubMed
.
- R. A. Gibson, M. A. Neumann, E. L. Lien, K. A. Boyd and W. C. Tu, Docosahexaenoic acid synthesis from alpha-linolenic acid is inhibited by diets high in polyunsaturated fatty acids, Prostaglandins, Leukotrienes Essent. Fatty Acids, 2013, 88(1), 139–146 CrossRef CAS PubMed
.
- K. E. Wood, E. Mantzioris, R. A. Gibson, C. E. Ramsden and B. S. Muhlhausler, The effect of modifying dietary LA and ALA intakes on omega-3 long chain polyunsaturated fatty acid (n-3 LCPUFA) status in human adults: A systematic review and commentary, Prostaglandins, Leukotrienes Essent. Fatty Acids, 2015, 95, 47–55 CrossRef CAS PubMed
.
- J. Li, Z. Gu, Y. Pan, S. Wang, H. Chen, H. Zhang, W. Chen and Y. Q. Chen, Dietary supplementation of α-linolenic acid induced conversion of n-3 LCPUFAs and reduced prostate cancer growth in a mouse model, Lipids Health Dis., 2017, 16, 136 CrossRef PubMed
.
- T. Greupner, L. Kutzner, S. Pagenkopf, H. Kohrs, A. Hahn, N. H. Schebb and J. P. Schuchardt, Effects of a low and a high dietary LA/ALA ratio on long-chain PUFA concentrations in red blood cells, Food Funct., 2018, 9, 4742–4754 RSC
.
- H. Hejr, M. Ghareghani, K. Zibara, M. Ghafari, F. Sadri, Z. Salehpour, A. Hamedi, K. Negintaji, H. Azari and A. Ghanbari, The ratio of 1/3 linoleic acid to alpha linolenic acid is optimal for oligodendrogenesis of embryonic neural stem cells, Neurosci. Lett., 2017, 651, 216–225 CrossRef CAS PubMed
.
- M. A. Rincón-Cervera, R. Valenzuela, M. C. Hernandez-Rodas, C. Barrera, A. Espinosa, M. Marambio and A. Valenzuela, Vegetable oils rich in alpha linolenic acid increment hepatic n-3 LCPUFA, modulating the fatty acid metabolism and antioxidant response in rats, Prostaglandins, Leukotrienes Essent. Fatty Acids, 2016, 111, 25–35 CrossRef PubMed
.
- M. Hu, F. Xie, S. Zhang, B. Qi and Y. Li, Effect of nanoemulsion particle size on the bioavailability and bioactivity of perilla oil in rats, J. Food Sci., 2021, 86(1), 206–214 CrossRef CAS PubMed
.
- E. Murru, P. A. Lopes, G. Carta, C. Manca, A. Abolghasemi, J. L. Guil-Guerrero, J. A. M. Prates and S. Banni, Different dietary n-3 polyunsaturated fatty acid formulations distinctively modify tissue fatty acid and N-acylethanolamine profiles, Nutrients, 2021, 13, 625 CrossRef CAS PubMed
.
- J. R. Hibbeln, L. R. Nieminen, T. L. Blasbalg, J. A. Riggs and W. E. Lands, Healthy intakes of n-3 and n-6 fatty acids: estimations considering worldwide diversity, Am. J. Clin. Nutr., 2006, 83(6 Suppl.), 1483S–1493S CrossRef CAS PubMed
.
- T. H. Borges, L. C. López, J. A. Pereira, C. Cabrera-Vique and I. Seiquer, Comparative analysis of minor bioactive constituents (CoQ10, tocopherols and phenolic compounds) in Arbequina extra virgin olive oils from Brazil and Spain, J. Food Compos. Anal., 2017, 63, 47–54 CrossRef CAS
.
- J. Giacometti, Determination of aliphatic alcohols, squalene, α-tocopherol and sterols in olive oils: direct method involving gas chromatography of the unsaponifiable fraction following silylation, Analyst, 2001, 126, 472–475 RSC
.
- A. Zarrouk, L. Martine, S. Grégoire, T. Nury, W. Meddeb, E. Camus, A. Badreddine, P. Durand, A. Namsi, A. Yammine, B. Nasser, M. Mejri, L. Bretillon, J. J. Mackrill, M. Cherkaoui-Malki, M. Hammami and G. Lizard, Profile of fatty acids, tocopherols, phytosterols and polyphenols in mediterranean oils (argan oils, olive oils, milk thistle seed oils and nigella seed oil) and evaluation of their antioxidant and cytoprotective activities, Curr. Pharm. Des., 2019, 25, 1791–1805 CrossRef CAS PubMed
.
- O. Kyçyk, M. P. Aguilera, J. J. Gaforio, A. Jiménez and G. Beltrán, Sterol composition of virgin olive oil of forty-three olive cultivars from the World Collection Olive Germplasm Bank of Cordoba, J. Sci. Food Agric., 2016, 96, 4143–4150 CrossRef PubMed
.
- O. Demirag and D. B. Konuskan, Quality properties, fatty acid and sterol compositions of east mediterranean region olive oils, J. Oleo Sci., 2021, 70(1), 51–58 CrossRef CAS PubMed
.
-
D. Boskou, G. Blekas and M. TsimidouOlive oil composition, in Olive Oil: Chemistry and Technology, AOCS Press, 2nd edn, 2006, pp. 41–72, DOI:10.1201/9781439832028.pt2
.
|
This journal is © The Royal Society of Chemistry 2022 |
Click here to see how this site uses Cookies. View our privacy policy here.