DOI:
10.1039/D2FO00604A
(Paper)
Food Funct., 2022,
13, 5365-5380
Bioaccessibility of essential lipophilic nutrients in a chloroplast-rich fraction (CRF) from agricultural green waste during simulated human gastrointestinal tract digestion†
Received
28th February 2022
, Accepted 8th April 2022
First published on 26th April 2022
Abstract
An in vitro gastrointestinal human digestion model, with and without additional rapeseed oil, was used to measure the bioaccessibility of the major lipophilic nutrients enriched in chloroplasts: β-carotene; lutein; α-tocopherol; and α-linolenic acid. Chloroplast-rich fraction (CRF) material for this work was prepared from post-harvest pea vine field residue (pea vine haulm, or PVH), an abundant source of freely available, underutilised green biomass. PVH was either steam sterilised (100 °C for 4 min) and then juiced (heat-treated PVH, or HPVH), or was juiced fresh and the juice heated (90 °C for 3 min) (heat-treated juice, or HJ); the CRF from all biomass treatments was recovered from the juice by centrifugation. The impact of postharvest heat treatment of the biomass (HPVH), or of heat treatment of the juice (HJ) derived from the biomass, on the retention and bioaccessibility of the target nutrients was determined. The results showed that both heat treatments increased the apparent retention of β-carotene, lutein, α-tocopherol, and α-linolenic acid in the CRF material during digestion. The presence of edible oil during digestion did not dramatically affect the retention of these nutrients, but it did increase the bioaccessibility of β-carotene, lutein, and α-tocopherol from CRF material derived from heated biomass or juice. The presence of oil also increased the bioaccessibility of β-carotene, but not of lutein, α-tocopherol, or α-linolenic acid, from fresh CRF material.
1. Introduction
Green biomass is full of subcellular organelles called chloroplasts; their green colour is due to the presence of chlorophyll, central to the process of photosynthesis (conversion of sunlight energy into chemical energy). Recent work within Food Sciences at the University of Nottingham has shown that these organelles are composed of proteins (50–60%) and lipids (25–35%) and are enriched with a range of lipophilic micronutrients and essential trace elements (iron and manganese).1,2 Chloroplasts liberated from their cellular environment, should deliver more nutritional impact for the consumer than cell-bound chloroplasts since their digestion would not be impeded by the presence of the cell wall.
To be absorbed in the gastrointestinal (GI) tract, nutrients need to be bioaccessible. Bioaccessibility is the fraction of a compound which is released from its food matrix within the GI tract and becomes available for absorption.3In vitro digestion is a popular method of determining bioaccessibility since it is cheap, rapid and gives a preliminary evaluation before executing in vivo animal or human studies.4,5 The bioaccessibility of carotenoids, vitamin E and fatty acids from vegetables and fruit is reported to be relatively low.6–8 This has been attributed to several causes, including limited release from plant tissue9–11 and low solubilisation in gastrointestinal fluids.6,12 Due to the low solubilisation of lipophilic micronutrients in the aqueous phase in the gastrointestinal tract, movement from a food matrix to micelles/mixed micelles is necessary prior to uptake by epithelial cell. Some lipophilic nutrients (carotenoids, for example), are made more bioaccessible if there is some oil present in the diet to aid solubilisation.12,13 Work by Rich and co-workers (2003)14 showed that carotenoids from spinach leaves can move to micelles either directly or after transfer to an oil phase (oil droplets). The ability of oil to effectively dissolve lipophilic micronutrients and to provide products of digestion such as free fatty acids that form micelles means that the addition of oil to the diet can enhance the uptake of lipophilic micronutrients. Lipid hydrolysis takes place predominantly in the small intestine, but 10% to 25% of lipids can be hydrolysed in the gastric phase in solid and liquid meals respectively. Recently it has been shown that lipid digestion, initiated by gastric lipase can also enhance carotenoid bioaccessibility from plant food matrices.15 From the results of an in vitro study to test the ability of digestive lipases to hydrolyse galactolipids, we demonstrated that fatty acids released by pancreatic enzymes from liberated spinach chloroplasts are bioaccessible.16 The bioaccessiblity, and bioavailability, of fatty acids and lipophilic nutrients from liberated spinach chloroplasts has been shown in fish,17 but is yet to be established in a human feeding trial. A tractable step towards this goal is to measure the bioaccessibility of carotenoids and tocopherol (Fig. 1) from liberated chloroplasts, the so-called ‘chloroplast-rich fraction’ (CRF), in a human in vitro digestion model.
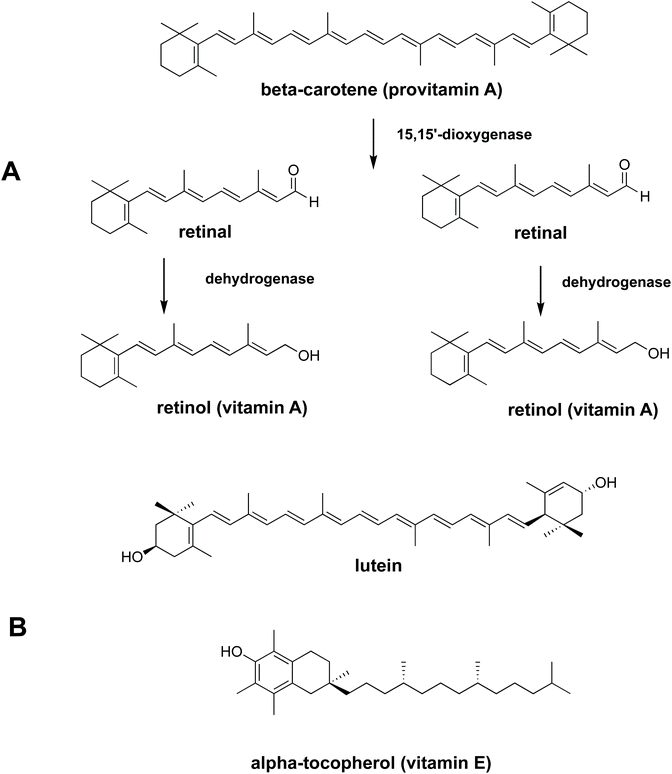 |
| Fig. 1 Chemical structures of essential lipophilic nutrients (A) conversion of β-carotene (provitamin A) to retinol (vitamin A) (B) chemical structures of lutein and α-tocopherol. | |
The UK is the largest producer of green peas for freezing in Europe. In terms of land area, 35
000 hectares of peas are cultivated in the UK.16 We estimate that the mass of postharvest field residue (called pea vine haulm (PVH) made up of leaves, pods, stems) in the UK is 373 thousand tonnes per year. Currently this biomass is either ploughed back into the field or used to feed livestock. Soil health benefits from the former, but it is generally accepted that at least half the biomass could be removed from the field for other purposes. Our laboratory has demonstrated that the CRF from postharvest pea vine field residue i.e. pea vine haulm (PVH), has the nutritional credentials to be used as a food supplement or food ingredient.2,16,18 Heat treatment of the biomass is an effective way of knocking out endogenous enzymes that might cause a deterioration in the quality of PVH CRF material during storage.16,18 Heat treatment of the juice recovered from the biomass is another way of preserving the nutrients in the final CRF material/dry powder.16 CRF material is prepared by centrifuging leaf-juice, collecting the pellet which is then freeze-dried. Although there are reports of the positive impact of juicing/homogenising green biomass on the bioaccessiblity and bioavailability of leaf-bound lipophilic nutrients,7,12,14,19–22 this is the first bioaccessibility study where chloroplasts have been intentionally liberated from cells, concentrated/separated from the bulk of the aqueous phase, and dried. This current work measures, both in the presence and absence of edible oil, the bioaccessibility of the major lipophilic nutrients in a chloroplast-rich fraction (CRF) derived from fresh juice (FJ), heat-treated pea vine haulm (HPVH), and heat-treated PVH juice (HJ).
2. Materials and methods
2.1. Materials
Pea (Pisum sativum L.) vine haulm, composed of a mixture of vines, stems, leaves, peas, and pods, was kindly provided by the Green Pea Company (Yorkshire, United Kingdom). The biomass was collected from the side of the field during the pea harvest (July 2017) and immediately brought to our laboratory facilities in Leicestershire, UK to be processed. Rapeseed oil and spinach leaves were purchased from Tesco, UK.
2.2. Chemicals1–51
All solvents were HPLC grade and purchased from Fisher Scientific (Loughborough, UK). Standards (β-carotene and α-tocopherol) were HPLC grade and purchased from Sigma Aldrich (Merck, Darmstadt, Germany), except lutein which was purchased from PanReac AppliChem (Darmstadt, Germany). Enzymes, including α-amylase from human saliva type XIII-A, 300–1500 U mg−1 protein (A1031), pepsin from porcine gastric mucosa (P6887), pancreatin from porcine pancreas (8xUSP; P7545), and porcine bile extract (B8631), were purchased from Sigma Aldrich (Merck, Darmstadt, Germany). Other chemicals used were of analytical grade.
2.3. Post-harvest treatment of pea vine haulm (PVH) and preparation of juice
The fresh biomass was washed with tap water to remove any soil or stones, before removing excess water in an industrial salad spinner (Sammic ES-200, UK). The haulm was split into two batches. One batch (13 kg) was packed into vacuum sealed bags for steam treatment (section 2.3.1). The other batch (5 kg) was juiced immediately, using a twin gear juicer (Angel 7500, Korea) to separate the fibrous pulp from the nutrient-rich juice. This juice was filtered through a 75 μm stainless steel mesh sieve and then processed further to make the CRF material (section 2.5).
2.3.1 Heat treatment of PVH (HPVH).
The PVH was packed into vacuum sealed, clear, perforated bags (500 g of pea vine per bag). These bags were placed in the rack of a Retort (Lagarde RP362, Malataverne, France). The chamber was sealed, vented, and heated for 5 minutes 30 seconds to reach 100 °C and 1 bar, then held for 4 minutes before cooling and depressurising for 5 minutes 45 seconds. The bags were then rapidly cooled in an ice-water bath. The steam-treated PVH (HPVH) was immediately juiced using a twin-gear juicer (Angel 7500, Korea) and processed further to make the CRF material (section 2.5).
2.3.2 Heat treatment of juice extracted from pea vine haulm (HJ).
Pea vine juice (HJ) (500 mL), in a capped amber Duran bottle, was heated in a water bath with a magnetic stirrer (800 rpm). The temperature was increased from room temperature (20 °C) to 90 °C over 20 minutes, held for 3 minutes, then cooled rapidly in an ice-water bath to room temperature. The CRF material was then prepared (section 2.5).
2.4. Treatment of spinach leaves
Spinach leaves (3 kg) were juiced using a twin-gear juicer (Angel 7500, Korea); the juice was filtered through a 75 μm stainless steel mesh sieve and processed further to make the CRF material (section 2.5).
2.5. Isolation of the chloroplast-rich fraction (CRF)
The CRF material was prepared using a method from Torcello-Gomez et al. (2019),2 who proved that the CRF contains intact chloroplasts after the processing using microscopic technique. The juice samples prepared from section 2.3, 2.3.1, 2.3.2 and 2.4 were centrifuged twice at 17
700 RCF or 10
000 rpm (Beckman Coulter JS-21M centrifuge with JA-10 rotor, US) for 10 minutes at 4 °C. The pellets containing the CRF were pooled, weighed and frozen at −80 °C before being freeze-dried (Edwards Freeze Dryer Super Modulyo, UK) for 3–5 days. Freeze-dried CRFs of fresh juice (FJ), heated PVH (HPVH), heated juice of PVH (HJ), and spinach were then ground in a pestle and mortar and stored in a vacuum-sealed foil pouch at −20 °C.
2.6. Peroxidase (POD) enzyme activity assay
General enzyme activity was measured using a peroxidase (POD) enzyme assay adapted from Gökmen et al. (2005).23 Samples of juice were diluted 1 in 10 with deionised water to be added to an assay mix of 500 μL of each hydrogen peroxide and guaiacol in a total volume of 100 mL deionised water. The rate of colour change induced by enzyme activity was measured using a spectrophotometer (UV-Vis spectrophotometer, Genesys 10S, US) set at 420 nm. Readings were taken every 2 seconds over a 90-second period, after 100 μL diluted juice was added to 2.9 mL assay mix. The gradient of the increased absorbance for 90 seconds was a measure of POD enzyme activity, any reduction in POD activity after heat treatment was an indication of a reduction of enzyme activity in general.
2.7. Static in vitro digestion
The digestibility of nutrients in vitro was determined using the method of Minekus et al. (2014),4 with slight modifications (see ESI Fig. 1†). The CRF (0.5 g) was dissolved in 4.5 mL or 4.0 mL of ultra-pure water when rapeseed oil (0.5 g) was absent or added respectively. Rapeseed oil was chosen for the study because it has been reported to give the highest bioaccessibility of lipophilic nutrients (β-carotene) from vegetables when compared with oil from soybean, safflower seed, olive fruit, sesame seed, palm fruit, beef tallow and lard.12 The rapeseed oil and was homogenised with the solution and then mixed with 4 mL of simulated salivary fluid (SSF) electrolyte stock solution. Human salivary α-amylase solution (75 U mL−1; 0.5 mL) was added, followed by CaCl2·2H2O (0.3 M; 25 μL) and ultra-pure water (0.475 mL). This mixture was thoroughly mixed and shaken (150 rpm) for 2 minutes at 37 °C and then simulated gastric fluid (SGF) electrolyte stock solution (8 mL) was added to the digestion mixture. Gastric lipase, recommended in the latest iteration of the INFOGEST model,5 was not used at the time we performed these experiments; however, we have shown that the main lipids present in CRF, galactolipids, are not substrates for gastric lipase and their digestion only starts under intestinal conditions.5 Pepsin from porcine gastric mucosa (1 mL; 2000 U mL−1) and CaCl2·2H2O (0.3 M; 5 μL) were then added to achieve 2000 U mL−1 pepsin in the final gastric digestion mixture. The pH was then reduced to 3 using 2 N HCl and the volume was made up to 20 mL with ultra-pure water. The samples were then shaken (150 rpm) for 2 hours at 37 °C. Next, the digesta from the gastric phase (20 mL) was mixed with simulated intestinal fluid (SIF) electrolyte stock solution (11 mL), pancreatin solution (5 mL made up in SIF electrolyte stock solution to reach 100 U mL−1 of trypsin activity), and bile extract (2.5 mL at 10 mM bile salts) and CaCl2·2H2O (0.3 M; 40 μL). The pH was then increased to 7 using 1 N NaOH and the volume made up to 40 mL with ultra-pure water. The intestinal digestion mixture was again incubated in the shaker (150 rpm) for 2 hours at 37 °C. The digestion sample was acidified with 8 mL of 1 N HCl to stop the enzymatic reaction. To assess the bioaccessibility of lipophilic nutrients (carotenoids, vitamin E and fatty acids), 10 mL of the intestinal digestion mixture was fractioned by centrifugation at 5000 rpm or 4863 RCF (Beckman Coulter JS-21M centrifuge with JA-10 rotor, US) for 10 minutes at 4 °C for 1 hour and the resulting supernatant was filtered through 0.2 μm polytetrafluoroethylene (PTFE) filter membrane to obtain the micellar phase. Then lipids were extracted from both the non-centrifuged sample (digesta) and the micellar phase (section 2.8). The percentages (%) of nutrient retention after digestion, bioaccessibility, and nutrient in CRF available for uptake were calculated using eqn (1)–(3): | %Nutrient retention = [(Cdigesta)/(Cinitial)] × 100 | (1) |
|  | (2) |
|  | (3) |
Cmicellar is the concentration of the nutrient in the micellar fraction, Cdigesta is the concentration of the nutrient in the complete digestion mixture before centrifugation, and Cinitial is the concentration of the nutrient in CRF before digestion.
2.8. Lipid extraction
Lipid extraction was performed using the method of Folch et al. (1957).24 The micellar phase or total digesta (5 mL) were mixed with a 2
:
1 v/v mixture of chloroform and methanol (5 mL) and vortexed for 1 minute. Sodium chloride solution (150 mM; 2 mL) was then added to the mixture which was vortexed again (1 minute) and centrifuged (using Thermo Electron Corporation, Jouan CR3i multifunction, US) at 3000 rpm or 1750 RCF for 10 minutes at 4 °C, after which it had separated into two phases (aqueous and organic) and an interphase. The lower clear organic phase, which contained lipids, was transferred into another tube. The remaining two phases were then extracted and centrifuged again according to the same methods. The lower organic phase was collected and pooled with the previous one. The lipid extract was filtered through a 0.45 μm PTFE filter membrane and then dried under a flow of nitrogen gas. The dried lipid extracts were weighed to calculate the total lipid content and subsequently used for the analysis of β-carotene, lutein, α-tocopherol, and α-linolenic acid.
2.9. Protein analysis
The protein content was determined using Pierce™ BCA protein assay kit (Thermo Fisher Scientific). 2% of Sodium dodecyl sulfate (SDS) solution (1 mL) was added to 0.1 g of dried sample. The sample was then heated at 60 °C in the water bath for 30 min and vortexed for 1 minute. After that the sample was centrifuged (using ROTINA 380R) at 4000 rpm or 3112 RCF for 10 minutes and further diluted 1/100 with 2% SDS solution. A standard working reagent (2 mL) was added to 100 μL of each sample and standard solutions and further incubated at 37 °C for 30 minutes. The standard working reagent was prepared by using 50 parts of reagent A and 1 part of reagent B (supplied in the assay kit). The absorbance was measured at 562 nm using an UV-Vis spectrophotometer (Thermo Scientific, Genesys 10S, US) blanked with reverse osmosis (RO) water. The concentration was determined using linear equation produced from a range of bovine serum albumin (BSA) standards.
2.10. Mineral analysis
The freeze dried CRF from spinach and PVH were transferred into Pyrex test tubes (16 × 100 mm) and weighed (5–10 mg). The trace metal grade nitric acid Primar Plus (Fisher Chemicals) spiked with indium internal standard was added to the tubes (2 mL per tube). The samples were then digested in dry block heater (DigiPREP MS, SCP Science; QMX Laboratories, Essex, UK) at 115 °C for 4 hours. Once the samples cooled down, 1 mL of hydrogen peroxide (Primar, for trace metal analysis, Fisher Chemicals) was added to the tubes and samples were digested in dry block heater for additional 4 hours at 115 °C. The digested samples were diluted to 20 mL with 18.2 MΩ cm Milli-Q Direct water (Merck Millipore). Elemental analysis was performed with an inductively coupled plasma-mass spectrometry (ICP-MS), PerkinElmer NexION 2000 equipped with Elemental Scientific Inc. autosampler, in the collision mode (He). Twenty-two elements (Li, B, Na, Mg, P, S, K, Ca, Cr, Mn, Fe, Co, Ni, Cu, Zn, As, Se, Rb, Sr, Mo, Cd and Pb) were monitored. Liquid reference material composed of pooled samples of the digested CRF powders was prepared before the beginning of sample run and was used throughout the whole samples run. It was run after every ninth sample to correct for variation within ICP-MS analysis run.25 The calibration standards (with indium internal standard and blanks) were prepared from single element standards (Inorganic Ventures; Essex Scientific Laboratory Supplies Ltd, Essex, UK) solutions. Sample concentrations were calculated using external calibration method within the instrument software. The ash contents of PVH CRF and spinach CRF were determined from the total amount of minerals presented in samples.
2.11. Estimation of carbohydrate content
The percentage of carbohydrate content of freeze-dried CRF was estimated by difference of the other macronutrient and total minerals (ash) using eqn (4). | Carbohydrate (%) = 100 − lipid − protein − total mineral (ash) | (4) |
2.12. Chlorophyll analysis
The pigment content was analysed using an UV-Vis spectrophotometer (Thermo Scientific, Genesys 10S, US). The lipid extract was diluted with acetone to be a factor of 1
:
100 for PVH CRF and a factor 1
:
500 for spinach CRF. The sample solution was measured at two different wavelengths; 662 nm (chlorophyll a) and 645 nm (chlorophyll b). Chlorophyll concentration (μg mL−1) was calculated using eqn (5)–(7) by Lichtenthaler and Buschmann (2001).26
Chlorophyll a:
| Chlorophyll a (μg mL−1) = (11.24 × A662) − (2.04 × A645) | (5) |
Chlorophyll b:
| Chlorophyll b (μg mL−1) = (20.13 × A645) − (4.19 × A662) | (6) |
Total chlorophylls:
| Total chlorophylls (μg mL−1) = chlorophyll a + chlorophyll b | (7) |
2.13. β-Carotene and lutein analyses
The amount of β-carotene and lutein were analysed using high-performance liquid chromatography (HPLC) with photo diode array (PDA) detection (Agilent 1100, Germany) using the method described in Wattanakul et al. (2021).18 The dried lipid extract was dissolved with acetone (containing 0.1% butylated hydroxytoluene (BHT)) (1 mL) and filtered through a 0.45 μm PTFE filter membrane into amber HPLC vials. The mobile phase consisted of acetonitrile, methanol, ethyl acetate containing 0.05% triethylamine (TEA) at a flow rate of 0.5 mL min−1. At the start of the run the proportions of the solvents were 95
:
5
:
0, which changed to 60
:
20
:
20 after 25 minutes; the latter proportions were maintained until the end of the run. Re-equilibration took 15 minutes. Samples were injected at 10 μL of volume through a guard column and separated on a Waters, Nova-Pak C18 analytical column (4 μm, 3.9 × 200 mm) with the column temperature set at 22 °C. β-Carotene and lutein were detected at a wavelength of 454 nm. The β-carotene and lutein contents were determined using linear equations from standard curves.
2.14. α-Tocopherol analysis
α-Tocopherol content was analysed using HPLC (Agilent 1100, Germany) with fluorescence detector (Jasco FP-920, Japan) using a method of Wattanakul et al. (2021).18 The dried lipid extract was dissolved with methanol (containing 0.1% BHT) (1 mL) and filtered through 0.45 μm PTFE filter membrane into amber HPLC vials. A gradient system of two solvents, A (acetonitrile
:
methanol
:
isopropanol
:
1% acetic acid solution 45
:
45
:
5
:
5, v/v/v/v) and B (acetonitrile
:
methanol
:
isopropanol, 25
:
70
:
5 v/v/v), was employed. The flow rate of the mobile phase was set at 0.8 mL min−1 starting with 100% of solvent A for 6 minutes. Then the mobile phase changed linearly to become 100% of solvent B over the following 10 minutes. This system was held for 12 minutes before being returned to the initial conditions with a total run time of 36 minutes. Samples were injected at a volume of 10 μL through a guard column and separated on a Zorbax RX, C8 analytical column (5 μm, 4.6 × 250 mm) with the column temperature set at 20 °C. α-Tocopherol was detected at the wavelength of 298 nm for excitation and 328 nm for fluorescence emission. The α-tocopherol content was determined using a linear equation from the standards curve.
2.15. α-Linolenic acid analysis
The amount of α-linolenic acid was analysed using gas chromatography coupled to mass spectrometry detection (GC-MS) (Thermo Scientific, DSQ) using the method described in Wattanakul et al. (2021).18 A dried lipid extract was dissolved in chloroform (1 mL). Methylpentadecanoate (100 μL) was added as an internal standard and trimethylsulfoniumhydroxide (TMSH) (200 μL) was added to convert the fatty acids and fatty acyl groups to fatty acid methyl esters. The lipid extract was then left for at least 10 minutes to ensure completed conversion before filtering the mixture (1 mL) through 0.45 μm PTFE filter membrane into amber vials. The sample (10 μL) was injected into a Phenomenex Zebron ZB-FFAP (30 m × 0.25 mm) column using a vaporising injector with a split flow of 50 mL min−1. The oven temperature was maintained at 120 °C for 1 minute and then increased to 250 °C at a rate of 5 °C min−1 and held for 2 minutes. α-Linolenic acid was detected using a mass spectrometer and identified using retention times and comparison of molecular masses to a mass spectrum library of FAME standards.
2.16. Microscopic imaging
The chloroplast morphology was observed by light microscope with polarised lens and dark field (Leitz, Diaplan Microscope, Germany) and EVOS microscope with 40× magnification (EVOS FL Imaging System, Advanced Microscopy Group).
2.17. Statistical analysis
All experiments were performed in triplicate, and the data analysed using IBM SPSS statistics 27. Analysis of variance (ANOVA) was carried out according to the Tukey post hoc approach; statistical significance was set at p < 0.05. The data were expressed as mean ± standard deviation. Differences of means at p < 0.05 were considered significant.
3. Results and discussion
3.1. Composition of CRF materials before in vitro digestion
Three separate CRF materials were produced from pea vine haulm (PVH): fresh/unheated PVH juice (FJ); heated PVH (HPVH); and heated juice (HJ). Spinach CRF prepared from fresh juice was used as reference material: it is commonly studied in our group and it has a relatively high nutrient composition.1 Spinach is also a standard material for plant biologists and research on photosynthesis derived compounds.27 The proximate and selected lipid micronutrient compositions of these materials were measured (Table 1). Overall, the total lipid content (a good measure of chloroplasts in green biomass) in the three CRF materials was in the same order of magnitude, with fluctuations explained by the varying levels of proteins and carbohydrate in the three CRF materials. Most of the samples, including the spinach leaf CRF reference sample, show a protein
:
lipid ratio of 2
:
1; however, the CRF from HJ has a ratio of 2.8
:
1. It is not clear why this should be; perhaps some cytoplasmic proteins dropped out of solution due to heat-induced denaturation and were then recovered in the CRF pellet upon centrifugation. The carbohydrate content of the CRF in no-heat-treated material was significantly higher (p < 0.05) (1 to 2-fold) than the carbohydrate content of the heat-treated materials. The observation of Maltese crosses using a polarised microscopy indicated the presence of native starch in the no-heat treated CRF sample (FJ). Heat treatment of the PVH directly (HPVH) or of the juice (HJ), resulted in the loss of native starch structure (Fig. 2B and C). HJ appears to have the fewest native starch grains suggesting that the juice experienced even greater thermal impact than the HPVH material, this is reflected in the heating protocols (section 2.3.1 and 2.3.2) and echoed by the reduction in peroxidase activity results (see later). It appears from these observations that the heat treatment of biomass or juice caused gelatinisation of starch and that much of the gelatinised starch material remains in the supernatant during the preparation of CRF. The presence of starch effectively dilutes the other nutrients so this must be taken into account when comparing the concentration of nutrients in the CRF materials. One way of doing this is to express the nutrient/pigment concentration values in terms of mass per unit mass of total lipid, as we can assume that most of the lipid extracted from CRF originate from the chloroplast membranes. This normalised measure enables a comparison of the nutrient concentrations across the CRF materials. Expressing concentration values relative to total lipid mass reveals that heat treatment reduces the chlorophyll content of PVH CRF (Table 1 and Fig. 2A); about 40% of the chlorophyll was lost during the heat treatment of the biomass or juice, probably because of the loss of magnesium bound in the chlorophyll and due to some chlorophyll changing to pheophytin and other derivatives, such as chlorophyllide and pheophorbide.28 Experiments were carried out in the dark, so it is unlikely that singlet oxygen was generated. It is worth bearing in mind that if any singlet oxygen were produced then thermal inactivation of enzymes that have antioxidant activity may explain some of the loss of chlorophyll.29,30 The concentration of the carotenoids, β-carotene and lutein, was relatively consistent between the FJ and HJ samples, but pre-heating the biomass (HPVH) appears to cause a loss in these micronutrients (34% and 40% losses respectively). Heated juice caused a higher loss of peroxidase (POD) enzyme activity (data shown in ESI Table 1†) than heated biomass (100% compared with 66% loss of POD), therefore it may be that it also knocks out enzymes that catalyse the breakdown of carotenoids. The highest value for α-tocopherol is found in the CRF material from heated juice; perhaps this was also explained in part by heat-induced disabling of tocopherol oxidase that can breakdown tocopherol.31 Any losses of nutrients in these CRF materials may be explained by endogenous enzyme activity or by heat-induced breakdown; any commercial heat treatment would have to optimise conditions to minimise carotenoid degradation and to maximise enzyme inactivation. These figures taken overall indicate that all CRF preparations contain measurable amounts of β-carotene, lutein, and α-tocopherol.
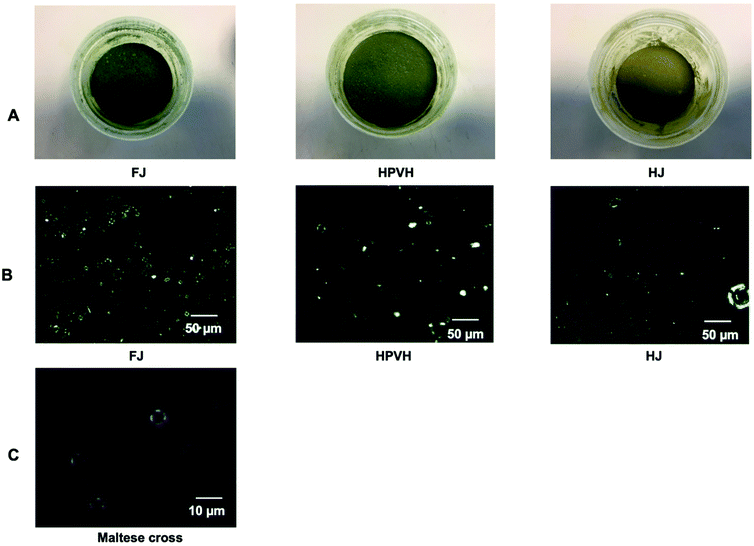 |
| Fig. 2 Physical characteristics of different freeze-dried CRF from PVH, including CRF from fresh juice (FJ), CRF obtained after heated PVH before juicing (HPVH) and CRF prepared after heated PVH juice (HJ); (A) physical characteristic of freeze-dried CRF (B) light microscope polarised image with dark field; (C) close-up view of the Maltese cross. Maltese crosses can be observed in the non-heat treated CRF sample, which indicates the presence of native starch. | |
Table 1 Nutrient composition of chloroplast-rich fractions (CRFs) from pea vine haulm (PVH) before digestion
Nutrient |
|
PVH CRF |
Spinach CRF |
FJ |
HPVH |
HJ |
CRF: chloroplast-rich fraction; DW: dry weight; FJ: fresh juice; HPVH: heated PVH; HJ: heated juice; data were presented as a mean ± SD of 3 separated samples and analysed using post hoc analysis of variance (ANOVA) and according to a Tukey test with statistical significance at p < 0.0.5, a > b. |
Protein |
mg g−1 CRF, DW |
293.27 ± 10.11d |
354.33 ± 10.36c |
448.32 ± 3.29b |
483.64 ± 6.66a |
Lipid |
mg g−1 CRF, DW |
150.82 ± 3.78b |
173.34 ± 31.23b |
160.13 ± 9.87b |
239.92 ± 13.12a |
Protein to lipid ratio |
w/w |
1.94 |
2.04 |
2.80 |
2.01 |
Carbohydrate |
mg g−1 CRF, DW |
525.73 ± 0.14a |
391.11 ± 1.27b |
289.80 ± 0.62c |
215.12 ± 1.76d |
Ash |
mg g−1 CRF, DW |
30.18 ± 0.14d |
81.22 ± 1.27b |
101.75 ± 0.62a |
61.32 ± 1.76c |
Total chlorophyll |
mg g−1 CRF, DW |
15.99 ± 1.33b |
10.73 ± 0.73c |
10.44 ± 0.44c |
46.16 ± 1.56a |
mg g−1 lipid |
106.01 ± 8.79b |
61.92 ± 4.4c |
65.21 ± 2.85c |
192.40 ± 6.49a |
β-Carotene |
mg g−1 CRF, DW |
0.99 ± 0.06bc |
0.74 ± 0.08c |
1.21 ± 0.07b |
5.29 ± 0.33a |
mg g−1 lipid |
6.53 ± 0.42b |
4.29 ± 0.45c |
7.55 ± 0.45b |
22.07 ± 1.36a |
Lutein |
mg g−1 CRF, DW |
4.81 ± 0.29a |
3.34 ± 0.39b |
4.62 ± 0.34a |
3.90 ± 0.39ab |
mg g−1 lipid |
31.88 ± 1.89a |
19.30 ± 2.28b |
28.83 ± 2.09a |
16.25 ± 1.64b |
α-Tocopherol |
mg g−1 CRF, DW |
0.29 ± 0.02b |
0.29 ± 0.02b |
0.45 ± 0.04a |
0.52 ± 0.04a |
mg g−1 lipid |
1.94 ± 0.14bc |
1.65 ± 0.14c |
2.82 ± 0.24a |
2.17 ± 0.19b |
3.2. Bioaccessibility of lipophilic nutrients from CRFs derived from FJ, HPVH and HJ
The microscope images (ESI Fig. 2†) were taken to show the impact of in vitro digestion on the different treatments of PVH CRF at each digestion phase. Images of the materials sampled at the end of oral phase indicates that the CRF generated from the fresh juice contains larger more opaque aggregates compared with the other CRF preparations. In all cases there is a gradual reduction in the size of aggregates during in vitro digestion. The bioaccessibility of micronutrients from these three CRF powders was measured by using an in vitro digestion model. Initially, water (4.5 mL) was added to CRF powder (0.5 g); in all three cases, the water penetrated the powder to some extent, but complete dispersion of the particulate material did not occur (ESI Fig. 3†). Over the digestion period, residual CRF material can be seen sticking on the wall of the glass bottle; this material was re-suspended into the incubation mixture at the start of each new phase (oral, gastric or intestinal) by inverting the bottles, but some of the particulate material remained stuck to the glass indicating incomplete dispersion in some cases (ESI Fig. 3†). It could be deduced that the dispersion of the CRF material in the digestion mixture was enhanced if the PVH or its juice was heat treated, or if rapeseed oil was added to the incubation mixture (see ESI Fig. 3†). Perhaps the presence of native starch in the no-heat treated CRF material impaired particulate dispersion, and this phenomenon could be overcome in the presence of oil. Bioaccessibility is a measure of the release of nutrients from a matrix; it is therefore necessary to establish loss of nutrients during incubation as this can affect the measurement of bioaccessibility (see 3.2.2–3.2.4).
3.2.1. Isomerisation of β-carotene before and after CRF digestion.
A small peak of cis-β-carotene in the HPLC chromatogram was present in the heat-treated samples (HPVH and HJ) before in vitro digestion (ESI Fig. 4†). This result was consistent with Chandler & Schwartz (1988), who reported that thermal processing can cause the isomerisation of β-carotene from trans to cis-isomer (ESI Fig. 5†).32 After in vitro digestion, there was an increasing amount of cis-β-carotene (9-cis and 13-cis forms) in both non-heat (FJ) and heat-treated samples (HPVH and HJ), compared with before digestion (ESI Fig. 4†). The isomerisation of trans to cis-β-carotene in PVH CRF therefore also occurred during in vitro digestion. cis-β-Carotene is reported to be easier to micellarise than all-trans-β-carotene during simulated digestion; both isomers are transferred across the brush-border surface of the enterocyte from mixed micelles and absorbed in humans by passive diffusion.33 Measurement of β-carotene in this work was the sum of the all-trans and the cis isoforms.
3.2.2. Nutrient retention.
In this work, nutrient retention is defined as the proportion of nutrients in the whole digesta (at the end of the digestion process) compared with the initial nutrient concentration in the CRF. It reflects the stability of lipophilic nutrients. To make this measurement it is assumed that all lipids are extractable from the total digesta milieux. From Fig. 3 one can see that our internal marker for chloroplast lipid (α-linolenic acid), which we assume is not affected by the incubation conditions, appears to be lost during digestion: 40% is apparently lost from the CRF derived from FJ, and 20% is apparently lost from the CRF derived from heat treated samples (HPVH and HJ). It has already been noted that the CRF materials vary in their degree of dispersibility; from qualitative observations it appears that CRF from fresh juice was the least well dispersed (ESI Fig. 3†). The robust physical nature of CRF material appears to be reduced after heat treatment of the biomass or juice. This may explain in part the ‘apparent’ improved retention of β-carotene, lutein, and α-tocopherol in HPVH and HJ CRF materials; it is possible that the less robust, more easily dispersed CRF materials from heat treated biomass or juice, simply release their lipids more readily during extraction, or that representative sampling of the digesta was impaired by the larger, less well-dispersed CRF particles derived from fresh, unheated juice.
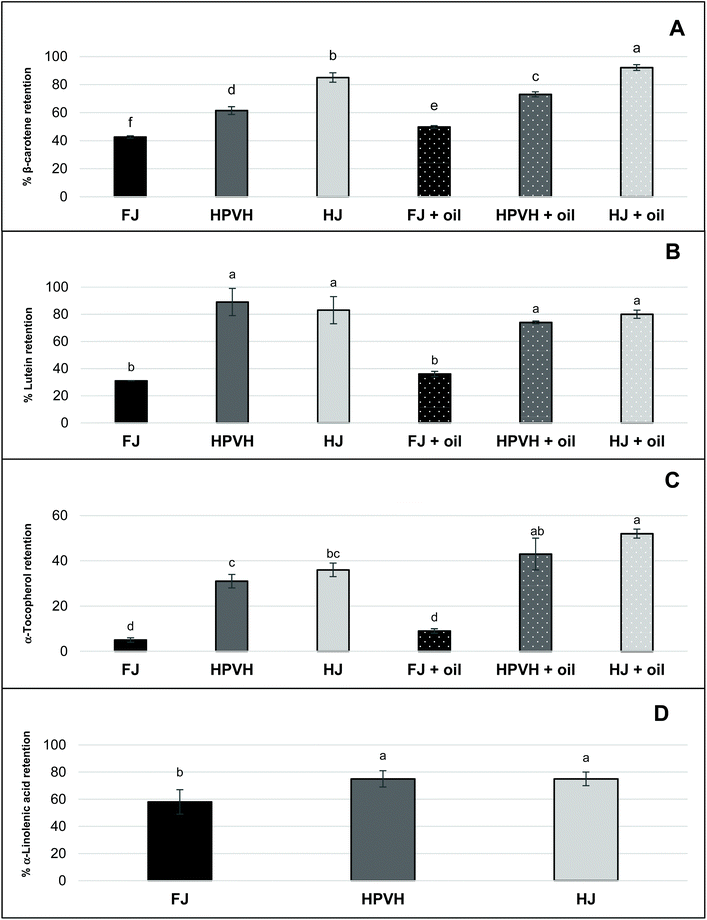 |
| Fig. 3 Effect of heat treatment and the presence of oil on nutrient retention after in vitro digestion in CRF from PVH (A) β-carotene retention (B) lutein (C) α-tocopherol (D) α-linolenic acid; FJ: fresh juice; HPVH: heated PVH; HJ: heated juice; data were presented as a mean ± SD of 3 separated in vitro digestion and analysed using post hoc analysis of variance (ANOVA) and according to a Tukey test with statistical significance at p < 0.0.5, a > b. | |
The presence of oil during digestion appears to improve the retention of β-carotene and α-tocopherol, but lutein is not affected. Another possible explanation is that oil helps to dissolve the most lipophilic of the analytes (β-carotene and α-tocopherol) from the particulate CRF material, making these nutrients more easily extractable using the Folch method.24 This reflects our proposition that the physical nature of the CRF material affects how readily the lipids are extracted from the total digesta. Work in our group has shown that our lipid extraction protocol is effective at extracting all the lipids from the dry CRF parent material, but there appears to be more work to be done to refine the protocol to obtain quantitative amounts of lipid from CRF dispersed in an aqueous medium. Indeed, some of the retention data may be explained by various factors. Our study suggests that heat treatment of biomass or juice improves the retention of β-carotene, lutein, and α-tocopherol during simulated human digestion of CRF material (Fig. 3A–C). This may be in part due to heat-induced disabling of the enzymes that cause the degradation of carotenoids and vitamin E.34,35 In addition, thermal treatment might cause more efficient release of carotenoids from the CRF matrix and/or induce disruption of carotenoid-protein complexes.34 The presence of oil in PVH CRF digestion appeared to increase the retention of β-carotene compared with the absence of oil. One can assume that rapeseed oil hydrolysis during in vitro digestion generates lipolysis products, which contribute to the micellar solubilisation and protection of β-carotene under intestinal conditions.15,36
3.2.3. Nutrient bioaccessibilty (micellar solubilisation).
“Bioacessibility” or “micellar solubilisation” are defined as the percentage of nutrient present in the whole digesta that is dispersed in solution, and thus. Presumably micellarised and available for intestinal absorption. Notwithstanding the likely role of the physical nature of the CRF material in rendering lipophilic nutrients more of less accessible for extraction from the digesta phase, it is clear from the bioaccessibility results (Fig. 4) that not all the extractable lipid nutrients end up in the micellar phase. This suggests that a proportion of these lipophilic nutrients is hindered from moving into the micellar phase; this will be due to mass transfer constraints and/or because some of these nutrients are caught up in non-micellar entities such as vesicles or undigested lipid droplets, and/or are still attached to the CRF matrix in some fashion. Bioaccessibility data in this study (Fig. 4) therefore still carries useful information despite the incomplete recovery of lipophilic nutrients from the digesta phase. It does not mirror the profile of the ‘retention’ data (Fig. 3), indicating that there are molecule-specific drivers for micellarisation.
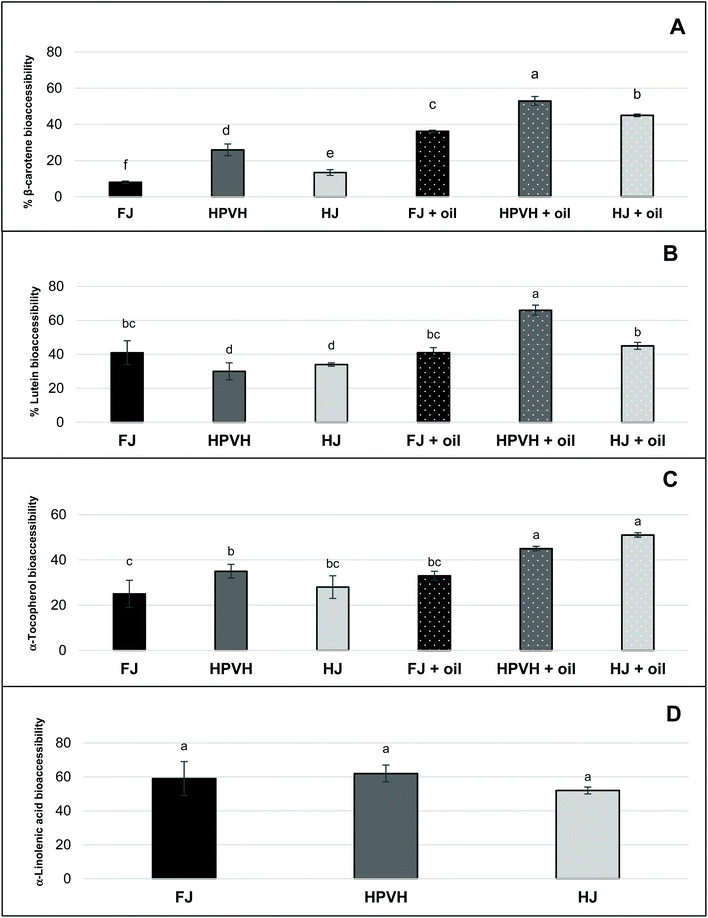 |
| Fig. 4 Effect of heat treatment and the presence of oil on nutrient bioaccessibility (micellar solubilisation) after in vitro digestion in CRF from PVH (A) β-carotene bioaccessibility (B) lutein (C) α-tocopherol (D) α-linolenic acid; FJ: fresh juice; HPVH: heated PVH; HJ: heated juice; data were presented as a mean ± SD of 3 separated in vitro digestion and analysed using post hoc analysis of variance (ANOVA) and according to a Tukey test with statistical significance at p < 0.0.5, a > b. | |
Galactolipids from thylakoid membranes appear to be digested as approximately 60% of α-linolenic acid (our internal marker for chloroplast lipid) moves from the digesta phase into the micellar phase in all three CRF materials (Fig. 4D); there is no data for α-linolenic acid retention or bioaccessibility in the presence of oil. Our study of the hydrolysis of galactolipids from CRF material in an in vitro simulation of human digestion indicated that a very high percentage of fatty acyl groups (up to 70%) is released as FFAs during CRF material preparation (presumably by endogenous galactolipase enzymes present in the green biomass) if the PVH biomass has not been heat treated.16 Conversely heat treatment of PVH protects the integrity of galactolipids to a great extent since only 25% of fatty acyl groups are converted into FFAs. Although we did not measure FFAs directly in the present work, we assume that CRF from FJ is high in FFAs (including the abundant α-linolenic acid), whereas CRF from HPVH or HJ will have a significantly lower amount of FFA prior to digestion. The bioaccessibility data for α-linolenic acid (Fig. 4D) therefore suggests that FFAs move easily into the micellar phase (indeed free fatty acids are key constituents of micelles) from the FJ CRF material, and that the PPE (porcine pancreatic extract) used in this work is able to hydrolyse galactolipids to some extent, which is consistent with our previous findings16 and the fact that some galactolipase activity has been identified in PPE.37 The latter deduction is based on the assumption that galactolipids do not move into the micellar phase intact, but rather are hydrolysed first, liberating FFAs and lysogalactolipids that are inclined to form mixed micelles with bile salts. It is also possible that, instead of being directly hydrolysed within thylakoid membranes, intact galactolipids form vesicles and mixed micelles that are dispersed in the aqueous phase, prior to being hydrolysed.38 The apparent bioaccessibility of β-carotene was highest when oil was included during digestion and when CRF material was derived from heat-treated materials (Fig. 4A). The lipolysis of oils and fats generate FFAs and monoglycerides that partition to mixed micelles that assist in the solubilisation of carotenoids during the transfer of gastric chyme to the duodenum.12,13,36,39 This process in which both the initial lipid and its digestion products are important for solubilisation is similar to what is observed with lipid-based formulations (LBF) for the oral delivery of poorly water-soluble drugs.40 Although not pertinent to this in vitro study, it is interesting to note that FFAs, in vivo act as stimulants of both pancreatic and biliary secretions, thus facilitating lipolysis and micellar solubilisation by bile salts.36 Furthermore, hydrophobic carotenoids (i.e. β-carotene) have a higher propensity to be localised in the bulk oil phase, and require the production of lipolysis products interacting with water in order to be transferred to the micelles.41 Most lutein bioaccesssiblity values (Fig. 4B) are around 40% which reflects the best of the β-carotene data (Fig. 4A). There appears to be greater release of lutein than β-carotene from CRF from fresh juice (40% and 8% respectively); interestingly heating appears to slightly reduce the release of lutein in the absence of oil. Adding oil increases lutein bioaccessibility, but only from CRF derived from heated biomass or juice, processes that, unlike β-carotene, appear not to impact lutein bioaccessibility (Fig. 4B). As discussed earlier, heat treatment can improve the bioaccessibility of carotenoids through disrupting the carotenoid-protein complex in chloroplasts. Thermal treatments (i.e. boiling, steaming, and grilling) have been reported to enhance the bioaccessibility of lutein in spinach and courgette.7,10,42 Our results may appear inconsistent with those studies, as it seems that thermal treatment, did not improve the bioaccessibility of lutein in PVH and HJ CRF (Fig. 4B). Thermal treatment is credited with promoting cell wall weakening (reducing a bioencapsulation barrier) as well as increasing the release of carotenoids from complexes; given that our material is effectively chloroplasts liberated from their cells, there is no cell wall barrier to overcome, so it is perhaps not surprising that heating our biomass or juice does not have such a dramatic effect on lutein results. In several studies lutein appears to move more easily from a food matrix to micelles compared with carotenes.7,14,21,43,44 This is in part due to its more polar nature, but it also suggests it is less tightly held in the light harvesting antenna complex in thylakoid membranes than β-carotene is in the reaction centre complex.44 The fact that β-carotene micellization is increased on heat treatment of biomass or juice (Fig. 4A) suggests that heat has disentangled, at least to a degree, the reaction centre complex. The % bioaccessibility of α-tocopherol from CRF material (Fig. 4C), like that of β-carotene, does seem to increase on heating and is further enhanced by the addition of oil. It appears that heating PVH prior to juicing promotes the release on digestion of β-carotene and α-tocopherol. Studies on the bioavailability of various pharmaceutical formulations of vitamin E have shown that the plasma concentration of vitamin E can be increased following the oral administration of vitamin E in the form of a self-emulsifying preparation compared with a bulk oil formulation.45 In addition, it has been reported that the amount of vitamin E solubilised within the micelle phase depends on carrier type of oil and molecular form of vitamin E. Yang & McClements (2013) discovered that the bioaccessibility of vitamin E was around 17% when medium-chain triglyceride (TAG) was used as the carrier oil type, but around 39% when long-chain TAG was used.46
3.2.4. Nutrient available for uptake.
‘Nutrient available for uptake’ is a measure of the percentage of nutrient available for absorption by the body. It takes into account the stability of the nutrient during digestion and the liberation of the nutrient into micellar phase. This is to provide a better understanding on how much nutrient that is available for absorption by the body, relative to the initial concentration of the nutrient in the freeze-dried PVH CRF (see Fig. 5) and mg per g dry weight (mg per g, DW) (see Table 2). Given the question marks over whether certain lipophilic nutrients are lost during digestion or are simply less easily extracted, ‘nutrient available for uptake’ is a particularly helpful measurement in this work since the concentration values of the nutrients in the starting material and in the micellar phase are reliable. From Fig. 5D one can see that approximately 38–40% of the α-linolenic acid from the parent CRF material is recovered in the micellar phase (with a suggestion that heating the biomass increases this value to just over 40%); this was in the absence of oil. For all lipophilic micronutrients measured, β-carotene, lutein and α-tocopherol, a very similar trend is seen; heat treatment increases the amount of each lipophilic micronutrient in the micellar phase, and this is further improved, especially in the case of β-carotene, by the presence of oil, except in the case of lutein from HJ where the addition of oil did not seem to change the % of lutein available for uptake. The maximum % of β-carotene, lutein and α-tocopherol available for uptake was approximately 40%, 40% and 20% respectively.
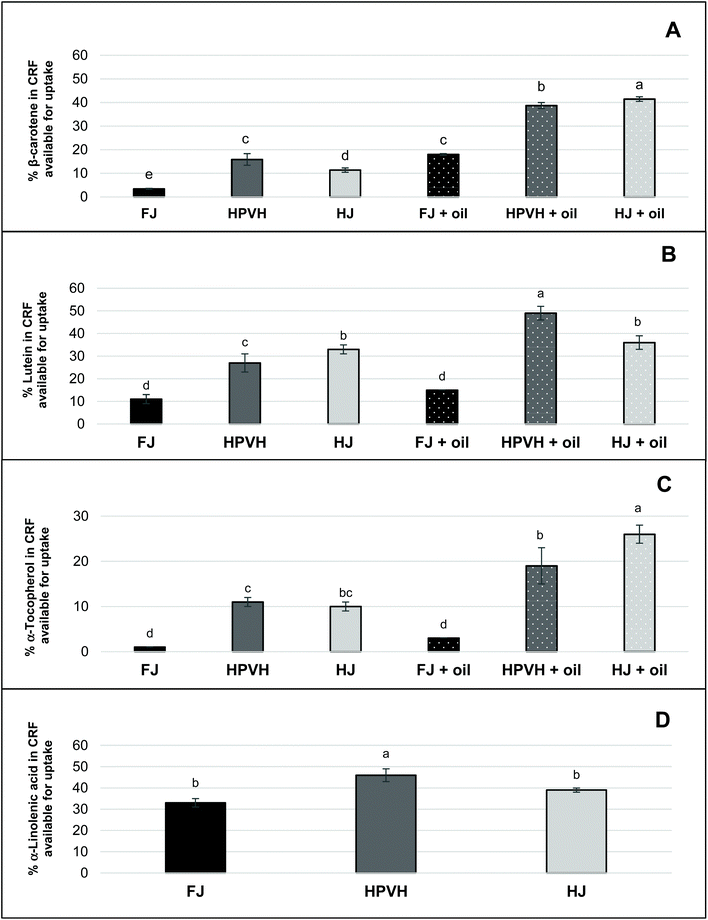 |
| Fig. 5 Effect of heat treatment and the presence of oil on nutrient in CRF available for uptake after in vitro digestion in CRF from PVH (A) β-carotene available for uptake after in vitro digestion (B) lutein (C) α-tocopherol (D) α-linolenic acid; FJ: fresh juice; HPVH: heated PVH; HJ: heated juice; data were presented as a mean ± SD of 3 separated in vitro digestion and analysed using post hoc analysis of variance (ANOVA) and according to a Tukey test with statistical significance at p < 0.0.5, a > b. | |
Table 2 Carotenoid, vitamin E and fatty acid contents of CRF from PVH both before and after digestion
|
β-Carotene |
Lutein |
α-Tocopherol |
α-Linolenic acid |
Pre-digestion |
Post-digestion |
Pre-digestion |
Post-digestion |
Pre-digestion |
Post-digestion |
Pre-digestion |
Post-digestion |
β-Carotene (mg per 100 g CRF, DW) |
β-Carotene in available for uptake (mg per 100 g CRF, DW) |
Lutein (mg per 100 g CRF, DW) |
Lutein in CRF available for uptake (mg per 100 g CRF, DW) |
α-Tocopherol (mg per 100 g CRF, DW) |
α-Tocopherol in CRF available for uptake (mg per 100 g CRF, DW) |
α-Linolenic acid (mg per 100 g CRF, DW) |
α-Linolenic acid in CRF available for uptake (mg per 100 g CRF, DW) |
FJ: fresh juice; HPVH: heated PVH; HJ: heated juice; data were presented as a mean ± SD of 3 separated in vitro digestion and analysed using post hoc analysis of variance (ANOVA) and according to a Tukey test with statistical significance at p < 0.05, a > b. DW means dry weight. |
FJ |
99 ± 6b |
4 ± 0.2e |
481 ± 29a |
51 ± 8c |
29 ± 2b |
0.4 ± 0d |
1670 ± 89 |
559 ± 40b |
FJ + oil |
|
18 ± 0.3c |
|
71 ± 2bc |
|
1 ± 0d |
|
|
HPVH |
74 ± 8c |
12 ± 1d |
334 ± 39b |
90 ± 13b |
29 ± 2b |
3 ± 0c |
1429 ± 206 |
660 ± 41a |
HPVH + oil |
|
29 ± 1b |
|
162 ± 10a |
|
6 ± 1b |
|
|
HJ |
121 ± 7a |
14 ± 1d |
462 ± 34a |
154 ± 10a |
45 ± 3a |
5 ± 1bc |
1489 ± 47 |
580 ± 12ab |
HJ + oil |
|
50 ± 1a |
|
167 ± 13a |
|
12 ± 1a |
|
|
4. Final discussion and conclusions
In the work presented here, the bioaccessibility of lipophilic nutrients β-carotene, lutein, α-tocopherol, and α-linolenic acid from a concentrated and freeze-dried chloroplast-rich fraction (CRF) has been measured for the first time. The authors are also not aware of pea vine haulm being used in any bioaccessibility studies before. Although pea vine haulm (PVH) and spinach are different, the carotenoids are located in chloroplasts, so our results from chloroplasts liberated/recovered from PVH can be placed next to published work on the impact of spinach leaf juicing/homogenisation on the bioaccessibility of lipophilic micronutrients.7,12,14,20,22 One must be very careful when comparing bioaccessibility results as small changes in food matrix preparation or in vitro digestion protocol can make a significant difference in the results.44 Broadly speaking there is agreement between these studies and our study: oil addition improved the bioaccessibilty of lipophilic micronutrients especially β-carotene. In the work by Nagao and co-workers (2013)12 spinach leaves were blanched for 2 minutes cooled, then homogenised (polytron) in water (2
:
1). Homogenates were stored at −80 °C until required. It is therefore possible that these homogenates contained released and perhaps broken chloroplasts. Fats and oils enhanced the bioaccessibility of beta carotene, but not of lutein or tocopherol. They also observed that TAG digestion products (FFA and MAGs/DAGs) enhance the bioaccessibility of β-carotene. In earlier work by Reboul and co-workers (2006)7 the in vitro digestion model was improved. Gastric pH was moved from 2 to 4, and intestinal pH moved from 7.5 to 6: these pH values better reflect the pH that most of a meal (vegetable-based in this case) would experience.47 The importance of pH on determining the movement of carotenoids from lipid droplets to micelles should not be overlooked.48 Overall, Reboul and co-workers7 demonstrated a good correlation of β-carotene, lutein and α-tocopherol bioaccessibility using this improved model with published results from feeding trials. They argue that no model is ideal; for example, although micellization of vitamin E may be low compared with carotenoids it is recognised that it is better absorbed compared with carotenoids. In their work they included data from feeding trials using carrots, peas, pumpkins, spinach, tomato, and watermelon to establish the veracity of their in vitro method to predict bioavailability of carotenoids which are enriched in plastids in these plant materials. Different types of subcellular plastids exist in plants: both chloroplasts and chromoplasts contain carotenoids, but these molecules are arranged in different plastid-specific substructures which are likely to affect the bioaccessibility of these pigments during digestion. Chloroplasts, ubiquitous in green photosynthetic tissue such as leaves, contain stacks of thylakoid membranes with the carotenoids woven into lipoprotein complexes that harness the energy from sunlight to make sugars. The profile of carotenoids in the chloroplasts of green leaf tissues is well conserved; lutein and β-carotene are the major pigments, followed by violaxanthin and neoxanthin. Britton et al. (2008)49 notes that β-carotene is in the reaction centre complexes, whereas the xanthophylls such as lutein are part of the light harvesting antenna complexes. Chromoplasts, found in fruits, roots, tubers, and flowers, synthesise and store in various substructures high concentrations of carotenoids. Most chromoplasts contain a range of substructures but based on the morphology of the predominant carotenoid-containing substructures chromoplasts can be split into four50 categories: globular, tubular, membranous and crystalloid. The Schweiggert and Carle review50 concludes that, at least in the raw state, the bioaccessibility of carotenoids increases in the order protein-carotenoid complex (thylakoids in the chloroplasts of green leaves), then crystalline chromoplasts (carrots and tomatoes), and then globular-tubular chromoplasts (papaya and mango). The reviewers claim that the deposition form of carotenoids is a decisive factor in determining the bioavailability of carotenoids from foods. Work by Palmero and co-workers (2013)51 also revealed that the degree of bioencapsulation of carotenoids has a significant impact on the bioaccessibility of β-carotene from carrots and tomatoes. Liberated chromoplasts release their carotenoids to a greater extent than cell-bound chromoplasts. We measured for the first time the release of α-linolenic acid from chloroplast thylakoid membranes during an in vitro measurement of lipophilic micronutrient bioaccessibility. Significant levels of α-linolenic acid were present in the micellar phase (60%). It is assumed this is mostly in the form of free fatty acids released from galactolipids and probably playing a direct role in the formation of micelles and the micellization of carotenoids from the same organelle. Further work is required to fully characterise the nature of α-linolenic acid in the micellar phase after the digestion of CRF material, and to what extent this fatty acid, or indeed the parent galactolipids, helps to micellise lipophilic micronutrients. The material properties of chloroplast-rich fractions (CRF) clearly affect the release of lipophilic nutrients within this model of human digestion. More work needs to be done to probe the impact of heat treatment of the biomass or juice on the physical nature of the derived CRF material. We assumed that releasing the chloroplasts from the confines of their cell environment would result in unhindered release of nutrients, but it appears that the CRF material we have generated has a certain recalcitrance that hinders, to some extent, the release of lipophilic micronutrients; this effect is eased on exposing the biomass or juice to heat prior to making the CRF material. It is likely that heating denatures native proteins which perhaps leads to a more porous CRF structure and the release of carotenoids from protein complexes. It is also possible that the inclusion of higher mechanical forces during digestion would better emulate human digestion and improve the release of nutrients. The static digestion model is also limited in terms of products of hydrolysis increasing in concentration in the micellar phase, thus slowing down digestion due to the principles of mass transfer. For future in vitro experiments we would recommend the inclusion of an impeller to mimic in-gut mechanic forces, the addition of gastric lipase (rabbit gastric lipase) as this has been shown to increase the bioaccessibility of carotenoids from spinach,15 and the in situ measurement of lipid hydrolysis, a key process for micellising lipophilic micronutrients.
5. Conclusions
A chloroplast-rich fraction (CRF) can be prepared from post-harvest field residue from pea vine cultivation (pea vine haulm – PVH) by a simple process of extrusion juicing, filtration, centrifugation, and freeze-drying of the pellet. The composition of this PVH CRF material is similar to that of spinach CRF material that we have prepared and analysed.1 The CRF material prepared from fresh and heat-treated PVH or PVH juice was ground into a powder and used in an in vitro human digestion model to measure the bioaccessibility of β-carotene, lutein, α-tocopherol and α-linolenic acid. The static digestion model used did not fully disperse the CRF powder from fresh biomass; this limitation was less pronounced when CRF from heat-treated biomass or juice was used. The addition of oil to the incubation mixture increased the bioaccessibility of most of analytes (data not available for α-linolenic) and may have improved the dispersion of CRF made from fresh PVH. Heating the biomass or juice appears to promote the liberation of more tightly bound lipophilic micronutrients (in this case β-carotene). Bioaccessibility measurements in this study suggest that significant amounts of lipophilic nutrients in CRF are released and made available for uptake into the body. Thus, liberating chloroplasts from their cell wall confines (reducing their degree of bioencapsulation) and concentrating them into a chloroplast-rich fraction (CRF) is an excellent way to prepare a natural, multi-nutrient material for humans.
Conflicts of interest
The authors declare that there are no conflicts of interest.
Acknowledgements
The PhD thesis of Miss Jutarat Wattanakul was supported by Thai Royal Government, Thailand. The PhD thesis of Moulay Sahaka was supported by Aix-Marseille University.
Notes and references
- M. A. Gedi, R. Briars, F. Yuseli, N. Zainol, R. Darwish, A. M. Salter and D. A. Gray, Component analysis of nutritionally rich chloroplasts: recovery from conventional and unconventional green plant species, J. Food Sci. Technol., 2017, 54, 2746–2757 CrossRef CAS PubMed.
- A. Torcello-Gomez, M. A. Gedi, R. Ibbett, K. Nawaz Husain, R. Briars and D. Gray, Chloroplast-rich material from the physical fractionation of pea vine (Pisum sativum) postharvest field residue (Haulm), Food Chem., 2019, 272, 18–25 CrossRef CAS PubMed.
- J. M. Carbonell-Capella, M. Buniowska, F. J. Barba, M. J. Esteve and A. Frígola, Analytical Methods for Determining Bioavailability and Bioaccessibility of Bioactive Compounds from Fruits and Vegetables: A Review, Compr. Rev. Food Sci. Food Saf., 2014, 13, 155–171 CrossRef CAS PubMed.
- M. Minekus, M. Alminger, P. Alvito, S. Ballance, T. Bohn, C. Bourlieu, F. Carriere, R. Boutrou, M. Corredig, D. Dupont, C. Dufour, L. Egger, M. Golding, S. Karakaya, B. Kirkhus, S. Le Feunteun, U. Lesmes, A. Macierzanka, A. Mackie, S. Marze, D. J. McClements, O. Menard, I. Recio, C. N. Santos, R. P. Singh, G. E. Vegarud, M. S. Wickham, W. Weitschies and A. Brodkorb, A standardised static in vitro digestion method suitable for food - an international consensus, Food Funct., 2014, 5, 1113–1124 RSC.
- A. Brodkorb, L. Egger, M. Alminger, P. Alvito, R. Assuncao, S. Ballance, T. Bohn, C. Bourlieu-Lacanal, R. Boutrou, F. Carriere, A. Clemente, M. Corredig, D. Dupont, C. Dufour, C. Edwards, M. Golding, S. Karakaya, B. Kirkhus, S. Le Feunteun, U. Lesmes, A. Macierzanka, A. R. Mackie, C. Martins, S. Marze, D. J. McClements, O. Menard, M. Minekus, R. Portmann, C. N. Santos, I. Souchon, R. P. Singh, G. E. Vegarud, M. S. J. Wickham, W. Weitschies and I. Recio, INFOGEST static in vitro simulation of gastrointestinal food digestion, Nat. Protoc., 2019, 14, 991–1014 CrossRef CAS PubMed.
- M. J. Brown, M. G. Ferruzzi, M. L. Nguyen, D. A. Cooper, A. L. Eldridge, S. J. Schwartz and W. S. White, Carotenoid bioavailability is higher from salads ingested with full-fat than with fat-reduced salad dressings as measured with electrochemical detection, Am. J. Clin. Nutr., 2004, 80, 396–403 CrossRef CAS PubMed.
- E. Reboul, M. Richelle, E. Perrot, C. Desmoulins-Malezet, V. Pirisi and P. Borel, Bioaccessibility of carotenoids and vitamin E from their main dietary sources, J. Agric. Food Chem., 2006, 54, 8749–8755 CrossRef CAS PubMed.
- J. Francisco, A. Horta, R. Pedrosa, C. Afonso, C. Cardoso, N. M. Bandarra and M. M. Gil, Bioaccessibility of Antioxidants and Fatty Acids from Fucus Spiralis, Foods, 2020, 9, 440 CrossRef CAS PubMed.
- C. L. Rock, J. L. Lovalvo, C. Emenhiser, M. T. Ruffin, S. W. Flatt and S. J. Schwartz, Bioavailability of beta-carotene is lower in raw than in processed carrots and spinach in women, J. Nutr., 1998, 128, 913–916 CrossRef CAS PubMed.
- L. Ryan, O. O'Connell, L. O'Sullivan, S. A. Aherne and N. M. O'Brien, Micellarisation of carotenoids from raw and cooked vegetables, Plant
Foods Hum. Nutr., 2008, 63, 127–133 CrossRef CAS PubMed.
- G. A. Tumuhimbise, A. Namutebi and J. H. Muyonga, Microstructure and in vitro beta carotene bioaccessibility of heat processed orange fleshed sweet potato, Plant Foods Hum. Nutr., 2009, 64, 312–318 CrossRef CAS PubMed.
- A. Nagao, E. Kotake-Nara and M. Hase, Effects of fats and oils on the bioaccessibility of carotenoids and vitamin E in vegetables, Biosci., Biotechnol., Biochem., 2013, 77, 1055–1060 CrossRef CAS PubMed.
- D. Hornero-Méndez and M. I. Mínguez-Mosquera, Bioaccessibility of carotenes from carrots: Effect of cooking and addition of oil, Innovative Food Sci. Emerging Technol., 2007, 8, 407–412 CrossRef.
- G. T. Rich, A. L. Bailey, R. M. Faulks, M. L. Parker, M. S. Wickham and A. Fillery-Travis, Solubilization of carotenoids from carrot juice and spinach in lipid phases: I. Modeling the gastric lumen, Lipids, 2003, 38, 933–945 CrossRef CAS PubMed.
- M. Iddir, J. F. Porras Yaruro, Y. Larondelle and T. Bohn, Gastric lipase can significantly increase lipolysis and carotenoid bioaccessibility from plant food matrices in the harmonized INFOGEST static in vitro digestion model, Food Funct., 2021, 12, 9043–9053 RSC.
- J. Wattanakul, M. Sahaka, S. Amara, S. Mansor, B. Gontero, F. Carrière and D. Gray, In vitro digestion of galactolipids from chloroplast-rich fraction (CRF) of postharvest, pea vine field residue (haulm) and spinach leaves, Food Funct., 2019, 10, 7806–7817 RSC.
- M. A. Gedi, K. J. Magee, R. Darwish, P. Eakpetch, I. Young and D. A. Gray, Impact of the partial replacement of fish meal with a chloroplast rich fraction on the growth and selected nutrient profile of zebrafish (Danio rerio), Food Funct., 2019, 10, 733–745 RSC.
- J. Wattanakul, M. Syamila, R. Briars, C. Ayed, R. Price, R. Darwish, M. A. Gedi and D. A. Gray, Effect of steam sterilisation on lipophilic nutrient stability in a chloroplast-rich fraction (CRF) recovered from postharvest, pea vine field residue (haulm), Food Chem., 2021, 334, 127589 CrossRef CAS PubMed.
-
J. J. M. Castenmiller, Ph.D., Wageningen University, 2000.
- M. G. Ferruzzi, M. L. Failla and S. J. Schwartz, Assessment of degradation and intestinal cell uptake of carotenoids and chlorophyll derivatives from spinach puree using an in vitro digestion and Caco-2 human cell model, J. Agric. Food Chem., 2001, 49, 2082–2089 CrossRef CAS PubMed.
- C. Chitchumroonchokchai, S. J. Schwartz and M. L. Failla, Assessment of lutein bioavailability from meals and a supplement using simulated digestion and caco-2 human intestinal cells, J. Nutr., 2004, 134, 2280–2286 CrossRef CAS PubMed.
- M. Hayes, S. Corbin, C. Nunn, M. Pottorff, C. D. Kay, M. A. Lila, M. Iorrizo and M. G. Ferruzzi, Influence of simulated food and oral processing on carotenoid and chlorophyll in vitro bioaccessibility among six spinach genotypes, Food Funct., 2021, 12, 7001–7016 RSC.
- V. Gökmen, K. Savaş Bahçeci, A. Serpen and J. Acar, Study of lipoxygenase and peroxidase as blanching indicator enzymes in peas: change of enzyme activity, ascorbic acid and chlorophylls during frozen storage, LWT – Food Sci. Technol., 2005, 38, 903–908 CrossRef.
- J. Folch, M. Lees and G. H. Sloane Stanley, A simple method for the isolation and purification of total lipides from animal tissues, J. Biol. Chem., 1957, 226, 497–509 CrossRef CAS.
-
J. M. C. Danku, B. Lahner, E. Yakubova and D. E. Salt, Large-scale plant ionomics, in Methods in Molecular Biology, ed. F. J. M. Maathuis, Humana Press, New York, US, 2013 Search PubMed.
- H. K. Lichtenthaler and C. Buschmann, Chlorophylls and Carotenoids: Measurement and Characterization by UV-VIS Spectroscopy, Curr. Protoc. Food Anal. Chem., 2001, 1, F4.3.1–F4.3.8 CrossRef.
- B. Gontero, G. Mulliert, M. Rault, M. T. Giudici-Orticoni and J. Ricard, Structural and functional properties of a multi-enzyme complex from spinach chloroplasts. 2. Modulation of the kinetic properties of enzymes in the aggregated state, Eur. J. Biochem., 1993, 217, 1075–1082 CrossRef CAS PubMed.
- S. J. Schwartz and J. H. Von Elbe, Kinetics of Chlorophyll Degradation to Pyropheophytin in Vegetables, J. Food Sci., 1983, 48, 1303–1306 CrossRef CAS.
- B. B. Fischer, É. Hideg and A. Krieger-Liszkay, Production, Detection, and Signaling of Singlet Oxygen in Photosynthetic Organisms, Antioxid. Redox Signaling, 2013, 18, 2145–2162 CrossRef CAS PubMed.
- A. L. Petrou, P. L. Petrou, T. Ntanos and A. Liapis, A Possible Role for Singlet Oxygen in the Degradation of Various Antioxidants. A Meta-Analysis and Review of Literature Data, Antioxidants, 2018, 7, 35 CrossRef PubMed.
- S. Lee, Y. Choi, H. S. Jeong, J. Lee and J. Sung, Effect of different cooking methods on the content of vitamins and true retention in selected vegetables, Food Sci. Biotechnol., 2018, 27, 333–342 CAS.
- L. A. Chandler and S. J. Schwartz, Isomerization and losses of trans-.beta.-carotene in sweet potatoes as affected by processing treatments, J. Agric. Food Chem., 1988, 36, 129–133 CrossRef CAS.
- M. G. Ferruzzi, J. L. Lumpkin, S. J. Schwartz and M. Failla, Digestive Stability, micellarization, and uptake of beta-carotene isomers by Caco-2 human intestinal cells, J. Agric. Food Chem., 2006, 54, 2780–2785 CrossRef CAS PubMed.
- E. Hedrén, V. Diaz and U. Svanberg, Estimation of carotenoid accessibility from carrots determined by an in vitro digestion method, Eur. J. Clin. Nutr., 2002, 56, 425–430 CrossRef PubMed.
- N. Koca, H. S. Burdurlu and F. Karadeniz, Kinetics of colour changes in dehydrated carrots, J. Food Eng., 2007, 78, 449–455 CrossRef CAS.
-
D. B. Rodriguez-Amaya, Food Carotenoids: Chemistry, Biology, and Technology, John Wiley & Sons, Ltd, West Sussex, UK, 2016 Search PubMed.
- A. Salhi, S. Amara, P. Mansuelle, R. Puppo, R. Lebrun, B. Gontero, A. Aloulou and F. Carriere, Characterization of all the lipolytic activities in pancreatin and comparison with porcine and human pancreatic juices, Biochimie, 2020, 169, 106–120 CrossRef CAS PubMed.
- L. Ohlsson, A. Rosenquist, J. F. Rehfeld and M. Härröd, Postprandial effects on plasma lipids and satiety hormones from intake of liposomes made from fractionated oat oil: two randomized crossover studies, Food Nutr. Res., 2014, 58 DOI:10.3402/fnr.v58.24465.
- G. Mulokozi, E. Hedrén and U. Svanberg, In vitro accessibility and intake of beta-carotene from cooked green leafy vegetables and their estimated contribution to vitamin A requirements, Plant Foods Hum. Nutr., 2004, 59, 1–9 CrossRef CAS PubMed.
- F. Carriere, Impact of gastrointestinal lipolysis on oral lipid-based formulations and bioavailability of lipophilic drugs, Biochimie, 2016, 125, 297–305 CrossRef CAS PubMed.
- P. Borel, P. Grolier, M. Armand, A. Partier, H. Lafont, D. Lairon and V. Azais-Braesco, Carotenoids in biological emulsions: solubility, surface-to-core distribution, and release from lipid droplets, J. Lipid Res., 1996, 37, 250–261 CrossRef CAS.
- J. Courraud, J. Berger, J. P. Cristol and S. Avallone, Stability and bioaccessibility of different forms of carotenoids and vitamin A during in vitro digestion, Food Chem., 2013, 136, 871–877 CrossRef CAS PubMed.
- M. Margier, C. Buffière, P. Goupy, D. Remond, C. Halimi, C. Caris-Veyrat, P. Borel and E. Reboul, Opposite Effects of the Spinach Food Matrix on Lutein Bioaccessibility and Intestinal Uptake Lead to Unchanged Bioavailability Compared to Pure Lutein, Mol. Nutr. Food Res., 2018, 62, 1800185 CrossRef PubMed.
- T. Chacón-Ordóñez, R. Carle and R. Schweiggert, Bioaccessibility of carotenoids from plant and animal foods, J. Sci. Food Agric., 2019, 99, 3220–3239 CrossRef PubMed.
- T. Julianto, K. H. Yuen and A. M. Noor, Improved bioavailability of vitamin E with a self emulsifying formulation, Int. J. Pharm., 2000, 200, 53–57 CrossRef CAS PubMed.
- Y. Yang and D. J. McClements, Vitamin E bioaccessibility: influence of carrier
oil type on digestion and release of emulsified alpha-tocopherol acetate, Food Chem., 2013, 141, 473–481 CrossRef CAS PubMed.
- V. Tyssandier, E. Reboul, J. F. Dumas, C. Bouteloup-Demange, M. Armand, J. Marcand, M. Sallas and P. Borel, Processing of vegetable-borne carotenoids in the human stomach and duodenum, Am. J. Physiol.: Gastrointest. Liver Physiol., 2003, 284, G913–G923 CrossRef CAS PubMed.
- V. Tyssandier, B. Lyan and P. Borel, Main factors governing the transfer of carotenoids from emulsion lipid droplets to micelles, Biochim. Biophys. Acta, Mol. Cell Biol. Lipids, 2001, 1533, 285–292 CrossRef CAS.
-
G. Britton, Functions of Intact Carotenoids, Birkhäuser Verlag Basel, 2008, 4, 189–212 Search PubMed.
- R. M. Schweiggert and R. Carle, Carotenoid deposition in plant and animal foods and its impact on bioavailability, Crit. Rev. Food Sci. Nutr., 2017, 57, 1807–1830 CAS.
- P. Palmero, L. Lemmens, A. Ribas-Agustí, C. Sosa, K. Met, J. de Dieu Umutoni, M. Hendrickx and A. Van Loey, Novel targeted approach to better understand how natural structural barriers govern carotenoid in vitro bioaccessibility in vegetable-based systems, Food Chem., 2013, 141, 2036–2043 CrossRef CAS PubMed.
|
This journal is © The Royal Society of Chemistry 2022 |