Impact of nitrite on the formation of trichloronitromethane during the UV-LED/chlorine process†
Received
24th June 2021
, Accepted 16th September 2021
First published on 25th October 2021
Abstract
A UV-LED at a fixed wavelength is increasingly employed in the UV/chlorine process to degrade contaminants of emerging concern. However, degradation of contaminants and formation of disinfection by-products (DBPs) in post-chlorination would be significantly altered in the presence of nitrite because of the generation of reactive nitrogen species (RNS). As nitrite has a maximum absorption at ∼350 nm, its impact on the performance of the UV-LED365/chlorine process and subsequent formation of DBPs was investigated. The presence of nitrite in the UV365 irradiation process accelerated the degradation of bisphenol A (BPA), while slightly inhibiting the degradation of BPA in the UV365/chlorine process; nitrite in UV365 and UV365/chlorine processes resulted in the non-negligible formation of trichloronitromethane (TCNM). The concentration of generated DBPs and the corresponding cytotoxicity and genotoxicity all changed because of the presence of nitrite; the concentrations of nitrite, pH, and UV fluence were important factors for the formation of TCNM during the UV365/chlorine/nitrite process. Therefore, the impact of nitrite on the TCNM formation in the UV-LED365/chlorine process is worth noting.
Water impact
When the UV-LED365/chlorine process is used as the pretreatment of drinking water, the presence of nitrite will affect the removal of pollutants and the generation of DBPs in the subsequent chlorine disinfection. Comparing UV-LED365 and UV-LED365/chlorine pretreatment processes, the UV-LED365/chlorine process significantly enhances the formation of TCNM and TCM and inhibits the degradation of BPA.
|
1. Introduction
Since the discovery and characterization of trihalomethanes (THMs) as disinfection by-products (DBPs) in the 1970s, numerous researchers have focused on the formation and control of DBPs in the following decades.1,2 DBPs are typically generated from the reactions between disinfectants (e.g. chlorine, monochloramine, and chlorine dioxide) and natural/anthropogenic organic matter, bromide, and iodide,3–7 which are inevitable in drinking water because of the necessity of disinfection processes. To date, countless DBPs with cytotoxicity, carcinogenicity, and genotoxicity have been recognized in drinking water, while only a small fraction is regulated by environmental protection departments around the world.8,9 DBPs are generally made up of carbonaceous DBPs (C-DBPs, e.g. THMs) and nitrogenous DBPs (N-DBPs).4,10,11 Among them, concentrations of N-DBPs including haloacetamides (HAMs), halonitromethanes (HNMs), and haloacetonitriles (HANs) detected in drinking water were about 10–15 μg L−1, which are much lower than those of regulated C-DBPs (i.e. THMs and haloacetic acids); however, genotoxicity and cytotoxicity of N-DBPs are orders of magnitude higher than those of C-DBPs.12 Thus, formation and control of N-DBPs is always an important issue for drinking water.
Recently, advanced oxidation processes (AOPs) especially UV-based AOPs have been frequently applied as pretreatment processes to control the formation of DBPs in subsequent disinfection.13–15 Among various UV-AOPs, enhanced research in the UV/chlorine process brought a promising alternative to upgrade water treatment plants due to the already existing chlorination process.16,17 Reactive chlorine species (RCS) and hydroxyl radicals (HO˙) generated from chlorine photolysis play important roles in the control of DBP formation. However, many studies reported that application of UV/chlorine pretreatment processes can lead to increasing yields of DBPs in post-chlorination: Xiang et al. found that generation of all detected DBPs in their research was promoted when the UV/chlorine process was applied to deal with β-cyclocitral.18 Guo et al. noticed that yields of N-DBPs were significantly enhanced in the UV/chlorine and post-chlorination processes.19 Similar phenomena were also observed in some other research.20,21 Therefore, more attention needs to be paid when the UV/chlorine process is used to control the formation of DBPs (e.g. determination of UV fluence and chlorine dosage).
Apart from the aforementioned phenomenon, the presence of nitrite during the UV/chlorine and post-chlorination processes is another important issue. In our previous studies, nitrite in the UV/persulfate process and ammonia in the UV/chlorine process both showed significant impacts on the formation of TCNM,22,23 because of the possible generation of reactive nitrogen species (RNS). RNS are generally produced via the reactions of nitrite and radicals or the photolysis of nitrite (eqn (1)–(5)).24,25 Reactions of RNS with organic compounds may lead to the increasing concentration of organic nitrogen, which is the typical precursor of N-DBPs. Besides, RNS and HO˙ generated from the photolysis of nitrite can also significantly impact the performance of the UV/chlorine process. However, it is worth noting that little is known about the impact of nitrite on the UV/chlorine and post-chlorination processes. Notably, nitrite is ubiquitous in groundwater and surface water, especially in agricultural runoffs. It was reported that nitrite can accumulate up to 2 mg L−1 (in terms of N) in cold seasons due to low activity of nitrite oxidizers,26 despite its instability compared to other inorganic nitrogen species (i.e. nitrate or ammonia). Calza et al. reported that photolysis of phenol was enhanced by four times in the presence of 10 μM nitrite (around 0.14 mg L−1 N).27 Some researchers observed that the presence of nitrite promoted/reduced the yields of DBPs during chlorination of drinking water,28,29 but nitrite was not deemed as a typical precursor of N-DBPs. Of note, nitrated compounds transformed from nitrite in the UV/chlorine process cannot be ignored.
| HOCl/OCl– + hv → HO˙/O˙− + Cl˙ | (1) |
| HO˙ + NO2− → OH− + NO2˙ | (2) |
| Cl˙ + NO2− → Cl– + NO2˙ | (3) |
| NO2− + hv → [NO2−]* → NO˙ + O˙− | (4) |
Furthermore, among UV wavelength bands, low-pressure (LP) mercury light of UV254 is the predominant light source used in drinking water treatment. LP-UV254 has obvious drawbacks of low quantum yields of peroxide, high energy demand, short lifespans and leaching of mercury.30,31 Therefore, UV light emitting diodes (UV-LEDs) appeared as potential alternatives for LP-UV, and can be manufactured at a fixed wavelength in the range of 210–400 nm.31,32 Some studies found that a longer UV wavelength showed better performance on degrading model pollutants in the UV/chlorine process,33,34 which provides a promising application prospect for UV-LEDs. There have also been many studies focusing on the performance of the UV-LED/chlorine process in recent years.35,36 In a previous study, Guo et al. found that nitrite has a negligible impact on DBP formation during the sequential use of UV and chlorine,37 indicating that photolysis of nitrite by UV254 is not significant. This is because the UV absorption maximum of nitrite occurs at the wavelength of ∼350 nm.38 Therefore, a UV-LED at the wavelength of 365 nm was chosen as the light source in this study to explore the formation of DBPs when nitrite is present in the UV-LED/chlorine process.
Overall, the main aims of this study are to (1) investigate the performance on the degradation of bisphenol A (BPA) by the UV and UV/chlorine processes at the wavelength of 365 nm, with/without the presence of nitrite; (2) clarify the formation mechanism of N-DBPs in the UV/chlorine process at the wavelength of 365 nm, in the presence of nitrite; (3) evaluate the impacts of irradiation time, solution pH, and nitrite concentration on the yields of DBPs at UV365 in the presence of nitrite.
2. Materials and methods
2.1 Chemicals
Chemicals used in this research were all obtained from a chemical company and ESI† Text S1 provides the detailed information.
2.2 Experimental procedures
All the experiments were conducted with UV irradiation using a UV-LED at 365 nm (Fig. S1†). The irradiation intensity was determined as 3.05 mW cm−2 using the ferrioxalate actinometer method. Pre-treatment in 50 mL of BPA-containing solution (20.0 mg L−1 NaClO (as Cl2)) with the designed concentration of nitrite was carried out in all the experiments. A 10 mM phosphate buffer solution was used for maintaining the experimental pH (6.0, 6.5 and 7.0 in this study). Upon the termination of the UV/chlorine process, the reaction solution with 20 mg L−1 disinfectant (as Cl2) was poured into a bottle without headspace and incubated at 25 °C for 24 hours in the dark. Subsequently, a sufficient amount of ascorbic acid was spiked into the bottle with no headspace to quench the residual disinfectant. The experiments were all repeated at least three times, and the error bars represent the standard deviation of the average values.
2.3 Analytical methods
HPLC (USA, Agilent, 1260) was used for the detection of BPA concentration. A Hach DR3900 (USA), an instrument that can measure various parameters of water quality, was used to find the concentration of chlorine. A combination of a purge and trap instrument (USA, Tekmar Lumin) with a GC-MS (USA, Agilent, 7890A/5975C) was used for analyzing and quantifying DBP (THMs and TCNM) yields and ESI† Text S2 provides the specific method. In addition, the theoretical toxicity values (by dividing the concentration of DBPs in each group by the concentration determined in the toxicological assay (i.e., LC50 value), and assuming that the toxicity is additive) of the detected DBPs were computed and ESI† Text S3 provides the specific information.39
3. Results and discussion
3.1 Degradation of BPA by the UV and UV/chlorine processes at a wavelength of 365 nm in the absence/presence of nitrite
To better understand the role of nitrite in the UV and UV/chlorine processes at a wavelength of 365 nm, we compared the evolution profiles of BPA in the absence/presence of nitrite in the aforementioned two processes. As shown in Fig. 1, results showed that UV365 showed insignificant degradation of BPA, while 65% of BPA was degraded by direct chlorination. On the other hand, up to 74% of BPA was degraded under the same UV intensity in the UV365/chlorine process, because of the abundant generation of HO˙ and RCS.40 The phenomenon indicated that free chlorine (i.e. OCl− and HOCl) can be efficiently decomposed by UV365 despite its relatively lower molar absorption coefficient at UV365.41 In addition, no more than 10% of BPA was degraded under the nitrite/chlorine system, which can be attributed to the fact that chlorine can be quickly consumed by nitrite.42 When 5.0 mg L−1 nitrite was added to the UV365 irradiation process, the removal of BPA was significantly enhanced to 69%, which is comparable to the UV365/chlorine process. The promotion of BPA degradation can be explained by the generated RNS and HO˙ from the photolysis of nitrite (eqn (4) and (5)), and nitrite has a maximum molar absorption coefficient at UV365.38 For the UV365/chlorine process, although 78% of BPA can be removed in the presence of nitrite, the degradation of BPA was generally inhibited during the process, especially in the first 15 min (Fig. 1). This can be attributed to the dual role of nitrite in the UV365/chlorine process: (1) nitrite can quench HO˙, RCS and chlorine because of the reducing ability of nitrite, leading to the decrease in BPA degradation; (2) nitrite can be photolyzed to generate radicals (i.e. RNS and HO˙) in the UV365/chlorine process,43 leading to the increase in BPA degradation. The dual roles of nitrite offset each other in the UV365/chlorine process.
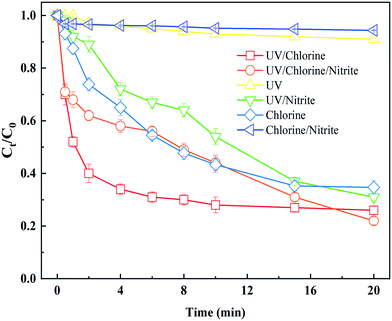 |
| Fig. 1 Evolution profiles of BPA in the UV365 and UV365/chlorine processes, in the absence/presence of nitrite. Experimental conditions: [Cl2]0 (in the UV/chlorine process) = 5 mg L−1, [BPA]0 = 5.0 mg L−1 (in terms of C), [NO2−]0 = 5 mg L−1 (in terms of N), pH = 7.0, [phosphate buffer] = 10 mM, irradiation time = 20 min, UV intensity = 3.05 mW cm−2. | |
3.2 Formation of DBPs in the UV and UV/chlorine processes at a wavelength of 365 nm in the presence of nitrite
As discussed above, the presence of nitrite (5 mg L−1) did not weaken the degradation of BPA in the UV365 and UV365/chlorine processes. However, the involvement of RNS in the degradation of BPA may result in the generation of nitrated compounds44 and subsequent formation of HNMs.22,23 Therefore, the UV and UV/chlorine processes were used to treat BPA-containing water (in the presence of 5 mg L−1 nitrite-N) at a wavelength of 365 nm, and the formation mechanism of DBPs in post-chlorination will be discussed in this section (Fig. 2). As shown in Fig. 2a, TCM was the only detected DBP in the chlorination of BPA in the absence/presence of nitrite, and TCNM appeared when the UV365 and UV365/chlorine processes were used as the pretreatment processes. Specifically, the direct chlorination of BPA in the absence and presence of 5 mg L−1 nitrite-N can respectively generate 66.72 and 1.91 μg L−1 TCM. This is because that chlorine prefers to react with nitrite at a molar ratio of 1
:
1, compared to organic compounds, leading to the low yield of TCM in the presence of nitrite. Moreover, when UV365 was applied to treat BPA-containing water before chlorination, 11.36 μg L−1 TCM and 0.82 μg L−1 TCNM were generated. As the concentration of nitrite was stable during UV photolysis (data not shown), the increased concentration of TCM and TCNM could be attributed to the transformed compounds of BPA with lower molecular weights, which more readily react with chlorine to form chlorinated DBPs. At last, we observed the formation of 88.23 μg L−1 TCM and 8.72 μg L−1 TCNM from BPA in the UV365/chlorine and post-chlorination processes. The increased formation of DBPs was ascribed to the UV365/chlorine process having a stronger ability to transform BPA compared to UV365 irradiation (Fig. 1). 8.72 μg L−1 TCNM can be attributed to the large formation of RNS in the UV365/chlorine/nitrite process, which comes from the photolysis of nitrite and the reactions of nitrite with HO˙ (or RCS). Of note, although the concentrations of detected DBPs in group A were much higher than those in group C, their toxicity values were comparable, especially the genotoxicity (Fig. 2b). Therefore, the presence of nitrite in the UV365 and UV365/chlorine processes needs more investigation to avoid the formation of TCNM.
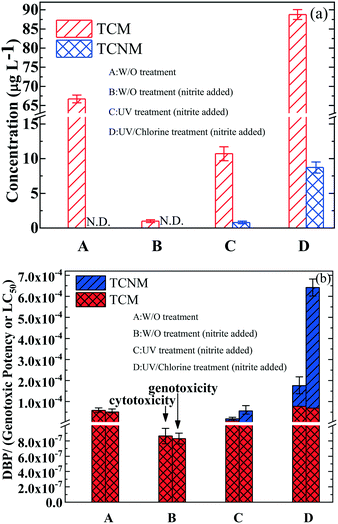 |
| Fig. 2 Impacts of different pretreatments on the formation of DBPs (a) and their toxicity (b) during the chlorination of BPA. Experimental conditions: [Cl2]0 (in the UV/chlorine process) = 20 mg L−1, [BPA]0 = 5.0 mg L−1 (in terms of C), [NO2−]0 = 5 mg L−1 (in terms of N), pH = 7.0, [phosphate buffer] = 10 mM, irradiation time = 20 min, UV intensity = 3.05 mW cm−2, [Cl2]0 (in post-chlorination) = 20 mg L−1. | |
3.3 Impact of nitrite concentration on the DBP formation in the UV/chlorine process at a wavelength of 365 nm
As discussed in section 3.2, the formation potential of TCNM from the chlorination of BPA pretreated by the UV365/chlorine process (in the presence of 5 mg L−1 nitrite-N) was an important issue. The impact of nitrite concentration on the DBP formation during the UV365/chlorine and post-chlorination processes was investigated. As presented in Fig. 3a, the formed concentration of TCM decreased from 323.54 to 88.23 μg L−1 with the increasing nitrite-N concentration from 0 to 5 mg L−1 at the wavelength of 365 nm, indicating that nitrite significantly impacted the radical chain reactions in the UV365/chlorine process.45 In contrast, the concentration of TCNM increased from 0 to 8.72 μg L−1 when the nitrite-N concentration increased from 0 to 5 mg L−1 in the UV365/chlorine process (Fig. 3a). This phenomenon occurs because more RNS can be generated when more nitrite was exposed to the UV365/chlorine process, further resulting in more generation of nitrated compounds. Besides, it can be found that the total concentrations of the detected DBPs (i.e., TCM and TCNM) kept decreasing with the increase of nitrite concentration in the investigated process. However, due to the much higher genotoxicity and cytotoxicity of TCNM than those of TCM, the genotoxicity and cytotoxicity of the generated DBPs showed a different trend from the concentrations. As shown in Fig. 3b, TCM is the main contributor to the total cytotoxicity of the generated DBPs, and TCNM mainly contributed to the total genotoxicity of the generated DBPs. Therefore, when the concentration of nitrite was increased in the UV365/chlorine process, the cytotoxicity of the generated DBPs showed a slight decrease, while the corresponding genotoxicity of the generated DBPs exhibited a significant enhancement.
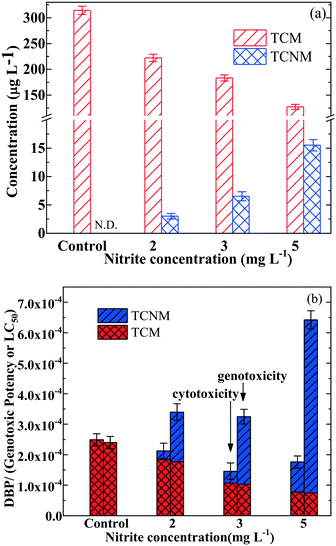 |
| Fig. 3 Impacts of nitrite concentration on the yields of DBPs (a) and their toxicity (b) generated from BPA treated by the UV365/chlorine process. Experimental conditions: [Cl2]0 (in the UV/chlorine process) = 20 mg L−1, [BPA]0 = 5.0 mg L−1 (in terms of C), [NO2−]0 = 0–5 mg L−1 (in terms of N), pH = 7.0, [phosphate buffer] = 10 mM, irradiation time = 20 min, UV intensity = 3.05 mW cm−2, [Cl2]0 (in post-chlorination) = 20 mg L−1. | |
3.4 Impact of solution pH on the DBP formation in the UV/chlorine process at a wavelength of 365 nm in the presence of nitrite
The impact of pH on the yields of TCNM and TCM from BPA treated by the UV365/chlorine process (in the presence of 5 mg L−1 nitrite-N) was evaluated, and the results are shown in Fig. 4. The formation of TCM generally increased from 72.51 to 88.23 μg L−1 as the pH values increased from 6.0 to 7.0 (Fig. 4a). Similar pH dependency on the TCM formation was documented in the chlorination of chlortoluron,19 ronidazole46,47 and humic acid48 after the UV/chlorine pretreatment process. On the other hand, the concentrations of formed TCNM were 10.37, 5.78, and 8.72 μg L−1 at pH 6.0, 6.5, and 7.0, respectively. As discussed above, the formation of TCNM was closely related to NO2˙, which could be generated from the reaction of nitrite with HO˙.49 Therefore, the pH-dependent formation of TCNM could be explained by the concentration of nitrite and HO˙ in the process. Of note, the concentration of HO˙ decreased with the increase of pH from 6.0 to 7.0 in the UV/chlorine process,50 while the concentration of nitrite increased with the increase of pH.51 Hence, the TCNM concentration reached the minimum value at pH 6.5. Besides, as presented in Fig. 4b, both the cytotoxicity and genotoxicity of the generated DBPs at different pH values showed the same trend as the concentrations of TCNM. The phenomenon occurs because that the genotoxic potency of TCNM is two orders of magnitude higher than that of TCM, and the LC50 of TCNM is one order of magnitude higher than that of TCM (Table S1†). Hence, TCNM made the major contribution to the total cytotoxicity and genotoxicity of the generated DBPs.
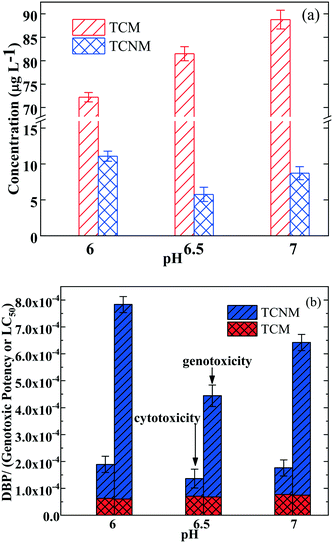 |
| Fig. 4 Impacts of pH on the yields of DBPs (a) and their toxicity (b) generated from BPA treated by the UV365/chlorine process. Experimental conditions: [Cl2]0 (in the UV/chlorine process) = 20 mg L−1, [BPA]0 = 5.0 mg L−1 (in terms of C), [NO2−]0 = 5 mg L−1 (in terms of N), pH = 6.0–7.0, [phosphate buffer] = 10 mM, irradiation time = 20 min, UV intensity = 3.05 mW cm−2, [Cl2]0 (in post-chlorination) = 20 mg L−1. | |
3.5 Impact of UV fluence on the DBP formation in the UV/chlorine process at a wavelength of 365 nm in the presence of nitrite
As the UV fluence significantly impacts the structures of the DBP precursors, we investigated its impact on the DBP formation in the UV365/chlorine process, in the presence of nitrite. Fig. 5 presents the concentration and toxicity of TCNM and TCM in the UV365/chlorine/nitrite process. As shown in Fig. 5a, the formation of TCNM and TCM showed similar trends under different UV fluence values: the concentrations of TCM were 55.97, 88.23, and 81.72 μg L−1 with the UV irradiation times at 10, 20, and 30 min (UV intensity = 3.05 mW cm−2). Meanwhile, the formation of TCNM increased from 6.93 to 8.72 μg L−1 with the irradiation time increasing from 10 to 20 min, and then reduced to 6.17 μg L−1 with the irradiation time further increasing to 30 min. The phenomena can be explained by: (1) when the irradiation time increased from 10 to 20 min, more RNS and nitrated compounds were generated, leading to higher production of TCNM; (2) when the irradiation time further increased to 30 min, the formed nitrated compounds were degraded, resulting in the decrease of TCNM production. Besides, the cytotoxicity and genotoxicity of the generated DBPs showed an identical trend to the concentrations (Fig. 5b). Therefore, increasing the UV fluence can decrease the risk of TCNM formation in the UV365/chlorine/nitrite process, via completely degrading the precursors.
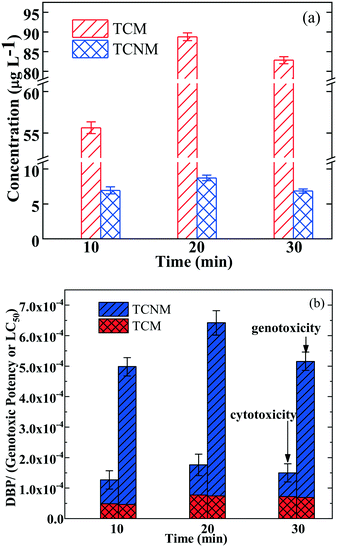 |
| Fig. 5 Impacts of irradiation time on the yields of DBPs (a) and their toxicity (b) generated from BPA treated by the UV365/chlorine process. Experimental conditions: [Cl2]0 (in the UV/chlorine process) = 20 mg L−1, [BPA]0 = 5.0 mg L−1 (in terms of C), [NO2−]0 = 5 mg L−1 (in terms of N), pH = 7.0, [phosphate buffer] = 10 mM, irradiation time = 0–30 min, UV intensity = 3.05 mW cm−2, [Cl2]0 (in post-chlorination) = 20 mg L−1. | |
4. Conclusions
The influences of nitrite on the degradation of BPA and the formation of TCNM in the UV365/chlorine process were investigated in this study, and the following conclusions were obtained:
(1) The presence of nitrite in the UV365 irradiation process significantly enhanced the BPA degradation, while the presence of nitrite in the UV365/chlorine process generally inhibited BPA degradation.
(2) Non-negligible formation of TCNM was observed from the chlorination of BPA in the presence of nitrite, when the UV365 or UV365/chlorine processes were used as the pretreatment processes, of which the UV365/chlorine process significantly enhanced the formation of both TCNM and TCM. Therefore, when nitrite is present in the treated water, UV-LED wavelengths of ∼350 nm should be avoided.
(3) Higher concentration of nitrite (0–5 mg-N L−1), lower pH (6.0–7.0), and moderate UV fluence (30.5–91.5 mJ cm−2) are favorable for the formation of TCNM in the UV365/chlorine and post-chlorination processes. The cytotoxicity and genotoxicity of the generated DBPs mainly depend on the formation of TCM and TCNM, respectively.
Conflicts of interest
There are no conflicts to declare.
Acknowledgements
This work was financially supported by the Natural Science Foundation of Hunan Province (2021JJ40066), the National Natural Science Foundation (51878257), the Changsha Municipal Natural Science Foundation (kq2007028) and the State Key Laboratory of Pollution Control and Resource Reuse Foundation (PCRRF20003).
References
- T. A. Bellar, J. J. Lichtenberg and R. C. Kroner, The occurrence of organohalides in chlorinated drinking waters, J. - Am. Water Works Assoc., 1974, 66, 703–706 CrossRef CAS.
- J. J. Rook, Formation of haloforms during chlorination of natural waters, Water Treat. Exam., 1974, 23, 234–243 Search PubMed.
- G. Hua, D. A. Reckhow and J. Kim, Effect of bromide and iodide ions on the formation and speciation of disinfection byproducts during chlorination, Environ. Sci. Technol., 2006, 40, 3050–3056 CrossRef CAS PubMed.
- I. Kristiana, D. Liew, R. K. Henderson, C. A. Joll and K. L. Linge, Formation and control of nitrogenous DBPs from Western Australian source waters: investigating the impacts of high nitrogen and bromide concentrations, J. Environ. Sci., 2017, 58, 102–115 CrossRef CAS.
- S. D. Richardson, M. J. Plewa, E. D. Wagner, R. Schoeny and D. M. DeMarini, Occurrence, genotoxicity, and carcinogenicity of regulated and emerging disinfection by-products in drinking water: a review and roadmap for research, Mutat. Res., Rev. Mutat. Res., 2007, 636, 178–242 CrossRef CAS.
- S. D. Richardson, Disinfection by-products and other emerging contaminants in drinking water, TrAC, Trends Anal. Chem., 2003, 22, 666–684 CrossRef CAS.
- D. M. White, D. S. Garland, J. Narr and C. R. Woolard, Natural organic matter and DBP formation potential in Alaskan water supplies, Water Res., 2003, 37, 939–947 CrossRef CAS PubMed.
- E. R. Blatchley III, D. Margetas and R. Duggirala, Copper catalysis in chloroform formation during water chlorination, Water Res., 2003, 37, 4385–4394 CrossRef.
-
S. Rieder, T. Hofstetter and U. Von Gunten, The new generation of disinfection byproducts (DBPs), 2007 Search PubMed.
- T. Bond, M. R. Templeton, N. H. M. Kamal, N. Graham and R. Kanda, Nitrogenous disinfection byproducts in English drinking water supply systems: occurrence, bromine substitution and correlation analysis, Water Res., 2015, 85, 85–94 CrossRef CAS PubMed.
- D. Liew, K. L. Linge and C. A. Joll, Formation of nitrogenous disinfection by-products in 10 chlorinated and chloraminated drinking water supply systems, Environ. Monit. Assess., 2016, 188, 518 CrossRef.
-
M. J. Plewa and E. D. Wagner, Mammalian cell cytotoxicity and genotoxicity of disinfection by-products, Water Research Foundation, Denver, CO, 2009, vol. 134 Search PubMed.
- W. Chu, N. Gao, D. Yin, S. W. Krasner and W. A. Mitch, Impact of UV/H2O2 pre-oxidation on the formation of haloacetamides and other nitrogenous disinfection byproducts during chlorination, Environ. Sci. Technol., 2014, 48, 12190–12198 CrossRef CAS PubMed.
- W. Chu, D. Li, N. Gao, M. R. Templeton, C. Tan and Y. Gao, The control of emerging haloacetamide DBP precursors with UV/persulfate treatment, Water Res., 2015, 72, 340–348 CrossRef CAS PubMed.
- L. Bu, S. Zhou, S. Zhu, Y. Wu, X. Duan, Z. Shi and D. D. Dionysiou, Insight into carbamazepine degradation by UV/monochloramine: Reaction mechanism, oxidation products, and DBPs formation, Water Res., 2018, 146, 288–297 CrossRef CAS PubMed.
- X. Duan, T. Sanan, A. de la Cruz, X. He, M. Kong and D. D. Dionysiou, Susceptibility of the algal toxin microcystin-LR to UV/chlorine process: Comparison with chlorination, Environ. Sci. Technol., 2018, 52, 8252–8262 CrossRef CAS.
- S. Zhou, W. Zhang, J. Sun, S. Zhu, K. Li, X. Meng, J. Luo, Z. Shi, D. Zhou and J. C. Crittenden, Oxidation mechanisms of the UV/free chlorine process: Kinetic modeling and quantitative structure activity relationships, Environ. Sci. Technol., 2019, 53, 4335–4345 CrossRef CAS PubMed.
- H. Xiang, Y. Shao, N. Gao, X. Lu, N. An and W. Chu, Removal of β-cyclocitral by UV/persulfate and UV/chlorine process: degradation kinetics
and DBPs formation, Chem. Eng. J., 2019, 122659 Search PubMed.
- Z.-B. Guo, Y.-L. Lin, B. Xu, H. Huang, T.-Y. Zhang, F.-X. Tian and N.-Y. Gao, Degradation of chlortoluron during UV irradiation and UV/chlorine processes and formation of disinfection by-products in sequential chlorination, Chem. Eng. J., 2016, 283, 412–419 CrossRef CAS.
- W.-L. Wang, X. Zhang, Q.-Y. Wu, Y. Du and H.-Y. Hu, Degradation of natural organic matter by UV/chlorine oxidation: molecular decomposition, formation of oxidation byproducts and cytotoxicity, Water Res., 2017, 124, 251–258 CrossRef CAS PubMed.
- H. Xiang, Y. Shao, N. Gao, X. Lu, N. An, C. Tan and Z. Zheng, Degradation of diuron by chlorination and UV/chlorine process: degradation kinetics and the formation of disinfection by-products, Sep. Purif. Technol., 2018, 202, 365–372 CrossRef CAS.
- L. Bu, J. Sun, Y. Wu, W. Zhang, X. Duan, S. Zhou, D. D. Dionysiou and J. C. Crittenden, Non-negligible risk of chloropicrin formation during chlorination with the UV/persulfate pretreatment process in the presence of low concentrations of nitrite, Water Res., 2020, 168, 115194 CrossRef CAS PubMed.
- S. Zhou, Y. Wu, S. Zhu, J. Sun, L. Bu and D. D. Dionysiou, Nitrogen conversion from ammonia to trichloronitromethane: Potential risk during UV/chlorine process, Water Res., 2020, 115508 CrossRef CAS.
- A. D. Shah, A. D. Dotson, K. G. Linden and W. A. Mitch, Impact of UV disinfection combined with chlorination/chloramination on the formation of halonitromethanes and haloacetonitriles in drinking water, Environ. Sci. Technol., 2011, 45, 3657–3664 CrossRef CAS.
- L. Bu, N. Zhu, C. Li, Y. Huang, M. Kong, X. Duan and D. D. Dionysiou, Susceptibility of atrazine photo-degradation in the presence of nitrate: Impact of wavelengths and significant role of reactive nitrogen species, J. Hazard. Mater., 2020, 121760 CrossRef CAS.
- J. Shan, J. Hu, S. S. Kaplan-Bekaroglu, H. Song and T. Karanfil, The effects of pH, bromide and nitrite on halonitromethane and trihalomethane formation from amino acids and amino sugars, Chemosphere, 2012, 86, 323–328 CrossRef CAS PubMed.
- P. Calza, D. Vione, A. Novelli, E. Pelizzetti and C. Minero, The role of nitrite and nitrate ions as photosensitizers in the phototransformation of phenolic compounds in seawater, Sci. Total Environ., 2012, 439, 67–75 CrossRef CAS PubMed.
- H. Hong, Y. Xiong, M. Ruan, F. Liao, H. Lin and Y. Liang, Factors affecting THMs, HAAs and HNMs formation of Jin Lan Reservoir water exposed to chlorine and monochloramine, Sci. Total Environ., 2013, 444, 196–204 CrossRef CAS PubMed.
- J. Hu, H. Song and T. Karanfil, Comparative analysis of halonitromethane and trihalomethane formation and speciation in drinking water: the effects of disinfectants, pH, bromide, and nitrite, Environ. Sci. Technol., 2009, 44, 794–799 CrossRef PubMed.
- G. Matafonova and V. Batoev, Recent advances in application of UV light-emitting diodes for degrading organic pollutants in water through advanced oxidation processes: a review, Water Res., 2018, 132, 177–189 CrossRef CAS.
- M. A. Würtele, T. Kolbe, M. Lipsz, A. Külberg, M. Weyers, M. Kneissl and M. Jekel, Application of GaN-based ultraviolet-C light emitting diodes–UV LEDs–for water disinfection, Water Res., 2011, 45, 1481–1489 CrossRef.
- J. Chen, S. Loeb and J.-H. Kim, LED revolution: fundamentals and prospects for UV disinfection applications, Environ. Sci.: Water Res. Technol., 2017, 3, 188–202 RSC.
- R. Yin, L. Ling and C. Shang, Wavelength-dependent chlorine photolysis and subsequent radical production using UV-LEDs as light sources, Water Res., 2018, 142, 452–458 CrossRef CAS PubMed.
- X.-Y. Zou, Y.-L. Lin, B. Xu, T.-Y. Zhang, C.-Y. Hu, T.-C. Cao, W.-H. Chu, Y. Pan and N.-Y. Gao, Enhanced ronidazole degradation by UV-LED/chlorine compared with conventional low-pressure UV/chlorine at neutral and alkaline pH values, Water Res., 2019, 160, 296–303 CrossRef CAS PubMed.
- Z.-C. Gao, Y.-L. Lin, B. Xu, Y. Xia, C.-Y. Hu, T.-C. Cao, X.-Y. Zou and N.-Y. Gao, Evaluating iopamidol degradation performance and potential dual-wavelength synergy by UV-LED irradiation and UV-LED/chlorine treatment, Chem. Eng. J., 2019, 360, 806–816 CrossRef CAS.
- M. Kwon, Y. Yoon, S. Kim, Y. Jung, T.-M. Hwang and J.-W. Kang, Removal of sulfamethoxazole, ibuprofen and nitrobenzene by UV and UV/chlorine processes: a comparative evaluation of 275 nm LED-UV and 254 nm LP-UV, Sci. Total Environ., 2018, 637, 1351–1357 CrossRef PubMed.
- Z.-B. Guo, Y.-L. Lin, B. Xu, C.-Y. Hu, H. Huang, T.-Y. Zhang, W.-H. Chu and N.-Y. Gao, Factors affecting THM, HAN and HNM formation during UV-chlor (am) ination of drinking water, Chem. Eng. J., 2016, 306, 1180–1188 CrossRef CAS.
- H. O. N. Tugaoen, S. Garcia-Segura, K. Hristovski and P. Westerhoff, Challenges in photocatalytic reduction of nitrate as a water treatment technology, Sci. Total Environ., 2017, 599, 1524–1551 CrossRef PubMed.
- C. Liu, M. S. Ersan, M. J. Plewa, G. Amy and T. Karanfil, Formation of regulated and unregulated disinfection byproducts during chlorination of algal organic matter extracted from freshwater and marine algae, Water Res., 2018, 142, 313–324 CrossRef CAS PubMed.
- J. Fang, Y. Fu and C. Shang, The Roles of Reactive Species in Micropollutant Degradation in the UV/Free Chlorine System, Environ. Sci. Technol., 2014, 48, 1859–1868 CrossRef CAS.
- D. M. Bulman, S. P. Mezyk and C. K. Remucal, The impact of pH and irradiation wavelength on the production of reactive oxidants during chlorine photolysis, Environ. Sci. Technol., 2019, 53, 4450–4459 CrossRef CAS PubMed.
- D. G. Wahman and G. E. Speitel, Relative Importance of Nitrite Oxidation by Hypochlorous Acid under Chloramination Conditions, Environ. Sci. Technol., 2012, 46, 6056–6064 CrossRef CAS PubMed.
- J. Mack and J. R. Bolton, Photochemistry of nitrite and nitrate in aqueous solution: a review, J. Photochem. Photobiol., A, 1999, 128, 1–13 CrossRef CAS.
- Y. Huang, M. Kong, D. Westerman, E. G. Xu, S. Coffin, K. H. Cochran, Y. Liu, S. D. Richardson, D. Schlenk and D. D. Dionysiou, Effects of HCO3− on degradation of toxic contaminants of emerging concern by UV/NO3−, Environ. Sci. Technol., 2018, 52, 12697–12707 CrossRef CAS.
- A. Kroflič, M. Grilc and I. Grgić, Unraveling pathways of guaiacol nitration in atmospheric waters: nitrite, a source of reactive nitronium ion in the atmosphere, Environ. Sci. Technol., 2015, 49, 9150–9158 CrossRef.
- Z. Wu, K. Guo, J. Fang, X. Yang, H. Xiao, S. Hou, X. Kong, C. Shang, X. Yang, F. Meng and L. Chen, Factors affecting the roles of reactive species in the degradation of micropollutants by the UV/chlorine process, Water Res., 2017, 126, 351–360 CrossRef CAS PubMed.
- L. Qin, Y.-L. Lin, B. Xu, C.-Y. Hu, F.-X. Tian, T.-Y. Zhang, W.-Q. Zhu, H. Huang and N.-Y. Gao, Kinetic models and pathways of ronidazole degradation by chlorination, UV irradiation and UV/chlorine processes, Water Res., 2014, 65, 271–281 CrossRef CAS PubMed.
- Z.-C. Gao, Y.-L. Lin, B. Xu, Y. Xia, C.-Y. Hu, T.-Y. Zhang, T.-C. Cao, W.-H. Chu and N.-Y. Gao, Effect of UV wavelength on humic acid degradation and disinfection by-product formation during the UV/chlorine process, Water Res., 2019, 154, 199–209 CrossRef CAS PubMed.
- S. Zhou, L. Li, Y. Wu, S. Zhu, N. Zhu, L. Bu and D. D. Dionysiou, UV365 induced elimination of contaminants of emerging concern in the presence of residual nitrite: Roles of reactive nitrogen species, Water Res., 2020, 178, 115829 CrossRef CAS.
- K. Guo, Z. Wu, C. Shang, B. Yao, S. Hou, X. Yang, W. Song and J. Fang, Radical Chemistry and Structural Relationships of PPCP Degradation by UV/Chlorine Treatment in Simulated Drinking Water, Environ. Sci. Technol., 2017, 51, 10431–10439 CrossRef CAS PubMed.
- J. Li and E. R. Blatchley Iii, UV Photodegradation of Inorganic Chloramines, Environ. Sci. Technol., 2009, 43, 60–65 CrossRef CAS PubMed.
Footnote |
† Electronic supplementary information (ESI) available. See DOI: 10.1039/d1ew00435b |
|
This journal is © The Royal Society of Chemistry 2022 |
Click here to see how this site uses Cookies. View our privacy policy here.