DOI:
10.1039/D1CE01096D
(Paper)
CrystEngComm, 2022,
24, 119-131
Structurally characterised new twisted conformer for cyclen, controlled by metal ion complexation as seen in NiII and CuII complexes with halides and pseudohalides†
Received
15th August 2021
, Accepted 2nd October 2021
First published on 1st November 2021
Abstract
Serendipitous self-assembly is a potential approach for achieving structurally versatile and unexpected solids with chosen metal–ligand combinations. Cyclen (1,4,7,10-tetraazacyclododecane) is an interesting flexible molecule, well explored for biological and material applications of its metal complexes. The dihedral angle (θ) between four nitrogen atoms of free cyclen is close to 0°. Still, it may vary upon complexation, allowing flexibility to adopt any two conformers, viz., (i) planar and (ii) twisted. The present work establishes the crucial role of metal ions in controlling the conformation adopted by cyclen through metal complexation. SCXRD studies of the complexes of cyclen with CuII and NiII with halides and pseudohalides as counterions reveal the existence of a planar conformation (θ = ∼0–3°) for cyclen in CuII systems and a highly twisted conformation (hitherto unreported) in NiII systems (θ = ∼52–55°). Further, SQUID magnetic studies for the dimeric [Ni1] complex reveal antiferromagnetic interactions between the two Ni centres.
1. Introduction
Serendipitous self-assembly of metal ions with diverse types of chosen ligands is a potential approach in supramolecular coordination chemistry for deriving structurally versatile solids.1,2 Such an approach, under the influence of structure directing factors, has the potential to generate interesting structures of material relevance.3 The approach gains more prominence when the ligands are flexible and/or auxiliary anions have diverse binding modes.
Cyclen (1,4,7,10-tetraazacyclododecane) is an interesting flexible molecule, which has been extensively explored for biological and material applications of its metal complexes for a long time.4,5 Being flexible, cyclen can adopt several conformations (Chart 1) ranging from planar to non-planar, depending on the complexation environments. Literature reported Cu and Ni metal complexes of cyclen (Chart 2) have been found to display two conformations for cyclen, viz., a planar conformation for the d9 configuration of Cu(II) ions and a twisted conformer as the major one for the d8 configuration of Ni(II) ions. Early literature studies account for the favourable conformers of cyclen when all the ligand nitrogens are in the basal plane, for different coordination geometries of the metal complexes, without regard to the electronic configuration of the metal ions.5c,d,6,7 Interestingly, we have not come across such studies when ligand nitrogens are not necessarily in the basal plane.
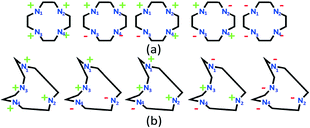 |
| Chart 1 Possible conformations of the coordinated tetraaza macrocycle (cyclen): (a) planar and (b) twisted. The + sign indicates that the hydrogen of the NH group is above the plane of the flattened macrocycle, while the − sign indicates that it is below. | |
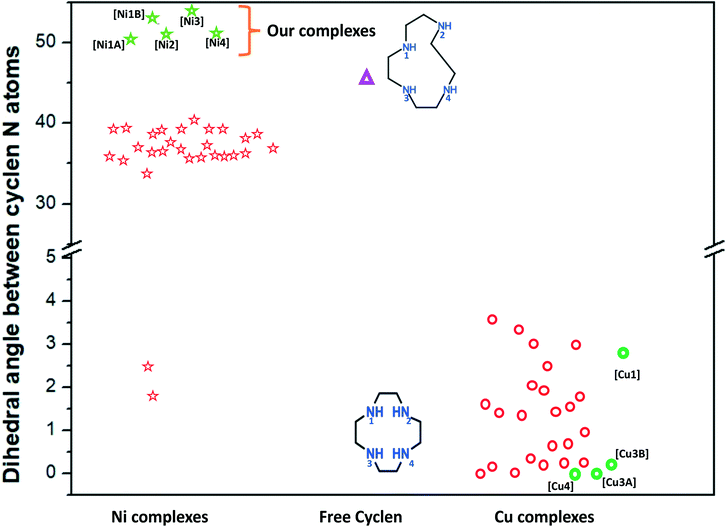 |
| Chart 2 Graphical presentation of the variation of the dihedral angle between the cyclen nitrogens in the reported CuII & NiII complexes, based on a CSD search (version 5.38, 01-03-2019) using the search input “complexes of cyclen with transition metals” without any filters applied. Green circles and stars correspond to the systems reported in this work while red circles and stars are the literature reported systems. | |
Early studies by Busch et al.5c focused on the planar conformation (a of Chart 1) of cyclen and reported the optimum M–N distances for metal ions sitting in the planar macrocyclic cavity. Boeyens and Hancock et al.5d summarized the optimal M–N distances for metal complexes under different coordination geometries, with ligand nitrogens forming the basal plane that minimizes repulsions, resulting in preferred conformations of the tetraaza macrocycles. According to Hancock et al., the ++++ conformer is the favoured conformer for the square planar and square pyramidal geometries, while the ++−− (b of Chart 1) conformer is the favourable conformer for the octahedral geometry for cyclen in which the cyclic nitrogens form the basal plane.
Continuing with our interest in synthesizing new molecules with a focus on their magnetic behaviour, our attempts with NiII, CuII, cyclen and various linkers based on halides and pseudo halides have resulted in complexes ([Ni1]–[Ni4] & [Cu1]–[Cu4]) having distinctly different conformations for cyclen. The observed conformations, i.e. planar in [Cu1]–[Cu4] and twisted in [Ni1]–[Ni4], have been found out to be due to the change in the dihedral angle of the ring nitrogens (N1 to N4) resulting from metal complexation. Interestingly, the dihedral angle of ∼52–55° between the ligand nitrogens of cyclen in the series of NiII complexes leading to the observed twisted conformation is the largest angle reported to date. The paper gives details regarding the synthesis, structure and spectral studies for all the complexes. Further, SQUID magnetic studies for the dimeric [Ni1] complex reveal the antiferromagnetic interactions between the two Ni centres.
2. Experimental section
2.1. Materials and methods
Cyclen, Ni(OAc)2·4H2O, and Cu(OAc)2·2H2O were purchased from TCI chemicals, whereas NaN3, KSCN, NaN(CN)2 & KBr were procured from Sigma Aldrich and used without further purification. A Perkin Elmer Spectrum-2 FTIR spectrometer was employed for FT-IR data collection with KBr pellets in the region 4000 to 400 cm−1. CHN analysis was performed on an Exeter Analytical Inc. CE 440 elemental analyzer. A BRUKER Quest X-ray (fixed-Chi geometry) diffractometer using Mo-I radiation (Kα = 0.71073 Å) was employed for single crystal X-ray data collection for complexes [Ni1]–[Ni4] at 110 K, as well as a PANalytical XRD equipped with a Rigaku two circle diffractometer using Mo-Kα radiation at 300 K for [Cu1] and a Bruker Kappa APEX-II CCD using a D8 X-ray generator (Cryoflex, model Tr-60) using Mo-Kα radiation at 300 K for [Cu3] and [Cu4]. Powder X-ray diffraction (PXRD) data were recorded and collected on a Rigaku Smartlab powder type diffractometer with Cu Kα1 (λ = 1.5406 Å; power 1600 W, 40 kV, 40 mA) at a scan speed of five degrees per minute. The magnetic measurement for [Ni1] was carried out with an MPMS-5 QUANTUM DESIGN SQUID magnetometer between 300 and 2 K with an applied field of 1 kOe. Data have been corrected for the sample holder and for the diamagnetic contribution of all atoms by using Pascal's tables.8a The isothermal field dependence of the magnetization was recorded up to 50 kOe. Modelling was performed using PHI.8b
2.2. Preparation of complexes [Ni1]–[Ni4] & [Cu1]–[Cu4]
To a methanolic solution (∼15 ml) of cyclen (0.10 g, 0.58 mmol), Ni(CH3COO)2·4H2O (0.15 g, 0.58 mmol) was added for the preparation of complexes [Ni1]–[Ni4] and Cu(CH3COO)2·2H2O (0.16 g, 0.58 mmol) was added for complexes [Cu1]–[Cu4]. After stirring for 10 minutes, NaN3 (0.06 g, 1.16 mmol), KSCN (0.08 g, 1.16 mmol), NaN(CN)2 (0.08 g, 1.16 mmol) or KBr (0.12 g, 1.16 mmol) was respectively added for the preparation of the corresponding complexes. The reaction mixture was stirred overnight at room temperature (25 °C). The clear mother liquor was filtered into a small test tube, which is placed inside a bigger stoppered test tube partially filled with ether. Slow diffusion of ether into the inner tube maintained at 18 °C afforded single crystals suitable for X-ray structural studies.
Suitable single crystals were obtained within 2 days in the case of the NiII complexes and one week for the CuII complexes. Yield: [Ni1] = 0.23 g (76.6%, 0.47 mmol), M.P. 214(±1) °C. Anal. calcd. for C16H40N14Ni2 (MW = 546.04): C, 35.20; H, 7.38; N, 35.92. Found: C, 34.92; H, 7.41; N, 35.42. [Ni2] = 0.28 g (87.5%, 0.77 mmol), M.P. 209(±1) °C. Anal. calcd. for C12H20N10Ni (MW = 363.09): C, 39.70; H, 5.55; N, 38.58. Found: C, 39.43; H, 5.34; N, 38.83. [Ni3] = 0.26 g (81.2%, 0.74 mmol), M.P. 212(±1) °C. Anal. calcd. for C10H20N6S2Ni (MW = 347.15): C, 34.60; H, 5.81; N, 24.21. Found: C, 35.02; H, 5.25; N, 23.94. [Ni4] = 0.32 g (91.6%, 0.84 mmol), M.P. 182(±1) °C. Anal. calcd. for C10H23N4O2BrNi (MW = 369.94): C, 32.47; H, 6.27; N, 15.15. Found: C, 32.24; H, 5.92; N, 15.24. [Cu1] = 0.30 g (93.7%, 0.79 mmol), M.P. 235(±1) °C. Anal. calcd. for C8H20N10Cu (MW = 319.88): C, 41.82; H, 7.02; N, 31.04. Found: C, 41.43; H, 7.23; N, 31.35. [Cu2] = 0.31 g (91.2%, 0.86 mmol), M.P. 215(±1) °C. [Cu3] = 0.28 g (82.3%, 0.79 mmol), M.P. 230(±1) °C. Anal. calcd. for C10H20N6S2Cu (MW = 351.98): C, 34.12; H, 5.73; N, 23.88. Found: C, 33.93; H, 5.34; N, 23.90 and [Cu4] = 0.32 g (84.2%, 0.81 mmol) M.P. 201(±1) °C. Anal. calcd. for C8H20N4Br2Cu (MW = 395.64): C, 24.29; H, 5.10; N, 14.16. Found: C, 24.01; H, 4.94; N, 14.31. The procedure for the preparation of these complexes is shown schematically in Scheme 1. All the complexes were completely characterized by FT-IR, CHN, and PXRD, in addition to SCXRD. Due to the poor quality of crystals, the SCXRD data for [Cu2] could not be collected.
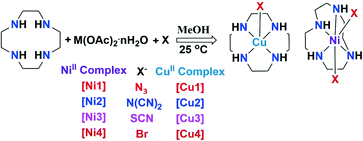 |
| Scheme 1 Schematic representation for the preparation of complexes. M = NiII for [Ni1]–[Ni4] and M = CuII for [Cu1]–[Cu4], where X = N3−, N(CN)2−, SCN− and Br−, respectively. | |
2.3. Crystallographic data collection and refinement
Data collection was done for the title complexes as detailed in section 2.1. Hydrogen atoms were set to be riding on the parent atoms after placing them in idealized positions around the respective parent atoms. The atoms were refined with anisotropic thermal parameters except for the hydrogen atoms. The absence of additional symmetry and voids was confirmed using PLATON (ADDSYM).9 The structures were solved by direct methods and refined by full-matrix least-squares on F2 using the latest version of SHELX-2018.10 The refinement converged to final values of R1 & wR2 = 0.0587 & 0.1399 for [Ni1], 0.0485 & 0.1524 for [Ni2], 0.0486 & 0.1178 for [Ni3], 0.0434 & 0.0814 for [Ni4], 0.0220 & 0.0525 for [Cu1], 0.0616 & 0.0683 for [Cu3] and 0.0288 & 0.0327 for [Cu4], respectively. Important crystal data are presented in Table 1, and the bond lengths and angles are presented in ESI† Table S1. The drawings are made using OLEX-II11 and MERCURY.12
Table 1 Important crystal data for the title complexes
Complex |
[Ni1]
|
[Ni2]
|
[Ni3]
|
[Ni4]
|
[Cu1]
|
[Cu3]
|
[Cu4]
|
R
1 = Σ‖Fo| − |Fc‖/Σ|Fo|.
wR
2 = [Σ[w(Fo2 − Fc2)2]/Σ[w(Fo2)2]1/2.
|
Empirical formula |
C16H40N14Ni2 |
C12H20N10Ni |
C10H20N6S2Ni |
C10H23BrN4O2Ni |
C8H20N10Cu |
C10H20N6S2Cu |
C8H20Br2N4Cu |
CCDC No. |
1889902
|
1889904
|
1889951
|
1889905
|
1890811
|
1890876
|
1890877
|
F.W. |
546.04 |
363.09 |
347.15 |
369.94 |
319.88 |
351.98 |
395.64 |
Space group |
C2/m |
Pbca
|
R
3
c
|
Pnma
|
P21/n |
P212121 |
P4/nmm |
Crystal system |
Monoclinic |
Orthorhombic |
Trigonal |
Orthorhombic |
Monoclinic |
Orthorhombic |
Tetragonal |
a (Å) |
13.2618(9) |
12.8420(6) |
22.9171(16) |
15.1276(11) |
8.3568(4) |
12.8292(9) |
9.5813(6) |
b (Å) |
8.7178(6) |
14.8998(6) |
22.9171(16) |
8.9116(7) |
14.4655(7) |
13.3387(10) |
9.5813(6) |
c (Å) |
13.2822(9) |
16.5728(7) |
15.2853(11) |
11.1889(8) |
11.2207(5) |
18.2848(14) |
7.5171(5) |
α, β, γ (°) |
90, 109.205(2), 90 |
90, 90, 90 |
90, 90, 120 |
90, 90, 90 |
90, 92.84(12), 90 |
90, 90, 90 |
90, 90, 90 |
V (Å3) |
1450.14(17) |
3171.1(2) |
6952.2(11) |
1508.39(19) |
1354.74(11) |
3129.0(4) |
690.08(10) |
T (K) |
110.0 |
110.0 |
110.0 |
110.0 |
273 |
110 |
300 |
Z
|
2 |
8 |
18 |
4 |
4 |
8 |
2 |
D
calc (mg m−3) |
1.251 |
1.521 |
1.492 |
1.629 |
1.568 |
1.494 |
1.904 |
μ (mm−1) |
1.327 |
1.240 |
1.522 |
3.936 |
1.618 |
1.659 |
7.351 |
GOF on F2 |
1.115 |
1.079 |
1.068 |
1.182 |
1.076 |
1.007 |
1.064 |
R (Fo2)a |
0.0587 |
0.0485 |
0.0486 |
0.0434 |
0.0220 |
0.0616 |
0.0288 |
wR (Fo2)b |
0.1399 |
0.1524 |
0.1178 |
0.0814 |
0.0525 |
0.0683 |
0.0327 |
3. Results and discussion
3.1. Synthesis and vibrational spectroscopic studies
A series of NiII and CuII complexes have been synthesized in situ in a step-wise manner with reasonable yields. The complexes are blue blocks with sharp melting points. The PXRD patterns (ESI,† Fig. S1) confirm the bulk purity of the products, as can be seen from the agreement of the experimental PXRD patterns with the simulated patterns, indicating that the crystal structures are true representatives of the bulk products.
All the complexes exhibit strong and sharp vibrational bands at 3000–3300 cm−1 in the FT-IR spectra (ESI,† Fig. S2), which correspond to the N–H symmetric and asymmetric stretching of the core moiety (cyclen) containing four N–H bonds.13 There are other sharp bands appearing at 1455 cm−1 (m), 968 cm−1 (s), and 883 cm−1 (s) and one broad band at about 1630 cm−1 (s) corresponding to the characteristic (C–H) bands for the core moiety. Due to the presence of an N3− group, sharp bands at 2127 cm−1 (s) and 2182 cm−1 (s) corresponding to the asymmetric μ1,3 and end-on stretching modes of azide are observed for complexes [Ni1] & [Cu1] respectively.14 Symmetric and asymmetric end-on stretching vibrations of N(CN)2− have been observed at 2140 cm−1 (m) & 2225 cm−1 (m) for [Ni2] and at 2127 cm−1 (m) and 2182 cm−1 (m) for [Cu2]. [Ni3] shows a sharp band at 2035 (s) corresponding to the symmetric end-on stretching of the NCS− group. The sharp bands at 2144 cm−1 (s) correspond to the end-on symmetric stretching of coordinated SCN−, and the bands at 2251 cm−1 (m) and 2287 cm−1 (m) correspond to the asymmetric stretching vibrations of uncoordinated SCN− of [Cu3]. The fingerprint region in all the complexes shows sharp bands at 526 cm−1 and 421 cm−1 corresponding to the Ni–N and Cu–N stretching frequencies, respectively. The rest of the spectral patterns and band positions of all the complexes are very similar and are shown in the ESI† (Fig. S2).
3.2. Single crystal X-ray diffraction structural studies
3.2.1. [Ni(cyclen)N3]2 [Ni1] di[μ-1,3-azido-bis(1,4,7,10-tetraazacyclododecan-1-yl)nickel(II)].
Complex [Ni1] crystallizes in the monoclinic space group C2/m. NiII adopts a distorted octahedral geometry, with three cyclen nitrogens (N2, N3, and N2I) forming the basal plane and azide nitrogen (N6I) occupying the fourth position. The axial positions of the octahedron are occupied by one cyclen nitrogen (N1) and nitrogen of the second azide (N4) (Fig. 1a). The M–N distances are in the range 2.03–2.12 Å, respectively (Table S1†). NiII is displaced by (d) 0.189 Å from the mean plane of the coordinating nitrogen atoms. The azide group acts as a symmetric μ-1,3 bridge and connects two NiII centres forming a dimer, where one N3− group orients equatorially while the other tends to be axial. The Ni–Naz distances in the [Ni1] dimer are slightly shorter than those in the double μ-1,3 azido bridged NiII dimers in which azide is in the axial–equatorial sites of the coordination polyhedron.15 The dihedral angles between the ligand nitrogens in the two-cyclen moieties are 51.8° and 53.3° (Table S2†). The intradimer separation between the two NiII centres (5.313 Å) is much shorter than the separation observed (ranging between 7–11 Å) for reported dimeric NiII complexes of cyclen (ESI† Table S3). Neighbouring dimers are non-interacting and are arranged parallel in 3-dimensions, as shown in Fig. 1b.
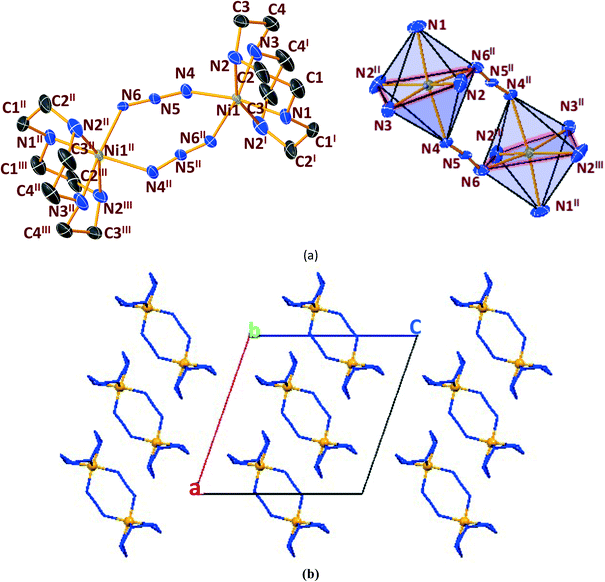 |
| Fig. 1 (a) (Left) ORTEP of [Ni1] showing atoms as 40% probability ellipsoids. Ring hydrogens have been omitted for clarity. (Right) Corresponding polyhedral drawing showing the coordination environment around the metal center. (b) Packing of dimers in the unit cell. Symmetry codes: I = x, −y, z; II = 1 − x, y, 1 − z; III = 1 − x, −y, 1 − z. | |
3.2.2. [Ni(cyclen)(N(CN)2)2] [Ni2] [bis(dicyanamido)(1,4,7,10-tetraazacyclododecan-1-yl)nickel(II)].
Complex [Ni2] crystallizes in the orthorhombic space group Pbca. Monomeric [Ni2] is in a distorted octahedral geometry with three cyclen nitrogens (N1, N2 & N3) forming the basal plane and one anionic dicyanamide nitrogen (N5) occupying the fourth position. The axial positions of the octahedron are occupied by one cyclen nitrogen (N4) and nitrogen (N8) of the second dicyanamide group (Fig. 2a). The coordination polyhedron around NiII is comparable to a previously reported dicyanamide bridged NiII dimer with cyclen.16 The M–N distances are 2.06–2.13 Å and 2.107 Å, respectively (Table S1†). NiII deviates by d = 0.167 Å from the mean plane of the coordinating nitrogen atoms. The dihedral angle between the four nitrogens of the ligand is 52.1° (Table S2†). Individual monomers act as hydrogen bond donors (through Ncy–H) and acceptors (through dicyanamide nitrogens). They are interconnected via weak hydrogen bonding interactions with neighbouring monomers (N1⋯N10: 3.0.023(5) Å; N4⋯N10 Å: 3.167(5) Å; N3⋯N7: 3.046(5) Å; C8⋯N7: 3.590(5) Å) (Fig. 2b), leading to a 2-D layer arrangement in the unit cell.
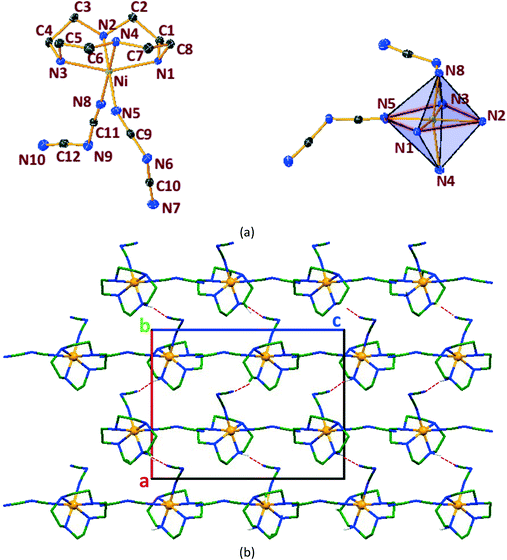 |
| Fig. 2 (a) (Left) Ortep of [Ni2] showing atoms as 40% probability ellipsoids. Ring hydrogens have been omitted for clarity. (Right) Coordination polyhedron around the metal center. (b) Packing of hydrogen bonded monomers in the unit cell. | |
3.2.3. [Ni(cyclen)(SCN)2] [Ni3] [(1,4,7,10-tetraazacyclododecan-1-yl)bis(thiocyanato)nickel(II)]:.
Complex [Ni3] crystallizes in the trigonal crystal system with the space group R3c. The asymmetric unit contains one metal centre, one ligand and two thiocyanate anions. Three nitrogen atoms from the ligand (N1, N2 & N3) form the octahedral geometry of NiII, along with the fourth nitrogen (N5) from one thiocyanate in the basal plane. The fourth ligand nitrogen (N4) and the nitrogen (N6) of the second thiocyanate moiety occupying the axial positions (Fig. 3a). The M–N distances are in the range 2.07–2.16 Å (Table S1†). The dihedral angle between the four nitrogen atoms of the ligand is 54.6° (Table S2†). The metal ion deviates by 0.121 Å from the mean plane of the coordinating nitrogens. The terminal sulphur atoms of the coordinated thiocyanate moieties are involved in bifurcated hydrogen bonding interactions with the amino hydrogens of the neighbouring cyclen units (N2⋯S2: 3.481(4) Å; N4⋯S2: 3.553(4) Å; N1⋯S2: 3.620(4) Å; N3⋯S1: 3.578(6) Å), resulting in a trigonal packing of the monomeric units when viewed down the b axis of the unit cell (Fig. 3b).
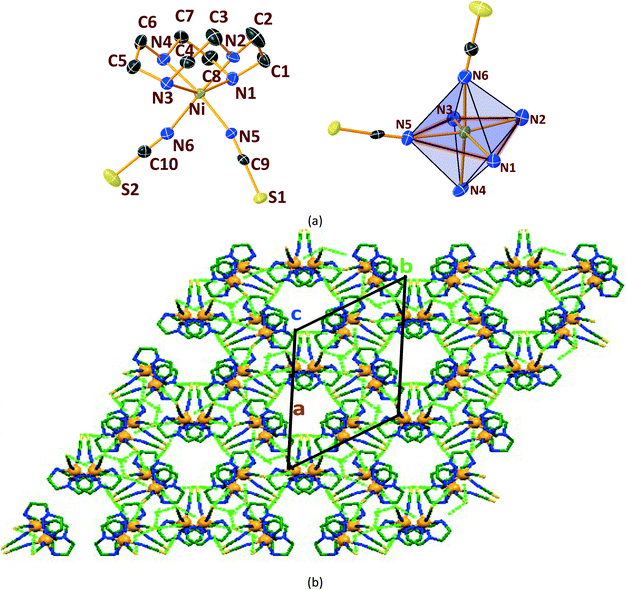 |
| Fig. 3 (a) (Left) Ortep of [Ni3] showing atoms as 40% probability ellipsoids. Ring hydrogens are omitted for clarity. (Right) Coordination polyhedron around the metal centre. (b) Packing of monomers in the unit cell. | |
3.2.4. [Ni(cyclen)(CH3COO)]Br [Ni4] [(acetato)-(1,4,7,10-tetraazacyclododecane) nickel(II)]bromide.
[Ni4] crystallizes in the orthorhombic space group Pnma. The asymmetric unit contains one metal ion, half a ligand, one acetate group and one bromide ion in the asymmetric unit lying on a symmetry plane. The other half of the ligand is symmetrically generated. Though the metal ion adopts an octahedral geometry, the equatorial sites are occupied by two nitrogen atoms from the ligand (N1 & N3) and two oxygen (O1 & O2) atoms from the acetate group, forming the mean plane. In addition, the axial positions are occupied by the other two nitrogen atoms of the ligand (N2 & N2I) (Fig. 4a). The M–N distances are in the range 2.03–2.06 Å (Table S1†). NiII is found to be displaced by 0.142 Å from the basal plane of the coordinating nitrogens. The dihedral angle between the four nitrogen atoms of the ligand is found to be 52.6° (Table S2†). The uncoordinated bromide ions connect the monomers into a zig-zag chain through hydrogen bonding interactions (N1⋯Br1: 3.346(4) Å), (Fig. 4b).
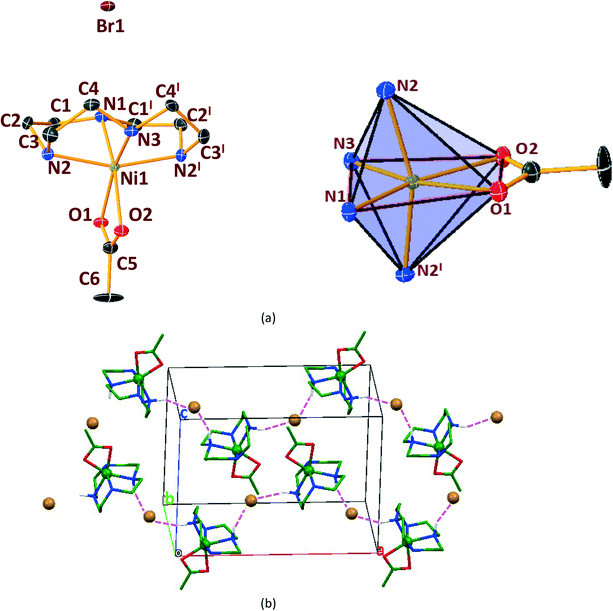 |
| Fig. 4 (a) (Left) Ortep of [Ni4] showing atoms as 40% probability ellipsoids. Ring hydrogens have been omitted for clarity. (Right) Coordination polyhedron around the metal center. (b) Hydrogen bonded zig-zag chain of monomers in the unit cell. Symmetry code: I = x, −y, z. | |
In general, cyclen adopts a twisted conformation in all the structures (Table S2†), similar to that observed in other Ni-cyclen complexes with diverse anions (Chart 2). The trends in the Ni–Ncy bond lengths are similar for all complexes and are in accordance with other reported Ni-cyclen complexes (ESI† Table S3). However, the dihedral angle between the cyclen nitrogens (∼52–55°) observed here is much higher than the angle (35–40°) in the NiII complexes of cyclen reported so far (Chart 2), thus revealing a new conformational angle and geometry for the twisted conformation of cyclen in the NiII complexes.
3.2.5. [Cu(cyclen)N3]N3 [Cu1] [azido(1,4,7,10-tetraazacyclododecan-1-yl)copper(II)]azide.
Monomeric [Cu1] crystallizes in the monoclinic space group P21/n with a distorted square pyramidal geometry around the metal centre. The asymmetric unit contains one metal centre, one ligand and two azide moieties. The ligand nitrogens (N1, N2, N3 & N4) form the basal plane, and azide nitrogen (N5) of one moiety occupies the pyramidal position (Fig. 5a) of the metal ion. The Cu–Ncy and Cu–Naz bond lengths are 2.01–2.04 Å and 2.10 Å, respectively (Table S1†). CuII is displaced by 0.5330(6) Å from the basal plane. The dihedral angle between the four nitrogen atoms of the ligand is found to be 2.7° (Table S2†). Both coordinated and uncoordinated azides are involved in hydrogen bonding interactions with the amino hydrogens of the ligand. The coordinated azides connect the symmetry-related monomers into a dimer through amino Ncy–H⋯Naz (H⋯A 1.994 Å) hydrogen bonding interactions (red dashed lines, Fig. 5b). The uncoordinated azides not only connect the monomers of the same symmetry into a hydrogen-bonded chain involving amino N–H⋯Naz (N1⋯N10: 2.9734(18); N3⋯N8: 3.0523(18) Å) hydrogen bonds but also connect the symmetry-related dimers to neighbouring dimers (N4⋯N10: 2.9718(17) Å), leading to a 2-D layer (black dashed lines, Fig. 5b).
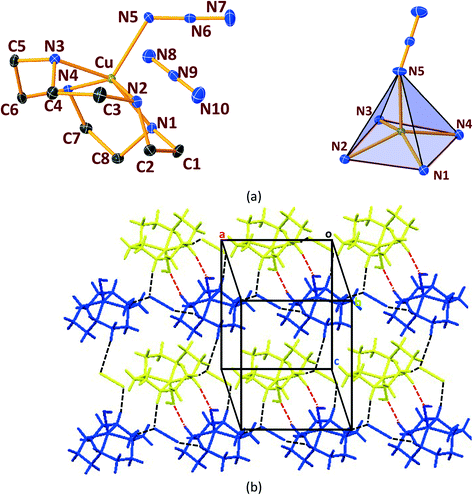 |
| Fig. 5 (a) (Left) Ortep of [Cu1] showing atoms as 40% probability ellipsoids. Ring hydrogens have been omitted for clarity. (Right) Coordination polyhedron around the metal center. (b) Packing of H-bonded dimers into a 2-D layer in the unit cell. | |
3.2.6. [Cu2(cyclen)2(SCN)2](SCN)2 [Cu3] di[(1,4,7,10-tetraazacyclododecan-1-yl)bis(thiocyanato)copper]dithiocyanate.
Complex [Cu3] crystallizes in the orthorhombic space group P212121. The asymmetric unit contains two independent [Cu(cyclen)(NCS)]+ moieties and two uncoordinated NCS− units. While the metal ion exhibits a square pyramidal geometry, the two units are distinct by the coordination mode of the thiocyanate moiety in the pyramidal position. In the first unit, the distorted square pyramidal geometry is constructed from four nitrogen atoms of the ligand (N1A, N2A, N3A & N4A) forming the basal plane and the S (S1A) atom of the thiocyanate moiety (Fig. 6a) occupying the fifth axial site. However, in the second unit, the distorted square pyramidal geometry of the metal center is constructed from the ligand nitrogens (N1B, N2B, N3B & N4B) forming the basal plane and the nitrogen of second thiocyanate moiety (N5B) occupying the pyramidal position. The displacement of CuII from the basal plane is 0.556 Å & 0.589 Å for both units, respectively. The dihedral angle between four nitrogen atoms of the ligand is 0.2° and 0.0° for the two independent units, respectively (Table S2†). The two cyclen units are arranged in a flip-flop manner as pairs connected through hydrogen bond interactions (Ncy–H⋯NSCN = 2.960 Å) between the ring amino hydrogens of the first unit and the N end of the coordinated thiocyanate of the second unit (red dashed lines in Fig. 6c). The uncoordinated thiocyanate moieties connect the neighbouring flipped pairs into a 2-D layer arrangement in the unit cell through hydrogen-bonding interactions with ring amino hydrogens (N4A⋯S1C: 3.318(5) Å; N1B⋯N1C: 2.957(8) Å; N3A⋯S1D: 3.439(6); N3B⋯S1D: 3.338(5) Å, black lines in Fig. 6b).
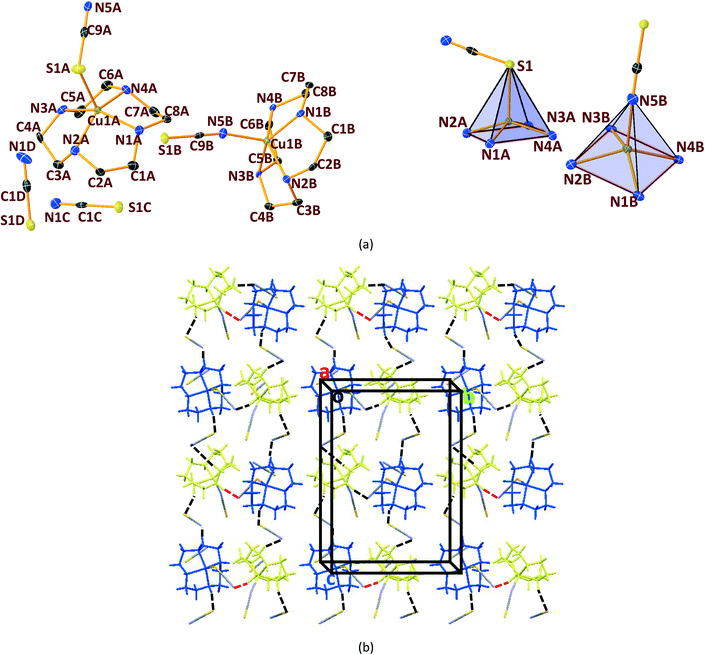 |
| Fig. 6 (a) (Left) Ortep of [Cu3] showing atoms as 40% probability ellipsoids. Ring hydrogens have been omitted for clarity. (Right) Coordination polyhedron around the metal center. (b) 2-D layer arrangement of the flipped pairs. | |
3.2.7. [Cu(cyclen)Br]Br [Cu4] bromido[(1,4,7,10-tetraazacyclododecan-1-yl)copper(II)]bromide.
Complex [Cu4] crystallizes in the tetragonal space group P4/nmm with a square pyramidal geometry around the metal center. Nitrogens of the ligand occupy the four equatorial positions (N1, N2, N3 & N4) forming the basal plane, whereas the bromide ion (Br1) occupies the pyramidal position (Fig. 7a). The CuII ion is displaced by 0.593(2) Å from the basal plane. The dihedral angle for the four ligand nitrogen atoms is found to be 0.0° (Table S2†). In the asymmetric unit, there is another bromide ion (Br2) that is uncoordinated and connects the neighbouring [Cu(cyclen)Br]+ units into a 2-D network through hydrogen bonding interactions (N1⋯Br2 = 3.319 Å) (Fig. 7b).
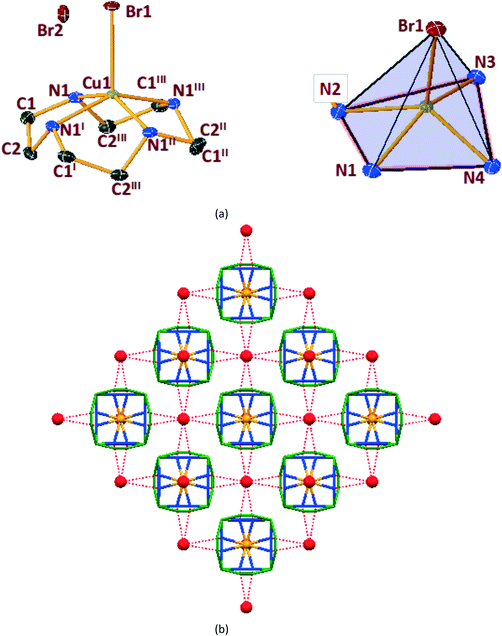 |
| Fig. 7 (Left) Ortep of [Cu4] showing atoms as 40% probability ellipsoids. Ring hydrogens have been omitted for clarity. (Right) Coordination polyhedron around the metal center. (b) Packing of monomers through hydrogen bonding interactions. Symmetry code: I = x, 3/2 − y, z; II = 3/2 − x, 3/2 − y, z; III = 3/2 − x, y, z. | |
3.3. Structural comparisons between Ni & Cu series.
The diverse shapes exhibited by all the metal complexes may be attributed to the conformational flexibility of the ligand to support the metal ions' geometrical preferences arising out of the CFSE, supported by the shape of the anion. Cyclen being flexible, when put in a solution containing metal ions and anions, adjusts its conformation to suit the metal ions' geometrical preferences, thereby adopting a twisted conformation with a NiII ion and a planar conformation with a CuII ion, maximising the CFSE of the respective metal ions.
By doing so, it supports the metal complex to attain diverse shapes in association with the anion, which may be depicted in a cartoon representation as an azide bridged dumbbell for [Ni1], running crown for [Ni2] with bent dicyanamide, standing crown for [Ni3] with a linear thiocyanate, and a wine glass shape for [Ni4] with coordinated acetate (Scheme 2). With a larger twist in the ligand conformation (dihedral angle of ligand nitrogens = ∼52–55°), this anionic effect is more pronounced in the NiII series. However, with a planar ligand around the Cu(II) ion (a dihedral angle of ligand nitrogens of ∼0–3°), the gross shape of the molecule remains the same (an inverted umbrella – cartoon representation) similar to other reported complexes (ESI,† Table S4), with a minimal effect of the anion that shows up in the packing patterns only.
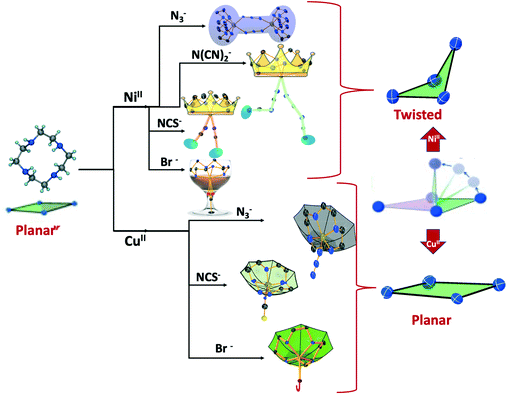 |
| Scheme 2 Schematic presentation of the diverse shapes arising out of the metal ion coordination supported by anions. | |
The planar conformation (++++) of cyclen in all the copper complexes is in accordance with the predictions of Hancock et al. for a square pyramidal geometry.6 However, in NiII complexes, cyclen displays several conformations such as ++++ for [Ni1] and [Ni3], +++− for [Ni2], and −+++ for [Ni4], which are very much different from the predictions (++−−) of Hancock et al. for an octahedral geometry of a metal ion, where all the ligand amino nitrogens are in the basal plane. The variations in ligand conformation in our case appear to arise from the difference in the planar atoms for the octahedral geometry, where only three amino nitrogens of the ligand are in the basal plane and the fourth position is occupied by a different anionic atom.
3.4. Magnetic studies of [Ni1]
The magnetic behaviour for the azide-bridged Ni dimer [Ni1] has been investigated in the temperature domain 2–300 K. The temperature dependence of χMT (χM stands for the molar magnetic susceptibility) for [Ni1] is characteristic of a strong antiferromagnetic interaction between the two Ni centres (Fig. 8a). The value for χMT at 300 K, 1.45 cm3 mol−1 K, is below the Curie contribution of 2 cm3 mol−1 K expected for the two Ni centres (S = 1, g = 2.0) and steadily decreases as T is decreased to reach almost zero below 20 K. The related χM = f(T) shows a broad maximum at 110 K (Fig. 8(b)).
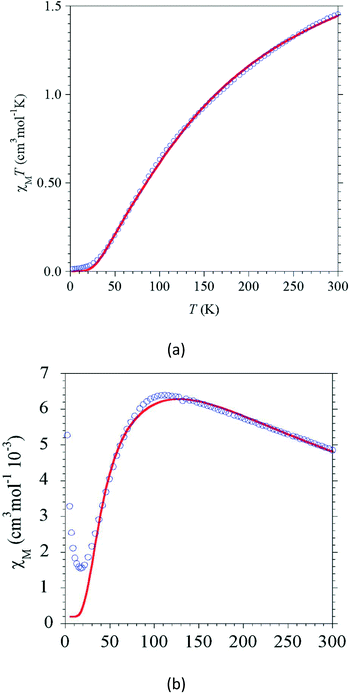 |
| Fig. 8 (a) Experimental ( ) and calculated ( ) temperature dependence of χMT and (b) χM for [Ni1]. | |
The modelling performed with a dimer model for two S = 1 centres (the reference Hamiltonian is Ĥ = −J(ŜNi1·ŜNi2)) leads to an interaction parameter of J = −82.1 ± 0.2 cm−1 for g = 2.0. The obtained exchange parameter is in accordance with the values reported for related compounds15–17 and confirms the strong Ni–Ni antiferromagnetic interaction mediated by the azido-bridges.
Conclusions
The self-assembly of NiII or CuII with conformationally flexible cyclen in the presence of pseudohalides has resulted in the title complexes which are characterised structurally. All the NiII complexes display a twisted conformer for the ligand with a large dihedral angle (52–55°, Table S2†) between the cyclen nitrogens, not reported till now, while the CuII complexes display a planar conformation, as predicted by Hancock et al. The variation in the cyclen conformers as seen in the NiII complexes (++++ for [Ni1] and [Ni3], +++− for [Ni2] and −+++ for [Ni4]) and CuII (++++) complexes has been explained by the changes in the dihedral angle of the ligand nitrogens. The change in ligand conformation from the twisted one for the NiII complexes to the planar one for the CuII complexes has been understood in terms of the metal ions' geometrical preferences imposed by the CFSE. The Jahn–Teller effect in CuII allows the sustenance of planar conformation of cyclen by adopting a square pyramidal geometry where all four N atoms of cyclen complex with CuII in the square plane and different anions (N3−, SCN− & Br−) occupy the pyramidal position resulting in a gross inverted umbrella structure for all the complexes ([Cu1]–[Cu4]) with no anion effect on the molecular shape. In the absence of J–T distortion, in the octahedral NiII complexes, the ligand is highly distorted (a dihedral angle of ∼52–55°) to support the octahedral geometry of the metal ion, thus attaining a twisted conformation, in which the ligand nitrogens occupy three positions of the square plane, and anionic nitrogen occupies the remaining one position, as seen through the X-ray structures. Due to the positioning of anionic nitrogens in the square plane, depending on the anion, different structures were obtained for Ni-cyclen-X complexes, viz., “dumbbell-shaped structure” with azide [Ni1], “running crown structure” with dicyanamide [Ni2], “standing crown structure” with thiocyanate [Ni3] and “wine glass structure” with bromide ions [Ni4]. The [Ni1] dumbbell shows antiferromagnetic interactions (J = −82.1 ± 0.2 cm−1) between the end-to-end azide bridged Ni centers.
The present study involving the serendipitous self-assembly approach is successful in providing a new conformation for cyclen with a large dihedral angle (∼52–55°), the largest reported so far between its ring nitrogens. The increased dihedral angle of the ligand in its non-planar mode of coordination to support the NiII octahedral geometry, in association with diversely shaped anions, resulted in interesting structures under self-assembly conditions. The examples are important as there are no literature reports predicting the conformations of cyclen for an octahedral geometry in which not all its ring nitrogens form the basal plane. This work provides a scope for in-depth theoretical studies in finding out all the possible conformations for cyclen in an octahedral coordination when all ligand nitrogens are not necessarily in the basal plane of the metal ion. More examples may be helpful in developing functional molecules and studying their functionality based on variations in the structure.
Author contributions
Abhineet Verma – conceptualization, data curation, and formal analysis; Nattamai Bhuvanesh, Joseph Reibenspies, Sakharam B. Tayade, and Avinash S. Kumbhar – X-ray data acquisition; Kateryna Bretosh & Jean-Pascal Sutter – magnetic data acquisition and analysis; Sailaja S. Sunkari – funding acquisition, supervision, writing the original draft, review and editing.
Conflicts of interest
There are no conflicts to declare.
Acknowledgements
This work is supported by funding from DST, New Delhi, India (Project code: DST/YSS/2015/000993). The infrastructural facilities at Department of Chemistry, Mahila Maha Vidyalaya, Banaras Hindu University, and instrumental facilities at Department of Chemistry, Institute of Science, Banaras Hindu University, are gratefully acknowledged.
Notes and references
-
(a) A. J. Metherell and M. D. Ward, Imposing Control On Self-Assembly: Rational Design And Synthesis Of A Mixed-Metal, Mixed-Ligand Coordination Cage Containing Four Types Of Component, Chem. Sci., 2016, 7(2), 910–915 RSC;
(b) Z. He, M. Li, W. Que and P. J. Stang, Self-Assembly Of Metal-Ion-Responsive Supramolecular Coordination Complexes And Their Photophysical Properties, Dalton Trans., 2017, 46(10), 3120–3124 RSC;
(c) L. Wang, B. Song, S. Khalife, Y. Li, L.-J. Ming, S. Bai, Y. Xu, H. Yu, M. Wang, H. Wang and X. Li, Introducing Seven Transition Metal Ions Into Terpyridine-Based Supramolecules: Self-Assembly And Dynamic Ligand Exchange Study, J. Am. Chem. Soc., 2020, 142(4), 1811–1821 CrossRef CAS.
- P. H. Dinolfo and J. T. Hupp, Supramolecular Coordination Chemistry And Functional Microporous Molecular Materials, Chem. Mater., 2001, 13(10), 3113–3125 CrossRef CAS.
- S. Evariste, C. Xu, G. Calvez and C. Lescop, Straightforward Coordination-Driven Supramolecular Chemistry Preparation Of A Discrete Solid-State Luminescent Cu4 Polymetallic Compact Assembly Based On Conformationally Flexible Building Blocks, Inorg. Chim. Acta, 2021, 516, 120115–120123 CrossRef CAS.
-
(a) J. P. Collman and P. W. Schneider, Complexes of Cobalt III and Rhodium III with A Cyclic Tetradentate Secondary Amine, Inorg. Chem., 1966, 5, 1380–1384 CrossRef CAS;
(b) A. J. Clarkson, D. A. Buckingham, A. J. Rogers, A. G. Blackman and C. R. Clark, Kinetic Origin of The Chelate Effect. Base Hydrolysis, H-Exchange Reactivity And Structures of syn, Anti-[Co(Cyclen)(NH3)2]3+and syn, Anti-[Co(Cyclen)(Diamine)]3+ Ions (Diamine = H2N(CH2)2NH2, H2N(CH2)3NH2), Inorg. Chem., 2000, 39, 4769–4775 CrossRef CAS.
-
(a) J. Rawlings, W. W. Cleland and A. C. Hengge, Metal-Catalyzed Phosphodiester Cleavage: Secondary 18O Isotope Effects as an Indicator of Mechanism, J. Am. Chem. Soc., 2006, 128, 17120–17125 CrossRef CAS;
(b) J. Y.-C. Chang, R. J. Stevenson, G.-L. Lu, P. J. Brothers, G. R. Clark, W. A. Denny and D. C. Ware, Syntheses of 8-Quinolinolato cobalt III Complexes Containing Cyclen Based Auxiliary Ligands as Models for Hypoxia-Activated Prodrugs, Dalton Trans., 2010, 39, 11535–11550 RSC;
(c) L. Y. Martin, L. J. De Hayes, L. J. Zompa and D. H. Busch, J. Am. Chem. Soc., 1974, 96, 4046–4048 CrossRef CAS;
(d) V. J. Thom, C. C. Fox, J. C. A. Boeyens and R. D. Hancock, Molecular Mechanics And Crystallographic Study Of Hole Sizes In Nitrogen-Donor Tetraaza Macrocycles, J. Am. Chem. Soc., 1984, 106(20), 5947–5955 CrossRef CAS.
- R. D. Hancock, H. Maumela and A. S. Sousa, Macrocyclic Ligands With Pendent Amide And Alcoholic Oxygen Donor Groups, Coord. Chem. Rev., 1996, 148, 315–347 CrossRef CAS.
- V. J. Thoem, J. C. Boeyens, G. J. McDougall and R. D. Hancock, Origin Of The High Ligand Field Strength And Macrocyclic Enthalpy In Complexes Of Nitrogen-Donor Macrocycles, J. Am. Chem. Soc., 1984, 106(11), 3198–3207 CrossRef CAS.
-
(a)
O. Kahn, Molecular magnetism, VCH, New York (N.Y.), 1993 Search PubMed;
(b) N. F. Chilton, R. P. Anderson, L. D. Turner, A. Soncini and K. S. Murray, PHI: A Powerful New Program for the Analysis of Anisotropic Monomeric and Exchange-Coupled Polynuclear d- and f-block Complexes, J. Comput. Chem., 2013, 34, 1164–1175 CrossRef CAS.
- L. J. Farrugia, WinGX and ORTEP for Windows: an Update, J. Appl. Crystallogr., 2012, 45, 849–854 CrossRef CAS.
- G. M. Sheldrick, Crystal Structure Refinemnet with SHELXL, Acta Crystallogr., Sect. C: Struct. Chem., 2015, 71, 3–8 Search PubMed.
- O. V. Dolomanov, L. J. Bourhis, R. J. Gildea, J. A. K. Howard and H. Puschmann, OLEX2: A Complete Structure Solution, Refinement and Analysis Program, J. Appl. Crystallogr., 2009, 42, 339–341 CrossRef CAS.
- C. F. Macrae, I. J. Bruno, J. A. Chisholm, P. R. Edgington, P. McCabe, E. Pidcock, L. Rodriguez-Monge, R. Taylor, J. van de Streek and P. A. Wood, Mercury CSD 3.0– New Features for the Visualization and Investigation of Crystal Structures, J. Appl. Crystallogr., 2008, 41(2), 466–470 CrossRef CAS.
-
K. Nakamoto, Infrared and Raman spectra of inorganic and coordination compounds, Wiley, Hoboken, N.J., 2009 Search PubMed.
-
(a) L. Thompson, S. Tandon, F. Lloret, J. Cano and M. Julve, An Azide-Bridged Copper(II) Ferromagnetic Chain Compound Exhibiting Metamagnetic Behavior, Inorg. Chem., 1997, 36(15), 3301–3306 CrossRef CAS;
(b) N. Dwivedi, S. K. Panja, A. Verma, T. Takaya, K. Iwata, S. S. Sunkari and S. Saha, NIR Luminescent Heterodinuclear [Zn(II) Ln(III)] Complexes: Synthesis, Crystal Structures and Photophysical Properties, J. Lumin., 2017, 192, 156–165 CrossRef CAS;
(c) P. Pandey, A. Verma, K. Bretosh, J.-P. Sutter and S. S. Sunkari, Template Directed Synthesis of Half Condensed Schiff Base Complexes of Cu(II) and Co(III): Structural and Magnetic Studies, Polyhedron, 2019, 164, 80–89 CrossRef CAS;
(d) N. Dwivedi, S. S. Sunkari, A. Verma and S. Saha, Molecular Packing Dependent Solid State Fluorescence Response of Supramolecular Metal–Organic Frameworks: Phenoxo-Bridged Trinuclear Zn(II) Centered Schiff Base Complexes with Halides and Pseudohalides, Cryst. Growth Des., 2018, 18(9), 5628–5637 CrossRef CAS.
-
(a) J. Ribas, M. Monfort, B. Ghosh, R. Cortés, X. Solans and M. Font-Bardía, Two New Antiferromagnetic Nickel(II) Complexes Bridged By Azido Ligands in The cis position. Effect of The Counteranion On the Crystal Structure and Magnetic Properties, Inorg. Chem., 1996, 35(4), 864–868 CrossRef CAS.
-
(a) J. Ribas, M. Monfort, C. Diaz, C. Bastos and X. Solans, New Antiferromagnetic Dinuclear Complexes Of Nickel(II) With Two Azides As Bridging Ligands. Magneto-Structural Correlations, Inorg. Chem., 1993, 32(16), 3557–3561 CrossRef CAS.
-
(a) S. Bai, C. Fang, Z. He, E. Gao, C. Yan and T. Hor, Chelating Schiff Base Assisted Azide-Bridged Mn(II), Ni(II) and Cu(II) Magnetic Coordination Polymers, Dalton Trans., 2012, 41(43), 13379–13387 RSC;
(b) F. A. Mautner, C. Berger, B. Sudi, R. C. Fischer, A. Escuer, R. Vicente, J. Cano, L. Öhrström, M. A. M. Abu-Youssef and M. A. S. Goher, Azido-Bridging Cobalt(II) Systems: Crystal Structures and Magnetic Properties, Acta Crystallogr., Sect. A: Found. Crystallogr., 2013, 69, 624–624 Search PubMed;
(c) E. Ruiz, J. Cano, S. Alvarez and P. Alemany, Magnetic Coupling in End-On Azido-Bridged Transition Metal Complexes: A Density Functional Study, J. Am. Chem. Soc., 1998, 120(43), 11122–11129 CrossRef CAS;
(d) S. Mistry, J. Sutter and S. Natarajan, Syntheses, Structures, and Magnetic Behavior of New Azide Linked Compounds with One- and Two-Dimensional Structures, J. Inorg. Gen. Chem., 2017, 643(22), 1722–1738 Search PubMed.
|
This journal is © The Royal Society of Chemistry 2022 |
Click here to see how this site uses Cookies. View our privacy policy here.