DOI:
10.1039/D2FO00165A
(Paper)
Food Funct., 2022,
13, 5240-5251
Lactobacillus paracasei PS23 improves cognitive deficits via modulating the hippocampal gene expression and the gut microbiota in D-galactose-induced aging mice†
Received
15th January 2022
, Accepted 24th March 2022
First published on 31st March 2022
Abstract
Probiotic supplements are potential therapeutic agents for age-related cognitive deficits. A prior study showed that probiotic Lactobacillus paracasei PS23 (PS23) supplementation delayed age-related cognitive decline in mice. However, the underlying mechanisms remain unclear. This study aimed to investigate the effects of live or heat-killed PS23 (HK-PS23) on cognitive function in D-galactose (D-gal)-induced aging mice and explore the underlying mechanisms. We designed four groups of mice: control, D-gal aging mice, and PS23 supplemented and HK-PS23 supplemented D-gal aging mice. We evaluated memory function and anxiety using Morris water maze and open field tests, respectively. Neural monoamines and activities of superoxide dismutase (SOD) in the hippocampus were evaluated. RNA-seq was used to evaluate hippocampal gene expression profiles in each group, and the composition of the gut microbiota was analyzed. We revealed that PS23 and HK-PS23 supplementation ameliorated D-gal-induced memory deficits and improved motor and anxiety-behaviors in aging mice. In the hippocampus, serotonin levels (5-HT) were increased and the genes involved in neuroplasticity, anti-inflammatory, and antioxidant functions were upregulated in PS23 and HK-PS23 supplemented groups. The gut microbiota showed specific changes. Our results suggest that PS23 and HK-PS23 supplements could ameliorate age-related cognitive decline, possibly by upregulating the genes involved in synaptic plasticity and preventing oxidation and inflammation.
1 Introduction
The global population is approaching an important demographic milestone; elderly individuals will soon outnumber young children. According to the World Health Organization (WHO), by 2030, one in six people will be aged 60 years or above (https://www.who.int/news-room/fact-sheets/detail/ageing-and-health). To face this inevitable population change, the United Nations General Assembly declared 2021–2030 the Decade of Healthy Ageing. As both the proportion of older people and the length of life increase worldwide, the key questions are whether older people can enjoy a longer period of good health, a sustained sense of well-being, and extended periods of social engagement and productivity. Therefore, measures to reduce ageing related cognitive decline and mood disorder will contribute tremendously to ensuring improved health and wellbeing in the elderly population.
Probiotics are health-promoting microorganisms that are generally considered extremely safe and have no serious adverse effects if consumed in adequate amounts. There are many types of probiotics; one of these, termed psychobiotics can affect the central nervous system by modulating the microbiota–gut–brain axis.1 Several preclinical studies have demonstrated that the probiotics Bifidobacterium infantis and Lactobacillus plantarum PS128 could reduce depression-like behaviors in mice in a maternal separation depression animal model.2,3Lactobacillus rhamnosus can further reduce stress-induced anxiety- and depression-like behaviors in mice.4 Similarly, Bifidobacterium longum 1714 and Lactobacillus helveticus NS8 improved the cognitive functions of anxious BALB/C mice and chronically stressed rats, respectively.5,6 There have also been clinical trials examining the effects of probiotics on improving the cognitive functions in elderly people with mild cognitive impairment and patients with Alzheimer's disease.7–11 The majority of the trial outcomes suggested improvements in cognitive functions; however, one clinical trial indicated that probiotic treatment had no significant effect on patients with severe Alzheimer's disease.12 Nonetheless, psychobiotics show great potential in alleviating emotional and cognitive deficits in the aging population.
Aging studies are time consuming and costly; therefore, we used a D-gal-induced aging mouse model in this study. D-Gal is an aldohexose that exists naturally in the body and brain. However, excessive levels of D-gal beyond the natural concentration can trigger oxidative stress, apoptosis, and inflammation in several organs, including the brain.13–15 Because D-gal induces pathological changes that resemble natural aging in animals, it is frequently used as an aging model to study age-related deficits.13,15 In particular, it has been demonstrated that D-galactose administration in rodents could induce brain aging phenotypically similar to human brain aging, including memory deficits, neuronal degeneration, increased oxidative stress, mitochondrial DNA mutation, and decreased ATP production.14,16–19
Lactobacillus paracasei PS23 (PS23) is a probiotic isolated from healthy human feces. Animal studies have demonstrated that PS23 administration could reduce anxiety- and depression-like behaviors in mice exposed to early life stress by maternal separation,20 as well as in mice exposed to chronic corticosterone injection.21 In addition, PS23 treatment ameliorated early life stress-induced elevations of serum proinflammatory cytokines and the reduction of anti-inflammatory cytokines.20 PS23 treatment also improved chronic corticosterone exposure induced reduction in brain-derived neurotrophic factor (BDNF) in the hippocampus.21 Furthermore, in our previous work, administration of PS23 could ameliorate muscle aging in D-gal-induced aging mice.22 Previously, a study involved the use of senescence-accelerated mouse prone 8 (SAMP8) mice to study the effects of probiotic PS23 supplementation on age-related deficits. The results demonstrated that PS23 administration could lower the scores of senescence, improve the gastrointestinal functions, and attenuate age-related sacropenia.23–25 PS23 could also reduce anxiety-like behaviors and memory impairment in SAMP8 mice.23 Furthermore, serum proinflammatory cytokines were reduced in PS23 treated SAMP8 mice, whereas serum anti-inflammatory cytokines and BDNF were increased.23 In addition, the levels of anti-oxidative enzymes superoxide dismutase (SOD) and glutathione peroxidase (GPx) were increased in both the serum and hippocampus of PS23-treated SAMP8 mice.23 However, the cellular and molecular mechanisms underlying PS23 mediated cognitive enhancement in aged mice remain elusive. In the present study, we supplemented the mice with PS23 for one week and then started the D-gal treatment for 4 weeks. We investigated the effects of PS23 on age-related cognitive decline and anxiety-like behaviors. More importantly, we used RNA sequencing (RNA-seq) to investigate the mechanisms underlying the mediation of the beneficial effects of PS23.
2 Materials and methods
2.1 Experimental animals and L. paracasei PS23
2.1.1 Animal and experimental design.
Eight-week-old male C57BL/6J mice were purchased from the National Laboratory Animal Center (NLAC, Taipei, Taiwan). Mice were housed in a controlled environment under ambient conditions of 22 °C, 50–60% humidity, and a 12 h light/dark cycle with light from 6:00 AM to 6:00 PM. Food and water were provided ad libitum. All procedures involving animal care and handling were approved by the Institutional Animal Care and Use Committee of the National Yang Ming Chiao Tung University (IACUC-1070916).
The mice were randomly assigned to one of the following four groups (6 mice per group): non-aging, aging, aging PS23, and aging HK-PS23 groups (Fig. 1). The aging mice in the PS23 and HK-PS23 groups were administered with PS23 (109 CFU per day per mice) and HK-PS23 (109 cells per day per mice) daily by oral gavage from weeks 0 to 9, respectively. The non-aging and aging groups were administered with 200 μL of PBS daily via oral gavage from weeks 0 to 9. The aging, aging PS23, and aging HK-PS23 groups were subcutaneously injected with D-galactose (100 mg kg−1 day−1) once a day from weeks 1 to 9. All behavioral tests were conducted between weeks 8 and 9. The mice were sacrificed after behavioral tests at week 9, and tissue samples were harvested for further analyses.
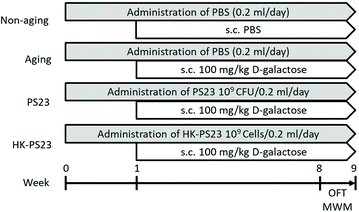 |
| Fig. 1 The experimental grouping and design. Mice were administered by oral gavage with PBS (non-aging and aging groups) or 109 CFU per mice of live PS23 or HK-PS23 (PS23 or HK-PS23 groups, respectively) once a day from week 0 to 9. D-Galactose (100 mg kg−1) was injected subcutaneously (s.c.) once a day from weeks 1 to 9 in all groups except the non-aging group. The behavioral tests such as the open field test (OFT) and Morris water maze test (MWM) were performed between weeks 8 and 9. | |
2.1.2 Preparation of L. paracasei PS23.
PS23 and HK-PS23 preparations were prepared based on the previous report.22 PS23 was inoculated in Man Rogosa Sharpe (MRS) broth (Difco Corp., MD, USA) and then cultured at 37 °C for 18 h. The PS23 culture was harvested by centrifugation at 6000g for 10 min, after which the PS23 pellet was resuspended in MRS broth supplemented with 12.5% glycerol to obtain a final concentration of 1010 colony-forming units per millilitre (CFU mL−1). The resuspended solution was then aliquoted in freezer tubes and stored at −80 °C. Before oral administration, aliquots of PS23 were thawed in a water bath maintained at 37 °C for 1 h and then centrifuged at 6000g for 10 min. The supernatant was removed, and the bacterial pellet was resuspended in phosphate-buffered saline (PBS). For the HK-PS23 preparation, the concentration of PS23 was adjusted to 1010 CFU mL−1 in PBS, heat-treated at 80 °C for 30 min in a water bath, and stored at −20 °C until use.
2.2 Behavioral tests
2.2.1 Morris water maze.
A Morris water maze (MWM) test was performed according to a previously published protocol [42]. The water maze comprised a circular pool (120 cm in diameter and 50 cm in height) containing water (22 °C) filled to a depth of 40 cm. The mice were trained to locate a transparent platform below the surface of the water five times per day for five days. Each day, the mice entered the pool at the north, south, east, and west positions once each, while the fifth entrance position was randomly chosen from the 4 directions. If the mouse did not find the platform within 60 s, the mouse was placed on the platform and allowed to stay there for 20 s to learn the location of the platform. On the sixth day, the platform was removed, and the mice were given 60 s to search for the maze. The swimming path was recorded by a ceiling-mounted video camera and analyzed using the Etho-Vision XT video tracking software (Noldus, Wageningen, the Netherlands). The swimming time in each quadrant was quantified. The time spent in the correct quadrant previously containing the platform and the quadrant opposite to the correct quadrant were presented.
2.2.2 Open field test.
The OFT procedure was modified from the previous report.21 Briefly, each mouse was placed in an open field arena (25.4 × 25.4 × 38 cm) for 10 min. The time spent in the center (12.5 × 12.5 cm), the number of entries with all four paws in the center, and the total travel distance were recorded and analyzed using the TruScan software (Version 2.2; Coulbourn Instruments, Allentown, PA, USA). To minimize odor interference, the chamber was cleaned with 70% (v/v) ethanol after each run.
2.3 Quantification of the antioxidant
Superoxide dismutase (SOD) activity was evaluated in the hippocampal samples using SOD assay kits (Cayman Chemicals Inc., Ann Arbor, MI, USA), following the manufacturer's instructions. SOD activity was calculated using the equation obtained from the linear regression of the standard curve.
2.4 Quantification of monoamines and metabolites
The detection of monoamines and metabolites was performed with liquid chromatography – electrochemical detection (HPLC–ECD) according to the previously published protocol.43 The hippocampus was lysed by sonication in perchloric acid buffer (0.1% perchloric acid, 0.1 mM ethylenediaminetetraacetic acid [EDTA], and 0.1 mM Na2S2O5). The samples were centrifuged at 12
000g for 10 min, and the supernatants were collected and filtered through a 0.22 mm polyvinylidene difluoride (PVDF) membrane (4 mm syringe filter; Millex-GV, Millipore, Burlington, MA, USA). The HPLC-ECD system comprised an S1130 HPLC pump system (Sykam, Eresing, Germany), an online S5300 sample injector (Sykam, Eresing, Germany), a DECADE II SDC electrochemical detector (Antec, Zoeterwoude, the Netherlands), and a reversed-phase column (Kinetex C18, 2.6 μm, 100 × 2.1 mm I.D.; Phenomenex, CA, USA). The potential was set at +700 mV for the glassy carbon working electrode of an Ag/AgCl reference electrode at room temperature (25 °C). In order to quantify dopamine (DA), 3,4-dihydroxyphenylacetic acid (DOPAC), homovanillic acid (HVA), 5-hydroxyindoleacetic acid (5-HIAA), and 5-HT, the mobile phase was pumped at a constant flow rate of 0.2 mL min−1. The mobile phase buffer contained 0.1 M NaH2PO4, 2 mM KCl, 0.74 mM 1-octanesulfonic acid (SOS, sodium salt), 0.03 mM (EDTA), and 8% methanol, and was adjusted to pH 3.74 H3PO4. Diluted filtrates (20 μL) were injected into a chromatographic system, which had been prepared at a suitable mobile phase and analysis state. Monoamine and metabolite concentrations were interpolated from standard curves obtained from sample standards (Sigma-Aldrich Corp., Saint Louis, MO, USA) ranging from 1 to 100 ng mL−1 using the DataApex Clarity chromatography software (version 5.0.3.180, DataApex Ltd, Prague, Czech Republic).
2.5 RNA-seq
Total RNA was extracted from hippocampal samples using the RNeasy kit (QI-AGEN, Germanton, MD, USA), and gene expression analysis was performed using Illumina Novaseq 150PE protocols and pipelines of RNA-seq (Illumina, Inc., San Diego, CA, USA). The RNA-seq library was prepared for sequencing using standard Illumina protocols. After quality control, clean reads were analyzed for the quantification of read numbers mapped to each gene using HTSeq v0.6.1. Differentially expressed genes (DEGs) were defined as a fold change greater than 2 and q-value (fdr, padj) less than 0.05.
2.6 DNA extraction of fecal samples
On the day of fecal sample collection, all mice were placed individually in empty autoclaved cages and allowed to defecate freely. Immediately after defecation, feces were collected in sterile tubes on ice and stored at −80 °C before use. Bacterial DNA was extracted from fecal samples and purified using previously described methods with slight modifications.43 Fecal samples (100 mg) were washed three times with PBS and resuspended in extraction buffer (200 mM Tris–HCl, 80 mM EDTA, 2% SDS; pH 8.0). Glass beads (0.1 mm in diameter, 300 mg) and buffer-saturated phenol (500 μL) were added, and the mixture was vortexed vigorously for 30 s using a FastPrep FP 120 homogenizer (Q-Biogene, Carlsbad, CA, USA) at 5.0 m s−1. The samples were then centrifuged at 12
000g for 5 min at 4 °C, and the supernatant (400 μL) was collected. Subsequently, phenol–chloroform extractions were performed, and 250 μL of the supernatant was precipitated with 3 M sodium acetate (pH 5.2) and isopropanol, and air-dried. The DNA was dissolved in 80 μL of TE buffer (10 mM Tris–HCl, 1 mM EDTA, pH 8.0) and stored at −80 °C.
2.7 Quantitative RT-PCR analysis
The quantitative RT-PCR assay was performed as previously described.22 One μg of RNA was transcribed into cDNA by using a RevertAid First Strand cDNA Synthesis Kit (Thermo Fisher Scientific Inc., Waltham, MA, USA) according to the manufacturer's instructions, and the cDNA samples were stored at −80 °C. The target genes were determined using the following primers: Mouse Lcn2: F 5′-CAGAAGGCAGCTTTACGATG-3′ and R 5′-CCTGGAGCTTGGAACAAATG-3′; Mouse Lao1: F 5′-TGGCCAAGAAGAGTGGAATC-3′ and R 5′-AGCTCCCACTACCACCACAC-3′; Mouse Plin4: F 5′-TTGGTAGCCATGAGATGCGG-3′ and R 5′-TCACTACCTGCCTCCGAAGA-3′; and Mouse beta-actin: F 5′-CGG TTC CGA TGC CCT GAG GCT CTT-3′ and R 5′-CGT CAC ACT TCA TGA TGG AAT TGA-3′. PCR was performed under the following conditions: one cycle of 95 °C for 3 min, 40 cycles of 95 °C for 3 s, and 60 °C for 30 s. Data were determined by the 2−ΔCt method.
2.8 Microbiota analysis
DNA amplicons from individual fecal samples were prepared according to the Illumina protocol. The V3–V4 regions of the 16S rRNA gene were amplified using a bacterial universal primer set (341F and 805R) by PCR. The PCR products were purified using the QIAquick Gel Extraction Kit (QIAGEN, Germantown, MD, USA). The 1st stage amplicons were then purified using an AMPure XP system (Beckman Coulter Inc., Brea, CA, USA), followed by the Nextera XT Index Kit v2 Set (Illumina, Inc., San Diego, CA, USA). The library quality was assessed using a Qubit 2.0 Fluorometer (Thermo Scientific, Waltham, MA) and a Fragment Analyzer 5200 system (Agilent Technologies, Santa Clara, CA, USA). The qualified libraries were then sequenced on an Illumina MiSeq platform with 300 bp paired-end reads generated by Genomics, BioSci & Tech Co (New Taipei City, Taiwan). The sequences were clustered into operational taxonomic units (OTUs) with 97% identity. The QIIME software (Version 1.9.0) and R software (Version 3.4.1) were used to analyze the α-diversity and β-diversity. Linear discriminant analysis effect size (LEfSE) was used to identify potential microbial biomarkers associated with the interventions. An effect size threshold of >2 is shown.
2.9 Statistical analysis
Data are presented as the mean ± standard error of the mean. Differences between the groups were analyzed using one-way analysis of variance with Tukey's post-hoc test. The statistical software used was GraphPad Prism 8 (GraphPad Prism, La Jolla, CA, USA). The association results were assessed using robust regression. The results with a two-tailed significance of P < 0.05 were considered statistically significant.
3 Results
3.1 PS23 supplementation improves the cognitive function of aging mice
The Morris water maze test was conducted to examine the cognitive functions of D-gal-induced aging mice and the effects of PS23 supplementation. After 5 days of training, the mice were subjected to the probe test 24 h later. Compared to the non-aging mice, D-gal-treated aging mice spent less time in the correct quadrant previously containing the hidden platform, suggesting that they exhibited impaired spatial memory (Fig. 2, non-aging: 26.6 ± 0.8 s, aging: 14.5 ± 2.2 s). In contrast, the PS23 and HK-PS23 treated D-gal mice spent significantly more time in the correct quadrant than the D-gal mice, and the time was not different from that of the non-aging mice (Fig. 2. PS23: 29.5 ± 1.3 s, HK-PS23: 30.6 ± 1.7 s). These results indicate that PS23 and HK-PS23 supplementation ameliorated D-gal-induced aging impairment in spatial memory.
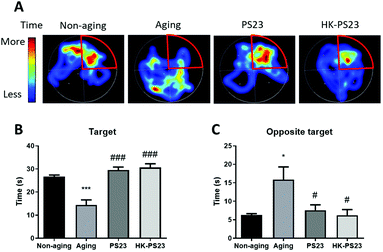 |
| Fig. 2 The learning and memory effects of PS23 on D-gal induced aging mice by a Morris water maze test. (A) Representative heat map images of the swim path of the mice from each experimental group, the target quadrant is highlighted in red. (B) Time spent in the target quadrant. (C) Time spent in the quadrant opposite to the target. N = 6, *p < 0.05; ***p < 0.001, compared with the non-aging group. #p < 0.05; ###p < 0.001, compared with the aging group. Data are presented as mean ± SEM. | |
3.2
L. paracasei PS23 reduces anxiety-like behavior
We further examined anxiety-like behaviors using an open field test. In the open field arena, the total distance travelled by the D-gal aged mice was lower than non-aged mice, although the difference was not significant (Fig. 3A, non-aging: 268.7 ± 15.3 cm, aging: 190.1 ± 24.0 cm). However, the PS23 and HK-PS23 supplemented aged mice both travelled significantly longer distances than the aged mice (Fig. 3A, PS23: 279.3 ± 19.7 cm, HK-PS23: 318.4 ± 25.8 cm). The time spent in the center area in the aged mice was not significantly different from that of the non-aged mice and PS23-treated aged mice, but displayed a trend of reduction (Fig. 3B, non-aging: 29.8 ± 9.0 s, aging: 14.4 ± 4.6 s, PS23: 35.7 ± 6.3 s). Only the HK-PS23 supplemented aged mice spent significantly more time in the central area than aged mice (Fig. 3B, HK-PS23: 38.2 ± 3.3 s). Furthermore, the HK-PS23 supplemented aged mice also displayed a significantly higher number of center entries than both the non-aged and aged mice (Fig. 3C, non-aging: 32.7 ± 1.6 s, aging: 30.9 ± 3.3 s, PS23: 41.1 ± 3.1 s, HK-PS23: 46.2 ± 3.4 s). Evidently, PS23 and HK-PS23 supplementation increased the activity of the aged mice. Furthermore, HK-PS23 supplementation exhibited more anxiolytic effects than PS23 supplementation.
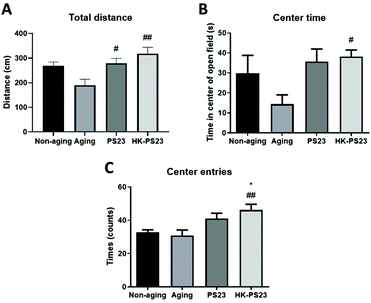 |
| Fig. 3 PS23 and HK-PS23 supplementation improves the locomotion and anxiety-like behaviors of aging mice in an open field test. (A) Total distance travelled in the open field. (B) Time spent in the center area. (C) Number of entries into the center area. N = 6, *p < 0.05, compared with the non-aging group. #p < 0.05; ##p < 0.01, compared with the aging group. Data are presented as mean ± SEM. | |
3.3 PS23 supplementation increases antioxidants in the hippocampus
The D-gal-aged mice displayed clear hippocampal-dependent spatial memory deficits (Fig. 2). Therefore, in the following experiments, we focused on investigating D-gal-induced changes in the hippocampus. We first examined the activity of antioxidant SOD in the hippocampus of the mice from each experimental group. The results revealed that the hippocampal SOD activity in the D-gal-induced aging group was significantly lower than that in the non-aging group (Fig. 4, non-aging: 11.1 ± 0.4, aging: 8.7 ± 0.2). PS23 supplementation ameliorated the reduction in SOD activity in the aged mice; however, HK-PS23 supplementation showed no such effect (Fig. 4, PS23: 10.6 ± 0.7, HK-PS23: 9.2 ± 0.2).
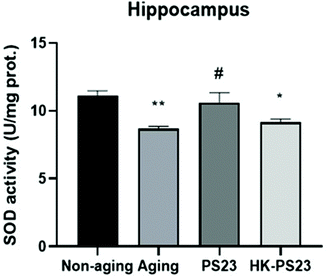 |
| Fig. 4 The effect of PS23 supplementation on superoxide dismutase (SOD) levels in the hippocampus. N = 6, *p < 0.05; **p < 0.01 as compared with the non-aging group. #p < 0.05 as compared with the aging group. Data are presented as mean ± SEM. | |
3.4
L. paracasei PS23 increases neurotransmitters
We next examined changes in the levels of monoamines and metabolites in the hippocampus. The dopamine (DA) level was very low in the hippocampus of D-gal-aged mice, and showed no significant differences between groups (Table 1). There were also no differences in the levels of the DA metabolites dihydroxyphenylacetic acid (DOPAC) and homovanillic acid (HVA) in each group; the DOPAC to DA and HVA to DA turnover ratios also did not differ between groups (Table 1). In contrast, we observed a significant elevation in hippocampal 5-hydroxytryptamine (5-HT) levels in both the PS23 and HK-PS23 supplementation groups (Table 1). The 5-HT metabolite 5-hydroxyindoleacetic acid (5-HIAA) levels did not differ between the groups (Table 1). However, the 5-HIAA to 5-HT turnover ratio was significantly lower in the PS23 treated mice than in the untreated aging mice (Table 1). These findings indicate that PS23 and HK-PS23 supplementation could increase the 5-HT levels in the hippocampus, and the reduced 5-HT turnover suggests that there was more 5-HT available in the hippocampus of PS23 supplemented mice.
Table 1 Hippocampal monoamines and metabolite concentrations of the aging mice
|
|
Hippocampus |
|
Non-aging |
Aging |
PS23 |
HK-PS23 |
Concentrations of monoamines and metabolites in the hippocampus are expressed as mean ± SEM (standard error of the mean). DA, dopamine; DOPAC, dihydroxyphenylacetic acid; HVA, homovanillic acid; 5-HT, 5-hydroxytryptamine; and 5-HIAA, 5-hydroxyindoleacetic acid. #p < 0.05 as compared to the aging group. |
Monoamines and metabolites (ng g−1 brain tissue) |
DA |
21 ± 16 |
1 ± 1 |
1 ± 0 |
9 ± 6 |
DOPAC |
152 ± 63 |
46 ± 15 |
9 ± 2 |
56 ± 28 |
HVA |
156 ± 13 |
148 ± 25 |
157 ± 12 |
181 ± 13 |
5-HT |
188 ± 23 |
144 ± 31 |
267 ± 11# |
280 ± 30# |
5-HIAA |
1643 ± 113 |
1430 ± 137 |
1257 ± 50 |
1697 ± 227 |
Turnover ratio (%) |
DOPAC: DA |
47 ± 26 |
74 ± 25 |
13 ± 4 |
19 ± 8 |
HVA: DA |
108 ± 64 |
270 ± 83 |
289 ± 115 |
198 ± 118 |
5-HIAA: 5-HT |
9 ± 1 |
11 ± 2 |
5 ± 0# |
6 ± 1 |
3.5
L. paracasei PS23 changes the age-related gene profiles in aging mice
To investigate the mechanisms underlying D-gal-induced hippocampal deficits and PS23 supplement-mediated improvements, we used RNA-seq analysis to delineate the genetic profiles of the hippocampus from each treatment group (Fig. 5A). We compared the profiles of the D-gal aged group with the PS23 and HK-PS23 supplemented aged groups (Fig. 5B and D). Genes displaying more than 2-fold changes were selected. Comparison between the D-gal aged group and PS23 supplemented aged group revealed 98 upregulated and 39 downregulated genes (Fig. 5B). Comparison between the D-gal aged group and the HK-PS23 supplemented group revealed 72 upregulated genes and 48 downregulated genes (Fig. 5D).
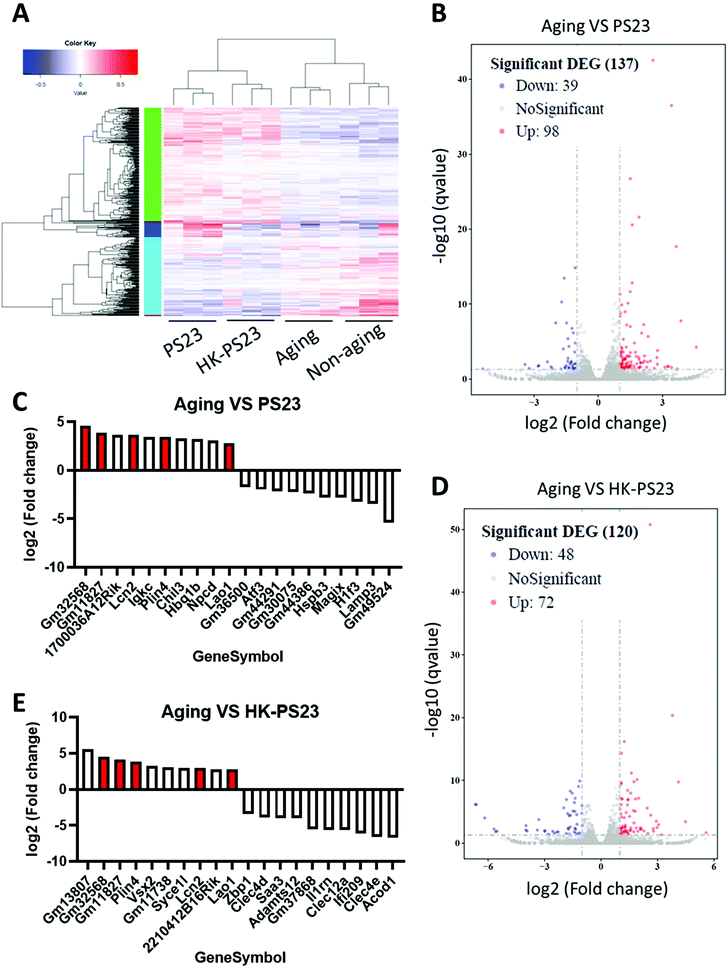 |
| Fig. 5 Effects of PS23 supplementation on gene profiles of the hippocampus. (A) Cluster analysis of differentially expressed genes. (B) Differential expression volcano plot of the D-gal aging group versus PS23 group. (C) Histogram of top 10 differentially expressed genes of the D-gal aging group versus PS23 group. (D) Differential expression volcano plot of the D-gal aging group versus HK-PS23 group. (E) Histogram of the top 10 differentially expressed genes of the D-gal aging group versus HK-PS23 group. Genes were shown in both PS23 and HK-PS23 are coloured red. | |
The top 10 overexpressed and underexpressed genes are presented in Fig. 5C and E. Among the top 10 overexpressed genes, we found that lipocalin 2 (Lcn2), perilipin 4 (Plin4), and L-amino acid oxidase (Lao1) were consistently upregulated in both PS23 and HK-PS23 groups (Fig. 5C and E). Further qPCR experiments confirmed that the expression levels of Lcn2, Plin4 and Lao1 genes were significantly elevated in both PS23 and HK-PS23 supplemented groups (Fig. S1†). Lcn2 is involved in the regulation of stress, anxiety-like behavior, and synaptic plasticity, and may protect the brain under inflammatory conditions.26–28 Plin4 is involved in lipid metabolism, while Lao1 has been demonstrated to play a role in hippocampal metabolism, fear learning, and memory.29 In addition, in the PS23 group, we discovered the downregulation of lysosome-associated membrane glycoprotein 3 (LAMP3). In the HK-PS23 group, there was a downregulation of aconitate decarboxylase 1 (Acod1), which regulates inflammation and infection.30
3.6
L. paracasei PS23 induced changes in the microbiota
Microbiota composition analysis revealed that the majority of the bacteria belonged to the phyla Bacteroidetes and Firmicutes (Fig. 6A). Among the different treatment groups, Bacteroidia was the major class representing the Bacteroidetes phylum, and Erysipelotrichia, Clostridia, and Bacilli were the major classes found in the Firmicutes phylum (Fig. 6A). We observed an increase in the number of bacteria from Actinobacteria in D-gal-aged mice (Fig. 6A). Alpha diversity was not significantly different between non-aging, D-gal aging, PS23, and HK-PS23 treated aging mice (Fig. 6B). NMDS analysis of beta diversity revealed that the microbial community of each treatment group did not separate into distinct clusters (Fig. 6C). The biodiversity of the microbes in each treatment group was not significantly different. Linear discriminant analysis effect size (LEfSE) was used to evaluate the differentially presented taxa. Actinobacteria abundance increased in the aging group (Fig. S2A and B†). Firmicutes, Bacteroidetes, and Tenerictes in the fecal microbiota were enriched in response to PS23 or HK-PS23 administration. We further used Metastats analysis to identify the differences in the relative abundance of microbes between groups (Fig. 6D and E). We found that Bifidobacterium, uncultured_Bacteroidales_bacterium, and Coriobacteriaceae _UCG_002 were significantly reduced in both PS23 and HK-PS23 treated aging mice (Fig. 6D and E). The relative abundance of microbes in the Dubosiella genus was increased in the PS23 supplemented group, whereas in the HK-PS23 supplemented group, the abundance decreased. In addition, in the PS23 supplemented group, the relative abundance of the Erysipelotrichaceae family was increased, whereas that of Oscillibacter and Ruminiclostridium_9 genera were reduced. Furthermore, in the HK-PS23 supplemented group, the relative abundance of microbes in the Muribaculaceae family was increased. These changes in the relative abundance of bacterial families and genera might contribute to the beneficial effects of PS23 and HK-PS23 supplementation.
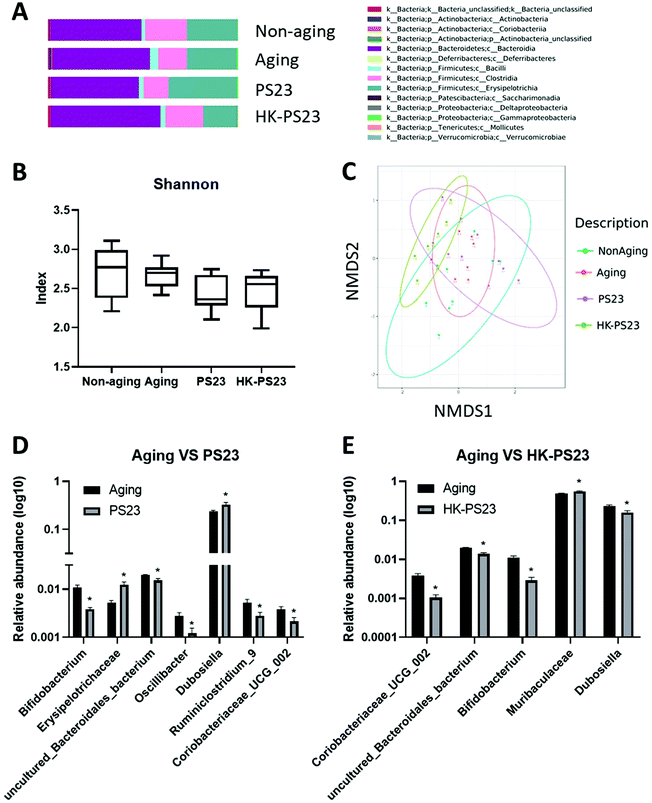 |
| Fig. 6 The effect of PS23 and HK-PS23 supplementation on the microbiota composition in D-gal aging mice. (A) Histogram of relative abundance at the class level. (B) Alpha diversity determined by Shannon′s diversity index. (C) Beta diversity determined by NMDS. (D) Metastats analysis of relative abundance of microbes between the aging and aging PS23 supplementation groups. (E) Metastats analysis of relative abundance of microbes between the aging and HK-PS23 supplementation groups. Data are presented as mean ± SEM. *p < 0.05 as compared with the aging group. | |
4 Discussion
The present study revealed that PS23 and HK-PS23 supplementation could improve spatial memory and anxiety-like behaviors in D-gal-induced aging mice. We focused our analysis on changes in hippocampal formation as the hippocampus is crucial for spatial memory. We discovered that PS23 supplementation significantly increased the SOD level in the hippocampus, which could reduce oxidative stress generated by D-gal-induced aging processes. PS23 and HK-PS23 supplementation further significantly increased the 5-HT levels in the hippocampus of aging mice, which could contribute to enhancing the hippocampal function, since previous studies have demonstrated that endogenous 5-HT could facilitate long-term potentiation in the hippocampus.31 Genetic profile analysis further uncovered several potential factors that might protect hippocampal formation against aging-induced inflammation and oxidative stress, in addition to factors that may contribute to neural plasticity. Furthermore, analysis of the gut microbiota revealed that PS23 and HK-PS23 supplementation did not drastically alter the gut microbiota composition; however, several bacterial genera displayed significant changes. These changes in specific bacterial genera may also contribute to the protective functions against aging-induced oxidative stress and inflammation, and help to enhance neuronal functions. These findings suggest that PS23 and HK-PS23 supplementation could alleviate cognitive deficits in D-gal-induced aging.
D-gal-induced aging mice displayed clear spatial memory deficits in the Morris water maze test. We demonstrated that PS23 and HK-PS23 supplementation alleviated spatial memory deficits in aging mice. These results were consistent with previous studies using another aging model, the SAMP8 mouse line, in which PS23 supplementation also prevented aging-induced spatial memory deficits.23 In the current study, we further demonstrated that HK-PS23 supplementation was effective at preventing aging-induced spatial memory deficits. Several prior studies have illustrated that HK-PS23 supplementation was as effective as live PS23 supplementation at inducing a number of beneficial effects. For example, HK-PS23 supplementation, like live PS23 supplementation, could reduce anxiety- and depression-like behaviors in mice in a maternal separation animal model of depression.20 Both HK-PS23 and live PS23 supplementation could increase anti-inflammatory cytokines and reduce proinflammatory cytokines in the serum.20,23 Two recent studies also demonstrated that HK-PS23 supplementation was as effective as live PS23 supplementation at reducing aging-induced muscle wastage and improving the gastrointestinal function in aging mice.22,24 Other probiotic species also exhibited biological effects even after heat-killing treatment. For example, both live and heat-killed Lactobacillus reuteri could reduce visceral pain induced by colorectal distension,32 and both live and dead Lactobacillus rhamnosus GG demonstrated anti-inflammatory effects in arthritis animal models.33 Although the exact bioactive compounds of HK-PS23 that underlie these beneficial effects are currently unknown, we speculate that some of these effects would be mediated by modulating the gut microbiota, as the microbiota composition of the HK-PS23 supplemented group is distinct from that of the other groups. Nevertheless, dead probiotic preparations have several advantages over live preparations, including improved safety, greater stability for long-term storage, and thus a longer shelf life.
D-Gal can increase reactive oxygen species and decrease antioxidant production in the hippocampus, which could lead to cell death and reductions in adult neurogenesis.14 These events can compromise hippocampal function, including spatial memory. We discovered that PS23 supplementation could increase the antioxidant SOD in the hippocampus, which could reduce the adverse effects of oxidative stress produced by D-gal administration. For example, SOD can reduce oxidative stress in cerebral endothelial cells to help maintain the integrity of the blood–brain barrier (BBB), thus preventing harmful materials from entering the brain.34 Previous studies using SAMP8 mice have demonstrated that PS23 supplementation could increase SOD and glutathione peroxidase in the serum.23 However, in the current study, only PS23 supplementation significantly increased the SOD levels in the hippocampus. Therefore, SOD might not play an important role in the beneficial effects of HK-PS23. Many gut bacteria can produce neural transmitters. We found that both PS23 and HK-PS23 supplementation increased 5-HT levels in the hippocampus (Table 1). As 5-HT does not pass through the BBB, it is unlikely that increases in hippocampal 5-HT would be directly produced by gut bacteria under the influence of PS23 or HK-PS23. Conversely, tryptophan, the precursor of 5-HT, can pass the BBB by active transportation and increase 5-HT production. However, tryptophan has to compete with other large neutral amino acids (LNAAs), including valine, isoleucine, leucine, tyrosine, phenylalanine, and methionine, for the common BBB transporter L-amino acid transporter-1; therefore, the ratio of tryptophan to LNAAs determines the availability of tryptophan in the brain.35 It is possible that PS23 and HK-PS23 supplementation increased the tryptophan to LNAAs ratio, thereby increasing the tryptophan level in the brain. Furthermore, it is also possible that tryptophan hydroxylase 2 (Tph2), the enzyme responsible for brain 5-HT production, was upregulated by probiotic supplementation. However, these speculations require further research. In the current study, neither PS23 nor HK-PS23 supplementation increased the DA levels in the hippocampus, which was inconsistent with the previous studies of SAMP8 senescence-accelerated mice, in which PS23 supplementation increased the DA levels in the hippocampus.23 This discrepancy may be due to the different animal models used. In the current study, we used chronic D-gal administration to facilitate the aging process, which was based on increasing free radical production and oxidative stress to accelerate cell aging. Compared to other neurons, dopaminergic neurons are more vulnerable to oxidative stress. Therefore, D-gal is likely to cause more damage to DA neurons than other neurons. Indeed, we observed significantly lower DA levels in the D-gal-induced aging mice compared to that of the non-aging mice, suggesting that DA neurons might be severely damaged and cannot be helped by PS23 supplementation. In contrast, SAMP8 mice were generated by generations of inbreeding36 and might not exhibit severe damage specific to DA neurons.
The results of the hippocampal RNA-seq analysis revealed several genetic candidates that could underlie the beneficial effects of PS23 and HK-PS23 supplementation on hippocampal function. Among the top 10 differentially expressed genes, Lcn2 and Lao1 were upregulated by both PS23 and HK-PS23 supplementation. Previous studies have demonstrated that Lcn2 could be induced by LPS administration, and was a potent protective factor against systemic inflammation in the central nervous system.28 In addition, Lcn2 is also involved in emotional behaviors and cognitive function; for example, Lcn2 knockout mice displayed enhanced anxiety-like behaviors after stress27 and upregulation of Lcn2 in the amygdala may represent adaptation responses to psychological stress.26 Therefore, the upregulation of Lcn2 in PS23 and HK-PS23 mice might facilitate the reduction in anxiety-like behaviors and improve their performance in the Morris water maze. Lao1 is an amino acid metabolic enzyme; prior studies have demonstrated that the Lao1 knockout mice display impairments in hippocampal-dependent fear learning and memory function in a passive avoidance test.29 Behavioral impairment also coincided with a reduction in hippocampal acetylcholine and 5-HT levels compared to the wild-type mice.29 It is plausible that Lao1, by modulating the metabolism of L-amino acids, indirectly facilitates the production of acetylcholine and 5-HT and improves hippocampal function. Neuronal pentraxin with the chromodomain (NPCD) gene is another gene that is significantly upregulated in PS23 supplemented mice. It has previously been demonstrated that NPCD may play a role in neuronal differentiation and synaptic plasticity37,38 and therefore, upregulation of NPCD might increase hippocampal neural plasticity and improve memory function.
The microbiota composition analysis demonstrated that the aging group displayed a significant increase in the bacteria of the phylum Actinobacteria. From the relative abundance analysis, we observed a reduction in the relative abundance of Bifidobacterium, which belongs to the Actinobacteria phylum, in both PS23 and HK-PS23 supplemented groups. Bifidobacterium is generally considered to comprise “good bacteria”, as many probiotics belong to this genus. The reduced relative abundance of Bifidobacterium in PS23 and HK-PS23 seemed contradictory to the beneficial effects of PS23 and HK-PS23 supplementation. However, we believe that this reduction in the relative abundance of Bifidobacterium was due to an increase in Bifidobacterium in the aging group instead of reductions in Bifidobacterium in the PS23 and HK-PS23 supplemented groups. Bifidobacterium might be increased in the aging group as a compensatory response to the adverse effects of D-gal treatment, whereas the adverse effects of D-gal treatment were reduced in the PS23 and HK-PS23 supplemented groups, and therefore Bifidobacterium was not significantly increased. Coriobacteriaceae _UCG_002 has been positively associated with serum fatty acid,39 and the family Coriobacteriaceae has been shown to increase significantly in the ceca of stressed mice.40 The reduced relative abundance of Coriobacteriaceae _UCG_002 in PS23 and HK-PS23 supplemented mice might reflect healthier fatty acid metabolism and reduced stress. Interestingly, the previous study had demonstrated that PS23 supplementation could increase the levels of short chain fatty acid, acetic acid and butyric acid, in the cecal contents of mice in a dextran sulfate sodium-induced colitis animal model.41 Modulating the short chain fatty acid production is a potential beneficial effect of PS23 supplementation. Furthermore, the relative abundance of Dubosiella was increased in the PS23 supplemented group and reduced in the HK-PS23 supplemented group. In a colitis animal model study, Dubosiella showed a negative correlation with the mRNA expression of IL-1β, IL-6, and TNF-α, and a positive correlation with IL-10.42 Increased levels of Dubosiella might provide anti-inflammatory benefits in PS23 supplemented mice; conversely, HK-PS23 supplementation might induce a reduction in anti-inflammatory function due to a reduction in Dubosiella. However, it is also possible that HK-PS23 supplementation may reduce inflammation by other means, for example, by downregulating the Acod1 gene to modulate inflammation in the hippocampus.
5 Conclusions
This study demonstrated that PS23 and HK-PS23 supplementation ameliorated aging-induced memory impairments, improved motor function, and reduced anxiety-like behaviors. The beneficial effects were mediated by increasing the 5-HT levels in the hippocampus, upregulating genes involved in synaptic plasticity, and protection against inflammation and oxidative stress. Changes in the gut microbiota may also contribute to the beneficial effects of PS23 and HK-PS23 supplementation. Thus, PS23 and HK-PS23 show great potential as dietary supplements for improving cognitive and motor functions in the elderly.
Author contributions
Conceptualization: L.-H.C. and S.W.; methodology: L.-H.C.; software: C.-L.H., W.-L.S. and P.-Y.C.; validation: L.-H.C., C.-L.H., A.-T.H. and P.-Y.C.; formal analysis: C.-L.H. and P.-Y.C.; investigation, L.-H.C., C.-L.H., A.-T.H., W.-L.S. and P.-Y.C.; resources: L.-H.C.; data curation: L.-H.C.; writing—original draft preparation: S.W.; writing—review and editing: L.-H.C and S.W.; visualization: S.W.; supervision: L.-H.C.; project administration: S.W.; and funding acquisition: S.W. All authors have read and agreed to the published version of the manuscript.
Conflicts of interest
L. H.C., C.-L.H., and P.-Y.C were employed by Bened. The funders had no role in the study design; in the collection, analyses, or interpretation of data; in the writing of the manuscript; or in the decision to publish the results.
Acknowledgements
This work was supported by BENED Biomedical Co., Ltd.
References
- T. G. Dinan, C. Stanton and J. F. Cryan, Psychobiotics: A Novel Class of Psychotropic, Biol. Psychiatry, 2013, 74, 720–726 CrossRef CAS PubMed.
- L. Desbonnet, L. Garrett, G. Clarke, B. Kiely, J. F. Cryan and T. G. Dinan, Effects of the probiotic Bifidobacterium infantis in the maternal separation model of depression, Neuroscience, 2010, 170, 1179–1188 CrossRef CAS PubMed.
- Y. W. Liu, W. H. Liu, C. C. Wu, Y. C. Juan, Y. C. Wu, H. P. Tsai, S. Wang and Y. C. Tsai, Psychotropic effects of Lactobacillus plantarum PS128 in early life-stressed and naive adult mice, Brain Res., 2016, 1631, 1–12 CrossRef CAS PubMed.
- J. A. Bravo, P. Forsythe, M. V. Chew, E. Escaravage, H. M. Savignac, T. G. Dinan, J. Bienenstock and J. F. Cryan, Ingestion of Lactobacillus strain regulates emotional behavior and central GABA receptor expression in a mouse via the vagus nerve, Proc. Natl. Acad. Sci. U. S. A., 2011, 108, 16050–16055 CrossRef CAS PubMed.
- H. M. Savignac, M. Tramullas, B. Kiely, T. G. Dinan and J. F. Cryan, Bifidobacteria modulate cognitive processes in an anxious mouse strain, Behav. Brain Res., 2015, 287, 59–72 CrossRef CAS PubMed.
- S. Liang, T. Wang, X. Hu, J. Luo, W. Li, X. Wu, Y. Duan and F. Jin, Administration of Lactobacillus helveticus NS8 improves behavioral, cognitive, and biochemical aberrations caused by chronic restraint stress, Neuroscience, 2015, 310, 561–577 CrossRef CAS PubMed.
- E. Akbari, Z. Asemi, R. D. Kakhaki, F. Bahmani, E. Kouchaki, O. R. Tamtaji, G. A. Hamidi and M. Salami, Effect of Probiotic Supplementation on Cognitive Function and Metabolic Status in Alzheimer's Disease: A Randomized, Double-Blind and Controlled Trial, Front. Aging Neurosci., 2016, 8, 256 Search PubMed.
- J. Xiao, N. Katsumata, F. Bernier, K. Ohno, Y. Yamauchi, T. Odamaki, K. Yoshikawa, K. Ito and T. Kaneko, Probiotic Bifidobacterium breve in Improving Cognitive Functions of Older Adults with Suspected Mild Cognitive Impairment: A Randomized, Double-Blind, Placebo-Controlled Trial, J. Alzheimer's Dis., 2020, 77, 139–147 CAS.
- Y. Kobayashi, T. Kuhara, M. Oki and J. Z. Xiao, Effects of Bifidobacterium breve A1 on the cognitive function of older adults with memory complaints: a randomised, double-blind, placebo-controlled trial, Benefic. Microbes, 2019, 10, 511–520 CrossRef CAS PubMed.
- Y.-H. Hwang, S. Park, J.-W. Paik, S.-W. Chae, D.-H. Kim, D.-G. Jeong, E. Ha, M. Kim, G. Hong, S.-H. Park, S.-J. Jung, S.-M. Lee, K.-H. Na, J. Kim and Y.-C. Chung, Efficacy and Safety of Lactobacillus Plantarum C29-Fermented Soybean (DW2009) in Individuals with Mild Cognitive Impairment: A 12-Week, Multi-Center, Randomized, Double-Blind, Placebo-Controlled Clinical Trial, Nutrients, 2019, 11, 305 CrossRef CAS PubMed.
- O. R. Tamtaji, R. Heidari-Soureshjani, N. Mirhosseini, E. Kouchaki, F. Bahmani, E. Aghadavod, M. Tajabadi-Ebrahimi and Z. Asemi, Probiotic and selenium co-supplementation, and the effects on clinical, metabolic and genetic status in Alzheimer's disease: A randomized, double-blind, controlled trial, Clin. Nutr., 2019, 38, 2569–2575 CrossRef CAS PubMed.
- A. Agahi, G. A. Hamidi, R. Daneshvar, M. Hamdieh, M. Soheili, A. Alinaghipour, S. M. E. Taba and M. Salami, Does Severity of Alzheimer's Disease Contribute to Its Responsiveness to Modifying Gut Microbiota? A Double Blind Clinical Trial, Front. Neurol., 2018, 9, 662 CrossRef PubMed.
- S. C. Ho, J. H. Liu and R. Y. Wu, Establishment of the mimetic aging effect in mice caused by D-galactose, Biogerontology, 2003, 4, 15–18 CrossRef CAS PubMed.
- Q. Zhang, X. Li, X. Cui and P. Zuo, D-galactose injured neurogenesis in the hippocampus of adult mice, Neurol. Res., 2005, 27, 552–556 CrossRef CAS PubMed.
- S. Haider, L. Liaquat, S. Shahzad, S. Sadir, S. Madiha, Z. Batool, S. Tabassum, S. Saleem, F. Naqvi and T. Perveen, A high dose of short term exogenous D-galactose administration in young male rats produces symptoms simulating the natural aging process, Life Sci., 2015, 124, 110–119 CrossRef CAS PubMed.
- M. Lei, X. Hua, M. Xiao, J. Ding, Q. Han and G. Hu, Impairments of astrocytes are involved in the d-galactose-induced brain aging, Biochem. Biophys. Res. Commun., 2008, 369, 1082–1087 CrossRef CAS PubMed.
- A. Prakash and A. Kumar, Pioglitazone alleviates the mitochondrial apoptotic pathway and mito-oxidative damage in the d-galactose-induced mouse model, Clin. Exp. Pharmacol. Physiol., 2013, 40, 644–651 CrossRef CAS PubMed.
- O. J. Banji, D. Banji and K. Ch, Curcumin and hesperidin improve cognition by suppressing mitochondrial dysfunction and apoptosis induced by D-galactose in rat brain, Food Chem. Toxicol., 2014, 74, 51–59 CrossRef CAS PubMed.
- F. Ullah, T. Ali, N. Ullah and M. O. Kim, Caffeine prevents d-galactose-induced cognitive deficits, oxidative stress, neuroinflammation and neurodegeneration in the adult rat brain, Neurochem. Int., 2015, 90, 114–124 CrossRef CAS PubMed.
- J. F. Liao, C. C. Hsu, G. T. Chou, J. S. Hsu, M. T. Liong and Y. C. Tsai, Lactobacillus paracasei PS23 reduced early-life stress abnormalities in maternal separation mouse model, Benefic. Microbes, 2019, 10, 425–436 CrossRef CAS PubMed.
- C. L. Wei, S. Wang, J. T. Yen, Y. F. Cheng, C. L. Liao, C. C. Hsu, C. C. Wu and Y. C. Tsai, Antidepressant-like activities of live and heat-killed Lactobacillus paracasei PS23 in chronic corticosterone-treated mice and possible mechanisms, Brain Res., 2019, 1711, 202–213 CrossRef CAS PubMed.
- L.-H. Cheng, S.-H. Cheng, C.-C. Wu, C.-L. Huang, P.-J. Wen, M.-Y. Chang and Y.-C. Tsai, Lactobacillus paracasei PS23 dietary supplementation alleviates muscle aging via ghrelin stimulation in d-galactose-induced aging mice, J. Funct. Foods, 2021, 85, 104651 CrossRef CAS.
- S. Y. Huang, L. H. Chen, M. F. Wang, C. C. Hsu, C. H. Chan, J. X. Li and H. Y. Huang, Lactobacillus paracasei PS23 Delays Progression of Age-Related Cognitive Decline in Senescence Accelerated Mouse Prone 8 (SAMP8) Mice, Nutrients, 2018, 10, 894 CrossRef PubMed.
- L. H. Chen, S. Y. Huang, K. C. Huang, C. C. Hsu, K. C. Yang, L. A. Li, C. H. Chan and H. Y. Huang, Lactobacillus paracasei PS23 decelerated age-related muscle loss by ensuring mitochondrial function in SAMP8 mice, Aging, 2019, 11, 756–770 CrossRef CAS PubMed.
- L. H. Chen, M. F. Wang, C. C. Chang, S. Y. Huang, C. H. Pan, Y. T. Yeh, C. H. Huang, C. H. Chan and H. Y. Huang, Lacticaseibacillus paracasei PS23 Effectively Modulates Gut Microbiota Composition and Improves Gastrointestinal Function in Aged SAMP8 Mice, Nutrients, 2021, 13, 1116 CrossRef CAS PubMed.
- A. E. Skrzypiec, R. S. Shah, E. Schiavon, E. Baker, N. Skene, R. Pawlak and M. Mucha, Stress-induced lipocalin-2 controls dendritic spine formation and neuronal activity in the amygdala, PLoS One, 2013, 8, e61046 CrossRef CAS PubMed.
- M. Mucha, A. E. Skrzypiec, E. Schiavon, B. K. Attwood, E. Kucerova and R. Pawlak, Lipocalin-2 controls neuronal excitability and anxiety by regulating dendritic spine formation and maturation, Proc. Natl. Acad. Sci. U. S. A., 2011, 108, 18436–18441 CrossRef CAS PubMed.
- S. S. Kang, Y. Ren, C. C. Liu, A. Kurti, K. E. Baker, G. Bu, Y. Asmann and J. D. Fryer, Lipocalin-2 protects the brain during inflammatory conditions, Mol. Psychiatry, 2018, 23, 344–350 CrossRef CAS PubMed.
- K. Usuda, T. Kawase, Y. Shigeno, S. Fukuzawa, K. Fujii, H. Zhang, T. Tsukahara, S. Tomonaga, G. Watanabe, W. Jin and K. Nagaoka, Hippocampal metabolism of amino acids by L-amino acid oxidase is involved in fear learning and memory, Sci. Rep., 2018, 8, 11073 CrossRef PubMed.
- R. Wu, F. Chen, N. Wang, D. Tang and R. Kang, ACOD1 in immunometabolism and disease, Cell. Mol. Immunol., 2020, 17, 822–833 CrossRef CAS PubMed.
- B. Mlinar, G. Stocca and R. Corradetti, Endogenous serotonin facilitates hippocampal long-term potentiation at CA3/CA1 synapses, J. Neural Transm., 2015, 122, 177–185 CrossRef CAS PubMed.
- T. Kamiya, L. Wang, P. Forsythe, G. Goettsche, Y. Mao, Y. Wang, G. Tougas and J. Bienenstock, Inhibitory effects of Lactobacillus reuteri on visceral pain induced by colorectal distension in Sprague-Dawley rats, Gut, 2006, 55, 191–196 CrossRef CAS PubMed.
- E. Baharav, F. Mor, M. Halpern and A. Weinberger, Lactobacillus GG bacteria ameliorate arthritis in Lewis rats, J. Nutr., 2004, 134, 1964–1969 CrossRef CAS PubMed.
- L. R. Freeman and J. N. Keller, Oxidative stress and cerebral endothelial cells: Regulation of the blood–brain-barrier and antioxidant based interventions, Biochim. Biophys. Acta, Mol. Basis Dis., 2012, 1822, 822–829 CrossRef CAS PubMed.
- E. Höglund, Ø. Øverli and S. Winberg, Tryptophan Metabolic Pathways and Brain Serotonergic Activity: A Comparative Review, Front. Endocrinol., 2019, 10, 158 CrossRef PubMed.
- T. Takeda, M. Hosokawa and K. Higuchi, Senescence-accelerated mouse (SAM): A novel murine model of senescence, Exp. Gerontol., 1997, 32, 105–109 CrossRef CAS PubMed.
- B. Chen and J. L. Bixby, Neuronal pentraxin with chromo domain (NPCD) is a novel class of protein expressed in multiple neuronal domains, J. Comp. Neurol., 2005, 481, 391–402 CrossRef CAS PubMed.
- N. Gómez de San José, F. Massa, S. Halbgebauer, P. Oeckl, P. Steinacker and M. Otto, Neuronal pentraxins as biomarkers of synaptic activity: from physiological functions to pathological changes in neurodegeneration, J. Neural Transm., 2022, 129, 207–230 CrossRef PubMed.
- H. Mu, Q. Zhou, R. Yang, J. Zeng, X. Li, R. Zhang, W. Tang, H. Li, S. Wang, T. Shen, X. Huang, L. Dou and J. Dong, Naringin Attenuates High Fat Diet Induced Non-alcoholic Fatty Liver Disease and Gut Bacterial Dysbiosis in Mice, Front. Microbiol., 2020, 11, 585066 CrossRef PubMed.
- K. M. Bangsgaard Bendtsen, L. Krych, D. B. Sørensen, W. Pang, D. S. Nielsen, K. Josefsen, L. H. Hansen, S. J. Sørensen and A. K. Hansen, Gut microbiota composition is correlated to grid floor induced stress and behavior in the BALB/c mouse, PLoS One, 2012, 7, e46231 CrossRef PubMed.
- K. Y. Lee, Y. C. Tsai, S. Y. Wang, Y. P. Chen and M. J. Chen, Coculture Strategy for Developing Lactobacillus paracasei PS23 Fermented Milk with Anti-Colitis Effect, Foods, 2021, 10, 2337 CrossRef CAS PubMed.
- F. Wan, H. Han, R. Zhong, M. Wang, S. Tang, S. Zhang, F. Hou, B. Yi and H. Zhang, Dihydroquercetin supplement alleviates colonic inflammation potentially through improved gut microbiota community in mice, Food Funct., 2021, 12, 11420–11434 RSC.
- J. F. Liao, Y. F. Cheng, S. T. You, W. C. Kuo, C. W. Huang, J. J. Chiou, C. C. Hsu, H. M. Hsieh-Li, S. Wang and Y. C. Tsai,
Lactobacillus plantarum PS128 alleviates neurodegenerative progression in 1-methyl-4-phenyl-1,2,3,6-tetrahydropyridine-induced mouse models of Parkinson's disease, Brain Behav. Immun., 2020, 90, 26–46 CrossRef CAS PubMed.
|
This journal is © The Royal Society of Chemistry 2022 |
Click here to see how this site uses Cookies. View our privacy policy here.