DOI:
10.1039/D2FO00048B
(Paper)
Food Funct., 2022,
13, 9254-9267
Native and fermented waxy cassava starch as a novel gluten-free and clean label ingredient for baking and expanded product development†
Received
6th January 2022
, Accepted 13th May 2022
First published on 18th August 2022
Abstract
Amylose-free and wild-type cassava starches were fermented for up to 30 days and oven- or sun-dried. The specific volume (ν) after baking was measured in native and fermented starches. The average ν (across treatments) for waxy starch was 3.5 times higher than that in wild-type starches (17.6 vs. 4.8 cm3 g−1). The best wild-type starch (obtained after fermentation and sun-drying) had considerably poorer breadmaking potential than native waxy cassava (8.4 vs. 16.4 cm3 g−1, respectively). The best results were generally obtained through the synergistic combination of fermentation (for about 10–14 days) and sun-drying. Fermentation reduced viscosities and the weight average molar mass led to denser macromolecules and increased branching degree, which are linked to a high loaf volume. The absence of amylose, however, was shown to be a main determinant as well. Native waxy starch (neutral in taste, gluten-free, and considerably less expensive than the current alternatives to cassava) could become a new ingredient for the formulation of clean label-baked or fried expanded products.
1. Introduction
Roots, tubers and bananas (RTB) play an essential staple food role in the tropics and subtropics, particularly in Africa where they are culturally highly valued.1 Cassava starch offers numerous advantages which include being a gluten-free and non-allergenic ingredient with a neutral taste, and having a high level of purity, high-paste clarity with freeze–thaw stability and excellent thickening characteristics.2 Therefore, over the last 50 years, cassava has gradually become the second most important source of starch. About 10 million tons of cassava starch are produced annually, representing 12% of the global starch production.3 Industrial production is mainly carried out in South-East Asia and South America.
Cassava flours have been used, among many applications, in composite flours and blends to improve the rheology of dough, but also to reduce bread production costs in cereal-importing countries.4 Tests showed that the sensory quality and texture of bread were little modified up to 25–30% substitution of wheat flour by cassava flour or starch.5
Ingredients for gluten-free formulations can be used in the diets of people who need food with low protein and phenylalanine contents. Among the medical foods, low-protein/protein-free foods have been shown to improve the physical manifestation of metabolic disorders in patients with amino acid or protein-related diseases, such as phenylketonuria, tyrosinaemia type I and chronic kidney and coeliac disease.6,7 The low protein content of cassava roots allows flours and starches to be used in the formulation of gluten-free bread to improve baking characteristics such as the specific volume, color and crumb structure and texture.8–11 A natural modification of cassava starch, after anaerobic fermentation for 15 to 30 days and exposure to ultraviolet light through sun-drying, is traditional in South America.12 If the fermented starch is not sun-dried, no expansion properties are acquired after processing. This naturally modified cassava sour starch is used for popular products in South America and Indonesia and the expanded products obtained are of the type of corn or wheat extrudates. Without expensive, high-tech and energy consuming equipment as extruders, the same characteristics are obtained during a simple oven cooking.13,14
Several studies report the unique properties of sour cassava starch expansion and the changes that occur during fermentation, exposure to sunlight and/or ultraviolet radiation and cooking.14,15–20 Oxidation, through different oxidative agents, is an alternative to overcome the typical sensory characteristics of fermented products.21–23 There are several commercial brands in South America, for the manufacture of cheese breads or gluten-free expanded products.24,25 In the last few years, there has been a sharp increase in the demand for clean label starch and for environmentally friendly products.26,27
Naturally occurring amylose-free (waxy) cassava mutations have been reported.28 Several programs are now developing waxy commercial varieties, and commercial production has begun in Thailand, Colombia and Brazil.29–31 Formulation trials and use of specific functional properties of waxy cassava starch are being studied at the industrial level.32–39
Molecular and supra-molecular structural characterization studies of waxy cassava amylopectin highlight a relatively high proportion of short branched chains of DP 6–12 and a low proportion of chains with DP 13–24, which is associated with retrogradation and its unique freeze–thaw and cold storage stability. Waxy cassava starch could serve as a clean-label thickener in frozen foods.34,40–42
The aim of this work was to assess the potential of waxy cassava starch and its macromolecular determinants for the development of gluten-free ingredients with ecofriendly production and clean labelling for baked products.
2. Materials and methods
2.1. Genotypes and growing conditions
Several cassava genotypes were grown at the CIAT experimental station in Palmira, Colombia (1000 meters above sea level – m.a.s.l.). AM 206-5 produces waxy starch. The remaining clones (Cumbre3, ICA-48, and HMC-1) produce starch with around 21% amylose.43 Cumbre3 was released for the highlands (1400 to 1800 m.a.s.l.). Starches were extracted and processed from plants grown in different growing seasons as described below.
2.2. Starch extraction and processing
The cassava starch extraction and the production of the samples were carried out following a published protocol.14 After the harvest, all the cassava roots were transported to a traditional factory (Rallandería) in the Cauca Department (Colombia) for starch extraction, using the following procedures: washing–peeling, rasping and extraction, whereby starch was drained off through sieving the mash under running water. After 24 h of decantation, the top liquid phase was discarded. Wet starch blocks were introduced in fermentation tanks of 1 m2 surface and 1.5 m height. Samples were collected using an auger and dried in an oven or directly in the sun.
2.3. Breadmaking test
Bread dough was prepared according to the CIAT protocol as previously described,14 with slight modification. 125 g of starch sample (12–13% moisture content), 75 g of “Costeño” cheese and 18.7 g of sunflower oil were hand mixed on a stainless steel plate. Secondly pre-gelatinized starch was prepared by mixing 37.25 g starch (dry matter) with 31.25 g of cold water and 62.5 g of water and homogenized using a heating plate Corning PC-351 (615 Watts) at a maximum temperature of 360 °C, using a “Classic Hamilton Beach Drink Master” stirrer. The native starch mix and pregelatinized starch were blended homogeneously with 100 mL of cold water using a Kitchen Aid Artisan mixer (velocity: 1). For each evaluation, 12 portions of 33 g of dough are shaped to produce 12 rings. These rings are placed on an independent baking tray. The weights and volumes of the breads were measured as in Maldonado Alvarado et al.14 The weights and volumes of the breads were measured using a scale and a pycnometer using the cauliflower seed displacement method.44 For each sample to be tested, two preparations of the dough were made independently. Two baking trays, required for each preparation, were introduced in the same oven with 12 rings of 33 g of dough on each tray. Breadmaking ability (or bread expansion) was characterized as a ratio: bread volume/bread weight (mL g−1). The specific volume of each loaf is evaluated by dividing the volume of the cooked product by the weight of the initial individual raw ring (33 g). The average of the 12 specific volumes obtained gives the specific volume of each independent preparation (replicate). The average of the two replicates obtained gives the specific volume of each sample.
2.4. Pasting properties
To inactivate the enzymes and amylase produced during fermentation, which could be active in the starch suspension, viscoamylographic profiles were carried out in the presence of an inhibitor. Starch (1.25 g db) was dispersed in a silver nitrate solution at 0.002 M (23 mL) to produce a 5% suspension as reported earlier.45 Five parameters were measured on the recorded visco-amylogram: pasting temperature (PT), pasting time (Pt), peak viscosity (PV), holding strength (trough) as the lowest hot paste viscosity (HPV) and final viscosity (FV).14 Two additional parameters were then calculated: breakdown (BD), estimated as PVmax-HPV, and setback (SB), estimated as FV-HPV.
2.5. Macromolecular structures
AM 206-5, Cumbre3 and HMC-1 were fermented in 2011. Cumbre3 and HMC-1 starches were the same samples as studied in Maldonado Alvarado et al.,14 and the corresponding ν values were reported from that reference. The molar mass, size, conformation and average number of branching points were obtained using asymmetrical flow field flow fractionation (AF4) coupled with multi-angle laser light scattering (MALLS) using the same procedure and setup as described by several authors.33,34,46,47 The starches were first dissolved in a DMSO/water (95/5) mixture and then solubilized in water by microwave heating under pressure.46 After filtration through 5 μm Durapore™ membranes (Waters, Bedford, MA, USA), the solutions were injected into the AF4 device. The AF4 system (Consenxus, Ober-Hilbersheim, Germany), its configuration, the membrane (10 kDa cellulose) and the flow method were exactly the same as previously described.46 The carrier was Millipore water containing 0.2 g L−1 sodium azide. Two detectors were coupled with AF4: a Dawn® Heleos™ MALLS, fitted with a K5 flow cell and a GaAs laser, (λ = 658 nm), from Wyatt Technology Corporation (Santa Barbara, CA, USA) and a refractometer (ERC-7515A, Erma, Tokyo, Japan).
Solubilization and elution recoveries were calculated comparing the mass before and after filtration, and the mass eluted in the AF4 channel as previously described.46 The weight-average molar mass (
w) and z-average radius of gyration (
G) were established using ASTRA® software from Wyatt Technology Corporation (version 6.0 for PC), as previously described.46 Branching characteristics were calculated as described by Rolland-Sabaté and co-workers.33,34,46,47 The average number of branching points per macromolecule (
) and the average number of glucosyl units in a linear chain per branching point
were obtained from the molar mass and radius of gyration data through the average shrinking factor gM (gM =
2Gw(br)/
2Gw(lin), where
Gw(br) and
Gw(lin) are the weight average radii of gyration of the branched molecule and of its linear equivalent at the same molar mass).
Gw(lin) was calculated from the equation linking the molar mass and radius of gyration established for strictly linear amyloses using the ABC model. The branching degree (BDmC) was finally deduced using the modified ABC model corrected for cassava amylopectin.34,47
2.6. Starch crystallinity
Crystallinity information was determined by X-ray diffraction as previously described.28
2.7. Statistical analysis
Years, repetitions and rings within repetitions were the only source of variation considered as random effects. Genotypes, fermentation and drying treatments were considered fixed. Analysis of variance was conducted with SAS. In few cases, only 10 or 11 rings per starch sample could be prepared (instead of the standard 12 rings per repetition). In these cases, averages of the available rings were used to replace the missing values and provide a balanced data set. Analysis of variance was only conducted in balance data sets involving starches that have been processed simultaneously the same year and from plants that had also grown and been harvested simultaneously in the same season.
The available information was organized in three different experiments to guarantee uniformity of conditions in which plants grew, and starches were fermented and processed. Fermentation is a natural process without inoculum; the bacterial flora comes from the processing of the roots and the washing and sieving of the pulp. The first subsample was oven-dried at 40 °C for 24 h to obtain native, non-fermented, oven-dried cassava starch [N,O]. The second subsample was sun-dried for about 12 h to obtain non-fermented, sun-dried starch [N,S]. The third and fourth subsamples were fermented together in a tank at ambient temperature (between 20 and 30 °C) for up to 30 days. The third subsample was oven-dried for 24 h at 40 °C to obtain fermented, oven-dried starch [F,O], whereas the fourth was sun-dried for 12 h to obtain fermented, sun-dried starch [F,S].
Experiment 1 included three genotypes: AM 206-5, Cumbre3 and HMC-1 grown and processed at the same time in two different years (2013 and 2015A). For each genotype, four starch samples were considered: [N,O], native, oven-dried; [N,S], native, sun-dried; [F,O], fermented, oven-dried; and [F,S], fermented, sun-dried. Experiment 2 included two genotypes: AM 206-5 and ICA-48 grown and processed at the same time in 2015B. For each genotype, four starch samples were considered: [N,O], [N,S], [F,O] and [F,S]. Experiment 3 involved the assessment of the breadmaking capacity of mixtures of amylose-free (AM 206-5) and wild-type (HMC-1) native starches (0, 10, 20, 30, 40, 50, 60, 70, 80, 90 and 100% waxy starch).
3. Results and discussion
3.1. Breadmaking potential
The protocols used for assessing breadmaking capacity through the measurement of specific volume (ν) were reliable and resulted in very little experimental error (ESI Fig. S1†).
Fig. 1 shows the comparison of wild-type and waxy starches. Only sun-dried [S] starches were considered because oven-dried [O] did not allow development of the benefit of fermentation on expansion. The excellent breadmaking capacity of waxy starch (AM 206-5) is apparent. No other starch, regardless of the treatment, resulted in a better performance in comparison with native waxy starch. In general, ν of waxy starches increased consistently through fermentation time. On the other hand, ν of wild-type starches from Cumbre3 (2013), ICA-48 (2014A) and HMC-1 (2013) tended to increase sharply after one or two days of fermentation and then to decline. Data from 2015B, however, showed gradual increases of ν through the time of fermentation. The highland variety Cumbre3 provided the best results from non-waxy starches, particularly for the evaluation conducted in 2013. Different batches (years 2013, 2015A and 2015B) resulted in distinctive performances, suggesting that environmental and biological conditions during plant growth have strong effects as reported by Karlström48 on waxy cassava starch and on cassava landrace starches.49,50
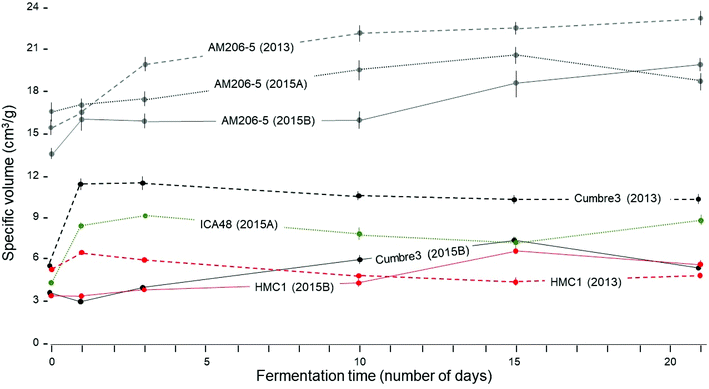 |
| Fig. 1 Effect of varying duration of fermentation on the breadmaking capacity of wild-type (Cumbre3, ICA48 and HMC-1) and amylose-free (AM 206-5) starches processed in different batches in 2013 and 2015A, and 2015B. Only responses of sun-dried starches have been considered. Bars at each point indicate the size of the standard deviations for each evaluation (n = 24). | |
By and large, responses to fermentation from each source of starch could be reliably summarized using data from 10 days of fermentation, which is a useful reference time in relation to commercial production of fermented starches. To facilitate the statistical analyses comparing native and fermented starches, only data from 0 and 10 days of fermentation were used (other times would also confirm the drastic differences between waxy and wild-type starches). All the main sources of variation in Experiments 1 and 2 were highly significant (P = 0.01, ANOVA tables are not presented). The effect of years (Experiment 1) was highly significant and resulted in strong interactions with other sources of variation. In every case, ν was lower in the year 2015A compared with the results from 2013 (Fig. 2).
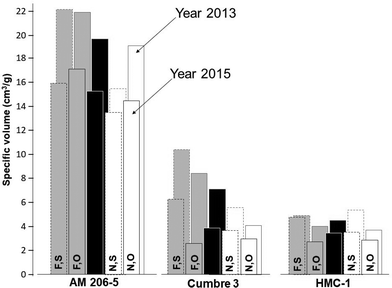 |
| Fig. 2 Average specific volumes (cm3 g−1) from Experiment 1 conducted in two different years (2015A and 2013 in the foreground and background, respectively). AM 206-5 is an amylose-free starch. Cumbre3 is a highland variety especially suited for the production of fermented starch. [F,S], fermented, sun-dried; [F,O], fermented, oven-dried; [N,S], native, sun-dried; [N,O], native, oven-dried. Black columns: genotype average across starch type and the drying method. Data from a 10-day fermentation process was used for the fermented starches. | |
It was decided, therefore, to conduct individual analyses for each year. All sources of variation were statistically significant, except for the triple interaction (genotype*fermenting*drying) in year 2015 (ANOVA tables are not presented). By far, the most important source of variation was genotypes, accounting for about 85% of the total sum of squares. Fig. 2 and Table 1 summarize the relevant results from Experiment 1.
Table 1 Averages for the specific volume of the three main treatments and their combinations in Experiments 1 and 2. Averages (within each box) followed by the same letter are not statistically significant at the 1% probability level based on the least significant difference. On top of the table are the averages for the main sources of variation (genotypes across starch type and the drying method; starch type across genotypes and the drying method; and the drying method across genotypes and starch type). Data from a 10-day fermentation process was used for the fermented starches
Experiment 1 |
Experiment 2 |
Source of variation |
2013 |
Source of variation |
2015A |
Source of variation |
2015B |
AM 206-5 |
19.7a |
AM 206-5 |
15.3a |
AM 206-5 |
17.6a |
Cumbre3 |
7.1b |
Cumbre3 |
3.9b |
ICA-48 |
4.9b |
HMC-1 |
4.5c |
HMC-1 |
3.5b |
|
|
Fermented [F] |
12.0a |
Fermented [F] |
8.2a |
Fermented [F] |
12.4a |
Native [N] |
8.9b |
Native [N] |
6.8b |
Native [N] |
10.1b |
Sun-dried [S] |
10.7a |
Sun-dried [S] |
7.9a |
Sun-dried [S] |
12.0a |
Oven-dried [O] |
10.2b |
Oven-dried [O] |
7.1b |
Oven-dried [O] |
10.5b |
AM 206-5 [F,S] |
22.2a |
AM 206-5 [F,O] |
17.1a |
AM 206-5 [F,S] |
19.5a |
AM 206-5 [F,O] |
21.9a |
AM 206-5 [F,S] |
15.9b |
AM 206-5 [F,O] |
18.6a |
AM 206-5 [N,O] |
19.1b |
AM 206-5 [N,O] |
14.5c |
AM 206-5 [N,S] |
16.4b |
AM 206-5 [N,S] |
15.5c |
AM 206-5 [N,S] |
13.5c |
AM 206-5 [N,O] |
16.0b |
Cumbre3 [F,S] |
10.4d |
Cumbre3 [F,S] |
6.3d |
ICA-48 [F,S] |
7.7c |
Cumbre3 [F,O] |
8.4e |
HMC-1 [F,S] |
4.8e |
ICA-48 [N,S] |
4.5d |
Cumbre3 [N,S] |
5.6f |
Cumbre3 [N,S] |
3.7fg |
ICA-48 [F,O] |
3.9d |
HMC-1 [N,S] |
5.4fg |
HMC-1 [N,S] |
3.5g |
ICA-48 [N,O] |
3.5d |
HMC-1 [F,S] |
4.9gh |
Cumbre3 [N,O] |
3.0gh |
|
|
Cumbre3 [N,O] |
4.1hi |
HMC-1 [N,O] |
2.9gh |
|
|
HMC-1 [F,O] |
4.0hi |
HMC-1 [F,O] |
2.8h |
|
|
HMC-1 [N,O] |
3.7i |
Cumbre3 [F,O] |
2.6h |
|
|
The averages (across fermented and native starches and after oven- and sun-drying) of the three genotypes were highly contrasting (provided at the top of Table 1). There is an unprecedented ν of the amylose-free starch from AM 206-5 (19.7 and 15.3 cm3 g−1 respectively for 2013 and 2015A), compared with the wild-types (7.1 and 3.9 cm3 g−1 for Cumbre3 and 4.5 and 3.5 cm3 g−1 HMC-1, respectively, for years 2013 and 2015A). ν from the highland variety Cumbre3 were higher than those of the lowland clone (HMC-1), particularly in year 2013. In general, for each genotype, fermented starches [F] resulted in higher ν compared with the native [N] counterparts.
Contrary to wild-type starches, the expansion of waxy starches was generally better in oven-dried samples than in sun-dried samples. In every case, however, fermentation resulted in increased expansion regardless of the drying method. Illumination of the starches with linearly polarised visible light induced depolymerisation–repolymerisation reactions of starch polysaccharide chains.51 Rearrangements of the molecular starch structure depend on the illumination time and the botanical source of the starch. Structural changes lead to the resulting products with different functional properties. On the other hand, [S] was always better than [O] (within starch type) in wild-type starches from Cumbre3 and HMC-1.
Results from Experiment 2 are presented in Table 1 and Fig. 3.
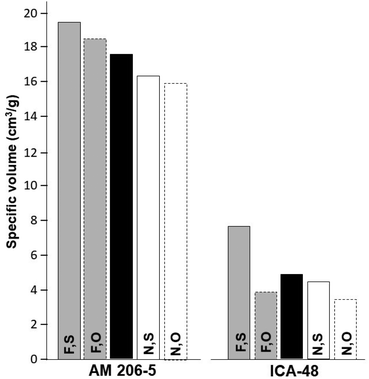 |
| Fig. 3 Average specific volumes (cm3 g−1) from Experiment 2 (year 2015B). Data based on two reps and 12 samples/rep for each type of starch. AM 206-5 is an amylose-free starch, whereas ICA-48 produces wild-type starch. [F,S], fermented, sun-dried; [F,O], fermented, oven-dried; [N,S], native, sun-dried; [N,O], native, oven-dried. Black columns: genotype average across starch type and the drying method. Data from a 10-day fermentation process was used for the fermented starches. | |
As in the case of Experiment 1, every main source of variation was significant, and the genotypes accounted for a large proportion of the total sum of squares (≈ 92%). Fermentation had a higher impact on ν than the drying method as it was in the case of Experiment 1. The average from AM 206-5 (17.6 cm3 g−1) was an intermediate between the values obtained in Experiment 1 and considerably higher than that of ICA-48 (4.9 cm3 g−1). Fermentation resulted in clearly higher ν values compared with native starches in both genotypes (Fig. 3). In contrast with Experiment 1, sun drying was better than oven-drying for AM 206-5. However, these differences were not significant (Table 1).
Experiment 3 assessed the breadmaking potential of the mixtures of wild-type and waxy native starches. Eleven mixtures (0 through 100% waxy starch, with 10% increments) were prepared and baked. Regression analysis (Fig. 4A) demonstrated a significant positive association between the percentage of waxy starch and ν, with R2 = 0.9656. Fig. 4B illustrates the differences between [N,O], [N,S] [F,O] and [F,O] from Cumbre3.
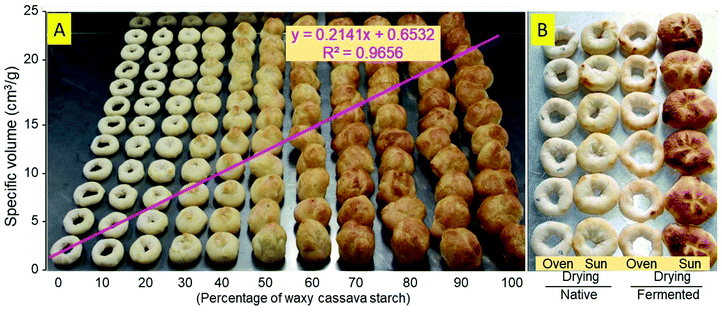 |
| Fig. 4 Effect of the amylose content, fermentation and drying method on the breadmaking potential of cassava starch. (A) Expanded products from native wild-type and amylose-free cassava blends (HMC-1/AM 206-5). The regression of specific volume on the % of waxy starch is also presented. (B) Effect of fermentation and the drying method on the expansion of wild-type cassava starch (Cumbre3). | |
3.2. Paste behaviour
Fig. 5a shows the presence of amylolytic enzymes in waxy cassava samples from cassava starch fermentation (2015). For each sample at 0 and 10 days of fermentation, the profiles without an inhibitor, in water (W), develop amylograms with viscosity values much lower than their equivalent with an inhibitor (silver nitrate, 0.002 M, as reported earlier45). The comparison of the 0 and 10 day fermentation times and the influence of oven- or sun-drying in the presence of an inhibitor highlights the changes in the pasting properties during the fermentation and drying processes and provide information on the structural modifications of the starch.
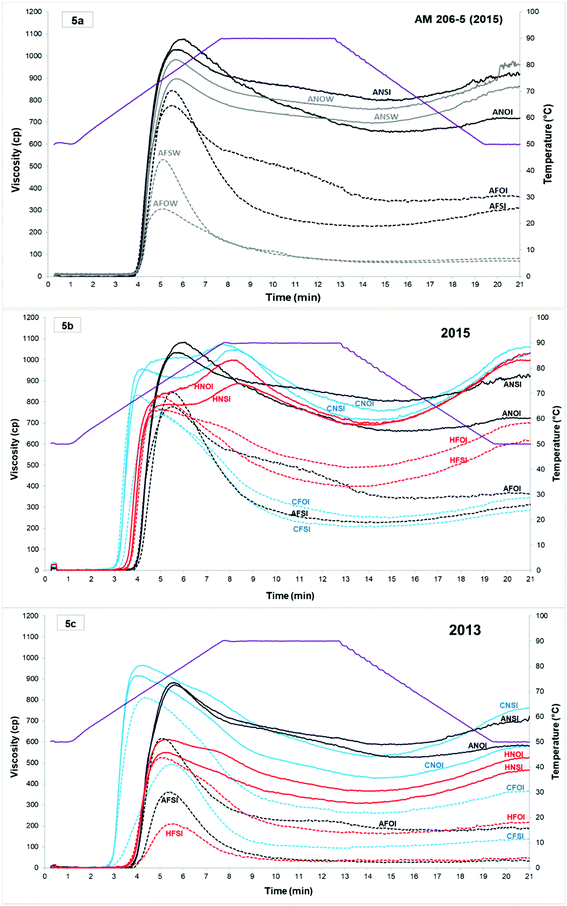 |
| Fig. 5 Amylograms obtained in Experiment 1 from native and fermented (10 days) cassava starches obtained in two different years (2013 at the bottom (c) and 2015A in the middle (b)). The top graph (a) shows the effect of the enzyme inhibitor (silver nitrate, 0.002 M) on the amylographic profiles for AM206-5 obtained for the same samples in water (W) or in the presence of inhibitor (I). Solid and dotted lines represent respectively the native and fermented starches. Each line is identified with a 4-letter code. The first letter identifies the genotype (A: AM 206-5 in black; C: Cumbre3 in blue; and H: HMC-1 in red). The second letter identifies the native (N) or fermented (F) starches. The third letter distinguishes between the sun (S) and the oven-dried (O) starches. The fourth letter indicates the presence of an inhibitor in the suspension (I) or its absence in the water (W). | |
Visco-amylograms and the extracted relevant pasting properties from Experiment 1 (2013 and 2015A) are shown in Fig. 5 and Table 2.
Table 2 Parameters measured in the visco-amylogram (Experiment 1: 2013 and 2015A)14,45
Genotypes |
Starch type |
Drying method |
Year |
Pasting |
Viscosity |
Pasting time (Pt) |
Pasting temperature (PT) |
Peak viscosity (PV1) |
Peak viscosity (PV2) |
Holding strength (HPV) |
Final viscosity (FV) |
Breakdown (PVmax-HPV) |
Setback (FV-HPV) |
(min) |
(°C) |
(cP) |
Fermentation drastically reduced viscosities with a stronger effect of fermentation compared to UV irradiation. Reductions were larger in [S] than in [O] starches. |
AM 206-5 |
[N] |
[O] |
2013 |
3.8 |
67.0 |
872 |
* |
526 |
578 |
346 |
52 |
AM 206-5 |
[N] |
[S] |
2013 |
3.9 |
67.2 |
884 |
* |
587 |
715 |
297 |
128 |
AM 206-5 |
[F] |
[O] |
2013 |
3.8 |
66.7 |
617 |
* |
178 |
181 |
439 |
3 |
AM 206-5 |
[F] |
[S] |
2013 |
4.0 |
68.2 |
364 |
* |
24 |
32 |
340 |
8 |
AM 206-5 |
[N] |
[O] |
2015 |
3.9 |
67.3 |
1083 |
* |
659 |
723 |
424 |
64 |
AM 206-5 |
[N] |
[S] |
2015 |
3.9 |
67.5 |
1035 |
* |
802 |
911 |
233 |
109 |
AM 206-5 |
[F] |
[O] |
2015 |
3.9 |
67.0 |
778 |
* |
338 |
360 |
440 |
22 |
AM 206-5 |
[F] |
[S] |
2015 |
4.0 |
67.7 |
844 |
* |
227 |
316 |
617 |
89 |
Cumbre3 |
[N] |
[O] |
2013 |
2.8 |
61.0 |
917 |
* |
427 |
586 |
490 |
159 |
Cumbre3 |
[N] |
[S] |
2013 |
2.9 |
61.0 |
965 |
* |
530 |
766 |
435 |
236 |
Cumbre3 |
[F] |
[O] |
2013 |
2.8 |
60.6 |
811 |
* |
259 |
361 |
552 |
102 |
Cumbre3 |
[F] |
[S] |
2013 |
3.1 |
62.5 |
497 |
* |
93 |
137 |
404 |
44 |
Cumbre3 |
[N] |
[O] |
2015 |
3.0 |
62.0 |
1012 |
1077 |
756 |
1067 |
321 |
311 |
Cumbre3 |
[N] |
[S] |
2015 |
3.0 |
62.1 |
957 |
1047 |
711 |
1028 |
336 |
317 |
Cumbre3 |
[F] |
[O] |
2015 |
3.0 |
62.0 |
833 |
* |
249 |
346 |
584 |
97 |
Cumbre3 |
[F] |
[S] |
2015 |
3.2 |
63.5 |
738 |
* |
206 |
290 |
532 |
84 |
HMC-1 |
[N] |
[O] |
2013 |
3.7 |
66.0 |
611 |
* |
365 |
530 |
246 |
165 |
HMC-1 |
[N] |
[S] |
2013 |
3.7 |
65.9 |
550 |
* |
307 |
470 |
243 |
163 |
HMC-1 |
[F] |
[O] |
2013 |
3.6 |
65.6 |
527 |
* |
160 |
215 |
367 |
55 |
HMC-1 |
[F] |
[S] |
2013 |
4.0 |
67.8 |
211 |
* |
28 |
45 |
183 |
17 |
HMC-1 |
[N] |
[O] |
2015 |
3.4 |
64.6 |
888 |
999 |
694 |
1029 |
305 |
335 |
HMC-1 |
[N] |
[S] |
2015 |
3.6 |
65.6 |
791 |
890 |
689 |
999 |
201 |
310 |
HMC-1 |
[F] |
[O] |
2015 |
3.6 |
65.3 |
764 |
* |
487 |
700 |
277 |
213 |
HMC-1 |
[F] |
[S] |
2015 |
3.6 |
65.6 |
824 |
* |
396 |
620 |
428 |
224 |
Analysis of RVA amylograms from different genotypes confirmed the previous results (Maldonado Alvarado et al., 2013).14
The year of harvest significantly influences the viscosity profile of native starches. In 2015 amylograms, two very distinct peaks were observed for the wild types and only one for the amylose-free starch, whereas in 2013 only one peak was visible for all native starches (Fig. 5).
The hypothesis put forward by Maldonado et al.14 concerning a correlation between breakdown and breadmaking ability has not been confirmed in our trials, even if breakdown is much higher for Cumbre3 than for HCM-1. This is no longer true for waxy cassava starch which develops the highest breadmaking ability.
3.3. Macromolecular and supramolecular structures
In order to gain insight on the mechanism underlying this breadmaking capacity, macromolecular characteristics of three starches from the 2011 harvest exhibiting contrasting expansion properties were determined by AF4-MALLS. AF4 elugrams from native AM 206-5 (regardless of the treatment) revealed a single concentration peak, corresponding to amylopectin (Fig. 6A and B). Native Cumbre3 and HMC-1 showed an additional shoulder at smaller elution volumes (i.e. smaller molecular sizes) corresponding to amylose (Fig. 6C and D). Similar AF4 elugrams and molar mass distributions were obtained for the two native non-waxy starches, therefore only results from Cumbre3 are shown. Although native AM 206-5 exhibited the highest amylopectin
w and ν after baking, no relationship could be demonstrated between the amylopectin
w of native starches and ν (Table 3). Thus, the macromolecular determining factor for expansion seemed to be the amylose content in native samples, as when this decreased (HMC-1 (21.7%) > Cumbre3 (16.7%) > AM 206-5 (0%)), the ν increased.14,28 These findings confirmed the previous reports on wild type cassava, postulating that amylose prevents expansion through the formation of a sample-wide network of amylose-lipid complexes.14
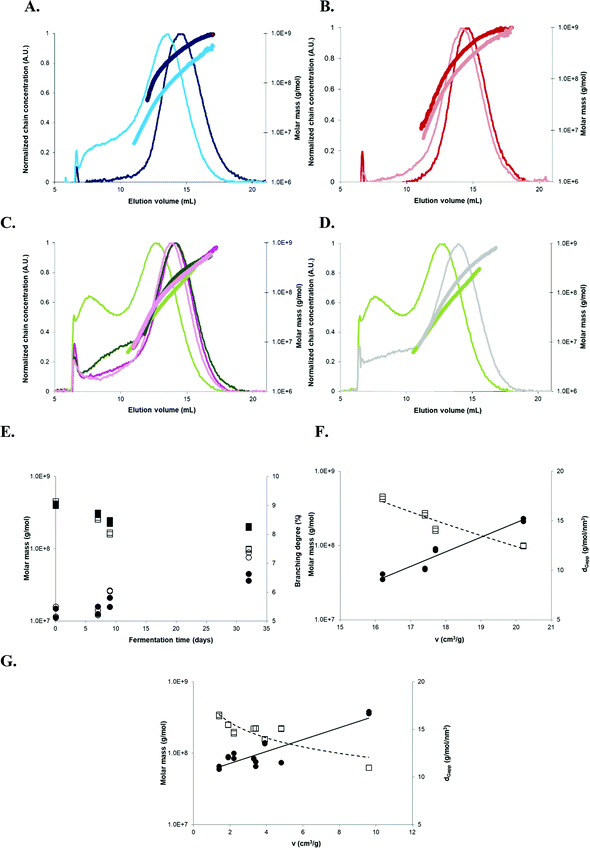 |
| Fig. 6 AF4 elugrams, molar mass distributions, and evolution of the macromolecular characteristics with fermentation in relation to the breadmaking capacity. (A) Sun-dried AM 206-5 native starches (dark blue), 30-days fermented (clear blue); (B) oven-dried AM 206-5 native starches (dark red), 30-days fermented (clear red); (C) Cumbre3 native starches, 30-days fermented and sun-dried (dark and clear green) and Cumbre3 native starches, 30-days fermented and oven-dried (dark and clear pink); (D) 30-days fermented and sun-dried Cumbre3 starches (clear green) and HMC-1 starches (clear grey); (E) evolution of the weight-average molar mass (squares) and branching degree (circles) of AM 206-5 after sun drying (empty symbols) and oven drying (filled symbols) according to the fermentation time; and (F) evolution of the weight-average molar mass (empty squares) and dGapp (filled circles) of AM 206-5 after different fermentation times and sun drying according to the specific volume ν (breadmaking capacity). (G) Evolution of the weight-average molar mass (empty squares) and dGapp (filled circles) of starches containing amylose (Cumbre3 and HMC-1) before and after 30 days of fermentation and sun- and oven-drying according to the specific volume ν (breadmaking capacity). The branching degree obtained from the molar mass and radius of gyration data using the modified ABC model corrected for cassava amylopectin;34,47dGapp: apparent molecular density , with GW as the weight-average radius of gyration. For A to D, the thin and thick lines correspond to the elugrams and molar masses, respectively. The dotted and continuous lines in F represent the linear correlations of ν with the molar mass and dGapp, respectively (R2 = 0.91 and 0.93, respectively). The dotted and continuous lines in G represent the linear correlations of ν with the molar mass and dGapp, respectively (R2 = 0.76 and 0.76, respectively). Starches were obtained from the cassava harvested in 2011; Cumbre3 and HMC-1 starches were the same as studied in Maldonado Alvarado et al.,14 and the amylose content in native samples was HMC-1 (21.7%) > Cumbre3 (16.7%) > AM 206-5 (0%). | |
Table 3 Macromolecular characteristics of amylopectin from native and 30-day fermented starches and the corresponding baking expansion obtained by AF4-MALLS
Clone |
Fermentation |
Drying |
w × 107 (g mol−1) |
G (nm) |
d
Gapp (g/mol nm−3) |
BDmC (%) |
ν (cm3 g−1) |
w: weight-average molar mass; G: z-average radius of gyration; dGapp: apparent molecular density ( , with GW as the weight-average radius of gyration); BDmC: the branching degree obtained from Mw and RG data using the modified ABC model corrected for cassava amylopectin;34,47ν: the specific volume (breadmaking capacity). For all macromolecular characteristics, values were taken over the whole amylopectin peak, and standard deviations were about 5%. Cumbre3 and HMC-1 starches were the same as studied in Maldonado Alvarado et al.,14 and the corresponding ν were reported from that reference. |
AM 206-5 |
Native |
Sun |
44.0 |
267 |
9.4 |
5.3 |
16 |
AM 206-5 |
Native |
Oven |
40.7 |
261 |
9.4 |
5.3 |
11 |
AM 206-5 |
Fermented |
Sun |
9.9 |
156 |
15.1 |
7.3 |
20 |
AM 206-5 |
Fermented |
Oven |
20.2 |
202 |
12.4 |
6.5 |
19 |
Cumbre3 |
Native |
Sun |
22.2 |
211 |
11.4 |
6.0 |
3 |
Cumbre3 |
Native |
Oven |
19.4 |
205 |
12.2 |
6.4 |
2 |
Cumbre3 |
Fermented |
Sun |
6.3 |
141 |
16.8 |
7.6 |
10 |
Cumbre3 |
Fermented |
Oven |
15.7 |
184 |
13.6 |
7.0 |
4 |
HMC-1 |
Native |
Sun |
25.1 |
220 |
a.12.1 |
b.6.7 |
c.2 |
d.HMC-1 |
e.Native |
f.Oven |
g.33.9 |
h.256 |
i.11.0 |
j.6.3 |
k.1 |
l.HMC-1 |
m.Fermented |
n.Sun |
o.22.2 |
p.231 |
q.11.5 |
r.6.0 |
s.5 |
t.HMC-1 |
u.Fermented |
v.Oven |
w.22.2 |
x.217 |
y.12.0 |
z.6.4 |
aa.3 |
After fermentation, AM 206-5 and Cumbre3 showed significant modifications of AF4 elugrams and molar mass patterns, especially after sun-drying (Fig. 6A–C). The main population shifted towards a lower molecular size, and a new peak appeared for AM 206-5 [F,S], and a dramatic increase of the shoulder corresponding to amylose was observed for Cumbre3 (Fig. 5C). Moreover, the amylopectin
w decreased, and the apparent molecular density dGapp and branching degree BDmC determined from AF4-MALLS data showed higher values after fermentation for AM 206-5 and Cumbre3, especially after sun-drying (Table 3).34,47
Fermentation thus produced smaller and more branched macromolecules in line with the production of highly branched dextrins through amylopectin main chain scission.52,53 As ν increased after fermentation for AM 206-5 and Cumbre3, it could be related to the capacity of the production of highly branched dextrins. Moreover, and in line with this hypothesis, fermentation seemed to have no impact on the amylopectin macromolecular characteristics of HMC-1 which showed a poor ν performance (Fig. 6D and Table 3).
The
w of AM 206-5 amylopectin decreased and BDmC increased with fermentation time (Fig. 6E), with a more dramatic evolution after sun-drying. Actually, the UV exposure during sun-drying induced additional photodegradation of amylopectin favoured by the oxidation caused by fermentation,52 which is also observed here for Cumbre3 (Table 3). After 7 days of fermentation, the synergy of the two treatments appeared (Fig. 6E). The drastic modifications of amylopectin with this dual treatment were also highlighted by the lower molar mass distributions observed in Fig. 6A, which indicated an extension of the macromolecular conformation in solution. This behaviour could be the result of chain repulsion caused by the high chain oxidation produced by the synergetic action of lactic fermentation and photodegradation. The
w of native AM 206-5 amylopectin was the highest but was also the most drastically reduced after fermentation, particularly after sun-drying (Table 3); this could be due to the absence of amylose.
The v performance for sun-dried AM 206-5 showed linear correlations with
w, dGapp (Fig. 6F and Table 3) and the branching degree (results not shown): the ν is higher when the molar mass decreases and the amylopectin branching increases. As AM 206-5 represented the amylopectin component of wild type starches, it can be concluded that besides the amylose content, the production of low molar mass and highly branched dextrins is the determinant for starch baking performance. This hypothesis was confirmed by the linear correlations also observed between the v performance and
w and dGapp for starches containing amylose (Fig. 6G and Table 3).
Crystallinity and % of A type crystallites (Fig. 7) were not affected by the level of fermentation nor by the way of drying, as was expected from a previous report,52 but contrary to the observations of Qi et al.53 This demonstrated that amorphous lamellae as well as the starch semi-crystalline domains were degraded under the present conditions.
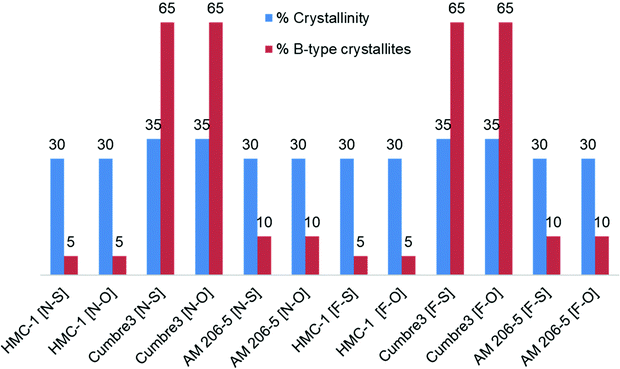 |
| Fig. 7 Crystallinity characteristics of native and 30-day fermented starches, oven-dried and sun-dried. [N-S]: native and sun-dried; [N-O]: native and oven-dried; [F-S]: fermented and sun-dried; [F-O]: fermented and oven-dried; % of crystallinity (blue); % of B-type crystallites (red). Starches were obtained from the cassava harvested in 2011; Cumbre3 and HMC-1 starches were the same as studied in Maldonado Alvarado et al.,14 and the amylose content in native samples was HMC-1 (21.7%) > Cumbre3 (16.7%) > AM 206-5 (0%). | |
However, Cumbre3 mainly exhibited B-type crystallites (65%), whereas AM 206-5 and HMC-1 exhibited a majority of A-type crystals (90–95%), and the crystallinity of all starches were not significantly different (Fig. 7). B-type crystals, which are loosely packed, are believed to be more susceptible to oxidation; the better expansion properties of fermented Cumbre3 after sun-drying compared to HMC-1 might thus be due to the combination of a lower amylose content (HMC-1 (21.7%) > Cumbre3 (16.7%))14 and higher macromolecular degradation (Fig. 6C and D) favored by the crystalline type of Cumbre3. The difference of expansion behavior between Cumbre3 and HMC-1 after sun-drying does not seem to be linked to a difference in the fermentation extent in Cumbre3 and HMC-1. Indeed, modification of the macromolecular characteristics of starches from Cumbre3 and HMC-1 has been shown to be negligible after 30 days fermentation and oven-drying (see above, Fig. 6C and D and Table 3). Nevertheless, the higher value of the molar mass of native HMC-1 and consequently of its residual molar mass after 30 days fermentation (Table 3) might also play a role in its weaker expansion properties.
4. Conclusions
Several conclusions can be drawn from this study. The effect of harvest years on ν was strong, suggesting that environmental conditions affect not only the fermentation process but also the quality of native starches. There was a clear synergism between fermentation and sun-drying to achieve high expansion capacities in wild-type starches, in agreement with previous studies.14 In contrast, oven-drying did not confer high expansion capacity after fermentation (except for the waxy starch). One of the challenges of fermentation is that the best duration of the process is difficult to define a priori, with drastic variations among years and genotypes. Finally, RVA data confirmed that fermentation reduces viscosity.
Macromolecular information shows that the breadmaking potential is mainly determined by the number of linear chains and is favored by fermentation and sun-drying through a reduction in amylopectin molar mass and the production of macromolecules with increased branching degree. Some of these characteristics, together with the absence of amylose, are outstanding in the case of waxy cassava and explain its excellent breadmaking potential.
The most important conclusion is the outstanding expansion performance of amylose-free starches. The most economical version of this starch (native and oven-dried) offered obvious advantages over the more processed (fermented and sun-dried) alternatives from wild-type genotypes. Waxy cassava starch, therefore, can emerge as a less-expensive, gluten-free, clean label, and neutral taste alternative for baked and expanded products.
Author contributions
D. Dufour and H. Ceballos: conceptualization, methodology, validation, formal analysis, investigation, resources, data curation, writing – original draft, writing – review and editing, visualization, supervision, project administration, and funding acquisition. A. Rolland-Sabaté: writing – original draft, investigation, methodology, formal analysis, visualization, validation, and writing – review and editing. T. Tran: methodology, validation, investigation, writing – original draft, and writing – review and editing. J. L. Moreno Alzate: validation, investigation and visualization. H. Mina Cordoba, J. L. Luna Melendez and M. Pizarro: validation and investigation. S. Guilois-Dubois: investigation, methodology, and writing – review and editing. T. Sánchez and J. Belalcazar: conceptualization, methodology, validation, investigation and resources. N. Morante: investigation and resources. M. A. Moreno Santander: conceptualization, methodology, investigation and resources. G. Vélez-Hernández: methodology, investigation and resources.
Abbreviations
RTB | Roots, tubers and bananas |
ν
| Specific volume |
[N] | Native |
[F] | Fermented |
[O] | Oven-dried |
[S] | Sun-dried |
[N,O] | Native, oven-dried |
[N,S] | Native, sun-dried |
[F,O] | Fermented, oven-dried |
[F,S] | Fermented, sun-dried |
(PT) | Pasting temperature |
(Pt) | Pasting time |
(PV) | Peak viscosity |
(HPV) | Holding strength |
(FV) | Final viscosity |
(BD) | Breakdown |
(SB) | Setback |
AF4 | Asymmetrical flow field flow fractionation |
MALLS | Multi-angle laser light scattering |
M
w
| Weight-average molar mass |
G
|
z-Average radius of gyration |
d
Gapp
| Apparent molecular density 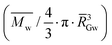 |
BDmC | Branching degree |
Conflicts of interest
There is no conflict to declare.
Acknowledgements
The present research was carried out within the framework of a Memorandum of Understanding signed between CIRAD: French Agricultural Research Centre for International Development, Montpellier, France and ABC: The Alliance of Bioversity International and the International Center for Tropical Agriculture (CIAT), formerly CIAT, Cali, Colombia. The authors thank Max Reynes, Antoine Collignan, Dominique Pallet in charge of the agreement at CIRAD, Joe Thome and Clair Hershey at CIAT for their administrative support. Financial support from the authors’ respective organizations (CIAT, CIRAD and INRAe) made the reported research possible. CIRAD has contributed financially toward the salaries and benefits of the French food scientist researchers posted at CIAT, Cali, Colombia. The authors thank Bruno Pontoire for the realization of the crystallinity analysis within UR1268 Biopolymères Interactions Assemblages, INRAe, F-44300 Nantes, France. The research was carried out within the framework of the CGIAR Research Program on Roots, Tubers and Bananas – RTB (https://www.cgiar.org/funders/). RTB is supported by the CGIAR Trust Fund contributors. The original manuscript underwent significant improvements suggested during the review process. The authors express their appreciation to the reviewers for their effort and help.
References
- D. Dufour, C. Hershey, B. R. Hamaker and J. Lorenzen, Integrating end-user preferences into breeding programmes for roots, tubers and bananas, Int. J. Food Sci. Technol., 2021, 56, 1071–1075, DOI:10.1111/ijfs.14911.
- S. Li, Y. Cui, Y. Zhou, Z. Luo, J. Liu and M. Zhao, The industrial applications of cassava: current status, opportunities and prospects, J. Sci. Food Agric., 2017, 97(8), 2282–2290, DOI:10.1002/jsfa.8287.
- J. Waterschoot, S. V. Gomand, E. Fierens and J. A. Delcour, Production, structure, physicochemical and functional properties of maize, cassava, wheat, potato and rice starches, Starch/Staerke, 2015, 67, 14–29, DOI:10.1002/star.201300238.
- A. B. Abass, W. Awoyale, B. Alenkhe, N. Malu, B. W. Asiru, V. Manyong and N. Sanginga, Can food technology innovation change the status of a food security crop? A review of cassava transformation into “bread” in Africa, Food Rev. Int., 2018, 34(1), 87–102, DOI:10.1080/87559129.2016.1239207(2018).
- S. Jensen, L. H. Skibsted, U. Kidmose and A. K. Thybo, Addition of cassava flours in bread-making: Sensory and textural evaluation, LWT – Food Sci. Technol., 2015, 60(1), 292–299, DOI:10.1016/J.LWT.2014.08.037.
- G. J. Ahlborn, O. A. Pike, S. B. Hendrix, W. M. Hess and C. S. Huber, Sensory, Mechanical, and Microscopic Evaluation of Staling in Low-Protein and Gluten-Free Breads, Cereal Chem., 2005, 82, 328–335, DOI:10.1094/CC-82-0328.
- E. Zannini, W. Kingston, E. K. Arendt and D. M. Waters, Technological challenges and strategies for developing low-protein/protein-free cereal foods for specific dietary management, Food Res. Int., 2013, 54(1), 935–950, DOI:10.1016/j.foodres.2013.03.001.
- A. Pasqualone, F. Caponio, C. Summo, V. M. Paradiso, G. Bottega and M. A. Pagani, Gluten-free bread making trials from cassava (Manihot esculenta Crantz) flour and sensory evaluation of the final product, Int. J. Food Prop., 2010, 13, 562–573, DOI:10.1080/10942910802713172.
- S. W. Horstmann, K. M. Lynch and E. K. Arendt, Starch Characteristics Linked to Gluten-Free Products, Foods, 2017, 6(4), 29, DOI:10.3390/foods6040029.
- T. Sigüenza-Andrés, C. Gallego and M. Gómez, Can cassava improve the quality of gluten free breads?, LWT – Food Sci. Technol., 2021, 149, e111923, DOI:10.1016/j.lwt.2021.111923.
- S. Punia Bangar, W. S. Whiteside, A. Singh, F. Özogul, A. Gupta and S. K. Gahlawat, Properties, preparation methods, and application of sour starches in the food, Trends Food Sci. Technol., 2022, 121, 44–58, DOI:10.1016/j.tifs.2022.01.029.
- C. Mestres and X. Rouau, Influence of natural fermentation and drying conditions on the physicochemical characteristics of cassava starch, J. Sci. Food Agric., 1997, 74(2), 147–155, DOI:10.1002/(SICI)1097-0010(199706)74:2<147::AID-JSFA781>3.0.CO;2-J.
- C. S. Cheow, Z. Y. Kyaw, N. K. Howell and M. H. Dzulkifly, Relationship between physicochemical properties of starches and expansion of fish cracker ‘Keropok’, J. Food Qual., 2004, 27(1), 1–12, DOI:10.1111/j.1745-4557.2004.tb00633.x.
- P. Maldonado Alvarado, L. Grosmaire, D. Dufour, A. Giraldo Toro, T. Sánchez, F. Calle, A. M. Moreno Santander, H. Ceballos, J. L. Delarbre and T. Tran, Combined effect of fermentation, sun-drying and genotype on breadmaking ability of sour cassava starch, Carbohydr. Polym., 2013, 98(1), 1137–1146, DOI:10.1016/j.carbpol.2013.07.012.
- A. C. Bertolini, C. Mestres and P. Colonna, Rheological Properties of Acidified and UV-Irradiated Starches, Starch/Staerke, 2000, 52, 340–344, DOI:10.1002/1521-379X(200010)52:10<340::AID-STAR340>3.0.CO;2-H.
- P. S. Hornung, C. Soltovski de Oliveira, M. Lazzarotto, S. Rosa da Silveira Lazzarotto and E. Schnitzler, Investigation of the photo-oxidation of cassava starch granules: Thermal, rheological and structural behavior, J. Therm. Anal. Calorim., 2016, 123(3), 2129–2137, DOI:10.1007/s10973-015-4706-x.
- Q. Qi, Y. Hong, Y. Zhang, Z. Gu, L. Cheng, Z. Li and C. Li, Combinatorial effect of fermentation and drying on the relationship between the structure and expansion properties of tapioca starch and potato starch, Int. J. Biol. Macromol., 2020, 145, 965–973, DOI:10.1016/j.ijbiomac.2019.09.187.
- S. Sumardiono, B. Jos, I. Pudjihastuti, R. J. Sari, W. D. N. Kumala and H. Cahyono, Effect of chemical modification, drying method, and drying temperature on baking expansion and the physicochemical properties of cassava starch, J. Food Process. Preserv., 2022, 46, e16111, DOI:10.1111/jfpp.16111.
- N. Vatanasuchart, O. Naivikul, S. Charoenrein and K. Sriroth, Molecular properties of cassava starch modified with different UV irradiations to enhance baking expansion, Carbohydr. Polym., 2005, 61(1), 80–87, DOI:10.1016/j.carbpol.2005.02.012.
- T. Paes Rodrigues dos Santos, M. Leonel, M. M. Mischan and C. Cabello, Study and application of photo-modified cassava starch with lactic acid and UV-C irradiation, LWT – Food Sci. Technol., 2021, 139, e110504, DOI:10.1016/j.lwt.2020.110504.
- K. Sangseethong, N. Termvejsayanon and K. Sriroth, Characterization of physicochemical properties of hypochlorite- and peroxide-oxidized cassava starches, Carbohydr. Polym., 2010, 82(2), 446–453, DOI:10.1016/j.carbpol.2010.05.003.
-
S. Sumardiono, I. Pudjihastuti, B. Yono, H. Hartanto and I. C. Sophiana, Combination process method of lactic acid hydrolysis and hydrogen peroxide oxidation for cassava starch modification, International Seminar on Fundamental and Application of Chemical Engineering (ISFAChE 2016), AIP Conference Proceedings 1840, 060006, 2017. DOI:10.1063/1.4982286.
- M. D. Matta Junior, N. Castanha, C. Boralli, P. dos Anjos, P. E. D. Augusto and S. B. S. Sarmento, Ozone technology as an alternative to fermentative processes to improve the oven-expansion properties of cassava starch, Food Res. Int., 2019, 123, 56–63, DOI:10.1016/j.foodres.2019.04.050.
- E. Mesa, K. Manjarres-Pinzon and E. Rodriguez-Sandoval, Gluten-free cheese bread from frozen dough: effect of modified cassava starch, Food Sci. Technol., 2019, 39(2), 654–661, DOI:10.1590/fst.30118.
- R. C. Dariva, D. Bucior, R. Colet, I. A. Fernandes, G. S. Hassemer, S. P. S. Miotto, R. L. Cansian, J. Zeni, G. T. Backes and E. Valduga, Techno-Functional Properties of Cheese Breads with Native and Modified Cassava Starch Produced in an Industrial System, Starch/Staerke, 2021, 73, 2000116, DOI:10.1002/star.202000116.
- B. C. Maniglia, N. Castanha, M. L. Rojas and P. E. D. Augusto, Emerging technologies to enhance starch performance, Curr. Opin. Food Sci., 2021, 39, 26–36, DOI:10.1016/j.cofs.2020.09.003.
- S. Park and Y. R. Kim, Clean label starch: production, physicochemical characteristics, and industrial applications, Food Sci. Biotechnol., 2021, 30, 1–17, DOI:10.1007/s10068-020-00834-3.
- N. Morante, H. Ceballos, T. Sánchez, A. Rolland-Sabaté, F. Calle, C. Hershey, O. Gibert and D. Dufour, Discovery of new spontaneous sources of amylose-free cassava starch and analysis of their structure and techno-functional properties, Food Hydrocolloids, 2016, 56, 383–395, DOI:10.1016/j.foodhyd.2015.12.025.
- H. Ceballos, C. Hershey, C. Iglesias and X. Zhang, Fifty years of a public cassava breeding program: evolution of breeding objectives, methods, and decision-making processes, Theor. Appl. Genet., 2021, 134, 2335–2353, DOI:10.1007/s00122-021-03852-9.
- A. Malik, P. Kongsil, V. A. Nguyen, W. J. Ou, Sholihin, P. Srean, M. N. Sheela, L. A. Becerra Lopez-Lavalle, Y. Utsumi, C. Lu, P. Kittipadakul, H. H. Nguyen, H. Ceballos, T. H. Nguyen, M. S. Gomez, P. Aiemnaka, R. Labarta, S. Chen, S. Amawan, S. Sok, L. Youabee, M. Seki, H. Tokunaga, W. Q. Wang, K. M. Li, H. A. Nguyen, V. D. Nguyen, L. H. Ham and M. Ishitani, Cassava breeding and agronomy in Asia: 50 years of history and future directions, Breed. Sci., 2020, 70, 145–166, DOI:10.1270/jsbbs.18180.
- C. D. do Carmo, M. B. E. Sousa, A. C. Brito and E. J. de Oliveira, Genome-wide association studies for waxy starch in cassava, Euphytica, 2020, 216, e82, DOI:10.1007/s10681-020-02615-9.
- A. Pulido Díaz, D. Lourdin, G. Della Valle, A. Fernández Quintero, H. Ceballos, T. Tran and D. Dufour, Thermomechanical characterization of an amylose-free starch extracted from cassava (Manihot esculenta, Crantz), Carbohydr. Polym., 2017, 157, 1777–1784, DOI:10.1016/j.carbpol.2016.11.058.
- A. Rolland-Sabaté, T. Sánchez, A. Buléon, P. Colonna, B. Jaillais, H. Ceballos and D. Dufour, Structural characterization of novel cassava starches with low and high amylose contents in comparison with other commercial sources, Food Hydrocolloids, 2012, 27(1), 161–174, DOI:10.1016/j.foodhyd.2011.07.008.
- A. Rolland-Sabaté, T. Sánchez, A. Buléon, P. Colonna, H. Ceballos, S. S. Zhao, P. Zhang and D. Dufour, Molecular and supra-molecular structure of waxy starches developed from cassava (Manihot esculenta Crantz), Carbohydr. Polym., 2013, 92(2), 1451–1462, DOI:10.1016/j.carbpol.2012.10.048.
- T. Sánchez, D. Dufour, I. X. Moreno and H. Ceballos, Comparison of Pasting and Gel Stabilities of Waxy and Normal Starches from Potato, Maize, and Rice with Those of a Novel Waxy Cassava Starch under Thermal, Chemical, and Mechanical Stress, J. Agric. Food Chem., 2010, 58, 5093–5099, DOI:10.1021/jf1001606.
- R. Toae, K. Sriroth, C. Rojanaridpiched, V. Vichukit, S. Chotineeranat, R. Wansuksri, P. Chatakanonda and K. Piyachomkwan, Outstanding Characteristics of Thai Non-GM Bred Waxy Cassava Starches Compared with Normal Cassava Starch, Waxy Cereal Starches and Stabilized Cassava Starches, Plants, 2019, 8(11), e447, DOI:10.3390/plants8110447.
- S. S. Zhao, D. Dufour, T. Sánchez, H. Ceballos and P. Zhang, Development of waxy cassava with different biological and physico-chemical characteristics of starches for industrial applications, Biotechnol. Bioeng., 2011, 108, 1925–1935, DOI:10.1002/bit.23120.
- W. Weil, R. C. Weil, S. Keawsompong, K. Sriroth, P. A. Seib and Y. C. Shi, Pyrodextrin from waxy and normal tapioca starches: Physicochemical properties, Food Hydrocolloids, 2020, 104, e105745, DOI:10.1016/j.foodhyd.2020.105745.
- W. Weil, R. C. Weil, S. Keawsompong, K. Sriroth, P. A. Seib and Y. C. Shi, Pyrodextrins from waxy and normal tapioca starches: Molecular structure and in vitro digestibility, Carbohydr. Polym., 2021, 252, 117140, DOI:10.1016/j.carbpol.2020.117140.
- S. V. Gomand, L. Lamberts, R. G. F. Visser and J. A. Delcour, Physicochemical properties of potato and cassava starches and their mutants in relation to their structural properties, Food Hydrocolloids, 2010, 24(4), 424–433, DOI:10.1016/j.foodhyd.2009.11.009.
- C. F. Hsieh, W. Liu, J. K. Whaley and Y. C. Shi, Structure and functional properties of waxy starches, Food Hydrocolloids, 2019, 94, 238–254, DOI:10.1016/j.foodhyd.2019.03.026.
- C. F. Hsieh, W. Liu, J. K. Whaley and Y. C. Shi, Structure, properties, and potential applications of waxy tapioca starches - A review, Trends Food Sci. Technol., 2019, 83, 225–234, DOI:10.1016/j.tifs.2018.11.022.
- T. Sánchez, E. Salcedo, H. Ceballos, D. Dufour, G. Mafla, N. Morante, F. Calle, J. C. Pérez, D. Debouck, G. Jaramillo and I. X. Moreno, Screening of starch quality traits in cassava (Manihot esculenta Crantz), Starch/Staerke, 2009, 61(1), 12–19, DOI:10.1002/star.200800058 ; [Erratum: Starch/Stärke, 61(5), 310 (2009)]. https://doi.org/10.1002/star.200990027.
-
AACC International (2001), Approved Methods of Analysis, 11th Ed. Method 10-05.01, Guidelines for Measurement of Volume by Rapeseed Displacement. First approval October 17, 2001. Cereals & Grains Association, St. Paul, MN, USA. https://www.cerealsgrains.org/resources/Methods/Pages/10BakingQuality.aspx.
- D. Dufour, O. Gibert, A. Giraldo, T. Sánchez, M. Reynes, J.-P. Pain, A. González, A. Fernández and A. Díaz, Differentiation between Cooking Bananas and Dessert Bananas. 2. Thermal and Functional Characterization of Cultivated Colombian Musaceae (Musa sp.), J. Agric. Food Chem., 2009, 57, 7870–7876, DOI:10.1021/jf900235a.
- A. Rolland-Sabaté, P. Colonna, G. Mendez-Montealvo and V. Planchot, Branching features of amylopectins and glycogen determined by asymmetrical flow field flow fractionation coupled with multiangle laser light scattering, Biomacromolecules, 2007, 8, 2520–2532, DOI:10.1021/bm070024z.
- A. Rolland-Sabaté, S. Guilois, B. Jaillais and P. Colonna, Molecular size and mass distributions of native starches using complementary separation methods: Asymmetrical Flow Field Flow Fractionation (A4F) and Hydrodynamic and Size-Exclusion Chromatography (HDC-SEC), Anal. Bioanal. Chem., 2011, 399, 1493–1505, DOI:10.1007/s00216-010-4208-4.
- A. Karlström, J. Belalcazar, T. Sánchez, J. I. Lenis, J. L. Moreno, M. Pizarro, J. Ricci, D. Dufour, T. Tran and H. Ceballos, Impact of Environment and Genotype-by-Environment Interaction on Functional Properties of Amylose-Free and Wildtype Cassava Starches, Starch/Staerke, 2019, 71, 1700278, DOI:10.1002/star.201700278.
- B. Gu, Q. Yao, K. Li and S. Chen, Change in physicochemical traits of cassava roots and starches associated with genotypes and environmental factors, Starch/Staerke, 2013, 65, 253–263, DOI:10.1002/star.201200028.
- K. Sriroth, V. Santisopasri, C. Petchalanuwat, K. Kurotjanawong, K. Piyachomkwan and C. G. Oates, Cassava starch granule structure–function properties: influence of time and conditions at harvest on four cultivars of cassava starch, Carbohydr. Polym., 1999, 38(2), 161–170, DOI:10.1016/S0144-8617(98)00117-9.
- E. Nowak, G. Khachatryan and A. Wisła-Świder, Structural changes of different starches illuminated with linearly polarised visible light, Food Chem., 2021, 344, 128693, DOI:10.1016/j.foodchem.2020.128693.
- A. C. Bertolini, C. Mestres, J. Raffi, A. Buléon, D. Lerner and P. Colonna, Photodegradation of cassava and corn starches, J. Agric. Food Chem., 2001, 49(2), 675–682, DOI:10.1021/jf0010174.
- Q. Qi, Y. Hong, Y. Zhang, Z. Gu, L. Cheng, Z. Li and C. Li, Effect of cassava starch structure on scalding of dough and baking expansion ability, Food Chem., 2021, 352, e129350, DOI:10.1016/j.foodchem.2021.129350.
|
This journal is © The Royal Society of Chemistry 2022 |
Click here to see how this site uses Cookies. View our privacy policy here.