Use and release of per- and polyfluoroalkyl substances (PFASs) in consumer food packaging in U.S. and Canada†
Received
20th April 2022
, Accepted 17th August 2022
First published on 11th October 2022
Abstract
Numerous per- and polyfluoroalkyl substances (PFASs) occur in consumer food packaging due to intentional and unintentional addition, despite increasing concern about their health and environmental hazards. We present a substance flow analysis framework to assess the flows of PFASs contained in plant fiber-based and plastic food packaging to the waste stream and environment. Each year between 2018 and 2020, an estimated 9000 (range 1100–25
000) and 940 (range 120–2600) tonnes per year of polymeric PFASs were used in 2% of food packaging in the U.S. and Canada, respectively. At least 11 tonnes per year of non-polymeric PFASs also moved through the food packaging life cycle. Approximately 6100 (range 690–13 000) and 700 (range 70–1600) tonnes per year of these PFASs were landfilled or entered composting facilities in the U.S. and Canada, respectively, with the potential to contaminate the environment. The results suggest that minimal food packaging contains intentionally added PFASs which, nonetheless, has the potential to contaminate the entire waste stream. Further, this indicates that PFASs are not needed for most food packaging. These results serve as a benchmark to judge the effectiveness of future industry and government initiatives to limit PFAS use in food packaging.
Environmental significance
Although we estimate that only ∼2% of U.S. and Canadian food packaging contains intentionally added PFASs, this level of addition results in PFASs flows of 9000 (range 1100–25 000) and 940 (range 120–2600) tonnes per year. Most of the PFASs are polymeric, but we estimate a minimum of 11 tonnes per year of nonpolymeric and hence mobile and potentially bioaccumulative PFASs are contained in U.S. and Canadian food packaging. PFASs in food packaging have the potential to contaminate the waste stream (e.g., recycled materials, compost, landfill leachate), and the environment from releases to air, water and soils. The estimated low usage of intentionally added PFASs suggests that alternatives are available and hence PFASs can be eliminated from this non-essential use. The prevalence of non-intentionally occurring PFASs in food packaging is more problematic. Our estimates are a benchmark for comparison, as jurisdictions deliberate PFASs reduction strategies, e.g., U.S. EPA PFAS Strategic Roadmap of 2021, and as other jurisdictions may follow the 7 U.S. states that have adopted legislative bans on PFAS use in food contact materials.
|
1 Introduction
Per- and polyfluoroalkyl substances (PFASs) are a class of more than 4700 non-polymeric (e.g., perfluoroalkyl acids) and polymeric (e.g., fluoropolymers, perfluoropolyethers, and side-chain fluorinated polymers) substances.1 PFASs have been detected in paper- and plastic-based consumer food packaging, e.g., popcorn microwave bags,2 dessert/bread wrappers,3 Tex Mex food packaging3 and “eco-friendly” plant fiber-based food packaging.4 Previous studies have revealed the presence of perfluoroalkylcarboxylic acids (PFCAs), perfluoroalkanesulfonic acids (PFSAs), fluorotelomer alcohols (FTOHs) and phosphate esters (PAPs and diPAPs) in food packaging,3,5–10 with recent evidence of the use of polymers such as side-chain fluorinated polymers.11,12 A recent European-wide survey has confirmed the widespread use of PFASs in disposable food packaging and tableware.13
The strong chemical and biological stability of the perfluorinated carbon moieties1,14 leads to the high persistence and accumulation of many PFASs in the environment,15,16 biota17,18 and humans,19,20 with the potential for serious environmental and human health harm.21,22 Some PFASs present in food packaging can be transferred to food or air through food contact and heating processes, leading to human exposure through ingestion and inhalation.23,24 Disposal of packaging waste can lead to the environmental release of PFASs via landfill leachate,25,26 compost application27 and biosolids, and effluent discharges from wastewater treatment plants (resulting from inputs due to human exposure).28–30
The United States (U.S.), through the Food and Drug Administration (FDA), has a list of PFASs authorized for use in food packaging;31 however, information is lacking on the actual usage of these PFASs in food packaging production, or whether manufacturers are using non-authorized PFASs. Canadian regulatory oversight of PFASs use in food packaging is more complicated due to the lack of a public list of authorized PFASs (ESI, Section S1 and Table S1†).32 However, as a signatory to the Stockholm Convention, Canada restricted the use, sale and importation of PFOS and PFOS-containing products in 2008 with exemptions, and in 2012 restrictions were applied to PFOA, its salts and precursors, namely long-chain or LC-PFCAs, again with specific exemptions.33–35 A further tightening of these regulations with reduced exemptions was recently introduced to the Canadian Parliament.36
The U.S. Environmental Protection Agency (EPA) initiated the PFOA (perfluorooctanoic acid) 2010/2015 Stewardship program in 2006, including PFOA, its long-chain homologues and their precursors. In 2020, a voluntary agreement was struck between the U.S. FDA and manufacturers of grease-proofing agents used in paper and paperboard food packaging, to phase out the use of PFASs derived from 6
:
2 FTOH by 2024.37 This agreement was prompted by new evidence suggesting significant potential human health risks arising from chronic dietary exposure.38 Further, a recent report from the Organisation for Economic Co-operation and Development (OECD) has argued for more regulation of these compounds in food packaging.39
Since the 2006 agreement, PFASs manufacturers in North America and Europe largely replaced long-chain PFASs with, for example, shorter-chain homologues and other PFASs such as perfluoropolyethers. Short-chain replacements are as persistent as long-chain PFASs, and even more difficult to remove from water treatment systems.40 The other major replacement, polymeric PFASs, notably side-chain fluorinated polymers, also present environmental and human health concerns because they contain non-polymeric PFASs impurities and can degrade into non-polymeric, persistent and mobile PFASs.11,14,28,41,42
Little is known about the fate of PFASs used in food packaging, such as how much is released into the environment and from where these releases occur. Substance flow analysis (SFA) is a useful tool for understanding the major inputs and outputs of a system in order to prioritise action,43 including, for example, PFASs in carpets.44 To date, the stock and flows of PFASs that transfer PFASs used in food packaging to various waste streams have not been estimated. Our goals were to develop a general SFA framework for PFASs in food packaging and, as a case study, apply it to the U.S. and Canada for a year representative of 2018 to 2020. This case study illustrates the potential of PFASs used in food packaging to contaminate the waste stream and environment, and it provides a benchmark against which to judge the effectiveness of jurisdictional initiatives to limit the use of PFASs in food packaging.
2 Methods
Substance flow analysis framework
The SFA framework includes the three life stages of food packaging: production, use and end-of-life (Fig. 1 and ESI Section S2†). Five transient and permanent sinks of PFASs were identified: food packaging storage, human storage, landfill storage, destruction by incineration, and environmental storage. Eqn (1) describes the variation of PFAS mass (MPFAS) in food packaging over time. |  | (1) |
where FFP is the flow of PFASs produced for food packaging, F4,1 is the flow from discarded food packaging that is recycled back to food packaging, F1 is the flow from the sales of food packaging, Fproduction is the flow released to the environment (i.e., air and surface water) during the production of food packaging, and Fstorage is the outflow in stored food packaging.
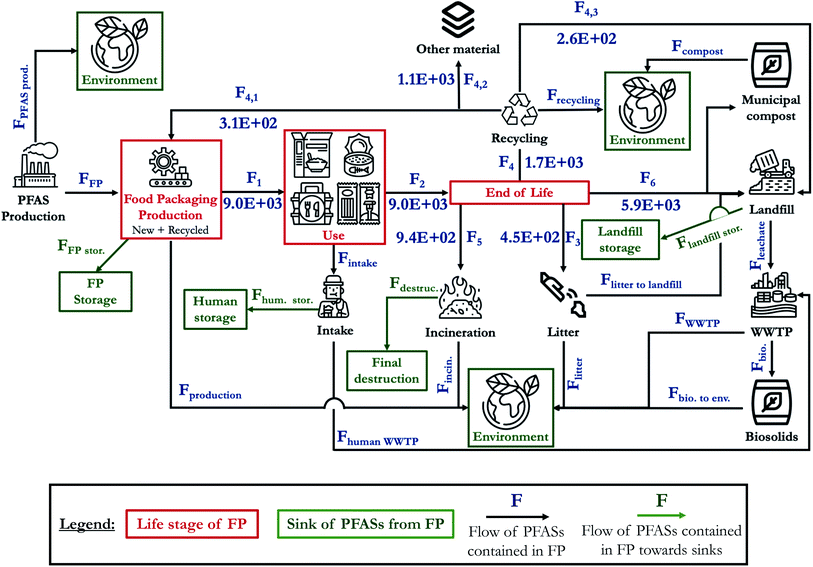 |
| Fig. 1 A schematic overview of the general substance flow analysis (SFA) framework for PFASs in consumer food packaging (FP). The numbered flows are quantified in the case study. “Environment” is used to designate air and surface water. Values indicated correspond to the estimated flows of total PFASs in the U.S. (in tonnes per year). | |
Fig. 1 describes the flows of PFASs present in consumer food packaging. The framework includes releases during PFASs production as a potential source to the environment,1 as identified by FPFAS
prod in Fig. 1. We also note that municipal compost originates from post-consumer use of food packaging that is sent to municipally run composting facilities and biosolids originate from wastewater treatment plants. Additionally, we have included the land application of biosolids for agricultural purposes through the flow as Fbio.
to
env.
Based on their PFAS content and origin (described in ESI Section S1†), food packaging can be divided into three categories. Category 1 (“functional” category) corresponds to food packaging with PFASs intentionally added, including plant fiber-based packaging with sufficient PFAS(s) to confer water- and grease-repellency, such as popcorn bags45 and plant fiber-based (marketed as “compostable”) bowls,3 as well as plastic packaging with fluoropolymers as extrusion aids.46,47 Category 2 (“non-functional” category) includes food packaging with the unintentional addition of PFASs during manufacturing (e.g., from recycled materials, cross contamination, or lubricants in machinery used to produce packaging) in a quantity insufficient to provide functionality such as repellency properties.48 Category 3 (“no PFASs” category) corresponds to food packaging free of PFASs.
SFAs were parameterised for the three categories of food packaging (see above) and two groups of PFASs: first, polymeric ones (including side-chain fluorinated polymers and perfluoropolyethers that are usually contained in paper-based food packaging,49 and fluoropolymers that have been found in plastic-based food packaging50), and, second, non-polymeric compounds, e.g., fluorotelomer (FT) alcohols (n:2 FTOHs), FT sulfonates (n:2 FTSs), FT acrylates (n:2 FTAcs), FT methacrylates (n:2 FTMAcs).
Case study
We constructed two SFAs for food packaging (e.g., paperboard, plastic pouches, fast food wrapping paper, plant-fiber bowls): one for the sum of polymeric PFASs and another for the sum of non-polymeric PFASs. Many of these non-polymeric compounds can be production residuals, impurities, by-products and degradation products of polymeric PFASs, or unintentional contaminants.11,48
We considered a reference year between 2018 and 2020. Only a limited number of flows could be estimated due to a lack of information. To address some of these data gaps and to recognize the uncertainty in our estimates, we provided a range of estimates approximating low, middle and high scenarios, presented as middle (low–high), for parameter values.
For the two categories of food packaging containing PFASs, i.e., with functional (e.g. PFASs added to confer grease- and water-repellency) and non-functional (e.g. inadvertent PFASs that do not confer grease- and water-repellency) concentrations, we estimated the polymeric and non-polymeric PFASs content as a middle value bounded by low and high estimates, by combining information provided in the U.S. FDA Inventory of Effective Food Contact Substance Notifications31 (ESI Table S1†) with data from the literature.
Using information provided in patents,51 we assumed the maximum concentration of polymeric PFASs in food packaging with functional PFASs addition (Cfunctional) to be 1.2% by weight of finished packaging (high scenario), and the middle scenario to be 0.5% (i.e., approximately half the maximum value recommended for use) (Table 1 and ESI Section S3†). The minimum concentration to confer functionality such as water- and oil-repellency is 0.1% by weight of finished paper.51 For food packaging with non-functional addition of PFASs, we took 0.01% by weight of finished paper as the maximum concentration of polymeric PFAS, which is the 10% of the minimum concentration assumed for functional addition of PFASs, and 0 and 0.005% for the low and middle scenarios, respectively.
Table 1 Input data for the substance flow analysis case study (FP = food packaging)a
Parameter/type of FP |
Low |
Middle |
High |
Source |
Functional refers to FP with PFASs added to confer grease- and water-repellency and non-functional refers to FP with inadvertent PFASs addition that does not confer grease- and water-repellency.
|
PFAS concentrations in FP
|
Non-polymeric PFASs |
C
functional
|
3.7 × 10−5% |
2.8 × 10−4% |
2.5 × 10−3% |
Literature (ESI Section S3.1) |
C
non-functional
|
0 |
2.0 × 10−5% |
3.7 × 10−5% |
Literature (ESI Section S3.1) |
C
no PFAS
|
0 |
0 |
0 |
— |
Polymeric PFASs |
C
functional
|
0.1% |
0.5% |
1.2% |
Patent51 |
C
non-functional
|
0 |
0.005% |
0.01% |
Assumed |
C
no PFAS
|
0 |
0 |
0 |
— |
![[thin space (1/6-em)]](https://www.rsc.org/images/entities/char_2009.gif) |
Proportion of FP types
|
%functional |
Category “functional” |
2% |
2% |
2% |
Back calculation and assumed |
%non-functional |
Category “non-functional” |
40% |
50% |
60% |
Back calculation and assumed |
%no PFAS |
Category “no PFAS” |
58% |
48% |
38% |
Back calculation and assumed |
![[thin space (1/6-em)]](https://www.rsc.org/images/entities/char_2009.gif) |
Mass of FP in municipal solid waste
|
Canada |
M
FP (megatonnes) |
5.9 |
7.6 |
8.8 |
Government of Canada,61 Statistics Canada62 |
U.S. |
57 |
72 |
83 |
U.S. EPA,63,64 Government of Canada61 |
![[thin space (1/6-em)]](https://www.rsc.org/images/entities/char_2009.gif) |
Proportion of FP littered
|
%litter |
5.0% |
15% |
30% |
Assumed |
![[thin space (1/6-em)]](https://www.rsc.org/images/entities/char_2009.gif) |
Proportion of FP recycled
|
%FP recycled |
10% |
20% |
30% |
Government of Canada,61,65 U.S. EPA64 |
![[thin space (1/6-em)]](https://www.rsc.org/images/entities/char_2009.gif) |
Proportion of FP recycled to FP
|
%FP recycled to FP |
5.0% |
18% |
33% |
U.S. EPA53 |
![[thin space (1/6-em)]](https://www.rsc.org/images/entities/char_2009.gif) |
Proportion of FP intended for recycling sent to landfills or exported
|
%recycling to landfill |
5.0% |
15% |
30% |
NY Times,54 CTV news,55 Globe and Mail56 |
![[thin space (1/6-em)]](https://www.rsc.org/images/entities/char_2009.gif) |
Proportion of FP incinerated
|
Canada |
%incineration |
3.0% |
5.0% |
8.0% |
Statistics Canada,57 Solid Waste & Recycling58 |
U.S. |
4.0% |
11% |
17% |
U.S. EPA59 |
![[thin space (1/6-em)]](https://www.rsc.org/images/entities/char_2009.gif) |
Proportion of Canadian FP waste sent to Michigan
|
%Can. Waste to Mich |
5.0% |
11% |
20% |
Michigan Department of Environment60 |
For non-polymeric PFASs in the “functional” category, we used 370, 2820 and 25
200 ng g−1 for the lower, middle and high values, respectively, estimated from the list of PFASs authorized for use by the U.S. Food and Drug Administration and from Liu et al. (see ESI Table S1†).31,52 For food packaging with non-functional addition of non-polymeric PFASs, we used 370 ng g−1 as our upper boundary, and 0 ng g−1 as the minimum, with 200 ng g−1 as the middle value. A complete listing of data sources and explanations for these choices are provided in ESI Section S3.1.†
We note that the SFA for polymeric PFASs provides inputs to the non-polymeric PFASs SFA through the release of side chains and other processes of polymeric breakdown. However, we had insufficient data to quantify this transfer and therefore did not quantify releases from polymeric breakdown as an input to the non-polymeric PFASs SFA. All values used in the SFA are listed in Table 1 with full details in ESI Section S2.†
F
1: flow in the sales of food packaging.
The flow in the sales of food packaging (F1) was calculated as: | F1 = MFP × (Cfunctional × %functional + Cnon-functional × %non-functional + CnoPFAS × %noPFAS) | (2) |
where MFP is the mass of food packaging generated in a given year (ESI Section S2.3†); Cfunctional, Cnon-functional, Cno
PFAS are the concentrations in each of the three categories of food packaging, which are present in the %functional, %non-functional, and %no
PFAS percentages, respectively. Due to a lack of data, the percentages of food packaging types were assumed such that the estimated flows accorded with 10
000 (1080–21
600) tonnes of PFASs used in food packaging annually in the U.S., which we estimated based on data available in the literature. We used the same percentages for Canada (ESI Section S2.3 and S3.2†) (Table 1).
F
2: flow in discarded food packaging.
We assumed a short lifetime of food packaging, i.e., all food packaging from food bought in a year is discarded the same year since food is typically consumed the same year it was purchased. Further, we assumed that significant loss during the lifetime of food packaging is minimal, although some losses occur.23,24 The flow in discarded food packaging, F2, is | F2 = F1 − Fintake ≅ F1 | (3) |
where Fintake is the flow of PFASs from food packaging digested or inhaled by humans resulting from food heating or consumption. Since we had insufficient information to estimate the human intake flow, we assumed that this intake would be negligible compared to the amount remaining within the food packaging (ESI Section S2.4†).
F
3: flow in littered food packaging.
A small portion of food packaging waste is littered, quantified aswhere %litter is the percentage of food packaging that is littered. Values for %litter were assumed (ESI Section 2.5).
F
4: flow in recycled food packaging.
The flow in recycled food packaging, F4, is the sum of several flows which depend on the percentage of food packaging recycled (%FP
recycled). It was divided into four parts, | F4 = F4,1 + F4,2 + F4,3 + Frecycling | (5) |
| F4 = (F2 − F3) × %FP recycled | (6) |
| F4,1 = F4 × %FP recycled to FP | (7) |
| F4,2 ≅ F4 − F4,1 − F4,3 | (8) |
| F4,3 = F4 × %recycling to landfill | (9) |
F
4,1 is the flow from recycled food packaging that is recycled back to food packaging, which depends on the percentage of food packaging recycled back to food packaging, %FP
recycled
to
FP, estimated using data on the use of recycled paper in the production of new paper in the U.S.53F4,2 is the flow from recycled food packaging that is recycled into other materials. F4,3 is the flow from food packaging intended for recycling but that is ultimately landfilled or exported (%recycling
to
landfill), which could represent up to one-third of discarded food packaging.54–56Frecycling is the flow from the recycling process of food packaging emitted to the environment, which is assumed to be negligible due to a lack of information sufficient for quantification (ESI Section S2.6†).
F
5: flow in incinerated food packaging.
The flow of PFASs in incinerated food packaging depends on the percentage of food packaging in the waste stream that is sent to incineration (%incineration) (Table 1 and ESI Section 2.7†). Values for %incineration were taken from U.S. and Canadian sources where waste incineration is more common in the U.S. than in Canada.57–59 | F5 = (F2 − F3) × %incineration | (10) |
F
6: flow in landfilled and composted food packaging.
Food packaging that is not littered, incinerated or recycled is sent to landfill and composting facilities in the U.S., Canada, or abroad (ESI Section 2.8†). We did not have access to data distinguishing municipal garbage that is landfilled versus composted, and thus have combined both flows into F6 (which separates into F6-compost and F6-landfill). Some waste for landfilling from Canada (specifically Ontario) is sent to Michigan, U.S., by mutual agreement (F6,
Michigan, %Can.
waste
to
Mich.),60 where MMich and MCan represent the mass of Canadian waste sent to Michigan and Canada landfills, respectively. | F6 = F2 − F3 – F4 − F5 | (11) |
For Canada more specifically:
| F6 = F6,Canada + F6,Michigan | (12) |
|  | (13) |
3 Results and discussion
The inflows of polymeric PFASs were 9000 (range 1100–25
000) and 940 (range 120–2600) tonnes per year for middle (low–high) scenarios in the U.S. and Canada, respectively (Fig. 1 and Table 2). Of this total inflow, >99% were polymeric forms. The total estimate inflows of non-polymeric PFASs were 11 (range 0.42–60) and 1.2 (range 0.044–6.4) tonnes per year for the U.S. and Canada, respectively. Total inflows were dominated by the intentional addition of PFASs: ∼80% and 35% of the polymeric and non-polymer PFASs flows, respectively, were estimated to have come from food packaging with PFASs added at levels to confer grease and water repellency (ESI Tables S9 and S10†). Conversely, 65% of the non-polymeric (environmentally mobile) inflow of 7.2 tonnes per year or ∼0–18 tonnes per year combined for U.S. and Canada, was attributable to the occurrence of PFASs found in food packaging at non-functional concentrations. The total PFASs inflow was due to ∼2% and 40–60% of total food packaging containing functional and non-functional addition of PFASs, respectively.
Table 2 Main calculated flows of PFASs from consumer food packaging in the U.S. and Canada (in tonnes per year), along with the percentage of the total inflow (i.e., F1) they represent
|
U.S. |
Canada |
Low |
Middle |
High |
Low |
Middle |
High |
Polymeric PFASs
|
F
1
|
Flow in the sales of FP |
1.13 × 103 |
9.03 × 103 |
2.49 × 104 |
1.18 × 102 |
9.43 × 102 |
2.64 × 103 |
F
2
|
Flow in discarded FP |
1.13 × 103 |
9.03 × 103 |
2.49 × 104 |
1.18 × 102 |
9.43 × 102 |
2.64 × 103 |
0% |
0% |
0% |
0% |
0% |
0% |
F
3
|
Flow in littered FP |
3.39 × 102 |
4.51 × 102 |
3.74 × 103 |
3.55 × 101 |
4.71 × 101 |
3.96 × 102 |
30% |
5% |
15% |
30% |
5% |
15% |
F
4
|
Flow in recycled FP |
7.92 × 101 |
1.71 × 103 |
6.36 × 103 |
8.28 |
1.79 × 102 |
6.73 × 102 |
7% |
19% |
26% |
7% |
19% |
26% |
F
4,1
|
Flow in recycled FP that is recycled back to FP |
3.96 |
3.09 × 102 |
2.10 × 103 |
4.14 × 10−1 |
3.22 × 101 |
2.22 × 102 |
0% |
3% |
8% |
0% |
3% |
8% |
F
4,2
|
Flow in recycled FP that is recycled into other materials |
7.13 × 101 |
1.15 × 103 |
2.35 × 103 |
7.45 |
1.20 × 102 |
2.49 × 102 |
6% |
13% |
9% |
6% |
13% |
9% |
F
4,3
|
Flow in FP initially intended for recycling but that is ultimately landfilled or exported |
3.96 |
2.57 × 102 |
1.91 × 103 |
4.14 × 10−1 |
2.69 × 101 |
2.02 × 102 |
0% |
3% |
8% |
0% |
3% |
8% |
F
5
|
Flow in incinerated FP |
3.17 × 101 |
9.43 × 102 |
3.60 × 103 |
2.48 |
4.48 × 101 |
1.80 × 102 |
3% |
10% |
14% |
2% |
5% |
7% |
F
6
|
Flow in landfilled and composted FP |
6.81 × 102 |
5.92 × 103 |
1.12 × 104 |
7.20 × 101 |
6.72 × 102 |
1.39 × 103 |
60% |
66% |
45% |
61% |
71% |
53% |
F
6,Michigan
|
Flow in landfilled FP sent to Michigan |
— |
— |
— |
3.60 |
7.39 × 101 |
2.78 × 102 |
— |
— |
— |
3% |
8% |
11% |
F
6,Canada
|
Flow in FP landfilled or composted in Canada |
— |
— |
— |
6.84 × 101 |
5.98 × 102 |
1.11 × 103 |
— |
— |
— |
58% |
63% |
42% |
![[thin space (1/6-em)]](https://www.rsc.org/images/entities/char_2009.gif) |
Non-polymeric PFASs
|
F
1
|
Flow in the sales of FP |
4.19 × 10−1 |
1.13 × 101 |
6.04 × 101 |
4.37 × 10−2 |
1.18 |
6.39 |
F
2
|
Flow in discarded FP |
4.19 × 10−1 |
1.13 × 101 |
6.04 × 101 |
4.37 × 10−2 |
1.18 |
6.39 |
0% |
0% |
0% |
0% |
0% |
0% |
F
3
|
Flow in littered FP |
1.26 × 10−1 |
5.63 × 10−1 |
9.06 |
1.31 × 10−2 |
5.88 × 10−2 |
9.58 × 10−1 |
30% |
5% |
15% |
30% |
5% |
15% |
F
4
|
Flow in recycled FP |
2.93 × 10−2 |
2.14 |
1.54 × 101 |
3.06 × 10−3 |
2.23 × 10−1 |
1.63 |
7% |
19% |
26% |
7% |
19% |
26% |
F
4,1
|
Flow in recycled FP that is recycled back to FP |
1.47 × 10−3 |
3.85 × 10−1 |
5.08 |
1.53 × 10−4 |
4.02 × 10−2 |
5.38 × 10−1 |
0% |
3% |
8% |
0% |
3% |
8% |
F
4,2
|
Flow in recycled FP that is recycled into other materials |
2.64 × 10−2 |
1.43 |
5.70 |
2.76 × 10−3 |
1.50 × 10−1 |
6.03 × 10−1 |
6% |
13% |
9% |
6% |
13% |
9% |
F
4,3
|
Flow in FP initially intended for recycling but that is ultimately landfilled or exported |
1.47 × 10−3 |
3.21 × 10−1 |
4.62 |
1.53 × 10−4 |
3.35 × 10−2 |
4.89 × 10−1 |
0% |
3% |
8% |
0% |
3% |
8% |
F
5
|
Flow in incinerated FP |
1.17 × 10−2 |
1.18 |
8.72 |
9.19 × 10−4 |
5.59 × 10−2 |
4.34 × 10−1 |
3% |
10% |
14% |
2% |
5% |
7% |
F
6
|
Flow in landfilled and composted FP |
2.52 × 10−1 |
7.38 |
2.72 × 101 |
2.66 × 10−2 |
8.38 × 10−1 |
3.37 |
60% |
66% |
45% |
61% |
71% |
53% |
F
6,Michigan
|
Flow in landfilled FP sent to Michigan |
— |
— |
— |
1.33 × 10−3 |
9.22 × 10−2 |
6.73 × 10−1 |
— |
— |
— |
3% |
8% |
11% |
F
6,Canada
|
Flow in FP landfilled or composted in Canada |
— |
— |
— |
2.53 × 10−2 |
7.46 × 10−1 |
2.69 |
— |
— |
— |
58% |
63% |
42% |
The scenario based on middle values provides the most realistic estimate of actual flows, benchmarked against an estimate of PFASs used in food packaging in the U.S. The flow of 9000 tonnes per year in the U.S. was about a third of the 21
600 tonnes per year of fluorinated substances that could be used annually for food packaging in the U.S. based on values from ∼2000 and 2015 (ESI Section S3.2†).5,66 Note that none of the upcoming bans in food packaging by three U.S. states or the U.S. voluntary phase-out from food packaging were considered in this analysis (the ban in New York will occur after 2022).67
All food packaging produced moved to end-of-life, according to our assumption of limited storage. For the U.S., ∼70% (60–50%, low–high values) or 6100 (range 690–13
000, flows F6 and F3.2) tonnes of polymeric PFASs from food packaging per year went to landfill and composting facilities, ∼5% (30–15%) or 450 (340–3700) tonnes per year to litter and ∼10% (3–14%) or 940 (32–3600) tonnes per year to incineration. An unknown fraction of food packaging sent to landfill and/or composting was sent abroad.68
As mentioned above, some breakdown of polymeric PFASs into non-polymeric forms is inevitable. However, degradation rates and yield, including degradation of side-chain fluorinated polymers, are highly uncertain.69,70 Van der Veen et al.71 studied releases from durable water-repellent clothes, measuring 5- to 100-fold increases of non-polymeric PFASs concentrations following weathering, probably due to the degradation and transformation of precursors used for the formation of the durable water-repellent polymers. Similar values were obtained by Schellenberger et al.72 These results suggest that even limited breakdown of the large mass of polymeric PFASs from food packaging would considerably increase the mass and flows of non-polymeric PFASs.
Releases of PFASs from food packaging to the environment occur at different life-cycle stages and from various sources. Wastewater treatment plants (WWTPs) receive PFASs from food packaging with associated releases from WWTPs to the environment through outgassing,73–76 biosolids applied to land,29,77,78 or the discharge of final effluent.79–82 Outgassing and leachate from landfills are substantial sources discharged to the environment. We estimated that every year at least 2.27 and 0.26 tonnes of non-polymeric PFASs are released to the U.S. and Canadian environment, respectively, due to the limited ability of WWTP to treat PFASs contained in landfill leachate (ESI Section S2.8†). Furthermore, Lang et al.25 estimated that the entire U.S. waste load (of which about 30% was food packaging) generates at least 563 to 638 kg per year of selected non-polymeric PFASs in landfill leachate, of which 5
:
3 fluorotelomer carboxylic acid (FTCA) was the dominant PFAS identified out of the limited PFASs analysed, noting that composition varies depending on the age of the landfill. Leachate is often directed to WWTPs that are not designed to efficiently treat PFASs.83
WWTPs also receive PFASs from food packaging via human waste, unquantified due to a lack of data. However, several studies have highlighted the tendency of PFASs to transfer from food packaging to food,2,23 which leads to ingestion through food consumption.84 The heating process of food packaging, such as popcorn microwave bags, can also lead to airborne releases of PFASs which can be inhaled.24
Although unquantified, recent analyses of municipal compost from the U.S. found higher concentrations of PFAAs in composts that included food packaging than in those that did not.27 Other major unquantified flows included PFASs released during the manufacturing and recycling of food packaging containing PFASs85–87 and from incineration.88,89
4 Implications and conclusions
This analysis is the first estimate of flows of PFASs used in food packaging – a total of 9000 and 940 tonnes per year in the U.S. and Canada, respectively. Of this, over 99% was polymeric, arising from an estimated intentional addition of PFASs to only ∼2% of food packaging. Despite this low rate of PFAS usage, our estimates indicate the potential to contaminate the entire waste stream, including materials intended for recycling, landfilling and litter.
In addition to illustrating the potential of PFASs used in food packaging to contaminate the environment, numerous parts of the waste stream, and new products that contain recycled paper content, these results highlight the lack of knowledge regarding the overall presence and fate of PFASs in food packaging. While substance-based use and emission estimates of PFASs species and groups have been compiled,42,69,90–92 it is difficult to compare the flows of PFASs in food packaging estimated here for the U.S. and Canada with PFASs used in other applications because national product-based inventories of PFAS uses and emissions have not been compiled. The one exception is of PFASs used in carpets in California, for which the flows to landfill are orders-of-magnitude lower than the flows of food packaging estimated in this study, on a per capita basis, likely due to the high recycling rate of carpets in the U.S.44
For environmental releases, particularly glaring knowledge gaps regarding PFASs use in food packaging, which are also instructional for other PFASs uses, are releases during the production with implications for human exposure, and fate at end-of-life (including food packaging intended for recycling but that is exported to low-income countries).93,94 Another knowledge gap is the contribution of polymeric PFASs to non-polymeric forms due to the presence of non-polymeric impurities, and degradation in landfills and the environment. Although total flows were dominated by polymeric PFASs, of concern are non-polymeric flows of at least ∼11 and 1.2 tonnes per year in the U.S. and Canada, given the mobility and higher toxicity of these substances. These knowledge gaps stress the need for chemicals management regimes to take a comprehensive life-cycle approach that considers all stages, from manufacturing to end-of-life, and types of PFASs, when adjudicating the use of chemicals in food packaging and other applications, which is not currently the case in the U.S. or in Canada.31,32
The flows of non-functionally added PFASs in food packaging (estimated at ∼20% of total PFASs flows), including 7.2 and 0.75 tonnes per year in the U.S. and Canada, respectively, of non-polymeric PFASs, highlight our lack of control of PFASs entering food packaging from unintentional use. However, it is the low intentional use of PFASs in food packaging (∼2% of the total food packaging) that leads to the largest releases of PFASs, and highlights hotspots/blindspots for the sound management of food packaging.95,96
These results, specifically the low percentage (∼2%) of food packaging with intentionally added PFASs, lend support to regulatory initiatives to eliminate the use of PFASs in food packaging in seven U.S. states,67 the voluntary agreement brokered between the food packaging industry and the U.S. FDA to restrict which PFAS can be used in food packaging,37 and initiatives among several European countries to restrict non-essential uses of PFAS.97–99 We question whether these initiatives would be able to control flows of PFASs from non-intentional introduction to food packaging in these jurisdictions. Canada has not yet introduced restrictions for PFASs use specific to food packaging and, as noted above, the regulatory process is neither transparent or comprehensive.100,101
Given the high persistence and potential for harm of the entire class of PFASs, both polymers and non-polymers, this analysis leads to questioning the use of PFASs in food packaging in any jurisdiction.
Conflicts of interest
There are no conflicts to declare.
Acknowledgements
We thank Graham White, Anastase Rulibikiye and Abdul Afghan (Health Canada) for discussions on chemicals management, and gratefully acknowledge the assistance of Alec Gonzales, Matt Roddy, Nick Caterisano, Chase Miller and Molly LaDuca in acquiring PIGE data, and Saleena Hak for shopping. Funding was provided by Environment and Climate Change Canada, Great Lakes Protection Initiative (3000694876, GCXE21P039), Natural Sciences and Engineering Research Council of Canada (NSERC, RGPIN-2017-06654), and by charitable contributions to the Green Science Policy Institute, including from the Target Foundation.
References
- R. C. Buck, J. Franklin, U. Berger, J. M. Conder, I. T. Cousins, P. de Voogt, A. A. Jensen, K. Kannan, S. A. Mabury and S. P. van Leeuwen, Perfluoroalkyl and polyfluoroalkyl substances in the environment: Terminology, classification, and origins, Integr. Environ. Assess. Manage., 2011, 7, 513–541 CrossRef CAS PubMed.
- T. H. Begley, K. White, P. Honigfort, M. L. Twaroski, R. Neches and R. A. Walker, Perfluorochemicals: Potential sources of and migration from food packaging, Food Addit. Contam., 2005, 22, 1023–1031 CrossRef CAS PubMed.
- L. A. Schaider, S. A. Balan, A. Blum, D. Q. Andrews, M. J. Strynar, M. E. Dickinson, D. M. Lunderberg, J. R. Lang and G. F. Peaslee, Fluorinated Compounds in U.S. Fast Food Packaging, Environ. Sci. Technol. Lett., 2017, 4, 105–111 CrossRef CAS PubMed.
- G. Yuan, H. Peng, C. Huang and J. Hu, Ubiquitous Occurrence of Fluorotelomer Alcohols in Eco-Friendly Paper-Made Food-Contact Materials and Their Implication for Human Exposure, Environ. Sci. Technol., 2016, 50, 942–950 CrossRef CAS PubMed.
- X. Trier, K. Granby and J. H. Christensen, Polyfluorinated surfactants (PFS) in paper and board coatings for food packaging, Environ. Sci. Pollut. Res., 2011, 18, 1108–1120 CrossRef CAS PubMed.
- L. Schultes, G. F. Peaslee, J. D. Brockman, A. Majumdar, S. R. Mcguinness, J. T. Wilkinson, O. Sandblom, R. A. Ngwenyama and J. P. Benskin, Total Fluorine Measurements in Food Packaging: How Do Current Methods Perform?, Environ. Sci. Technol. Lett., 2019, 6, 73–78 CrossRef CAS.
- T. Shoeib, Y. Hassan, C. Rauert and T. Harner, Poly- and perfluoroalkyl substances (PFASs) in indoor dust and food packaging materials in Egypt: Trends in developed and developing countries, Chemosphere, 2016, 144, 1573–1581 CrossRef CAS PubMed.
- I. Zabaleta, E. Bizkarguenaga, D. Bilbao, N. Etxebarria, A. Prieto and O. Zuloaga, Fast and simple determination of perfluorinated compounds and their potential precursors in different packaging materials, Talanta, 2016, 152, 353–363 CrossRef CAS PubMed.
- I. Zabaleta, N. Negreira, E. Bizkarguenaga, A. Prieto, A. Covaci and O. Zuloaga, Screening and identification of per- and polyfluoroalkyl substances in microwave popcorn bags, Food Chem., 2017, 230, 497–506 CrossRef CAS PubMed.
- B. Barhoumi, S. G. Sander and I. Tolosa, A review on per- and polyfluorinated alkyl substances (PFASs) in microplastic and food-contact materials, Environ. Res., 2022, 206, 112595 CrossRef CAS PubMed.
-
D. of T. S. C. (DTSC) CalEPA, Product-Chemical Profile for Food Packaging Containing Perfluoroalkyl or Polyfluoroalkyl Substances: Discussion Draft, 2020 Search PubMed.
- K. L. Vorst, N. Saab, P. Silva, G. Curtzwiler and A. Steketee, Risk assessment of per- and polyfluoroalkyl substances (PFAS) in food: Symposium proceedings, Trends Food Sci. Technol., 2021, 116, 1203–1211 CrossRef CAS.
-
J. Straková, J. Schneider, N. Cingotti, A. Bennett, A. den Boer, K. Brabcová, S. Brosché, M. Fernandez, F. Gorre, E. Hawke, G. Jensen, N. Malval, M. Möller, S. Müller, A. Roger, F. Veillerette, B. Wahlund and J.-L. Wietor, Throwaway Packaging, Forever Chemicals: European-wide Survey of PFAS in Disposable Food Packaging and Tableware, 2021 Search PubMed.
- C. F. Kwiatkowski, D. Q. Andrews, L. S. Birnbaum, T. A. Bruton, J. C. Dewitt, D. R. U. Knappe, M. V. Maffini, M. F. Miller, K. E. Pelch, A. Reade, A. Soehl, X. Trier, M. Venier, C. C. Wagner, Z. Wang and A. Blum, Environ. Sci. Technol. Lett., 2020, 7, 532–543 CrossRef CAS PubMed.
- C. M. Butt, U. Berger, R. Bossi and G. T. Tomy, Sci. Total Environ., 2010, 408, 2936–2965 CrossRef CAS PubMed.
- S. Chu and R. J. Letcher, Side-chain fluorinated polymer surfactants in aquatic sediment and biosolid-augmented agricultural soil from the Great Lakes basin of North America, Sci. Total Environ., 2017, 607–608, 262–270 CrossRef CAS PubMed.
- M. Houde, A. O. De Silva, D. C. G. Muir and R. J. Letcher, Monitoring of perfluorinated compounds in aquatic biota: An updated review, Environ. Sci. Technol., 2011, 45, 7962–7973 CrossRef CAS PubMed.
- J. P. Giesy and K. Kannan, Peer Reviewed: Perfluorochemical Surfactants in the Environment, Environ. Sci. Technol., 2002, 36, 146A–152A CrossRef CAS PubMed.
- L. S. Haug, C. Thomsen and G. Becher, Time trends and the influence of age and gender on serum concentrations of perfluorinated compounds in archived human samples, Environ. Sci. Technol., 2009, 43, 2131–2136 CrossRef CAS PubMed.
- T. Zhang, Q. Wu, H. W. Sun, X. Z. Zhang, S. H. Yun and K. Kannan, Perfluorinated compounds in whole blood samples from infants, children, and adults in China, Environ. Sci. Technol., 2010, 44, 4341–4347 CrossRef CAS PubMed.
- C. Lau, K. Anitole, C. Hodes, D. Lai, A. Pfahles-Hutchens and J. Seed, Perfluoroaklyl acids: A review of monitoring and toxicological finds, Toxicol. Sci., 2007, 99, 366–394 CrossRef CAS PubMed.
- V. Barry, A. Winquist and K. Steenland, Perfluorooctanoic Acid (PFOA) Exposures and Incident Cancers among Adults Living Near a Chemical Plant, Environ. Health Perspect., 2013, 121, 1313–1318 CrossRef PubMed.
- T. H. Begley, W. Hsu, G. Noonan and G. Diachenko, Migration of fluorochemical paper additives from food-contact paper into foods and food simulants, Food Addit. Contam., Part A, 2008, 25, 384–390 CrossRef CAS PubMed.
- E. Sinclair, S. K. Kim, H. B. Akinleye and K. Kannan, Quantitation of gas-phase perfluoroalkyl surfactants and fluorotelomer alcohols released from nonstick cookware and microwave popcorn bags, Environ. Sci. Technol., 2007, 41, 1180–1185 CrossRef CAS PubMed.
- J. R. Lang, B. Mckay Allred, J. A. Field, J. W. Levis and M. A. Barlaz, National Estimate of Per-and Polyfluoroalkyl Substance (PFAS) Release to U.S. Municipal Landfill Leachate, Environ. Sci. Technol., 2017, 51, 2197–2205 CrossRef CAS PubMed.
- J. P. Benskin, B. Li, M. G. Ikonomou, J. R. Grace and L. Y. Li, Per- and polyfluoroalkyl substances in landfill leachate: Patterns, time trends, and sources, Environ. Sci. Technol., 2012, 46, 11532–11540 CrossRef CAS PubMed.
- Y. J. Choi, R. K. Lazcano, P. Yousefi, H. Trim and L. S. Lee, Perfluoroalkyl Acid Characterization in U.S. Municipal Organic Solid Waste Composts, Environ. Sci. Technol. Lett., 2019, 6, 372–377 CrossRef CAS.
- A. C. Blaine, C. D. Rich, L. S. Hundal, C. Lau, M. A. Mills, K. M. Harris and C. P. Higgins, Uptake of perfluoroalkyl acids into edible crops via land applied biosolids: Field and greenhouse studies, Environ. Sci. Technol., 2013, 47, 14062–14069 CrossRef CAS PubMed.
- J. Yu, J. Hu, S. Tanaka and S. Fujii, Perfluorooctane sulfonate (PFOS) and perfluorooctanoic acid (PFOA) in sewage treatment plants, Water Res., 2009, 43, 2399–2408 CrossRef CAS PubMed.
- A. B. Lindstrom, M. J. Strynar, A. D. Delinsky, S. F. Nakayama, L. McMillan, E. L. Libelo, M. Neill and L. Thomas, Application of WWTP biosolids and resulting perfluorinated compound contamination of surface and well water in Decatur, Alabama, USA, Environ. Sci. Technol., 2011, 45, 8015–8021 CrossRef CAS PubMed.
-
U.S. Food and Drug Administration (FDA), Inventory of Effective Food Contact Substance (FCS) Notifications, accessed 9 December 2020, https://www.cfsanappsexternal.fda.gov/scripts/fdcc/index.cfm?set=FCN%26sort=FCN_No%26order=DESC%26startrow=1%26type=basic%26search= Search PubMed.
- PackagingLaw.com, Canada Considering Positive List System for Food-Contact Materials, accessed 22 December 2020, https://www.packaginglaw.com/news/canada-considering-positive-list-system-food-contact-materials.
-
Stockholm Convention, Perfluorooctanoic acid (PFOA), its salts and PFOA-related compounds, accessed 23 November 2020, http://chm.pops.int/Implementation/Alternatives/AlternativestoPOPs/ChemicalslistedinAnnexA/PFOA/tabid/8292/Default.aspx Search PubMed.
-
Stockholm Convention, The new POPs under the Stockholm Convention, accessed 23 November 2020, http://www.pops.int/TheConvention/ThePOPs/TheNewPOPs/tabid/2511/Default.aspx Search PubMed.
-
United States Environmental Protection Agency, Fact Sheet: 2010/2015 PFOA Stewardship Program, accessed 21 September 2021, https://www.epa.gov/assessing-and-managing-chemicals-under-tsca/fact-sheet-20102015-pfoa-stewardship-program Search PubMed.
-
Government of Canada, Canada Gazette, Part 1, Volume 156, Number 20: Prohibition of Certain Toxic Substances Regulations, 2022, accessed 28 July 2022, https://www.gazette.gc.ca/rp-pr/p1/2022/2022-05-14/html/reg2-eng.html Search PubMed.
-
U.S. Food and Drug Administration (FDA), Authorized Uses of PFAS in Food Contact Applications, https://www.fda.gov/food/chemical-contaminants-food/authorized-uses-pfas-food-contact-applications, accessed 7 March 2022 Search PubMed.
- S. V. Kabadi, J. W. Fisher, D. R. Doerge, D. Mehta, J. Aungst and P. Rice, Characterizing biopersistence potential of the metabolite 5:3 fluorotelomer carboxylic acid after repeated oral exposure to the 6:2 fluorotelomer alcohol, Toxicol. Appl. Pharmacol., 2020, 388, 114878 CrossRef CAS PubMed.
- OECD, PFASs and Alternatives in Food Packaging (Paper and Paperboard) Report on the Commercial Availability and Current Uses, 2020 Series on Risk Management No, 58, ENV Search PubMed.
- F. Li, J. Duan, S. Tian, H. Ji, Y. Zhu, Z. Wei and D. Zhao, Short-chain per- and polyfluoroalkyl substances in aquatic systems: Occurrence, impacts and treatment, Chem. Eng. J., 2020, 380, 122506 CrossRef CAS.
- A. K. Rosenmai, C. Taxvig, T. Svingen, X. Trier, B. M. A. van Vugt-Lussenburg, M. Pedersen, L. Lesné, B. Jégou and A. M. Vinggaard, Fluorinated alkyl substances and technical mixtures used in food paper-packaging exhibit endocrine-related activity in vitro, Andrology, 2016, 4, 662–672 CrossRef CAS PubMed.
- L. Li, J. Liu, J. Hu and F. Wania, Degradation of Fluorotelomer-Based Polymers Contributes to the Global Occurrence of Fluorotelomer Alcohol and Perfluoroalkyl Carboxylates: A Combined Dynamic Substance Flow and Environmental Fate Modeling Analysis, Environ. Sci. Technol., 2017, 51, 4461–4470 CrossRef CAS PubMed.
- D. Laner, J. Feketitsch, H. Rechberger and J. Fellner, A Novel Approach to Characterize Data Uncertainty in Material Flow Analysis and its Application to Plastics Flows in Austria, J. Ind. Ecol., 2016, 20, 1050–1063 CrossRef.
- J. Chen, L. Tang, W.-Q. Chen, G. F. Peaslee and D. Jiang, Flows, Stock, and Emissions of Poly-and Perfluoroalkyl Substances in California Carpet in 2000−2030 under Different Scenarios, Environ. Sci. Technol., 2020, 54, 6908–6918 CrossRef CAS PubMed.
- N. Seltenrich, PFAS in Food Packaging: A Hot, Greasy Exposure, Environ. Health Perspect., 2020, 128, 054002 CrossRef PubMed.
-
Arkema Kynar®, Polymer Processing and Recycling Aids for Polyolefins and other Polymers, accessed 7 September 2021, https://www.extremematerials-arkema.com/en/markets-and-applications/chemical-industry-and-general-industry/fluoropolymer-processing-aids/ Search PubMed.
- S. E. Amos, The Application of Fluoropolymer Based Processing Aids in Polypropylene and Thermoplastic Rubber, TAPPI Polym, Laminations, Coat. Conf., Proc., 1998 Search PubMed.
- G. W. Curtzwiler, P. Silva, A. Hall, A. Ivey and K. Vorst, Significance of Perfluoroalkyl Substances (PFAS) in Food Packaging, Integr. Environ. Assess. Manage., 2021, 17, 7–12 CrossRef CAS PubMed.
- R. J. Letcher, S. Chu and S. A. Smyth, Side-chain fluorinated polymer surfactants in biosolids from wastewater treatment plants, J. Hazard. Mater., 2020, 388, 122044 CrossRef CAS PubMed.
- B. J. Henry, J. P. Carlin, J. A. Hammerschmidt, R. C. Buck, L. W. Buxton, H. Fiedler, J. Seed and O. Hernandez, A critical review of the application of polymer of low concern and regulatory criteria to fluoropolymers, Integr. Environ. Assess. Manage., 2018, 14, 316–334 CrossRef CAS PubMed.
-
M. Eiji, T. Motohiro, K. Junsuke and M. Sho, JP Patent, JP2017078227A, 2014 Search PubMed.
- X. Liu, Z. Guo, E. E. Folk and N. F. Roache, Determination of fluorotelomer alcohols in selected consumer products and preliminary investigation of their fate in the indoor environment, Chemosphere, 2015, 129, 81–86 CrossRef CAS PubMed.
-
U.S. EPA, Wastes - Resource Conservation - Common Wastes & Materials - Paper Recycling, accessed 3 September 2020, https://archive.epa.gov/wastes/conserve/materials/paper/web/html/faqs.html Search PubMed.
-
Livia Albeck-Ripka, Your Recycling Gets Recycled, Right? Maybe, or Maybe Not, accessed 20 October 2020, https://www.nytimes.com/2018/05/29/climate/recycling-landfills-plastic-papers.html Search PubMed.
-
Christy Somos, This is why Canadian waste keeps ending up in Asia, accessed 20 October 2020, https://www.ctvnews.ca/world/this-is-why-canadian-waste-keeps-ending-up-in-asia-1.4441472 Search PubMed.
-
Frances Bula, China's tough new recycling standards leaving Canadian municipalities in a bind, accessed 20 October 2020, https://www.theglobeandmail.com/news/national/chinese-ban-on-foreign-recyclables-leaving-some-canadian-cities-in-the-lurch/article37536117/ Search PubMed.
-
Statistics Canada, Human Activity and the Environment - Annual Statistics 2005 - Feature Article Solid Waste in Canada, Ottawa, 2005 Search PubMed.
-
Solid Waste & Recycling, Incineration in Canada, https://www.solidwastemag.com/feature/incineration-in-canada/, accessed 16 September 2020 Search PubMed.
-
U.S. EPA, Advancing Sustainable Materials Management, 2019 Search PubMed.
-
Michigan Department of Environment, Report of Solid Waste Landfilled in Michigan, 2020 Search PubMed.
-
Government of Canada, Plastics Challenge – Food Packaging, accessed 13 August 2020, https://www.ic.gc.ca/eic/site/101.nsf/eng/00036.html Search PubMed.
-
Statistics Canada, Table 38-10-0032-01 Disposal of Waste, 2018 Search PubMed.
-
U.S. EPA, Reducing Wasted Food & Packaging: A Guide for Food Services and Restaurants, 2015 Search PubMed.
-
U.S. EPA, Containers and Packaging: Product-Specific Data, accessed 14 September 2020, https://www.epa.gov/facts-and-figures-about-materials-waste-and-recycling/containers-and-packaging-product-specific-data Search PubMed.
-
Resource Productivity & Recovery Authority (RPRA), Datacall Report 2018, 2018 Search PubMed.
-
F. Alpizar, T. Backhaus, N. Decker, I. Eilks, N. Escobar-Pemberthy, P. Fantke, K. Geiser, M. Ivanova, O. Jolliet and H.-S. Kim, UN Environment Global Chemicals Outlook II-From Legacies to Innovative Solutions: Implementing the 2030, Agenda for Sustainable Development, 2019 Search PubMed.
- Keller and Heckman LLP, New York Bans PFAS in Food Packaging, Natl. Law Rev., 2020, 11, 1 Search PubMed.
-
The Canadian Press, Canadian garbage on its way from Philippines to Vancouver, accessed 8 January 2021, https://www.cbc.ca/news/technology/canadian-garbage-from-philippines-departure-1.5156007 Search PubMed.
- Z. Wang, I. T. Cousins, M. Scheringer, R. C. Buck and K. Hungerbühler, Global emission inventories for C4-C14 perfluoroalkyl carboxylic acid (PFCA) homologues from 1951 to 2030, part II: The remaining pieces of the puzzle, Environ. Int., 2014, 69, 166–176 CrossRef CAS PubMed.
- I. Liagkouridis, R. Awad, S. Schellenberger, M. M. Plassmann, I. T. Cousins and J. P. Benskin, Combined Use of Total Fluorine and Oxidative Fingerprinting for Quantitative Determination of Side-Chain Fluorinated Polymers in Textiles, Environ. Sci. Technol. Lett., 2022, 9, 30–36 CrossRef CAS.
- I. van der Veen, A. C. Hanning, A. Stare, P. E. G. Leonards, J. de Boer and J. M. Weiss, The effect of weathering on per- and polyfluoroalkyl substances (PFASs) from durable water repellent (DWR) clothing, Chemosphere, 2020, 249, 126100 CrossRef CAS PubMed.
- S. Schellenberger, I. Liagkouridis, R. Awad, S. Khan, M. Plassmann, G. Peters, J. P. Benskin and I. T. Cousins, An Outdoor Aging Study to Investigate the Release of Per- and Polyfluoroalkyl Substances (PFAS) from Functional Textiles, Environ. Sci. Technol., 2022, 56, 3471–3479 CrossRef CAS PubMed.
- M. Shoeib, J. Schuster, C. Rauert, K. Su, S. A. Smyth and T. Harner, Emission of poly and perfluoroalkyl substances, UV-filters and siloxanes to air from wastewater treatment plants, Environ. Pollut., 2016, 218, 595–604 CrossRef CAS PubMed.
- I. Weinberg, A. Dreyer and R. Ebinghaus, Waste water treatment plants as sources of polyfluorinated compounds, polybrominated diphenyl ethers and musk fragrances to ambient air, Environ. Pollut., 2011, 159, 125–132 CrossRef CAS PubMed.
- L. Vierke, L. Ahrens, M. Shoeib, E. J. Reiner, R. Guo, W.-U. Palm, R. Ebinghaus and T. Harner, Air concentrations and particle–gas partitioning of polyfluoroalkyl compounds at a wastewater treatment plant, Environ. Chem., 2011, 8, 363 CrossRef CAS.
- L. Ahrens, M. Shoeib, T. Harner, S. C. Lee, R. Guo and E. J. Reiner, Wastewater treatment plant and landfills as sources of polyfluoroalkyl compounds to the atmosphere, Environ. Sci. Technol., 2011, 45, 8098–8105 CrossRef CAS PubMed.
- P. Guerra, M. Kim, L. Kinsman, T. Ng, M. Alaee and S. A. Smyth, Parameters affecting the formation of perfluoroalkyl acids during wastewater treatment, J. Hazard. Mater., 2014, 272, 148–154 CrossRef CAS PubMed.
- C. Zhang, H. Yan, F. Li, X. Hu and Q. Zhou, Sorption of short- and long-chain perfluoroalkyl surfactants on sewage sludges, J. Hazard. Mater., 2013, 260, 689–699 CrossRef CAS PubMed.
- L. Ahrens, S. Felizeter, R. Sturm, Z. Xie and R. Ebinghaus, Polyfluorinated compounds in waste water treatment plant effluents and surface waters along the River Elbe, Germany, Mar. Pollut. Bull., 2009, 58, 1326–1333 CrossRef CAS PubMed.
- L. Ahrens, Polyfluoroalkyl compounds in the aquatic environment: A review of their occurrence and fate, J. Environ. Monit., 2011, 13, 20–31 RSC.
- R. Bossi, J. Strand, O. Sortkjær and M. M. Larsen, Perfluoroalkyl compounds in Danish wastewater treatment plants and aquatic environments, Environ. Int., 2008, 34, 443–450 CrossRef CAS PubMed.
- R. Guo, W. J. Sim, E. S. Lee, J. H. Lee and J. E. Oh, Evaluation of the fate of perfluoroalkyl compounds in wastewater treatment plants, Water Res., 2010, 44, 3476–3486 CrossRef CAS PubMed.
- H. O. Kwon, H. Y. Kim, Y. M. Park, K. S. Seok, J. E. Oh and S. D. Choi, Updated national emission of perfluoroalkyl substances (PFASs) from wastewater treatment plants in South Korea, Environ. Pollut., 2017, 220, 298–306 CrossRef CAS PubMed.
- H. P. Susmann, L. A. Schaider, K. M. Rodgers and R. A. Rudel, Dietary Habits Related to Food Packaging and Population Exposure to PFASs, Environ. Health Perspect., 2019, 127, 107003 CrossRef PubMed.
-
Environmental Defense Fund (EDF), Paper Mills as a Significant Source of PFAS Contamination, but Who's Watching?, accessed 8 December 2020, http://blogs.edf.org/health/2018/05/21/pfas-paper-mills/ Search PubMed.
- H. A. Langberg, H. P. H. Arp, G. D. Breedveld, G. A. Slinde, Å. Høiseter, H. M. Grønning, M. Jartun, T. Rundberget, B. M. Jenssen and S. E. Hale, Paper product production identified as the main source of per- and polyfluoroalkyl substances (PFAS) in a Norwegian lake: source and historic emission tracking, Environ. Pollut., 2020, 116259 Search PubMed.
- H. A. Langberg, H. P. H. Arp, G. D. Breedveld, G. A. Slinde, Å. Høiseter, H. M. Grønning, M. Jartun, T. Rundberget, B. M. Jenssen and S. E. Hale, Paper product production identified as the main source of per- and polyfluoroalkyl substances (PFAS) in a Norwegian lake: Source and historic emission tracking, Environ. Pollut., 2021, 273, 116259 CrossRef CAS PubMed.
- M. Schlummer, C. Sölch, T. Meisel, M. Still, L. Gruber and G. Wolz, Emission of perfluoroalkyl carboxylic acids (PFCA) from heated surfaces made of polytetrafluoroethylene (PTFE) applied in food contact materials and consumer products, Chemosphere, 2015, 129, 46–53 CrossRef CAS PubMed.
- A. N. García, N. Viciano and R. Font, Products obtained in the fuel-rich combustion of PTFE at high temperature, J. Anal. Appl. Pyrolysis, 2007, 80, 85–91 CrossRef.
- Z. Wang, I. T. Cousins, M. Scheringer and K. Hungerbühler, Fluorinated alternatives to long-chain perfluoroalkyl carboxylic acids (PFCAs), perfluoroalkane sulfonic acids (PFSAs) and their potential precursors, Environ. Int., 2013, 60, 242–248 CrossRef CAS PubMed.
- Z. Wang, I. T. Cousins, M. Scheringer, R. C. Buck and K. Hungerbühler, Global emission inventories for C4-C14 perfluoroalkyl carboxylic acid (PFCA) homologues from 1951 to 2030, Part I: Production and emissions from quantifiable sources, Environ. Int., 2014, 70, 62–75 CrossRef CAS PubMed.
- J. Glüge, M. Scheringer, I. T. Cousins, J. C. DeWitt, G. Goldenman, D. Herzke, R. Lohmann, C. A. Ng, X. Trier and Z. Wang, An overview of the uses of per- and polyfluoroalkyl substances (PFAS), Environ. Sci.: Processes Impacts, 2020, 22, 2345–2373 RSC.
-
Organisation for Economic Cooperation and Development (OECD), Working towards a Global Emission Inventory of PFASs: Focus on PFCAs - Status Quo and the Way Forward, Paris, 2015 Search PubMed.
- K. L. Law, N. Starr, T. R. Siegler, J. R. Jambeck, N. J. Mallos and G. H. Leonard, The United States' contribution of plastic waste to land and ocean, Sci. Adv., 2020, 6, 1–8 Search PubMed.
- B. Geueke, K. Groh and J. Muncke, Food packaging in the circular economy: Overview of chemical safety aspects for commonly used materials, J. Cleaner Prod., 2018, 193, 491–505 CrossRef CAS.
- T. Savvaides, J. P. Koelmel, Y. Zhou, E. Z. Lin, P. Stelben, J. J. Aristizabal-Henao, J. A. Bowden and K. J. Godri Pollitt, Prevalence and Implications of Per-and Polyfluoroalkyl Substances (PFAS) in Settled Dust, Curr. Environ. Health Rep., 2022, 1–13 Search PubMed.
-
Danish Veterinary and Food Administration, Ban on fluorinated substances in paper and board food contact materials (FCM), accessed 7 March 2022, https://www.foedevarestyrelsen.dk/english/SiteCollectionDocuments/Kemiogfoedevarekvalitet/UK-Fact-sheet-fluorinated-substances.pdf Search PubMed.
-
SIAL Paris, PFAS rules for 2022 set to improve eco-safety of food packaging, accessed 7 March 2022, https://newsroom.sialparis.com/regional-spotlight/europe/pfas-rules/ Search PubMed.
-
European Chemical Agency (ECHA), Perfluoroalkyl chemicals (PFAS), accessed 7 March 2022, https://echa.europa.eu/hot-topics/perfluoroalkyl-chemicals-pfas Search PubMed.
-
Government of Canada, Information Requirements For Food Packaging Submissions, accessed 30 August 2021, https://www.canada.ca/en/health-canada/services/food-nutrition/legislation-guidelines/guidance-documents/information-requirements-food-packaging-submissions.html Search PubMed.
-
Government of Canada, Substance prohibition summary for PFOA, long-chain PFCAs and related substances, accessed 22 December 2020 Search PubMed.
Footnotes |
† Electronic supplementary information (ESI) available. See https://doi.org/10.1039/d2em00166g |
‡ This research was conducted while the first author was at the University of Toronto, Canada. |
§ Current address:California Department of Toxic Substances Control 1001 I St., Sacramento, CA, 95814, USA |
¶ Key Laboratory of Geographic Information Science (Ministry of Education), School of Geographic Sciences, East China Normal University, Shanghai 200241, China |
|
This journal is © The Royal Society of Chemistry 2022 |