Gold nanoclusters embedded in antimicrobial keyboard covers: life cycle assessment and environmental sustainability†
Received
23rd November 2021
, Accepted 30th March 2022
First published on 31st March 2022
Abstract
Gold nanoparticles and nanoclusters can be used in a variety of sectors in different applications. Research on new synthesis methods and procedures for their integration into products is flourishing with positive results. This study focuses on the environmental impacts of synthesising cysteine-capped gold nanoclusters [Au25(Cys)18] and their integration in silicone keyboard covers. The final product presents an antibactericidal activity that could make it suitable for use in hospital wards to tackle the cross-transmission of pathogens. Life cycle assessment (LCA) methodology is used here to analyse and compare the environmental impacts of antimicrobial keyboard covers against current procedures for cleaning keyboards in hospitals using gloves and alcohol wipes (70%). Furthermore, results have been compared with the normalisation factors based on two reference systems: global emissions and carrying-capacity values. Whilst the first reference system indicates the contribution of a production system relative to the global emissions; the latter provides an indication of the absolute environmental sustainability of the system evaluated. This study shows that adopting an antimicrobial keyboard cover results in lower environmental impacts than using gloves and alcohol wipes, almost for all the impact categories considered.
Environmental significance
Life cycle assessment (LCA) methodology has been used in numerous studies aiming to address the environmental impacts of the synthesis of nanoparticles and their applications against conventional processes. Here we have conducted an LCA study on a new process for the production of gold nanoclusters. We have performed a hot spot analysis and compared the environmental performance against a product presenting the same function. Furthermore, we have undertaken a carrying capacity based normalisation to assess the environmental sustainability of the new product developed. This work provides both a transparent LCA study and an attempt to defining whether antimicrobial keyboard covers are an environmentally sustainable solution.
|
1 Introduction
In the past years, nanoparticles have attracted considerable interest due to their potential in several hot-topic fields,1–3 especially the healthcare sector, where they can be employed for detection and diagnostic screening,4,5 drug delivery,6,7 oncological treatments8–10 and as antimicrobial agents.11,12 As a result, research on their synthesis methods, applications and integration in products has flourished, and the first products embedding nanoparticles have been released on the market.
Studies on the environmental impacts, specifically based on the life cycle assessment (LCA) methodology,13–15 have been published for several types of nanoparticles: silver nanoparticles,16,17 carbon nanotubes,18,19 gold nanoparticles,20–22 and titanium dioxide nanoparticles;23–25 as well as for products embedding nanoparticles, such as T-shirts26 and socks.27
LCA is an essential tool to identify, analyse and understand how to tackle a significant part of the environmental issues linked to manufacturing, use and end-of-life of products and services. It is widely used in the decision-making process and even for policy and regulatory purposes.28–30 Moreover, because of the comparative nature of LCA, it is possible to determine the improvements required in products or production systems to get better environmental performances in relation with the state-of-art or other market competitors.24,31 Scientific literature about the application of LCA in the nanotechnology sector shows that the methodology has been employed to evaluate different synthesis routes,16,17,21,32,33 the feasibility of scaling out/up20 laboratory set-ups, to identify and explore the advantages of recycling specific chemicals,22 along with issues linked to utilisation of the products.26,27,34 It is undeniable that all these studies provide insightful information on the synthesis of nanoparticles and nano-enabled products with regards to the environmental impacts. However, to the authors' best knowledge, none of them was aimed at assessing the environmental sustainability of such like goods.
This can be done by implementing the concept of planetary boundaries. This concept was proposed by Rockström and co-authors in 2009,35 and later updated by Steffen and colleagues.36 It defines a “safe operating space for humanity based on the intrinsic biophysical processes that regulate the stability of the Earth system”.36 Rockström and co-authors identified 9 system's boundaries, each one representing the boundary between the safe operating space (below the boundary) and a buffer-area of uncertainty. The safe operating space is located downward the buffer area, which is delimitated upwards by a threshold or tipping point of a specific and essential Earth system process. In addition, Steffen and collaborators estimated the current situation of transgression (at 2015) of each of the identified system boundaries.36
Based on the planetary boundaries concept, in 2015 Bjørn and Hauschild37 proposed new LCA normalisation references, namely “carrying-capacity” normalisation references. These authors proposed normalising the LCA results by taking the Earth's carrying capacity for different environmental areas as a reference system. Estimation of normalisation references was based on the carrying-capacity, defined as the “maximum sustained environmental intervention a natural system can withstand without experiencing negative changes […] that are difficult or impossible to revert”.37 The proposed carrying-capacity normalisation references were developed in compliance with 6 of the 15 ILCD (International Life Cycle Data systems reference) midpoint impact categories,38,39 and specifically: climate change, ozone depletion potential, photochemical ozone formation, freshwater eutrophication, marine eutrophication, and freshwater ecotoxicity. Their application would allow a better interpretation of the LCA results, in terms of identifying, in absolute terms, the most affected impact categories. Furthermore, it would be possible to define whether a system or product is environmentally sustainable, or not.
In this study, we apply the LCA methodology and the carrying-capacity normalisation references proposed by Bjørn and Hauschild37 and later reviewed by Sala et al.,40 to assess the environmental impacts and sustainability of an antimicrobial product. Antimicrobial keyboard covers embedding gold nanoclusters41–43 have been developed with the purpose of avoiding cross infections in hospital wards. Photobacterial agents as methylene blue and crystal violet are promising candidates to be used in sterilising surfaces.44–46 When used together with zinc oxide, silver and gold nanoparticles (NPs), they present a combined and synergistic antibacterial effect. In the case of gold NPs, the size is a pivotal feature, indeed the greatest enhancing effect was given by gold NPs with a size ranging 2–20 nm.42 When gold nanoclusters (<2 nm), which are made of a small number of atoms are added to crystal violet impregnated polymer the photobactericidal activity is improved even under low intensity white light conditions (200–429 lux).42 This is an advantages compared with other type of nanoparticles (Ag, ZnO, TiO2) requiring intense white light conditions (>1300 lux) which makes difficult their employment in almost all the healthcare facilities, except for the operating table and other specific room.42 In addition, gold NPs are a good option compared with other types like silver and copper NPs, because gold is considered a safe material. Even though, we must point out that it is not clear yet if gold nanoparticles present toxicity effects.47 Therefore, the antimicrobial keyboard covers integrating gold nanoclusters appear to be a promising tool to be used to deal with the problem of cross-infections in the healthcare sector. As highlighted from a publication of FitzGerald and colleagues in 2013,48 when caregivers are not compliant with hand-hygiene standards, cross-transmissions of pathogens amongst patients can happen. These authors observed the number of times the hospital staff (nurses, doctors, pharmacists) were moving from a location to another (from patient to patient, from ward to ward, hospital bay to another one), how many times the staff were compliant with the hand-hygiene procedures and the number of times they touched the computer keyboards and equipment trolleys. The conclusions drawn from FitzGerald et al.49 were mainly two: firstly, the necessity to educate hospital personnel on the causes and risks of bacterial spread, and their awareness that it can happen even during low risk perceived activities. Secondly, hospitals should implement education and intervention programmes to lower the risk of cross-infections from surfaces (door handlers, ward computers, etc.). Some hospitals adopted cleaning procedures, encouraging the personnel to clean the surfaces and equipment keyboards with alcohol wipes (70%) while wearing PPE (personal protective equipment), specifically butyl or nitrile gloves.49–51 Others adopted keyboard covers such as the Medigenic keyboard covers,50,52 with an integrated alert system to communicate to the user when the keyboard needs to be cleaned. Antimicrobial keyboard covers may help in preventing cross-transmissions of pathogens and bacteria in hospitals, and help in preventing the frequent uses of disposable alcohol wipes and PPE during the cleaning of the keyboards.
In this paper, we present an attributional LCA study to evaluate, from an environmental perspective, the negative and positive impacts that antimicrobial keyboard covers may have. The study aims at assessing the system at a large scale; therefore, 2 scenarios of usage have been selected: use in the United Kingdom and at the European continent level. In addition, a comparison with the environmental burdens of employing alcohol wipes and nitrile gloves has been performed. Together with these analyses, results are compared with normalisation factors based on the annual environmental emissions in UK and Europe, and with the normalisation factors based on carrying-capacity (annual emissions budget).
2 Methods
2.1 Description of the system: synthesis of gold nanoclusters and preparation of antimicrobial keyboard cover
The system is comprised of 2 main foreground activities: synthesis of nanoparticles and swell–encapsulation–shrink process. During the swell–encapsulation–shrink process, the silicone keyboard cover absorbs a solution of gold nanoclusters and crystal violet.41 As explained in Huang and Hwang's papers41,42 gold nanoclusters enhance the bactericidal activity of the crystal violet. Cysteine-capped gold nanoclusters, formed by 25 atoms of gold and 18 cysteine molecules [Au25(Cys)18],53 are produced using a 2 meters tube-in-tube membrane contactor in an oil bath at 80 °C. An aqueous solution containing HAuCl4 and cysteine with a molar ratio 1
:
1.5 is prepared with the addition of NaOH (2 M).41 The mixture enters in contact with CO gas (500 kPa) by means of the contactor tube, made of polytetrafluoroethylene (PTFE) tubing (inner diameter: 3.2 mm) and Teflon AF-2400 tubing (inner diameter: 0.88 mm). After a residence time of roughly 3 minutes only gold nanoclusters [Au25(Cys)18] are synthetised.42 Then the solution is diluted to get a final concentration of 0.1 g per litre. To produce the antimicrobial keyboard cover, roughly a 4 litre photo-bactericidal solution acetone (50% v/v), DI water (40% v/v), aqueous [Au25(Cys)18] solution (10% v/v, 12 mM [Au25(Cys)18]) and crystal violet (800 ppm) is prepared. Then, the keyboard cover is immersed in the solution and left to soak in it for 24 hours, going through a swell–encapsulation–shrink process.42 Results from the UV-vis spectra of [Au25(Cys)18] and spectra from the X-ray photoelectron spectroscopy of the [Au25(Cys)18] encapsulated silicone sample, performed by Hwang and colleagues,42 shows that the gold nanoclusters were present on the silicone surface, as well as into the silicone matrix. Specifically, 33% of the 7.2 × 1015 [Au25(Cys)18] per ml in solution penetrated the polymer.
2.2 Functional unit
Functional unit (FU) has been defined based on the main assumption that antimicrobial keyboard covers (akcs) have the same daily antimicrobial activity of using alcohol wipes (70%) and a pair of nitrile rubber gloves every 6 hours. This because the unavailability of comparable data on the functions of both products.
Therefore, the adopted FU is defined as the number of akcs to satisfy the annual demand of the hospitals located in the United Kingdom and the European continent, respectively (see ESI,† Annex I). Therefore, the two reference flows are equal to 223
000 and 1.70 million antimicrobial keyboard covers.
The total number of keyboards in hospitals has been estimated on the basis on the number of doctors and healthcare employees (such as nurses) in the UK54–56 and Europe57 in 2018, assuming a ratio of 2
:
1 between doctors and number of keyboards (computers and equipment requiring keyboards), as reported in Table 1, and explained in detail in the ESI,† Annex I.
Table 1 Estimated number of physicians and nurses in both the United Kingdom and in Europe. Quantities of gold incorporated in each antimicrobial keyboard cover and amount of product per year
|
United Kingdom |
Europe |
Number of physicians (doctors) in hospitals |
1.44 × 105 |
1.26 × 106 |
Number of nurses in hospitals |
3.12 × 105 |
2.35 × 106 |
Grams of Au/a.k.c. |
8.6 × 10−2 gr |
90% production yield from synthesis [Au25(Cys)18] |
9.5 × 10−2 gr |
Number of a.k.c. required in 1 year |
2.23 × 105 |
1.70 × 106 |
Kilogrammes of Au required to satisfy the annual demand |
2.1 × 10 kg |
1.62 × 102 kg |
2.3 System boundaries and scope: synthesis of gold nanoclusters and preparation of antimicrobial keyboard covers
The system boundary defines the activities and unit processes included within the study, and therefore identifies the environmental impacts that are accounted for. This study applies a “cradle-to-grave” approach assuming that in the use phase, antimicrobial keyboard covers do not release substances into the environment. All the life cycle phases from the extraction of raw materials through to product's manufacturing and end-of-life management are considered, as shown in Fig. 1.
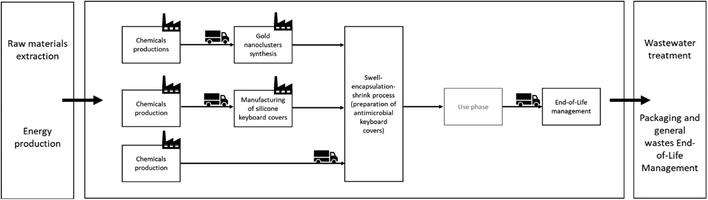 |
| Fig. 1 System boundary showing all the phases that have been considered in the LCA study of the antimicrobial keyboard covers, except for the use phase (box in grey). | |
2.4 Data collection, inventory and assumptions
The data used for compiling the life cycle inventory (LCI) are collected from the MAFuMa58 project collaborators, and the scientific literature and reports when necessary.
Milli-flow reactors are a fast and scalable synthesis methods, that would allow a transition from a lab-scale to a pilot scale, by numbering up the reactors.20 Therefore, in this case a numbering-up approach has been employed to estimate the quantities of chemicals, electricity, thermal energy and equipment required in all the total modules comprised of several milli-flow reactors, considered in the foreground system.
As introduced in section 2.1, integration of the [Au25(Cys)18] nanoclusters into silicone keyboard covers is executed through a swell–encapsulation–shrink process. Experts in the sectors agree that by the quantities of nanoclusters embedded in the silicone matrix during this process,41,42 it can be assumed that is likely to use 4 litre of photo-bactericidal solution to prepare 3 antimicrobial keyboard covers. In this case, the process was not scaled-up, thus the modelling of this process does not consider any automatization and optimisation, but only the required quantities of chemicals. Information on the inventory can be found in ESI† Annex II.
The antimicrobial keyboard covers production system assessed in this work is compared with the production of alcohol wipes and gloves,59,60 representing the traditional method used for cleaning computers and equipment. Estimation of the number of alcohol wipes and gloves assumes that each keyboard is cleaned every 6 hours, by using 1 alcohol wipe and 2 nitrile rubber gloves. By following the indications given by Gama Healthcare (one of the many NHS suppliers), the wipes are made of non-woven spun-bond impregnated in alcohol (70%).61 Modelling of nitrile rubber gloves is based on an LCA case study published in 2019.62Table 2 reported the quantities of wipes and gloves used in the UK and Europe.
Table 2 Estimated number of alcohol wipes and gloves necessary in the British and European hospitals per year
|
United Kingdom |
Europe |
Annual number of alcohol wipes used |
3.41 × 108 |
2.61 × 109 |
Annual number of gloves used |
6.82 × 108 |
5.22 × 109 |
For the modelling of both systems, the GaBi software by Sphera (previously Thinkstep, service package 40) is used, along with Sphera Professional database and Ecoinvent databases (versions 3.3 and 3.6).
Limitations of available data and information have required to make assumptions during both the compilation of the inventory and the modelling phase, such as:
• a faulty rate of 5% in the production of the antimicrobial keyboard covers is assumed, as well as for alcohol wipes and nitrile rubber silicone gloves production.
• The life-time of the antimicrobial keyboard covers was assumed to be equal to 1 year.
• By consulting experts in nanoparticles' synthesis, a 90% yield of the synthesis of gold nanoclusters has been assumed, along with the lifetimes of the equipment (ESI,† Annex II).
• Geographical reference for the nanoclusters and the final product production is assumed to be the United Kingdom. Concerning chemicals used in the system, it is assumed they are all produced in Europe and imported to the UK. Therefore, the electricity mixes used in this study refer to both the United Kingdom (in 2019) and the European region (EU-28, in 2019).
• Transport from Europe to the UK is modelled by assuming road transportation by means of Euro 3 truck (up to 7.5 tonnes gross weight/2.7 tonnes play-load at a capacity of 80%, and a distance of 1600 km per trip).
• Transport to the waste treatment facilities is supposed to occur in the United Kingdom, by means of Euro 3 truck, with a 2.7 tonnes play-load. Distances to the waste treatment facilities are set equal to 100 km per trip.
• Primary packaging of each finished chemical used in the system has been modelled and included within the system boundaries. When either data or datasets on the manufacturing of packaging were missing, the environmental impacts from the packaging production were calculated only on the basis of the estimated amount of material required, without considering energy inputs and emissions.
• It is assumed that all the glass packaging would be recycled. Whilst the management of the plastic packaging is modelled following the percentage of plastic going into landfill in the UK (around 30%).63
• No data on the production of L-cysteine has been found, thus D-lysine is used as a proxy of L-cysteine and modelled by using secondary data.64
• Because China is considered the principal exporter of plastic articles, it was assumed that the silicone rubber keyboard covers are manufactured there and shipped to the UK via a trans-oceanic ship.
Concerning the alcohol wipes and glove system, air emissions of propylene alcohol during the use phase of the alcohol wipes were not considered. This because the uncertainty on the quantity of the propylene employed in the using phase and the quantity retained in the wipe. In addition, the release of propylene is associated only to the impact category EF2.0 “photochemical ozone formation – human health”, which models the effect of “summer smog” due to non-methane volatile organic compounds (NMVOC) emissions (ESI† – Annex III). In this case, the propylene would be released in a confined environment where there wouldn't be the condition of producing ozone/“summer smog”, with negative effects on the human health, thus this emissions could be neglected.
2.5 Life cycle impact assessment (LCIA): impact categories and calculation methods
Life cycle impact assessment results are calculated at midpoint level by employing the Environmental Footprint method (version 2.0, EF 2.0).65,66 In addition, an endpoint impact category, “Metal depletion”, is calculated by employing the ReCiPe method 2016 (hierarchical).67–69 The hierarchical perspective was selected as it is based on “scientific consensus with regard to the time frame and plausibility of impact mechanisms”.67,68Table 3 reports the impact categories considered in this study. The “metal depletion [$]” impact category is considered only for assessing the antimicrobial keyboard covers to better understand the impact of mining gold. Indeed, this impact category could be defined as a more understandable indicator concerning the resource depletion issue. It refers to cost due to the “average extra amount of ore produced in the future caused by the extraction of mineral resources considering all future production of that mineral resource”.67 Further referencing of each impact category models included in the EF 2.0 are reported in the Annex III of the ESI.†
Table 3 List of impact categories used in this study for assessing the life cycle impacts of both antimicrobial keyboard covers and alcohol wipes and gloves. To be noted, the metal depletion [$] impact category has been used only for the antimicrobial keyboard covers and not for the other system under study – alcohol wipes and gloves
Environmental footprint method (EF 2.0) |
Unit |
Acidification terrestrial and freshwater |
Mole of H+ eq. |
Cancer human health effects |
CTUh |
Climate change |
kg CO2 eq. |
Climate change (fossil) |
kg CO2 eq. |
Climate change (biogenic) |
kg CO2 eq. |
Ecotoxicity freshwater |
CTUe |
Eutrophication freshwater |
kg P eq. |
Eutrophication marine |
kg N eq. |
Eutrophication terrestrial |
Mole of N eq. |
Non-cancer human health effects |
CTUh |
Ozone depletion |
kg CFC-11 eq. |
Photochemical ozone formation – human health |
kg NMVOC eq. |
Resource use, energy carriers |
MJ |
Resource use, mineral and metals |
kg Sb eq. |
Water scarcity |
m3 world equiv. |
ReCiPe method (2016) – hierarchical |
Unit |
Metal depletion |
$ |
2.6 Interpretation: environmental sustainability based carrying-capacity normalisation references
The results and discussion section presents the LCIA results and their discussions, together with further analysis on the environmental sustainability of both systems: antimicrobial keyboard cover and alcohol wipes and gloves. To achieve this objective, we apply the reviewed version of the carrying-capacity normalisation factors proposed by Bjørn and Hauschild,37,40 for 6 of the 15 ILCD impact categories. Carrying-capacity factors (CcFs) give information on the global and per capita impacts that the whole biosphere can tolerate to stay within the “safe operating space” defined within the planetary boundaries.36 As one of the purposes of this study is to assess the environmental sustainability of the two systems, as a function of the UK and continental Europe's possible demand, the CcFs are estimated by applying the egalitarian principle,70 as shown in equation [eqn (1)]. | CcFpc = CcFg/Global population | (1) |
where CcFpc is the carrying-capacity factor per capita and CcFg represents the carrying-capacity factor at the global level. Bjørn et al. calculated the CcFs per capita based on the global population in 2010 (6.91 + 09 people). In the case of this study, the CcFs per capita were estimated based on the global population in 2018, equal to 7.59 × 109.71 Afterwards, the CcFs for both the UK and Europe are evaluated by multiplying each CcFpc by their respective population. Table 4 shows only the CcFs considered in our study; the third column reports the carrying-capacity factors at the global level “CcF Global” presented by Sala et al.40 The fourth column presents the carrying-capacity factors specifically calculated for the European continent “CcF Europe” by Bjørn et al. The last two columns show the annual CcFs that we have estimated and used in this study.
Table 4 Carrying capacity factors (CcF) at global level and European level from scientific literature. CcF estimated using the CcF from scientific literature for both the United Kingdom and European continent
|
CcF global/year from Sala40 a |
CcF Europe/year from Bjørn37 a |
Estimated CcF UK/yearb |
Estimated CcF Europe/yearb |
Reference year: 2010. Considering the population in 2010.
Reference year: 2018. Considering the population in 2018.
|
Acidification terrestrial and freshwater [mole H+ eq.] |
1.00 × 1012 |
— |
8.78 × 109 |
8.27 × 1010 |
Climate change [kg CO2 eq.] |
6.18 × 1012 |
— |
3.17 × 1010 |
5.63 × 1011 |
Ecotoxicity freshwater [CTUe] |
1.31 × 1014 |
7.62 × 1012 |
8.09 × 1011 |
7.62 × 1012 |
Eutrophication freshwater [kg P eq.] |
5.81 × 109 |
3.41 × 108 |
3.62 × 107 |
3.41 × 108 |
Eutrophication marine [kg N eq.] |
2.01 × 1011 |
2.27 × 1010 |
2.41 × 109 |
2.27 × 1010 |
Eutrophication terrestrial [mole N eq.] |
6.13 × 1012 |
— |
1.14 × 1010 |
1.07 × 1011 |
Ozone depletion [kg CFC-11 eq.] |
5.39 × 108 |
— |
4.74 × 106 |
4.46 × 107 |
Photochemical ozone formation [kg NMVOC eq.] |
4.07 × 1011 |
— |
3.57 × 109 |
3.36 × 1010 |
Water scarcity [m3 world equiv.] |
1.82 × 1014 |
3.63 × 1011 |
3.85 × 1010 |
3.63 × 1011 |
2.7 Interpretation: uncertainty of the results
The case study presented in the article does not show any uncertainty analysis aiming at evaluating the numerical uncertainty of the results. The model of the antimicrobial keyboard covers is based on data from laboratory scale, which were used to scale the system to satisfy the FU. Whilst the process of preparing antimicrobial keyboard covers is not optimised, leading to conservative results. As it commonly happens when applying the LCA methodology, we encountered difficulties in retrieving information on the uncertainty of primary data and datasets from LCA databases. This made the evaluation of the uncertainty of the model not possible. Uncertainty of the results is also influenced by the LCIA methods employed in calculating the impacts, the modelling of the system and the scenario evaluated.72 The last two sources of uncertainty can be assessed by performing local uncertainty analysis, also known as sensitivity analysis. The article shows a sensitivity analysis on the length of the life-time of the antimicrobial keyboard covers, in section 3.2. Another sensitivity analysis on the production efficiency (faulty rate) of the antimicrobial keyboard covers system and the alcohol wipes and gloves in the Supplementary Information is reported in Annex V of the ESI.† Generally, the model employs conservative values, like the yield of the gold nanoclusters synthesis (section 2.4), lower than the values reported in the scientific literature (∼95%),41,73 for which no sensitivity was performed.
3 Results and discussions
This section reports the LCIA results, mainly focusing on the environmental impacts related to the antimicrobial keyboard covers (akcs). Section 3.1 presents a hot-spot analysis of antimicrobial keyboard covers; whilst section 3.2 a comparative analysis between the antimicrobial keyboard covers and the alcohol wipes and gloves is presented. Next, the outcomes on the environmental sustainability of both systems are shown in section 3.3, and finally, section 3.4 reports a short analysis of the carbon footprint due to the whole healthcare sector.
3.1 LCIA: antimicrobial keyboard covers
Fig. 2(a) displays the hot spot analysis for the production of the antimicrobial keyboard covers and shows contributions (in%) of the 3 main life cycle phases of the system: preparation of the solution for the swell–encapsulation–process including the synthesis of gold nanoclusters, manufacturing of clean-silicone keyboard covers and the end-of-life. As illustrated in Fig. 2(a), preparation of the solution used to prepare the akcs has the highest environmental burden in all the impact categories, followed by the manufacturing of the clean-silicone keyboard covers. Impacts from the preparation of the antimicrobial keyboard covers are mainly due to the gold precursor used in the synthesis of [Au25(Cys)18] nanoclusters, as shown in the hotspot analysis presented in Fig. 2(b). The hotspot analysis highlights that the synthesis of gold precursors, and specifically the mining of gold (see ESI,† Annex VI), is the activity contributing the most to almost all the impact categories (>70%). Exceptions are: ozone depletion, which is mainly affected by equipment manufacturing, and climate change and resource use-energy carriers impact categories. The impacts of these latter categories are mainly linked to gold mining, with a contribution of ∼76%, and the electricity required in the heating system (∼20%). Silicone keyboard covers show relatively high contribution to the water scarcity impact category (more than 20%), to the climate change category (more than 10%) and the ozone depletion (almost 10%) (ESI,† Annex VI). The analysis clearly shows that the silicone covers' overall production has a significant contribution, much higher than the transport process (from China to Europe by a container ship). As expected from the analysis of the results concerning the endpoint impact category “metal depletion”, the mining of gold is the activity with the highest forecasted rise in cost of extraction. The ReCiPe method (2016)67–69 estimates an average increase of the extraction cost of gold equal to $863.
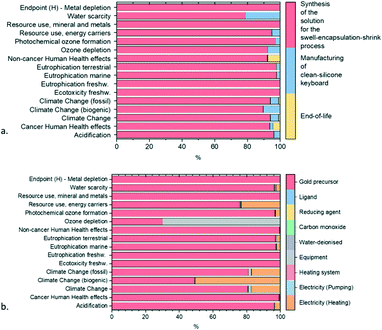 |
| Fig. 2 Hot-spots analysis for both the whole system for producing antimicrobial keyboard covers (figure a) and the synthesis of [Au25(Cys)18] nanoclusters (figure b). | |
3.2 Comparative analysis: antimicrobial keyboard covers vs. alcohol wipes and gloves
A comparison between the antimicrobial keyboard covers' environmental impacts and the alcohol wipes and gloves has been carried out. The total absolute values for the impact of antimicrobial keyboard covers is shown for all impact categories – for the British and European scenarios, in Fig. 3 and respectively (see yellow bar). Impacts range between orders of magnitude of 1.0 × 10−2 for the impact category “cancer human health effects” to 1.0 × 106 (British scenario) and 1.0 × 108 (European scenario) for the impact category “resource use, energy carrier”.
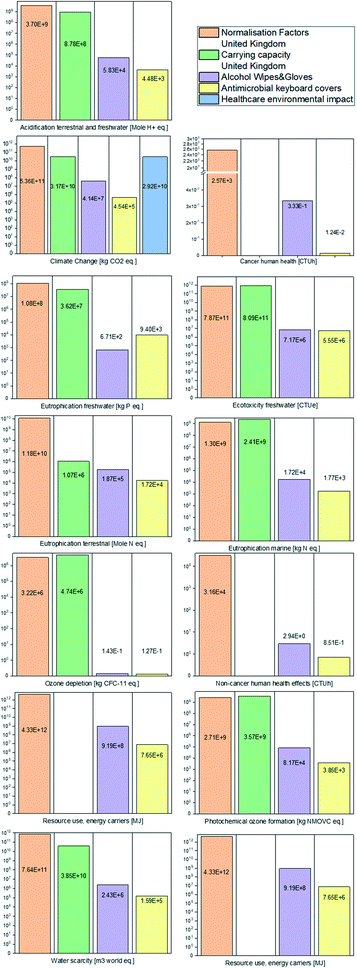 |
| Fig. 3 Absolute values concerning the British scenario. Graphs report values concerning the adjusted EF2.0 normalisation factors (2018), adjusted carrying-capacity factors (2018), impacts for producing alcohol wipes and gloves, impacts for producing antimicrobial keyboard covers. In addition, for the climate change impact category the healthcare annual environmental impact is presented. To be noted: some impact categories do not present values for the carrying-capacity. | |
Fig. 3 and 4 report the absolute results for both cleaning methods to satisfy British and European demands, respectively. Table 5 reports the relative changes (in%) between the two systems, taking the alcohol wipes and gloves system as baseline.
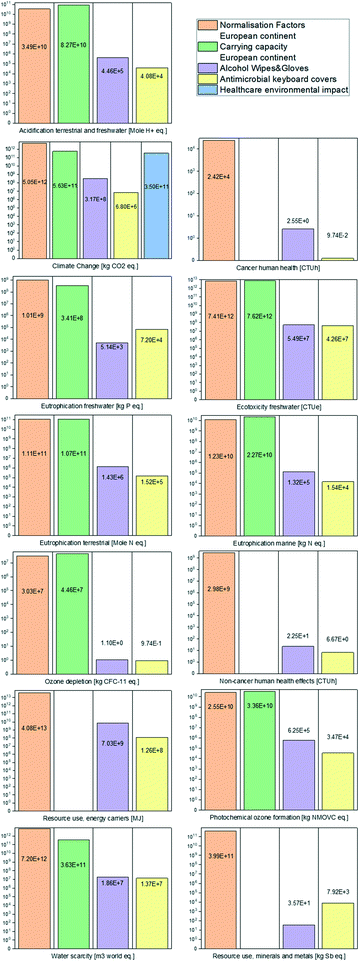 |
| Fig. 4 Absolute values concerning the European scenario. Graphs report values concerning the adjusted EF2.0 normalisation factors (2018), adjusted carrying-capacity factors (2018), impacts for producing alcohol wipes and gloves, impacts for producing antimicrobial keyboard covers. In addition, for the climate change impact category the healthcare annual environmental impact is presented. To be noted: some impact categories do not present values for the carrying-capacity. | |
Table 5 Relative change (in%) between the production of alcohol wipes and gloves and antimicrobial keyboard covers'system in both the United Kingdom and Europe. Figures in table represent the percentage of decrease from alcohol wipes and gloves to antimicrobial keyboard covers
Environmental footprint method (EF 2.0) |
Unit |
UK relative change (%) |
European relative change (%) |
Acidification terrestrial and freshwater |
Mole of H+ eq. |
−92.3% |
−90.9% |
Cancer human health effects |
CTUh |
−96.3% |
−96.2% |
Climate change |
kg CO2 eq. |
−98.9% |
−97.9% |
Climate change (fossil) |
kg CO2 eq. |
−98.9% |
−97.9% |
Climate change (biogenic) |
kg CO2 eq. |
−97.6% |
−91.1% |
Ecotoxicity freshwater |
CTUe |
−22.6% |
−22.4% |
Eutrophication freshwater |
kg P eq. |
1301.4% |
1300.8% |
Eutrophication marine |
kg N eq. |
−89.7% |
−88.3% |
Eutrophication terrestrial |
Mole of N eq. |
−90.8% |
−89.4% |
Non-cancer human health effects |
CTUh |
−71.1% |
−70.3% |
Ozone depletion |
kg CFC-11 eq. |
−10.8% |
−11.5% |
Photochemical ozone formation – human health |
kg NMVOC eq. |
−95.3% |
−94.4% |
Resource use, energy carriers |
MJ |
−99.2% |
−98.2% |
Resource use, mineral and metals |
kg Sb eq. |
22 088% |
22 099% |
Water scarcity |
m3 world equiv. |
−93.5% |
−92.6% |
The results, displayed in Table 5, highlight the substantial advantage in terms of environmental impacts associated to the antimicrobial keyboard covers compared to alcohol wipes and gloves. Because of the mining of gold, there are only two impact categories that do not benefit from a possible substitution: “eutrophication freshwater” – affected by the chemicals used in the mining process, and the beneficiation process, and “resource use, minerals and metals”.
When the parameter life-time of the antimicrobial keyboard covers varies (ESI,† Annex VII), the annual production of akcs to satisfy the FU changes, as well as the environmental impacts. A 6 month life-time of akcs still shows advantages in all the impact categories, expect for “eutrophication freshwater” and “resource use, minerals and metals”. In addition, the “ozone depletion”, mostly linked to the equipment used in the synthesis of gold nanoclusters, and “ecotoxicity freshwater” associated with the gold mining, overtake the respective impacts associated with the alcohol wipes and gloves system. On the other hand, when the life-time parameter is doubled, from 1 year to 2 years, the environmental impacts of the akcs halves. Nevertheless, when compared with the alcohol wipes and gloves, the impact categories “eutrophication freshwater” and “resource use, minerals and metals” still exceed the environmental impacts of the compared system.
3.3 Environmental sustainability based on carrying-capacity normalisation references
In this section, the attributional LCA study results are analysed to determine whether introducing antimicrobial keyboard covers in British and European hospitals could carry advantages from an environmental point of view. Moreover, the comparison helps to understand the magnitude of the depletion of the annual carrying-capacities that the antimicrobial keyboard covers would cause. As previously introduced, in this work, the carrying-capacity normalisation references estimated by Bjørn et al.37 and reviewed in Sala et al.40 are applied. Since those values were calculated on the basis of the population in 2010, we adapted them to the population in the United Kingdom and Europe in 2018. For simplicity, we assumed that the EF2.0 normalisation factors per capita,40 based on the emissions that occurred in 2010, would represent the burden that each person had on the environment in 2018. Based on these assumptions, we have calculated the annual environmental impacts that would be attributed to the population in the UK and Europe. Charts in Fig. 3 and 4 report values of the recalculated EF2.0 normalisation factors, the carrying-capacity factors and results from the LCA study. From the figures, it is possible to compare the 2 types of normalisation references and define the impact categories contributing the most to the depletion of the carrying-capacities allowance. In the case of antimicrobial keyboard covers, eutrophication freshwater, climate change and ecotoxicity freshwater are the impact categories with higher burdens on the carrying-capacities, respectively for 0.03%, 0.001% and 0.001% for both the European and the British scenarios.
Instead, when looking at the wipes and gloves, the impact categories presenting higher contributions to the carrying-capacities are: climate change (0.06–0.07%), water scarcity (0.01%), photochemical ozone formation (0.002%), and eutrophication freshwater (0.002%) for both the European and the British scenarios.
Table 6 reports the differences between 2 scenarios, one in which alcohol wipes and gloves are used in UK and Europe, (EF2.0 normalisation references in 2018), and a new scenario. This envisages a situation in which alcohol wipes and gloves are substituted by antimicrobial keyboard covers (namely new scenario). Results associated to the new scenario are calculated by subtracting from the impacts in 2018, represented by the EF2.0 normalisation factors, the environmental impacts of the akcs. Moreover, these differences have been normalised against the carrying-capacity references (in 2018) to visualise the reduction or increase of environmental impacts. The outcomes show that employing akcs over alcohol wipes and gloves would benefit almost all the impact categories. Climate change and water scarcity would be the 2 impact categories with the highest benefits. Instead, employing of akcs will have drawbacks for the eutrophication of freshwaters.
Table 6 Table reports the differences between the British/European overall annual impacts in a scenario in which the alcohol wipes and gloves procedure is applied (represented by the values EF2.0 NFs in 2018a) and a scenario in which they are substituted by the antimicrobial keyboard covers. In addition, such difference values have been normalised based on the carrying-capacity values adjusted to the year 2018. The normalised values are expressed as person eq. and help in the identification of the highest-burden reduction (savings) on the environment
EF 2.0 |
United Kingdom |
European continent |
Savings between the 2 scenarios |
Normalisation based on CCa |
Difference between the 2 scenarios |
Normalisation based on CCa |
Calculated based on the NFs global per person (in 2010) and the British/European population in 2018. It was assumed no variations in terms of impact per person have occurred in the last 8 years.
|
Acidification terrestrial and freshwater |
5.38 × 104 |
4.09 × 102 person eq. |
4.05 × 105 |
3.11 × 103 person eq. |
Mole of H+ eq. |
Mole of H+ eq. |
Climate change |
4.09 × 107 |
4.57 × 104 person eq. |
3.10 × 108 |
3.50 × 105 person eq. |
kg CO2 eq. |
kg CO2 eq. |
Ecotoxicity freshwater |
1.26 × 106 CTUe |
1.34 × 102 person eq. |
1.23 × 107 CTUe |
9.01 × 102 person eq. |
Eutrophication freshwater |
−8.73 × 103 |
−1.61 × 104 person eq. |
−6.69 × 104 |
−1.09 × 105 person eq. |
kg P eq. |
kg P eq. |
Eutrophication marine |
1.54 × 104 |
4.27 × 102 person eq. |
1.17 × 105 |
2.86 × 103 person eq. |
kg N eq. |
kg N eq. |
Eutrophication terrestrial |
1.70 × 105 |
9.92 × 102 person eq. |
1.28 × 106 |
7.54 × 103 person eq. |
Mole of N eq. |
Mole of N eq. |
Ozone depletion |
1.55 × 10−2 |
2.18 × 10−1 person eq. |
1.26 × 10−1 |
1.79 × 10 person eq. |
kg CFC-11 eq. |
kg CFC-11 eq. |
Photochemical ozone formation |
7.79 × 104 |
1.45 × 103 person eq. |
5.90 × 105 |
1.11 × 104 person eq. |
kg NMVOC eq. |
kg NMVOC eq. |
Water scarcity |
2.27 × 106 |
3.93 × 103 person eq. |
1.72 × 107 |
2.65 × 104 person eq. |
m3 world equiv. |
m3 world equiv. |
3.4 Carbon footprint of the healthcare sector
Finally, an analysis focusing on the carbon footprint, climate change impact category, is presented in this section. This analysis, which is a normalisation based on the NHS carbon footprint, should support the interpretation of the results. In addition to that, an estimation of the depletion of the climate change to the carrying-capacity by the NHS carbon footprint is presented. In 2019, Pichler et al.74 assessed the climate change of different healthcare systems in Europe and other nations. We used those values to estimate the impacts of both the British and the European healthcare systems and compared them with EF2.0 NFs and carrying-capacity (Cc) values. It must be noted that the data reported by Pichler and co-authors refer to 2014. We assume that the per capita impact has not changed since then and estimated the climate change impact based on population in the UK and in the continent Europe in 2018, the reference year of this paper.
As presented in Fig. 3, the British healthcare sector presents the emissions of CO2 eq. equal to 2.92 × 1010 kg CO2 eq., with 66% of these emissions associated with the supply chain of equipment, medicines and PPE.75 The total healthcare sector carbon footprint represents 5.4% of the EF2.0 normalisation factor (in 2018) for the “climate change” associated with the UK. The life cycle of alcohol wipes and gloves contributes to the NHS supply chain's impact for 0.22%; whilst antimicrobial keyboard covers 0.002%. Furthermore, 32.2% of the UK annual carrying-capacity allowance of CO2 eq. emissions are depleted only by the supply chain of the NHS, whilst the entire healthcare sector depletes around 49% of the national carrying-capacity.
Regarding the European healthcare sector, the estimated amount of 3.50 × 1011 kg CO2 eq. makes up 7% of the CO2 eq. emissions estimated for the European continent (EF2.0 NFs values, in 2018). Furthermore, the life cycle of alcohol wipes and gloves contributes to the carbon footprint of the healthcare system in Europe equal to 0.1% against the 0.002% of the antimicrobial keyboard covers. Ultimately, the European healthcare sector uses up to 62% of the annual European climate change carrying-capacity.
In both cases, the adoption of antimicrobial keyboard covers would help decrease the carbon footprint associated with cleaning and disinfecting the keyboards of the hospital equipment.
As we have already crossed 4 of the 9 safe-planetary boundaries,36 climate change, biosphere integrity, land-system change and biochemical flows (phosphorus and nitrogen), it is pivotal to address these issues and try to find new solutions with lower environmental burden. From the results of the comparative LCA study, it can be deduced that the adoption of antimicrobial keyboard covers would have some benefits, in terms of climate change, acidification terrestrial and freshwater and eutrophication marine and terrestrial. Moreover, it would assist in reducing the impact on photochemical ozone formation, ecotoxicity and human health-cancer and non-cancer effects. On the contrary, when primary gold is used, as it was assumed in this case study, impacts linked to freshwater ecosystems, such as eutrophication of freshwater would increase. Carrying-capacity values at both national and European, as expected, were not exceeded by the two systems. Although both alcohol wipes and gloves, and antimicrobial keyboard covers per se are environmentally sustainable, they should be considered within the healthcare sector, which covers circa 49–60% of the allowed annual climate change carrying-capacity. Employing the carrying-capacity normalisation values allowed identifying those impact categories that should get special attention over the others, such as “climate change”, “eutrophication freshwater” and “photochemical ozone formation”.
4 Conclusions
In this paper we employ an attributional life cycle assessment to evaluate the environmental impacts of a new product, namely antimicrobial keyboard cover, and compare these impacts with those arising from the usage of alcohol wipe and gloves. This latter option is currently employed in hospitals for cleaning keyboards of computers and other equipment. The case study presents an analysis at national level, taking the United Kingdom as a reference, and at European level (considering the whole continent).
Starting with the creation of an inventory for both options, life cycle impacts are calculated; in addition, by using data from recent publications, an attempt at evaluating the absolute environmental impacts of both systems is done. In order to do so, carrying-capacity factors from scientific literature37,40 are used and adapted to reflect the carrying-capacity associated to the population of the United Kingdom and the European continent. Then outcomes of the LCIA phase are compared with the new carrying-capacity values to understand whether the adoption of antimicrobial keyboard covers could bring significant benefits, or not. In addition, by using normalisation factors as reference for the annual environmental impacts due to the British and European population, a comparison between how the impact would change if antimicrobial keyboard covers replaced the currently used alcohol wipes and gloves is performed.
Results of the LCA study show that almost all the environmental impacts linked to the antimicrobial keyboard covers are caused by the extraction of primary gold for the synthesis of gold nanoclusters. Therefore it should be recommended to take particular care in the selection of suppliers providing secondary gold, i.e. recovered and recycled gold, as this would significantly help to reduce the environmental impacts of the system.76 Specifically, it would help in decreasing the resource consumption and all the impacts linked to the mining of primary gold, as investigated by B. Fritz and colleagues.76 To this end, if gold nanoclusters and nanoparticles were widely employed, it would be advisable to explore new techniques and methodologies for recovering nanomaterials from wastewater effluents and different solid matrices.22
Comparison between the LCIA results of the antimicrobial keyboard covers with the ones from the production of alcohol wipes and gloves highlights the overall environmental benefits of antimicrobial keyboard covers being adopted in hospitals. Except for the eutrophication freshwater and the water scarcity impact categories.
The interpretation phase emphasises the substantial difference between the environmental impacts when normalisation factors (NFs) and the annual carrying-capacity factors are used, as already highlighted in Bjørn and Hauschild.37 The EF 2.0 NFs exceed the carrying-capacity factors for some impact categories, such comparison can support the identification of which impact categories should be prioritized when drawing conclusions based on LCA studies.
It must be noted that normalisation based on carrying-capacity factors may lead to misleading labelling of many products and services to be environmentally sustainable in absolute terms. Indeed, each product contributes to climate change and environmental impacts, but it is the total sum of all the human activities to exceed the carrying-capacity allowance. If we consider the environmental impacts associated with all the objects, food, and services a person uses during a year, we can easily understand that maybe each of those objects would deplete a small portion of the carrying-capacity. But when considering what a person consumes in a year – as a whole – that can exceed the carrying-capacities. Therefore, it is crucial to understand the big picture and analyse the product environmental impacts within the sector. In this case, both antimicrobial keyboard covers, and alcohol wipes and gloves do not significantly contribute to both the NFs and carrying-capacities at national and European levels. On the other hand, the carbon footprint associated to the whole healthcare sector exceeds the climate change carrying-capacity estimated for both the UK and European continent. In case of adopting antimicrobial keyboard covers in hospitals, the healthcare sector would still present a carbon footprint exceeding the carrying capacity. However, the contribution associated with the activity of cleaning the keyboards in hospitals to reduce trans-transmissions of pathogens in patients would contribute only a 0.001% to it.
With this end, LCA could help to specifically create a high-level and more specific hotspot analysis and compare the state-of-the-art with other possible solutions. For instance, as pinpointed by “Delivering a ‘Net Zero’ National Health Service”75 report presented by NHS in 2020, 66% of the total GHGs impacts are due to the supply-chain providing PPE, medicines, equipment, etc. Therefore, it would be of utmost importance to try to reduce these impacts. This would mean exploring alternative and engaging with all the supply-chain stakeholders to determine where is possible to intervene to reduce the carbon footprint.
Author contributions
Martina Pucciarelli: conceptualization, methodology, investigation, software, writing – original draft, visualization, writing – review and editing. Fabio Grimaldi: conceptualization, visualization. Gaowei Wu: resources. Paola Lettieri: supervision, funding acquisition, writing – review and editing.
Conflicts of interest
Authors declare that there are no conflicts to declare.
Acknowledgements
The authors would like to thank EPSRC for funding (grant EP/M015157/1) and the Department of Chemical Engineering at University College London. Fabio Grimaldi thanks the Marie Skłodowska-Curie programme (grant no. 721290) for funding his PhD studentship. Professor Asterios Gavriilidis from the Department of Chemical Engineering at UCL. Andrea Paulillo and Charnett Chau from Paola Lettieri's group are acknowledged for their support.
References
- O. P. Bolade, A. B. Williams and N. U. Benson, Green Synthesis of Iron-Based Nanomaterials for Environmental Remediation: A Review, Environ. Nanotechnol., Monit. Manage., 2020, 13, 100279, DOI:10.1016/j.enmm.2019.100279.
- X. He, H. Deng and H. Hwang, The Current Application of Nanotechnology in Food and Agriculture, J. Food Drug Anal., 2019, 27(1), 1–21, DOI:10.1016/j.jfda.2018.12.002.
- G. Karthik Pandiyan and T. Prabaharan, Implementation of Nanotechnology in Fuel Cells, Mater. Today: Proc., 2020, 1–5, DOI:10.1016/j.matpr.2020.01.368.
- G. Cotin, S. Piant, D. Mertz, D. Felder-Flesch and S. Begin-Colin, Iron Oxide Nanoparticles for Biomedical Applications: Synthesis, Functionalization, and Application, Iron Oxide Nanopart. Biomed. Appl., 2018, 43–88, DOI:10.1016/B978-0-08-101925-2.00002-4.
- J. Lodhia, G. Mandarano, N. J. Ferris, P. Eu and S. F. Cowell, Development and Use of Iron Oxide Nanoparticles (Part 1): Synthesis of Iron Oxide Nanoparticles for MRI, Biomed. Imaging Intervention J., 2010, 6(2) DOI:10.2349/biij.6.2.e12.
- P. G. Jamkhande, N. W. Ghule, A. H. Bamer and M. G. Kalaskar, Metal Nanoparticles Synthesis: An Overview on Methods of Preparation, Advantages and Disadvantages, and Applications, J. Drug Delivery Sci. Technol., 2019, 53, 101174, DOI:10.1016/j.jddst.2019.101174.
- M. Soleimani, A. M. Al Zaabi, M. Merheb and R. Matar, Nanoparticles in Gene Therapy, Int. J. Integr. Biol., 2016, 17(1) DOI:10.1016/b978-0-12-416020-0.00013-9.
- E. S. Ali, S. M. Sharker, M. T. Islam, I. N. Khan, S. Shaw, M. A. Rahman, S. J. Uddin, M. C. Shill, S. Rehman, N. Das, S. Ahmad, J. A. Shilpi, S. Tripathi, S. K. Mishra and M. S. Mubarak, Targeting Cancer Cells with Nanotherapeutics and Nanodiagnostics: Current Status and Future Perspectives, Semin. Cancer Biol., 2020, 1–17, DOI:10.1016/j.semcancer.2020.01.011.
- M. Borkowska, M. Siek, D. V. Kolygina, Y. I. Sobolev, S. Lach, S. Kumar, Y.-K. Cho, K. Kandere-Grzybowska and B. A. Grzybowski, Targeted Crystallization of Mixed-Charge Nanoparticles in Lysosomes Induces Selective Death of Cancer Cells, Nat. Nanotechnol., 2020, 15, 331–341, DOI:10.1038/s41565-020-0643-3.
- E. C. Dreaden, M. a. Mackey, X. Huang, B. Kang and M. a. El-Sayed, Beating Cancer in Multiple Ways Using Nanogold, Chem. Soc. Rev., 2011, 40(7), 3391–3404, 10.1039/c0cs00180e.
- E. O. Ogunsona, R. Muthuraj, E. Ojogbo, O. Valerio and T. H. Mekonnen, Engineered Nanomaterials for Antimicrobial Applications: A Review, Appl. Mater. Today, 2020, 18, 100473, DOI:10.1016/j.apmt.2019.100473.
- D. Roe, B. Karandikar, N. Bonn-Savage, B. Gibbins and J. B. Roullet, Antimicrobial Surface Functionalization of Plastic Catheters by Silver Nanoparticles, J. Antimicrob. Chemother., 2008, 61(4), 869–876, DOI:10.1093/jac/dkn034.
-
ISO, Environmental Management — Life Cycle Assessment — Principles and Framework, ISO 14040, 2006, vol. 2006, pp. 1–28, DOI:10.1136/bmj.332.7550.1107.
-
ISO 14044, Life Cycle Assessment — Requirements and Guidelines, International Organization for Standardization, 2006, p. 46 Search PubMed.
-
JRC, ILCD Handbook - General Guide for Life Cycle Assessment- Detailed Guidance, 2010, DOI:10.2788/38479.
- M. Slotte and R. Zevenhoven, Energy Requirements and Life Cycle Assessment of Production and Product Integration of Silver, Copper and Zinc Nanoparticles, J. Cleaner Prod., 2017, 148, 948–957, DOI:10.1016/j.jclepro.2017.01.083.
- L. Pourzahedi and M. J. Eckelman, Comparative Life Cycle Assessment of Silver Nanoparticle Synthesis Routes, Environ. Sci.: Nano, 2015, 2(4), 361–369, 10.1039/C5EN00075K.
- R. Hischier and T. Walser, Life Cycle Assessment of Engineered Nanomaterials: State of the Art and Strategies to Overcome Existing Gaps, Sci. Total Environ., 2012, 425, 271–282, DOI:10.1016/j.scitotenv.2012.03.001.
- H. C. Kim and V. Fthenakis, Life Cycle Energy and Climate Change Implications of Nanotechnologies: A Critical Review, J. Ind. Ecol., 2013, 17(4), 528–541, DOI:10.1111/j.1530-9290.2012.00538.x.
- F. Grimaldi, M. Pucciarelli, A. Gavriilidis, P. Dobson and P. Lettieri, Anticipatory Life Cycle Assessment of Gold Nanoparticles Production: Comparison of Milli-Continuous Flow and Batch Synthesis, J. Cleaner Prod., 2020, 269(122335) DOI:10.1016/j.jclepro.2020.122335.
- P. Pati, S. McGinnis and P. J. Vikesland, Life Cycle Assessment of “Green” Nanoparticle Synthesis Methods, Environ. Eng. Sci., 2014, 31(7), 410–420, DOI:10.1089/ees.2013.0444.
- P. Pati, S. McGinnis and P. J. Vikesland, Waste Not Want Not: Life Cycle Implications of Gold Recovery and Recycling from Nanowaste, Environ. Sci.: Nano, 2016, 3(5), 1133–1143, 10.1039/C6EN00181E.
- M. Pini, P. Neri, R. Gamberini, B. Rimini, S. Marinelli and A. M. Ferrari, Life Cycle Assessment of a Nano-TiO2 Functionalized Enamel Applied on a Steel Panel, Int. J. Oper. Quant. Manag., 2017, 23(1), 41–60 Search PubMed.
- M. Pini, E. Cedillo González, P. Neri, C. Siligardi and A. Ferrari, Assessment of Environmental Performance of TiO2 Nanoparticles Coated Self-Cleaning Float Glass, Coatings, 2017, 7(1), 8, DOI:10.3390/coatings7010008.
- M. Pini, R. Rosa, P. Neri, F. Bondioli and A. M. Ferrari, Environmental Assessment of a Bottom-up Hydrolytic Synthesis of TiO 2 Nanoparticles, Green Chem., 2015, 17(1), 518–531, 10.1039/C4GC00919C.
- B. M. K. Manda, E. Worrell and M. K. Patel, Prospective Life Cycle Assessment of an Antibacterial T-Shirt and Supporting Business Decisions to Create Value, Resour., Conserv. Recycl., 2015, 103, 47–57, DOI:10.1016/j.resconrec.2015.07.010.
- D. E. Meyer, M. A. Curran and M. A. Gonzalez, An Examination of Silver Nanoparticles in Socks Using Screening-Level Life Cycle Assessment, J. Nanopart. Res., 2011, 13(1), 147–156, DOI:10.1007/s11051-010-0013-4.
-
S. Sala, F. Reale, J. Cristóbal-García, L. Marelli and P. Rana, Life Cycle Assessment for the Impact Assessment of Policies. Life Thinking and Assessment in the European Policies and for Evaluating Policy Options, 2016, vol. 28380, DOI:10.2788/318544.
- S. V. Ramasamy, N. J. Titchener-Hooker and P. Lettieri, Life Cycle Assessment as a Tool to Support Decision Making in the Biopharmaceutical Industry: Considerations and Challenges, Food Bioprod. Process., 2015, 94, 297–305, DOI:10.1016/j.fbp.2014.03.009.
-
Council of the European Union, REGULATION OF THE EUROPEAN PARLIAMENT AND OF THE COUNCIL on the Establishment of a Framework to Facilitate Sustainable Investment, and Amending Regulation 2019/2088 on Sustainability-Related Disclosures in the Financial Services Sector, 2009, vol. 2014 Search PubMed.
- H. Babaizadeh and M. Hassan, Life Cycle Assessment of Nano-Sized Titanium Dioxide Coating on Residential Windows, Constr. Build. Mater., 2013, 40, 314–321, DOI:10.1016/j.conbuildmat.2012.09.083.
- F. Martins, S. Machado, T. Albergaria and C. D. Matos, LCA Applied to Nano Scale Zero Valent Iron Green Synthesis, Recent Adv. Environ. Sci. Biomed., 2014, 33–38, DOI:10.1007/s11367-016-1258-7.
- M. Slotte, G. Metha and R. Zevenhoven, Life Cycle Indicator Comparison of Copper, Silver, Zinc and Aluminum Nanoparticle Production through Electric Arc Evaporation or Chemical Reduction, Int. J. Energy Environ. Eng., 2015, 6(3), 233–243, DOI:10.1007/s40095-015-0171-3.
- T. Walser, E. Demou, D. J. Lang and S. Hellweg, Prospective Environmental Life Cycle Assessment of Nanosilver, Environ. Sci. Technol., 2011, 4570–4578, DOI:10.1021/es2001248.
- J. Rockström, W. Steffen, K. Noone, E. Lambin, T. M. Lenton, M. Scheffer, C. Folke, H. J. Schellnhuber, C. A. Wit, T. Hughes, S. Leeuw, H. Rodhe, P. K. Snyder, R. Costanza, U. Svedin, M. Falkenmark, L. Karlberg, R. W. Corell, V. J. Fabry, J. Hansen, B. Walker, D. Liverman, K. Richardson, P. Crutzen and J. Foley, Planetary Boundaries : Exploring the Safe Operating Space for Humanity, Ecol. Soc., 2009, 14(2), 32 CrossRef.
- W. Steffen, K. Richardson, J. Rockstrom, S. E. Cornell, I. Fetzer, E. M. Bennett, R. Biggs, S. R. Carpenter, W. de Vries, C. A. de Wit, C. Folke, D. Gerten, J. Heinke, G. M. Mace, L. M. Persson, V. Ramanathan, B. Reyers and S. Sorlin, Planetary Boundaries: Guiding Human Development on a Changing Planet, Science, 2015, 347(6223), 1259855–1259855, DOI:10.1126/science.1259855.
- A. Bjørn and M. Z. Hauschild, Introducing Carrying Capacity-Based Normalisation in LCA: Framework and Development of References at Midpoint Level, Int. J. Life Cycle Assess., 2015, 20(7), 1005–1018, DOI:10.1007/s11367-015-0899-2.
-
S. Sala, L. Benini, E. Crenna and M. SecchiGlobal Environmental Impacts and Planetary Boundaries in LCA, 2016, DOI:10.2788/64552.
-
EC-JRC, Characterisation Factors of the ILCD Recommended Life Cycle Impact Assessment Methods: Database and Supporting Information, 2012, DOI:10.2788/60825.
- S. Sala, E. Crenna, M. Secchi and E. Sanyé-Mengual, Environmental Sustainability of European Production and Consumption Assessed against Planetary Boundaries, J. Environ. Manage., 2020, 269(110668) DOI:10.1016/j.jenvman.2020.110686.
- H. Huang, G. B. Hwang, G. Wu, K. Karu, H. Du Toit, H. Wu, J. Callison, I. P. Parkin and A. Gavriilidis, Rapid Synthesis of [Au25(Cys)18] Nanoclusters via Carbon Monoxide in Microfluidic Liquid-Liquid Segmented Flow System and Their Antimicrobial Performance, Chem. Eng. J., 2020, 383(123176) DOI:10.1016/j.cej.2019.123176.
- G. B. Hwang, H. Huang, G. Wu, J. Shin, A. Kafizas, K. Karu, H. Toit, A. M. Alotaibi, L. Mohammad-Hadi, E. Allan, A. J. MacRobert, A. Gavriilidis and I. P. Parkin, Photobactericidal Activity Activated by Thiolated Gold Nanoclusters at Low Flux Levels of White Light, Nat. Commun., 2020, 11(1), 4–13, DOI:10.1038/s41467-020-15004-6.
- G. B. Hwang, G. Wu, J. Shin, L. Panariello, V. Sebastian, K. Karu, E. Allan, A. Gavriilidis and I. P. Parkin, Continuous Single-Phase Synthesis of [Au 25 (Cys) 18 ] Nanoclusters and Their Photobactericidal Enhancement, ACS Appl. Mater. Interfaces, 2020, 12(43), 49021–49029, DOI:10.1021/acsami.0c07691.
- S. Perni, P. Prokopovich, C. Piccirillo, J. Pratten, I. P. Parkin and M. Wilson, Toluidine Blue-Containing Polymers Exhibit Potent Bactericidal Activity When Irradiated with Red Laser Light, J. Mater. Chem., 2009, 19(18), 2715–2723, 10.1039/b820561b.
- S. Noimark, E. Allan and I. P. Parkin, Light-Activated Antimicrobial Surfaces with Enhanced Efficacy Induced by a Dark-Activated Mechanism, Chem. Sci., 2014, 5, 2216, 10.1039/c3sc53186d.
- C. Piccirillo, S. Perni, J. Gil-Thomas, P. Prokopovich, M. Wilson, J. Pratten and I. P. Parkin, Antimicrobial Activity of Methylene Blue and Toluidine Blue O Covalently Bound to a Modified Silicone Polymer Surface, J. Mater. Chem., 2009, 19(34), 6167–6171, 10.1039/b905495b.
- A. Sani, C. Cao and D. Cui, Toxicity of Gold Nanoparticles (AuNPs): A Review, Biochem. Biophys. Rep., 2021, 26, 100991, DOI:10.1016/j.bbrep.2021.100991.
- G. Fitz Gerald, G. Moore and A. P. R. Wilson, Hand Hygiene after Touching a Patient's Surroundings: The Opportunities Most Commonly Missed, J. Hosp. Infect., 2013, 84(1), 27–31, DOI:10.1016/j.jhin.2013.01.008.
-
National Patient Safety Agency, The NHS Cleaning Manual, 2009 Search PubMed.
- Medigenic, https://www.medigenic.net/ (accessed 2019-02-04).
-
S. Morter, Trust Guideline for the Cleaning and Disinfection in the Hospital Trust Guideline for the Cleaning and Disinfection in the Hospital, 2019 Search PubMed.
- A. P. R. Wilson, P. Ostro, M. Magnussen and B. Cooper, Laboratory and In-Use Assessment of Methicillin-Resistant Staphylococcus Aureus Contamination of Ergonomic Computer Keyboards for Ward Use, Am. J. Infect. Control, 2008, 36(10), 19–25, DOI:10.1016/j.ajic.2008.09.001.
- C. Zeng, Y. Chen, A. Das and R. Jin, Transformation Chemistry of Gold Nanoclusters: From One Stable Size to Another, J. Phys. Chem. Lett., 2015, 6(15), 2976–2986, DOI:10.1021/acs.jpclett.5b01150.
-
TURAS Data Intelligence, NHSScotland Workforce, https://turasdata.nes.nhs.scot/workforce-official-statistics/nhsscotland-workforce/.
-
NHS Digital, NHS Workforce Statistics, https://digital.nhs.uk/data-and-information/publications/statistical/nhs-workforce-statistics.
-
J. O. Hagan, S. Court and C. Council, Health and Social Care Northern Ireland Quarterly Workforce Bulletin December 2019 Key Findings Responsible Statistician: Statistics Contact, 2020, vol. 222, pp. 8–9 Search PubMed.
- Eurostat, Electricity Price Statistics - Statistics Explained, Eur. Comm., 2020, 1–12 Search PubMed.
-
UCL, Manufacturing Advanced Functional Materials, https://www.ucl.ac.uk/chemical-engineering/research/manufacturing-advanced-functional-materials-mafuma.
-
Clinell, Safety Data Sheet. Clinell Alcohol Wipes, 2019 Search PubMed.
-
Gama Healthcare, Clinell: alcohol wipes range. How to use, https://gamahealthcare.com/products/alcohol-wipes-range.
-
NHS National Healthcare System, NHS supply chain, https://my.supplychain.nhs.uk/Catalogue/product/vjt421 (accessed 2019-06-13).
- G. K. X. Poh, I. M. L. Chew and J. Tan, Life Cycle Optimization for Synthetic Rubber Glove Manufacturing, Chem. Eng. Technol., 2019, 42(9), 1771–1779, DOI:10.1002/ceat.201800476.
-
DEFRA, UK Statistics on Waste Key Points Data Revisions in This Update, 2020 Search PubMed.
-
M. Marinussen and A. Kool, Environmental Impacts of Synthetic Amino Acid Production, Blonk Milieu Advies, Gouda, 2010, pp. 1–15 Search PubMed.
-
EC JRC, ILCD Handbook: Recommendations for Life Cycle Impact Assessment in the European Context, 2011, DOI:10.278/33030.
-
L. Benini, L. Mancini, S. Sala, E. Schau, S. Manfredi and R. Pant, Normalisation Method and Data for Environmental Footprints, 2014, DOI:10.2788/16415.
- M. A. J. Huijbregts, Z. J. N. Steinmann, P. M. F. Elshout, G. Stam, F. Verones, M. Vieira, M. Zijp, A. Hollander and R. van Zelm, ReCiPe2016: A Harmonised Life Cycle Impact Assessment Method at Midpoint and Endpoint Level, Int. J. Life Cycle Assess., 2017, 22(2), 138–147, DOI:10.1007/s11367-016-1246-y.
-
M. Goedkoop and M. Huijbregts, ReCiPe 2008 Characterisation, 2013, pp. 4–20 Search PubMed.
- M. D. M. Vieira, T. C. Ponsioen, M. J. Goedkoop and M. A. J. Huijbregts, Surplus Ore Potential as a Scarcity Indicator for Resource Extraction, J. Ind. Ecol., 2017, 21(2), 381–390, DOI:10.1111/jiec.12444.
- A. Bjørn, Review of Life-Cycle Based Methods for Absolute Environmental Sustaiability Assessment and Their Applications, Environ. Res. Lett., 2020, 15(8) DOI:10.1088/1748-9326/ab89d7Manuscript.
-
The World Bank, Population, total, https://data.worldbank.org/indicator/SP.POP.TOTL (accessed 2020-09-01).
-
R. K. Rosenbaum, S. Georgiadis and P. Fantke, Uncertainty Management and Sensitivity Analysis. Life Cycle Assessment, 2017, ch. 11, DOI:10.1111/jiec.12157.
- Y. Yu, Z. Luo, Y. Yu, J. Y. Lee and J. Xie, Observation of Cluster Size Growth in CO-Directed Synthesis of Au 25 (SR) 18 Nanoclusters, ACS Nano, 2012, 6(9), 7920–7927, DOI:10.1021/nn3023206.
- P. P. Pichler, I. S. Jaccard, U. Weisz and H. Weisz, International Comparison of Health Care Carbon Footprints, Environ. Res. Lett., 2019, 14(6) DOI:10.1088/1748-9326/ab19e1.
-
The NHS Net Zero Expert Panel, Delivering a “Net Zero” National Health Service, 2020 Search PubMed.
- B. Fritz, C. Aichele and M. Schmidt, Environmental Impact of High-Value Gold Scrap Recycling, Int. J. Life Cycle Assess., 2020, 25(10), 1930–1941, DOI:10.1007/s11367-020-01809-6.
|
This journal is © The Royal Society of Chemistry 2022 |