DOI:
10.1039/D1LC00960E
(Critical Review)
Lab Chip, 2022,
22, 2451-2475
Lateral flow assays for hormone detection
Received
25th October 2021
, Accepted 7th June 2022
First published on 17th June 2022
Abstract
Endocrine diseases are the fifth most common cause of death and have a considerable impact on society given that they induce long-term morbidity in patients. For many decades, the measurement of hormones has been of great interest since this can be used to diagnose a plethora of pathological conditions. As a result, the endocrine testing market has experienced exponential growth. Several techniques have been utilised for the detection of hormones; however, they are expensive, laborious and require specialist training. Conversely, lateral flow assays (LFAs) are cheap (<£1) and rapid (<5 min) devices. LFAs typically rely on biochemical interactions between antibodies and antigens to produce coloured signals proportional to analyte concentrations, which can be visually inspected. Given their simplicity, LFAs are now considered the most attractive point-of-care device in medicine. However, the measurement of hormones in biofluids using LFAs faces many challenges including (i) the necessity for sensitive detection methods, (ii) the need for multiplexed devices for the confirmation of a diagnosis, and (iii) difficulties in sample preparation and pre-concentration. As such, most hormone LFAs remain in the research phase, and the few that have been commercialised require further optimisation before they can be employed for routine use. This review summarises the basic principles underlying lateral flow technology and provides an overview of recent advances, challenges, and potential solutions for the detection of hormone biomarkers via LFAs. Finally, hormone LFA kits available on the market are presented, with a look towards future developments and trends in the field.
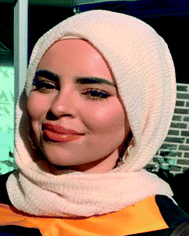 Leena Khelifa | Leena Khelifa is a medical student at Imperial College London. Leena achieved a bachelor's degree in Medical Sciences (Endocrinology) from Imperial College in 2021. She is currently undertaking an intercalated Ph.D. funded by the Cancer Research UK Convergence Science Centre. Her Ph.D. focuses on lateral-flow assays as a point-of-care diagnostic device for early neuroblastoma detection. |
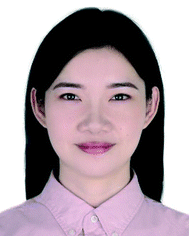 Yubing Hu | Dr. Yubing Hu is a Research Associate and Assistant Supervisor in the Department of Chemical Engineering at Imperial College London. Dr. Hu received a bachelor's degree from Zhejiang University in 2016 and earned a Ph.D. degree from the Hong Kong University of Science and Technology in 2020. Her PhD study focused on the development of fluorescent polymer materials for advanced sensing and imaging applications. Her current research aims to develop a variety of optical biosensors for portable, wearable and implantable diagnostic devices. She has been awarded FoE Julia Higgins Research Award at Imperial. |
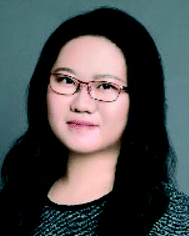 Nan Jiang | Dr. Nan Jiang earned her Ph.D. degree from Wuhan University of Technology. After her PhD study, she worked as a postdoctoral fellow and a research associate at Harvard University and Imperial College London. She is currently working as the Principal Investigator at Sichuan University. Her research is aimed at optical biosensors and microfluidic devices. She has been selected as the high-level overseas talent in Sichuan Province. She has published more than 30 peer-reviewed papers as first and corresponding authors. Some important works have been selected as “Cover Paper”, “Hot Paper”, and “Highly-cited Paper”. |
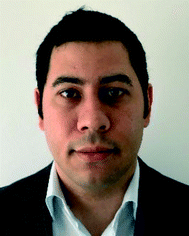 Ali K. Yetisen | Dr. Ali K. Yetisen is a Senior Lecturer and Associate Professor in the Department of Chemical Engineering at Imperial College London. He was previously a Tosteson fellow at Harvard University. He holds a Ph.D. degree in Chemical Engineering and Biotechnology from the University of Cambridge. He has been awarded several international prizes including IChemE Nicklin Medal, Birmingham Fellowship, MGH ECOR Award, Humboldt Research Fellowship Award, Carl Friedrich von Siemens Fellowship Award, and Fellowship of the Royal Society of Chemistry, Fellowship of the Higher Education Academy, and Fellowship of the Institute of Physics. |
1. Introduction
Finite control of vital metabolic functions is facilitated by the secretion of hormones by numerous glands in the body1,2 Hormone imbalances (excess or deficiency) are the result of a several conditions, many of which result in severe morbidity and a myriad of downstream systemic effects. Thus, the measurement and monitoring of hormones can be useful for the diagnosis of diseases. Furthermore, exogenous hormone administration can be used to treat many illnesses such as diabetes, and thus the ability to quantify hormone concentrations is essential for personalised and optimised therapy. Given the rise in endocrine-related disease, the global endocrine testing market is experiencing rapid growth.3 In 2016, the market was valued at £5.7 billion4 and it is expected to double by 2027.3 Factors such as lifestyle changes in the working population have led to increased hormonal imbalance. Growing incidence of diabetic, geriatric and obese populations worldwide also means further increase in the future is likely, due to the higher probability of chronic illness in these groups.5 Thus, hormonal monitoring and regulation is essential to the diagnosis and treatment of various diseases at present more than ever. Moreover, recent advances in technology have allowed for extremely rapid, portable, and affordable testing devices, which have all increased popularity and demand for endocrine tests.3
The development of several assays in the 20th century was pivotal to the current state of hormone biosensing. Radioimmunoassay, fluorescent assays and gas chromatography-mass spectrometry (GC-MS) are examples of conventional techniques for hormone measurement. However, these methods are time-consuming, expensive and require trained professionals and expensive equipment, thus limiting their use at point-of-care (POC). LFAs are rapid, paper-based diagnostic devices which can be employed for the detection of several analytes from a variety of biological samples.6 LFAs have been employed for the monitoring and diagnosis of various health states, including diabetes and pregnancy in both clinical and home settings. The implemetation of such devices will reduce hospitalisation duration and costs, thus helping to relieve the burden of endocrine diseases on the national health service (NHS). Furthermore, the use of LFAs at home by patients will promote awareness and responsibility and lead to accurate, continuous monitoring of hormone levels. In turn, abnormalities can be identified earlier, thus enabling earlier commencement of interventions, and reducing morbidity and mortality related to these illnesses.
This review summarises the basic components of LFAs and the different types of LFA which are available at present.We begin with a detailed discussion on the importance of hormone sensing and analysis in routine clinical settings followed by a brief introduction to LFAs and different mechanisms which can be used to enhance the performance of such devices. The use of LFAs in the endocrine world, their advantages and disadvantages and their function in comparison to gold standard devices in the field will be critically analysed. In this review, we also overview the analytical performance of developed and commercially available hormone LFAs that are paving the way for improved diagnosis and monitoring of endocrine disease. We will consider the current challenges in hormone detection via LFAs, the potential resolutions, and the future perspective and trends driving the LFA market to the forefront of endocrine testing.
2. Clinical significance and pitfalls in hormone monitoring
Several hormones are important to the control of fertility and pregnancy in women (Table 1). One of the first discovered hormones was human chorionic gonadotropin (hCG) which is produced in high quantities in the placenta.7 Direct measurement of molecules such as hCG was made possible following the invention of radioimmunoassay in 1959 and development of monoclonal antibodies later in the 1970s. In pre-menopausal women, levels of serum hCG remain below 5 IU L−1, rising to over 110
000 mIU mL−1 in pregnant women.8 Thus, the measurement of hCG has been implemented for pregnancy detection. Gonadotrophins luteinising hormone (LH) and follicle-stimulating hormone (FSH) are other important fertility hormones. In both men and women, these hormones are released from the anterior pituitary glands under direct control from gonadotrophin-releasing hormone. LH contributes to the maturation of primordial germ cells in both sexes. In men, LH stimulates testosterone production from Leydig cells, and in women it regulates the menstrual cycle and triggers hormone release from the ovaries.9 Levels of circulating and urinary LH, as well as another hormone estrone glucuronide (E3G), are a key indicator for fertility and reproductive health in women.10 Serum LH concentrations of 1.9–12.5 IU L−1 are typical of women in the follicular phase of the menstrual cycle.11 During the mid-cycle LH peak, a time that precedes ovulation, LH concentrations reach 8.7–76.3 IU L−1.11,12 In urine, similar trends are identified (Table 1). Thus, LH detection can be used to predict fertile windows for sexual intercourse to promote or avoid conception. FSH, on the other hand, promotes the function and maturation of follicles in females and seminiferous ducts in males. The increase in FSH concentration can also forecast ovulation. In women, FSH works closely with LH to control sexual function. For women of child-bearing age, physiological serum FSH lies between 4.7–21.5 mIU mL−1.13 After menopause, these levels rise dramatically to between 25.8–134.8 mIU mL−1.13 Therefore, FSH is considered a biomarker for the detection of infertility, and thus levels of this hormone can be used to identify the commencement of menopause in women. Measuring levels of oestrogen can also provide important information about the fertility, pregnancy, and menstrual cycle of patients. Oestrogen is produced in the ovaries, adipose tissue, adrenal glands, and placenta. One form of oestrogen, oestrone (E1), is produced by the body after menopause and serves as an oestrogen repository. The concentration of E1 in the blood varies greatly depending on the stage of the cycle that the patient is in. In post-menopausal women, serum E1 concentrations are around 14–102 pg mL−1.14 In saliva the normal physiological range for pre-menopausal women is between 3.2 and 7.9 pg mL−1, compared to much lower concentrations of 0.9–3.1 in post-menopausal women.14 The ratio of E1 to other oestrogens can be used to detect menopause in women. Oestradiol (E2) is the most common type of oestrogen in women of childbearing age. E2 plays a significant role in the growth of the cervix, fallopian tubes, myometrium, and vaginal lining, and is responsible for maintaining an appropriate environment in the ovaries for development of the egg cells. It also facilitates the development of secondary sex characteristics in women and the maintenance of pregnancy. E2 is also used in hormone replacement therapy (HRT).15 In menstruating women, normal E2 levels are around 15–350 pg mL−1.16 For post-menopausal women, normal levels should be below 10 pg mL−1.16 Thus, the measurement of E2 may be useful not only for assessment of normal female physiology, but also for optimisation of HRT and for determination of menopause. Another key regulator of the growth and development of a foetus is oestriol (E3). Low concentrations of this hormone in maternal circulation, alongside decreased alpha-foetoprotein (AFP) and increased hCG concentrations, can be indicative of foetal pathology (such as Down's syndrome). In non-pregnant women, serum estriol levels should remain below 0.18 ng mL−1.17 Salivary E3 tests are still in development and have not yet been standardised.
Table 1 An outline of vital hormone concentrations which can be measured in various biological fluids to diagnose diseases or predict health outcomes
Hormone |
Matrix |
Normal concentration |
Indication or pathology |
Associated concentration |
Ref. |
hCG |
Blood |
<5 mIU mL−1 |
Pregnancy |
>25 mIU mL−1 |
8
|
Urine |
<5 mIU mL−1 |
Pregnancy |
>25 mIU mL−1 |
8
|
LH |
Blood |
1.9–12.5 IU L−1 during follicular phase |
Ovulation |
8.7–76.3 IU L−1 |
11
|
Urine |
5–25 IU L−1 |
Ovulation |
12.2–118 IU L−1 |
18
|
E3G |
Urine |
<20 ng mL−1 |
Ovulation |
20–30 ng mL−1 |
10
|
FSH |
Blood |
4.7–21.5 mIU mL−1 |
Menopause/infertility |
25.8–134.8 mIU mL−1 |
13
|
E1 |
Blood |
Follicular phase: 37–138 pg mL−1 |
Menopause |
14–103 pg mL−1 |
14
|
Pregnancy |
>11 500 pg mL−1 |
14
|
Mid-cycle: 60–229 pg mL−1 |
Luteal phase: 50–114 pg mL−1 |
Saliva |
3.2–7.9 pg mL−1 |
Menopause |
0.9–3.1 pg mL−1 |
14
|
E2 |
Blood |
15–350 pg mL−1 in menstruating women |
Menopause |
25.8–134.8 mIU mL−1 |
16
|
E3 |
Blood |
<0.18 ng mL−1 |
Foetal pathology |
Low E3 |
17
|
Low AFP |
High hCG |
OS |
Blood |
<10 ng mL−1 |
Animal pregnancy |
>10 ng mL−1 |
19
|
Urine |
N/A |
Animal pregnancy |
N/A |
20
|
Progesterone |
Blood |
5–20 ng mL−1 in menstruating women |
Menopause |
<1 ng mL−1 |
21
|
Milk |
<4 ng mL−1 |
Animal pregnancy |
>4 ng mL−1 |
22
|
Testosterone |
|
Men: 300–1000 ng dL−1 |
Infertility |
Men: <200 ng dL−1 |
23
|
Women: 15–70 ng dL−1 |
Prolactin |
Blood |
<25 ng mL−1 |
Pregnancy |
80–400 ng mL−1 |
24
|
Pituitary tumours and other diseases |
>25 ng mL−1 |
25
|
Cortisol |
Blood |
8 am: 138–635 nmol L−1 |
Adrenal or pituitary disorders |
Cushing's: high |
26
|
4 pm: 83–359 nmol L−1 |
Adrenal insufficiency: low |
Urine |
<120 nmol/24 hour |
Adrenal or pituitary disorders |
Cushing's: high |
27
|
Adrenal insufficiency: low |
Saliva |
8 am: 10.2–27.3 ng mL−1 |
Adrenal or pituitary disorders |
Cushing's: high |
28
|
4 pm: 2.2–4.1 ng mL−1 |
Adrenal insufficiency: low |
Insulin |
Blood |
<25 mIU L−1 |
Diabetes |
<25 mIU L−1 after glucose load |
29
|
Vitamin D |
Blood |
30–100 ng mL−1 |
Deficiency |
<20 ng mL−1 |
30
|
TSH |
Blood |
0.5–5 mIU L−1 |
Hyperthyroidism |
<0.5 mIU L−1 |
31
|
Hypothyroidism |
>5 mIU L−1 |
T3 |
Blood |
100–200 ng dL−1 |
Hyperthyroidism |
>200 μg dL−1 |
31, 32 |
Hypothyroidism |
<100 μg dL−1 |
T4 |
Blood |
5–12 μg dL−1 |
Hyperthyroidism |
>12 μg dL−1 |
31, 32 |
Hypothyroidism |
<5 μg dL−1 |
PTHLH |
Blood |
<3.9 pmol L−1 |
Cancer |
>3.9 pmol L−1 |
33
|
In veterinary practise, oestrone sulfate (OS), a naturally occurring oestrogen precursor which can be measured in serum and urine, has shown to be almost 100% accurate in detection pregnancy in mares.20 OS is produced by the fetoplacental unit and its concentration peaks shortly after mating and in the last trimester of pregnancy. Milk progesterone levels are also an important hormone for on-site animal pregnancy monitoring. This provides information about pregnancy on day 19–21 following artificial insemination.34 Milk progesterone levels are higher and closely correlated to those in blood, thus providing a rapid and non-invasive testing method. Moreover, progesterone plays a significant role in regulating the menstrual cycle and maintaining pregnancy in humans. Serum levels of the hormone in post-menopausal women should be <1 ng mL−1, 5–20 ng mL−1 in menstruating women, and up to 90 ng mL−1 in pregnant women.21 Low levels of progesterone may indicate testosterone deficiency in men. Testosterone is an anabolic steroid hormone, synthesised primarily in the Leydig cells of male testes, female ovaries and adrenal glands of both sexes.35 The detection and quantification of this hormone is indicated for diagnosis of infertility and polycystic ovarian syndrome, among other diseases.36 A testosterone concentration of around 300–1000 ng dL−1 is considered normal in men and 15–70 ng dL−1 in women.23 Levels of prolactin, another key fertility hormone, fluctuate throughout pregnancy, reaching a peak at delivery and declining after lactation.25 The concentration of prolactin in the serum of non-pregnant women is less than 25 ng mL−1 compared to 80–400 ng mL−1 in pregnant women.24 Prolactin levels may be exceptionally high due to pituitary tumours, hypothalamic tumours and other conditions such as acromegaly and primary hypothyroidism.25 Therefore, the ability to rapidly measure these hormones could allow for faster diagnosis and treatment implementation for patients.
Cortisol is another key hormone released by the adrenal glands and regulated by the pituitary and hypothalamus.37 Fine control of cortisol levels is important as it is essential to the regulation of important metabolic functions, including glucoregulation and blood pressure control. Cortisol concentrations in blood follow a diurnal pattern: high in the morning (138–635 nmol L−1 at 8 am) and low in the evening (83–359 nmol L−1 at 4 pm),26 and similar trends are seen in urine. Imbalances in this could indicate diseases such as adrenal insufficiency or Cushing's disease. Insulin is another hormone involved in glucoregulation, and fasting levels of insulin should remain below 25 mIU L−1.29 After food, insulin levels in a healthy individual rise, however those with diabetes fail to increase their insulin to appropriate levels. Vitamin D is another endogenous hormone which is necessary for healthy growth and development. 25-Hydroxyvitamin D3 (25(OH)D3), measurable in blood, is most representative of vitamin D status. In the blood, optimal vitamin D levels are between 30–100 ng mL−1. Levels under 20 ng mL−1 indicate deficiency of the hormone,30 and thus can be used to identify patients who require vitamin D supplementation. The measurement of thyroid hormones is also essential for many individuals. Thyroid stimulating hormone (TSH) triggers the production and secretion of triiodothyronine (T3) and thyroxine (T4) which are important in controlling a myriad of biochemical processes essential for normal development.38 T3 and T4 are synthesised by the thyroid gland and work synergistically to regulate the body's metabolic rate, body weight, heart function, mood and bone maintenance. Under normal physiological circumstances, most T3 is derived from deiodination of T4. The quantification of these hormones allows patients and clinicians to monitor and evaluate thyroid functions. Normal blood T3 levels in adults should be between 100 and 200 ng dL−1, whilst T4 should be 5–12 μg dL−1.32 Deficiency of T3 and T4 may indicate hypothyroid diseases, whilst excess levels of these hormones in the blood could diagnose hyperthyroid conditions, such as thyroid carcinoma. Another hormone, parathyroid hormone-like hormone (PTHLH) is a poor prognostic marker for head and neck cancers and thus its measurement in tumour cell lysates can provide important diagnostic information for cancer.39
Great efforts have been taken to study hormones and obtain reference ranges to identify healthy and sick individuals. However, the ability to measure hormones accurately, rapidly, and sensitively is limited due to several factors such as the requirement of highly sensitive tests and matrix interferences. The most common hormone detection methods are immunometric assays, high-performance liquid chromatography (HPLC) and GC-MS. These methods have only been validated for certain hormones, and they are expensive, laborious and assays are often impacted by poor sensitivity. Most hormones circulate in the pico- (10−12) or nano (10−9) range,40 thus making it difficult to develop devices which are sensitive enough for their detection. Furthermore, there are often several closely related compounds present in biofluids which makes selectivity an issue for most tests; cross-reactivity is a particularly major issue for immunoassays given the utilisation of antibodies. This can cause false positive results leading to inappropriate follow up studies. Moreover, matrix components may cause interference in immunoassays, for example due to differences in binding proteins. Generally, bound and unbound hormones are measured together. This is because to measure total hormone levels, the hormone would need to be extracted from the binding protein first which requires several complex steps prior to assaying. Certain patients may have high or low binding protein concentrations, and this has been well-documented.40 For example, pregnant women and women on oral contraception have high binding protein levels whilst liver disease may lead to reduced levels of these proteins. Thus, while assays may perform well for healthy patients, they can lead to incorrect conclusions if used to test sick individuals.
Most hormones are measured in blood. The collection of blood for testing is invasive and comes with associated risks. Urine tests are becoming increasingly popular as non-invasive testing methods, although they often rely on 24 h collection which is time-consuming. For other biofluids, such as tears or sweat, there is a lack of research, and thus reference ranges and other important factors may not have been determined. Several important validation parameters must be considered during assay development (Table 2), through assay verification, analysis of controls and study samples, and comparison between different assays.41 The quality, robustness and diagnostic value of the device can then be determined. Home assay kits are limited to imprecisions during assay procedure by untrained individuals. Furthermore, coefficients of variants (CVs), which are used to determine assay precision, may be poor especially for samples which lower concentrations. Sample matrix can also influence results obtained from detection devices. Unstable hormones may degrade rapidly following sample collection, influencing the results obtained. Thus, timing and storage conditions used in a test procedure must be carefully controlled. Freeze–thaw cycles may impact results since some hormones are not resistant to repeated cycles.40 Differences in standardisation has also been a long-standing issue for hormone detection methods. Studies should ideally be carried out entirely in one location and under the same conditions. Further, duplicate (or triplicate) analyses can greatly improve test precision, due to reduction in human error. Thus, whilst the development of tests to investigate hormone concentrations are useful and entirely possible, several considerations must be made to ensure test validity, reliability and repeatability.
Table 2 Assay validation parameters for hormone detection
Parameter |
Sub-parameter |
Definition |
Precision |
Within-run |
Agreement of results of measurements obtained under the same conditions |
Between-run |
Agreement of results obtained from multiple analyses of samples over separate runs |
Measurement accuracy |
Method comparison |
Comparison between technique and well-established method in clinical use |
Spike-recovery |
Determination of impacts of sample matrix and diluent used to prepare the standard curve |
Detection limit (sensitivity) |
Limit of quantitation (LOQ) |
The lowest concentration of analyte that can be accurately detected |
Limit of detection (LOD) |
The smallest concentration of analyte which can be consistently and reliably distinguished from baseline |
Stability |
Ability of device to maintain integrity over a range of conditions |
Specificity/selectivity |
Ability of the test to distinguish the analyte from other components in the sample |
Diagnostic characteristics |
Reference interval |
A set of values used to interpret test results |
Threshold values |
Values used to provide positive and negative results from patient tests |
Detection range |
The highest and lowest quantifiable concentrations that yield results consistent with the accuracy and precision required by the assay |
3. Principles of LFAs
An LFA is a paper-based diagnostic device.6 It is used for the detection and quantification of biomarkers, such as hormones and antigens, from a variety of different samples, including whole blood and saliva.42 Conventional immunoassay techniques for biomarker detection such as enzyme-linked immunosorbent assay (ELISA) and radioimmunoassay require trained professionals and time for analysis. LFAs are cheap and simple to use, producing results within 5–30 minutes.6 LFAs are one-step assays that require low sample volumes to produce qualitative, semi-quantitative or quantitative results. The short development time, among the other advantages such as long shelf-life, make LFAs appealing for commercialisation. Despite this, inaccurate sample volumes may reduce the precision of LFAs, and restriction of sample volume can limit sensitivity. Good antibody preparation is necessary for the success of LFA analysis and bioreceptor cross-reactivity can limit sensitivity. Moreover, only one analyte can be detected; multiplexing of LFAs is extremely difficult, thus limiting the use of these devices for accurate POC differential diagnosis. Nonetheless, the global LFA market was estimated to be worth £4.4 billion in 2019 and is forecast to reach £5.8 billion by 2026.43
LFAs rely on the capillary flow of a sample through the device. Initially, the sample is introduced to the sample application pad, which ensures optimal characteristics such as viscosity, purity and pH to enable correct functioning of the assay.44 After this, the sample flows to the conjugate pad which, upon wetting, releases a labelled detection bioreceptor (for example an antibody conjugated to a nanoparticle). The bioreceptor is now able to bind to and ‘label’ the analyte in question. The sample then travels through the membrane which comprises at least 2 parts: a control line, to signify correct function of the LFA, and a test line which produces a colour representing the analyte concentration in the sample. Following successful binding, a line is generated on the LFA, signifying the presence or absence of the analyte. In generic sandwich LFAs, the labelled analyte binds to the capture bioreceptor at the test line (Fig. 1a). The stronger the signal formed at the test line, the greater the concentration of the analyte in the sample. Very small analytes are unable to provide sufficient binding space on their surface for adhesion to two bioreceptors. Therefore, a competitive LFA format is used (Fig. 1b). With this format, the analyte is absorbed onto the test line, and thus the labelled analyte from the sample competes with the immobilised analyte for binding to the labelled detection bioreceptor. Thus, test line signal is indirectly proportional to analyte concentration. The final aspect of the LFA is the absorbent pad, which enables complete flow of the sample. Depending on the bioreceptor utilised, results are most often visualised using an optical reader or by the naked eye. The design of an LFA depends on the analyte of interest. Different labels can be utilised to enhance the sensitivity of devices, as outlined in Table 3.
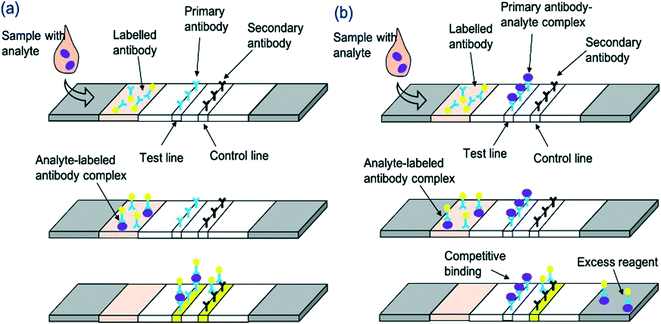 |
| Fig. 1 Schematic representation of LFA detection process utilising antibodies and nanoparticle conjugates for (a) sandwich LFA and (b) competitive LFA. | |
Table 3 Common labels in LFAs and their applications in hormone detection
Label |
Result |
Advantages |
Disadvantages |
Ref. |
Gold nanoparticles |
Qualitative, semi-quantitative or quantitative |
• Most used label |
Expensive if purchased for commercial use |
45–67
|
• High affinity toward biomolecules |
• Strong optical signal for naked eye detection |
Magnetic particles and aggregates |
Qualitative or quantitative |
• Coloured magnetic particles produce colour at the test line |
Requires a non-optical reader for magnetic measurements |
68–70
|
• Magnetic signals can also be measure quantitatively using a magnetic assay reader |
• Higher stability; increased sensitivity by 10 to 1000-fold |
Fluorescent and luminescent materials |
Quantitative |
• Amount of fluorescence can quantitate the concentration of the analyte |
Requires extra hardware which is expensive |
71–76
|
• Can be used with smartphones |
Enzymes |
Qualitative or quantitative |
• Colour produced at test line due to enzymatic reaction |
Longer process for detection |
77–80
|
• Enzymes can be loaded with gold nanoparticles for enhanced sensitivity |
Colloidal carbon |
Qualitative or quantitative |
• Cheap |
Weaker signal than gold nanoparticles |
34, 81 |
• Black colour so easily detected with high sensitivity |
4. Research advances in hormone LFAs
The success of diagnostic devices can be determined in the research phase by investigating the limit of detection (LOD), detection range, sensitivity, and specificity of the device, among other important parameters (Table 2). This section will discuss, to the best of our knowledge, the developments in LFA technology for hormone detection, including the use of mobile phone devices and multiplexing to enhance usability and effectiveness of these devices for disease diagnosis and monitoring. This information is essential to understanding the different methods that have been employed to detect each hormone and the success of these for their intended purpose, for example a sufficient range for diagnosis of disease. We will consider the pitfalls in current hormone LFAs, and future trends which will work to overcome these issues. This will enable future researchers to improve upon these methods and enhance the analytical ability of existing devices. In general, hormone LFA research has focussed on fertility hormone, stress hormone and thyroid hormone monitoring. Diagnosis of pregnancy through hCG monitoring is one of the most well-known applications of these devices, and the detection ranges, LOD and sensitivity provided by LFAs are sufficient for this. For investigation of fertility, LFAs for LH, oestrogens, and testosterone have been developed. Measurement of LH using LFAs has been successful, and smartphone applications have been developed to enhance these methods. Oestrogen and testosterone LFAs have all been developed in multiplexed formats, thus allowing for simultaneous diagnosis of infertility or diseases such as polycystic ovarian syndrome at POC. However, there has been no research into testosterone detection in biofluids using an LFA, and the ranges present in research are insufficient to detect elevated or deficient testosterone concentrations, thus providing a potential avenue for future research. The measurement of progesterone in particular has been researched in several formats, including using fluorescent and enzyme-based labels, which has been shown to enhance sensitivity for on-site monitoring of farm animals. Cortisol is another well-researched hormone, due to its implication is several disease states. Many methods have been employed to enhance the use of LFAs for cortisol detection, and these have provided good clinical ranges for the diagnosis of disease. Furthermore, LFAs exist for serum, salivary, plasma and sweat cortisol providing both non-invasive and quantitative techniques. In the future, the development of a multiplexed cortisol LFA could enhance the application of these devices by enabling POC differential diagnosis of disease. Smartphone applications for cortisol, LH and TSH LFAs have been developed in research, which enable patients to monitor their health at home. This will improve doctor-patient relationships and as the information can be shared from the app directly to the healthcare providers, for continuous patient monitoring. Here we analyse the different methods utilised and compare their analytical performances, as summarised in Table 4.
Table 4 Details of design and analytical performances related to hormone LFAs in literature
Hormone/analyte |
Sample/matrix |
Type of assay |
Label utilised |
Detection method |
Limit of detection |
Detection range |
Ref. |
hCG |
Urine |
Sandwich |
Colloidal selenium |
Colorimetric, naked eye |
50 mIU mL−1 |
>50 mIU mL−1 |
82
|
Sandwich |
Colloidal carbon |
Colorimetric, naked eye |
10 mIU mL−1 |
10–500 mIU mL−1 |
81
|
Serum |
Sandwich |
Gold nanoparticles |
Colorimetric, naked eye |
1 pg mL−1 |
>1 pg mL−1 |
46
|
Laboratory solutions |
Sandwich |
Super-paramagnetic nanoparticles |
Colorimetric, naked eye |
1 and 5 IU L−1 for magnetic particles with carboxyl content 13.6 and 136 micromol per mL respectively |
>5 IU L−1 |
70
|
LH |
Serum |
Sandwich |
Gold nanoparticles |
Electrochemiluminescence |
1 mIU mL−1 |
1–250 mIU mL−1 |
75
|
Sandwich |
Gold nanoparticles |
Electrochemiluminescence |
1 mIU mL−1 |
1–125 mIU mL−1 |
58
|
Oestrone sulphate |
Horse serum |
Competitive |
Blue-dyed polystyrene microspheres |
Colorimetric, naked eye |
5 ng mL−1 |
5–50 ng mL−1 |
19
|
E1 |
Serum |
Competitive |
Superparamagnetic particles |
Colorimetric |
0.25 ng mL−1 |
0.29–28.8 ng mL−1 |
69
|
E2 |
Powdered milk |
Competitive |
Colloidal gold |
Colorimetric, naked eye |
37.14 pg mL−1 |
37.14–1484.65 pg mL−1 |
67
|
E1, 17-β-E2 and E3 |
Milk |
Competitive |
Colloidal gold |
Colorimetric, naked eye |
0.053 ng mL−1, 0.061 ng mL−1, 0.038 ng mL−1 |
0.107–1.964 ng mL−1, 0.123–1.066 ng mL−1, 0.076–2.0315 ng mL−1 |
57
|
Progesterone |
Bovine milk |
Competitive |
Colloidal gold |
Colorimetric, naked eye |
5 ng mL−1 by visual inspection |
2–30 ng mL−1 |
47
|
2 ng mL−1 using photometric analysis |
Competitive |
Enzyme |
Colorimetric, strip reader |
0.8 ng mL−1 |
1–10 ng mL−1 |
78
|
Competitive |
Colloidal carbon |
Colorimetric, naked eye |
0.6 ng mL−1 |
0.6–1.2 ng mL−1 |
34
|
Cattle plasma |
Competitive |
Fluorophore labels |
Fluorescent, fluorescent reader |
0.1 ng mL−1 |
0.1–3.7 ng mL−1 |
71
|
Environmental samples |
Competitive |
Gold nanoparticles |
Colorimetric, naked eye |
5 nM |
>5 mM |
49
|
17-α-Hydroxy-progesterone |
Serum |
Competitive |
Colloidal gold |
Colorimetric, naked eye |
2.5 μg L−1 by visual observation |
2.5–50 μg L−1 |
55
|
Testosterone |
Water |
Competitive |
Gold nanoparticles |
Colorimetric, naked eye |
5 ng mL−1 |
>5 ng mL−1 |
52
|
Cortisol |
Saliva |
Competitive |
Luminol/enhancer/hydrogen peroxide |
Chemiluminescent, chemiluminescent reader |
0.4 ng mL−1 |
Covers relevant physiological range (0.6–10 ng mL−1) |
74
|
Competitive |
Enzyme |
Colorimetric, optical reader |
1 ng mL−1 |
1–10 ng mL−1 |
80
|
Competitive |
Enzyme |
Colorimetric, optical reader |
1 ng mL−1 |
1–10 ng mL−1 |
83
|
Competitive |
Gold nanoparticles with silver enhancement |
Colorimetric, naked eye |
0.5 ng mL−1 |
0.5–150 ng mL−1 |
62
|
trapLFI |
Enzyme and gold nanoparticles |
Colorimetric, strip reader |
0.01 ng mL−1 |
0.01–100 ng mL−1 |
77
|
Competitive |
Gold nanoparticles |
Colorimetric, naked eye |
0.37 ng mL−1 |
0.5–15 ng mL−1 |
60
|
Competitive |
Fluorescent dye |
Fluorescent, smartphone-based fluorescent reader |
0.1 ng mL−1 |
>0.1 ng mL−1 |
73
|
Competitive |
Luminol/enhancer/hydrogen peroxide |
Chemiluminescent, chemiluminescent reader |
0.3 ng mL−1 |
0.3–60 ng mL−1 |
72
|
Plasma |
Competitive |
Colloidal gold |
Colorimetric, naked eye |
3.5 ng mL−1 |
3.5–1280 ng mL−1 |
45
|
Serum |
Competitive |
Colloidal gold |
Colorimetric, naked eye |
30 ng mL−1 for visual detection |
30–250 ng mL−1 |
59
|
Sweat |
Competitive |
Gold nanoparticles |
Colorimetric, naked eye |
1 ng mL−1 |
Covers 10–100 ng mL−1 (normal cortisol level in sweat) |
61
|
TSH |
Serum |
Sandwich |
N/A |
N/A |
10 mlU L−1 |
>10 mIU L−1 |
84
|
Sandwich |
Gold nanoparticles |
Smartphone readout |
0.31 mlU L−1 |
0.31–0.5 mIU L−1 for hyperthyroidism, >5 mIU L−1 for hypothyroidism |
54
|
Sandwich |
Gold core nanocomposites |
Colorimetric, naked eye |
5 μIU mL−1 for colorimetric, 0.1 μIU mL−1 for fluorescent |
>5 μIIU mL−1 |
53
|
Fluorescent, fluorescent reader |
Sandwich |
Nanomagnetic |
Magnetic particle quantification readout |
0.017 mlU L−1 |
0.017–30 mIU L−1 |
68
|
Clinical fluids |
Sandwich |
Raman reporter-labelled gold nanoparticles |
Raman spectra/SERS mapping |
0.025 mlU L−1 |
>0.025 mIU L−1 |
65
|
Parathyroid hormone-like hormone |
Cancer cell cultures |
Sandwich |
Gold nanoparticle |
Colorimetric, naked eye |
1.42 ng mL−1 |
?1.42 ng mL−1 |
51
|
Insulin |
Whole blood |
Sandwich |
Gold nanoparticle |
Colorimetric, POC analyser |
9.72 pmol L−1 |
21.81–275.72 pmol L−1 |
48
|
Vitamin D |
Blood |
Competitive |
Gold nanoparticles |
Colorimetric, smartphone-assisted imaging platform |
— |
Covers complete physiologically relevant range 0–150 nmol L−1 |
66
|
4.1 Pregnancy and fertility
4.1.1 Human chorionic gonadotrophin.
Whilst pregnancy tests have been commercially available for some time, scientists have continued to research novel detection methods for enhanced sensitivity, rapidity, or ease of use. An example of a typical hCG LFA is the Abbott TestPack Plus™ hCG urine assay, which was described by Osikowicz et al.82 in 1990. The system demonstrated high specificity (no cross-reaction with LH or FSH, two hormones with similar homology to hCG). However, the LOD of 50 mIU mL−1 meant that early tests were not sensitive enough to detect the early stages of pregnancy and could only be used to determine pregnancy at a later stage once hCG levels had risen. Another issue identified was that assay sensitivity increased with time, meaning that low concentrations could show positive results after 30 minutes. However, such a lengthy assay time is not desirable with LFAs. The implementation of colloidal carbon nanoparticles81 later in in 1994 created a darker colour at the test line than typical LFAs, thus allowing more easily detectable results even at low analyte concentration. The LOD was significantly lower than the Abbott TestPack PlusTM hCG Urine assay (10 mIU mL−1 (ref. 81) compared to 50 mIU mL−1 (ref. 82)), meaning that all pregnant cases could be detected. Whilst promising, this study provided only pilot experiments as the LFA was not optimised in terms of sensitivity. Although both within run and between run CVs were under 10% at 50, 150 and 450 mIU mL−1, showing good precision of results at these concentrations, the detection range of 10–500 mIU mL−1 would only differentiate pregnancy up to 4 weeks, after which extremely high concentrations of hCG are observed.
Later, in 2006, Tanaka et al.46 described a novel urine hCG LFA format using gold conjugates with enhanced sensitivity (Fig. 2a and b). The conjugation of gold nanoparticles to the primary antibodies and conjugate antibodies enhanced the localised surface plasmon resonance (LSPR) of the colloidal gold, leading to a more intense colour formation at the test line and enhanced sensitivity (1 pg mL−1 under optimal conditions). Conjugation of hCG antibodies to superparamagnetic Fe3O4/poly(St-co-MPS)/SiO2 composites (average size 140 nm) was later trialled in a 2011 study.70 Analysis of the LFA showed that the carboxyl content in the functionalised particles affected the LOD and sensitivity of the test, leading to a low LOD of 1 ng mL−1,70 which is sufficient to cover the normal physiological range of hCG as well as pregnant concentrations. The use of superparamagnetic conjugates70 produced a 10-fold and 50-fold more sensitive LFA compared to carbon81 or gold82 respectively, although the gold enhancement method described by Tanaka et al.46 was superior.
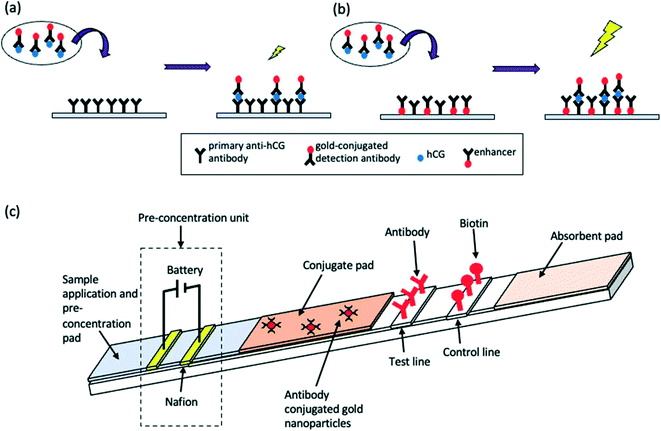 |
| Fig. 2 Schematic illustrations of enhancement methods for hCG LFAs. (a and b) Test principle of a sandwich hCG LFA (a) before and (b) after enhancement using gold nanoparticles. (c) LFA and pre-concentration unit. | |
More recently, researchers have attempted to modernise existing LFAs for digital and smartphone-based use. Hamad et al.85 achieved a promising proof-of-concept hybrid device which incorporated an electrochemical-based transducer on a conventional LFA strip in order to form an on-chip rapid hCG detection method. This study proved the possibility of digitalisation for commercially available rapid tests to allow for quantitative and reliable analyte detection. Around the same time, a battery operated pre-concentration-assisted LFA was described by Kim et al.64 in which the utilisation of a simple 9 V battery with a low power consumption (81 μW) allowed for a 25-fold pre-concentration factor, demonstrating significant sensitivity enhancement of a generic LFA (Fig. 2c). The researchers demonstrated the use of a single gate pre-concentrator (SGP) and dual gate pre-concentrator (DGP). The use to the DGP allowed for a significantly more stable preconcentration plug over an operation time of 20 minutes. The fluorescence intensity was also observed over various voltages utilising the DGP; a 25-fold preconcentration factor was acquired when 9 V DC was applied for 20 minutes. Consequently, clear colours were observed at the test lines which could otherwise not be monitored without the pre-concentration factor. Another novel and recent development for LFAs is multiplexing. One multiplexed LFAs has been developed to simultaneously detect hCG and pathogenic bacteria Escherichia coli O157
:
H7 and Salmonella typhimurium.86 The modified immunoassay allowed for novel POC detection and demonstrated the future move toward multiplexed LFAs. In accordance with the trends observed in the literature, it is likely that the future move for hCG LFAs will include implementation of novel labels for enhanced sensitivity, multiplexing for differential diagnosis of disease, and further digitalisation for enhanced ease of use. Since specificity does not seem to be an issue for hCG LFAs, the implementation of alternative recognition molecules (e.g., aptamers) could be trialled, but would be of less importance.
4.1.2 Luteinising hormone (LH) and estrone-3-glucuronide (E3G).
Like hCG, sandwich LFAs are used for LH detection. Behre et al.12 researched the use of the ClearPlan® fertility monitor: a commercially available LH dipstick at home for determination of fertile windows in women. The monitor was able to store data for several months to provide gynaecologists with valuable information about the menstrual cycles of patients being treated for infertility. The ClearPlan fertility monitor enabled non-invasive and rapidly detection of ovulation in 91.1% of instances. However, it seems that the analytical performance of the device itself was not tested in this study, thus questions remain about the sensitivity, specificity, and other analytical performance factors of the device, which would be of great importance. A variety of designs, including different labels and the use of smartphone software for quantitative detection, have since been suggested for LH detection. In 2017, Liu et al.58 coupled LH LFA technology with smartphone devices. Once images of a lateral flow strip were obtained using a smartphone, the test and control lines were extracted using canny edge detection operator and fuzzy c-means (FCM) clustering algorithms. The study concluded that the method was highly sensitivity as the LOD was very low (1 mIU mL−1). Detection of serum LH was complete within 15 minutes and a correlation coefficient of 0.964 was recorded when the method was tested on clinical serum samples.58 The method was later developed for detection of LH serum and urine samples. This test had a significantly larger detection range of 0–250 mIU mL−1 (ref. 75) compared to 0–125 mIU mL−1 (ref. 58) for the urine-only LFA. Despite success of these studies in confirming the ability of LH LFAs to determine fertile windows through measurement of LH surges, selectivity has not been determined. This is one of the most crucial parameters influencing the success of a device. Several similar molecules including hCG, FSH and TSH may interfere with the results obtained, meaning that in patients with illnesses such as thyroid disease, results obtained by the LFA may be impacted. Selectivity testing will be essential to the future optimisation of LH LFAs. Furthermore, the stability of the LFAs has not been tested, which is especially important for home monitoring tools, as a device must be able to maintain integrity in a wide range of conditions. This is another area for future studies.
E3G, a urinary metabolite of oestrogen, should be closely monitored alongside LH as it gives a direct measure of follicular growth. In 1999, Bonnar et al.87 carried out a large prospective study on personal hormone monitoring for contraception using the Persona LFA system. The hand-held device produced green and red light to indicate fertile and infertile phases, respectively. The study found that the detection of LH and E3G for contraception had a 93.8% efficacy, although 162 pregnancies occurred in 7209 cycles. Whilst not as effective as medical contraception, hormone monitoring is simple and an alternative for women who do not wish to use other forms of contraception. Recently, an “Inito” device was developed88 which, like the LFA by Bonnar et al.,87 measured both LH and E3G simultaneously. The test strips utilised blue latex particles. This device was also coupled to a smartphone to predict the fertile window in participants. Both the Inito and Persona systems are useful and provide a simple device for planning of pregnancy or contraception, or general monitoring of female reproductive health. However, the Inito reader has the advantage of quantitative results which can be used by gynaecologists. Furthermore, the Inito app could be used to collect and store data. Again, the experiments lacked in depth analytical testing, such as selecitivity testing, which would be a crucial future step to ensure the efficacy of the device for testing of patient samples.
4.1.3 Oestrone sulphate (OS).
Henderson et al.19 developed a serum OS LFA which was able to distinguish pregnant mares from non-pregnant ones. The test was based on a competitive principle whereby the intensity of the blue dot at the test line correlated inversely to the concentration of OS; this could be assessed visually or by computer analyses. The test had a short read-out time of 15–20 minutes. The developed device produced qualitative results and the results obtained were dependent on visual observation of ‘dark’ or ‘faint’ blue lines. This can be subjective, thus development of quantitative detection methods using fluorescent or smartphone-based readout systems, for example, could augment the use of such devices in the future. Furthermore, a major issue which may be identified during use of such a device is that the duration of OS peaks are short;20 so, negative results may be achieved if the test is performed too early or too late. Sample preparation was also an issue, since the test was dependent on blood collection, followed by mixing of the blood with buffer and label, before the dipstick could be dipped into the sample. This could be improved through development of novel OS LFAs for detection in urine, which has already been documented as a biofluid for OS detection. There seems to be a lack of research into LFAs for OS; development and testing should be undertaken in the future before commercialisation of these techniques. It is also likely that multiplexed OS LFAs will be developed in the future for the evaluation of high-risk pregnancies in horses, given that OS, along with other hormones such as progesterone, can be indicative of foetal pathology.
4.1.4 Oestrogens.
In 2017, a competitive LFA for the detection of E3 was described.69 It incorporated immobilised E3 at the test line, competing for anti-E3 antibodies conjugated to superparamagnetic particles. Results were obtained within 15 minutes and the LOD was 0.25 ng mL−1. In healthy individuals, E3 concentrations in blood remain under 0.25 ng mL−1, rising to 2.5 ng mL−1 in the first trimester of pregnancy, and reaching up to 14.6 ng mL−1 in the third trimester. A finding of low E3, coupled with low AFP and elevated hCG concentrations, can be used to diagnose Down's syndrome in a foetus. Thus, enhanced sensitivity would be important to detect very low E3 concentrations in these patients, and multiplexing would be necessary to make this device suited for the requirements. An important advantage of this assay was that it required no sample pre-treatment, enhancing ease of use. However, the inter- and intra-assay CVs ranged between 5 to 13%, showing slight lack in precision. There is a need for further exploration to find more appropriate antigen–antibody pairs to improve the performance of the system and feasibility of the system for testing blood samples in clinics.
Levels of oestrogens in powdered milk have been a growing concern in recent years, due to their unknown effects on human health following consumption. Recently, an LFA for the detection of oestradiol (E2) in milk was described.67 In 2018, Wang et al.57 also produced a competitive colloidal gold LFA which allowed the determination of E3, oestrone (E1) and 17-β-oestradiol (17-E2) in milk samples. The test consisted of one test line which could bind any of the hormones with sufficient sensitivity. Both tests were found to be rapid, although the multiplexed LFA produced results in 10 minutes (ref. 57) compared to only 8 minutes for the E2 LFA.67 The LOD for E2 detection was extremely low (37.14 pg mL−1)67 indicating high sensitivity. Furthermore, the results of the E2 LFA were relatively identical to those obtained by HPLC and required significantly shorter analysis time. While the results are promising and the LFAs for detection of oestrogens in milk have been well validated, more research into the implications of drinking milk with elevated oestrogen levels is required. This is important given the rise in health-consciousness in the population; if side-effects are identified, this could work to propel the development of such a device into the future.
4.1.5 Progesterone.
The first paper to describe an LFA for progesterone detection was published in 1996.47 The researchers demonstrated detection of the hapten using a competitive LFA with gold-labelled progesterone conjugates for competitive binding. Analysis took only 10 minutes, and the LOD was 5 ng mL−1 and 2 ng mL−1 for visual and photometric analysis, respectively. However, the results showed high variability; this could be improved in the future by preparing the detection zone with an ink jet rather than manually. Furthermore, some cross-reactivity was observed including 18.7% with pregnenolone and 39.12% with 11-alpha-progesterone. This could be enhanced through the use of aptamers instead of antibodies. To overcome these issues, colloidal carbon was later implemented as a label.34 Significantly increased sensitivity was observed; a LOD of 0.6 ng mL−1 was achieved, which was 3-fold more sensitive than the colloidal gold LFA.47 However, the assay was developed to detect the hormone in buffer. In future, spike recovery testing would be important since this measures the effects of the chosen matrix (milk) and its constituents on the results obtained through comparison of results to those obtained from buffer used in the calibration curve. Thus, the ability of the test to accurately quantify the analyte in milk can be established. The studies also concluded the requirement for additional sample pre-treatment (e.g., solid-phase extraction) for purification. Further studies would be required to develop an off-laboratory LFA.
In 2015, another milk progesterone LFA, this time utilising enzymes, was introduced78 (Fig. 3a). The test was rapid (results in 15 minutes) and provided good correlation with ELISA (R = 0.97, n = 46) (Fig. 3b). The LFA was also able detect progesterone in a sufficient range (Fig. 3c and d). The use of enzyme labels provided up to 30 times higher sensitivity compared to conventional LFA labels such as colloidal gold.47 Following the trend to implement new molecules to enhance LFA application, a competitive LFA utilising gold nanoparticle-duplexed aptamer conjugates49 for progesterone detection was described in 2019. As expected, this showed excellent sensitivity compared to antibody-based assays. The device was developed to test progesterone levels in water and was thus tested and validated using water samples. In future, a similar technique could be developed for detection of progesterone in cows' milk to detect pregnancy. The first serum progesterone LFA was described in 2020.71 This comprised of a disposable fluorescence immunoassay (FIA) coupled with a portable imaging device which allowed for estimation of circulating plasma concentrations of progesterone in bovine plasma. Such a device could be useful for rapid everyday use by farmers without the need for specialist equipment or training. However, collection of blood samples is difficult for on-site monitoring of cows by farmers. Furthermore, despite high sensitivity, selectivity remains an issue with progesterone measurement.
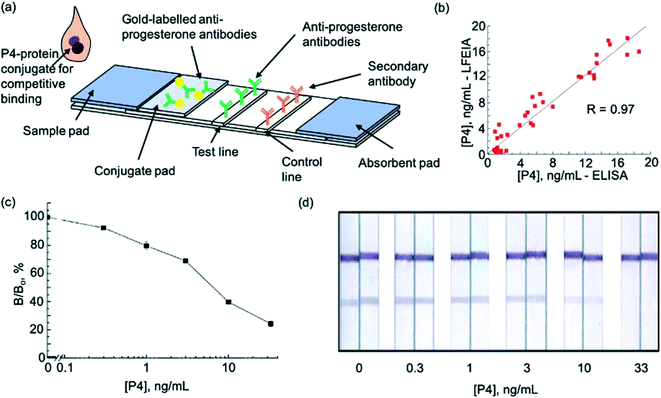 |
| Fig. 3 Enzyme lateral flow immunoassay for pregnancy testing in cows. (a) Schematic illustration of test strip (b) comparison of P4 measurements in cows' milk using ELISA and LFA (c) P4 LFA calibration curve. (d) Visualisation of calibration curve after staining. Reproduced with permission.57,78 Copyright 2015, Elsevier. | |
Pregnanediol glucuronide is a major metabolite of progesterone and can be measured accurately in urine since it correlates directly with levels of the hormone in serum, allowing for minor time delays. Monitoring the levels of this hormone is indicated for both ovulation and the fertility cycle. In 2018, Matías-García et al.89 described a smartphone-linked LFA for accurate quantitation of pregnanediol glucuronide for use in ovulation and fertility diagnostics. The assay was found to have a low LOD (4.8 μg mL−1). 17-α-Hydroxyprogesterone is another endogenous progesterone-related hormone for which a competitive LFA has been described.55 In serum, this hormone is a marker for congenital adrenal hyperplasia. The test strip for the detection of 17-α-hydroxyprogesterone utilised gold nanoparticles and produced results in only 15 minutes, making the device promising for on-site diagnosis of this disease from small plasma samples. Further research would be required to validate these assays and enhance their use. It should also be noted that progesterone can be used as a biomarker for menopause in women, and thus it should be expected that future LFAs will be developed for this application and tested using human blood or urine samples.
4.1.6 Testosterone.
The implementation of LFAs for testosterone detection has been a recent development and one for which there is little research. In 2014, the first LFA for testosterone detection was described.52 The test was a multiplexed, competitive LFA with 5 separate test lines, each of which comprised an immobilised antigen which would compete with its specific analyte for binding to gold-labelled antibodies (Fig. 4a and b). The LFA was highly sensitive and provided good accuracy and reproducibility of results. The results were obtained within 20 minutes. Furthermore, no pre-treatment of the samples was required, meaning the test was extremely rapid and simple to use and would not require trained personnel or equipment. However, to this day, no testosterone LFA has been developed for the measurement of the hormone in human biofluids. This will likely be developed soon, given the implications of testosterone in both male and female fertility and disease. Testosterone is morphologically very similar to other endogenous hormones such as oestriol, therefore selectivity is a potential issue that will be encountered. Researchers should thus look to the development of aptamer-based devices. Moreover, testosterone is present in blood at lower concentrations than other fertility hormones. The LOD provided by the multiplexed LFA was 5 ng mL−1; a 30-fold more sensitive device would be required to detect normal testosterone concentrations in females. Thus, emerging enhancement techniques must be considered, such as colloidal carbon labels, or fluorescent labels such as quantum dots.
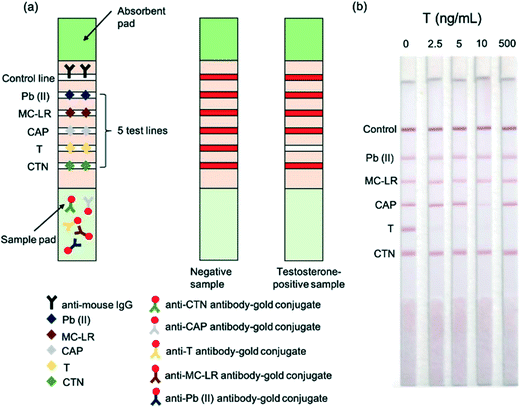 |
| Fig. 4 Functioning of the multiplexed testosterone LFA. (a) Schematic illustration of the multiplexed competitive LFA for the detection of heavy metal lead (Pb(II)), algal toxin microcystin-leucine-arginine (MC-LR), antibiotic chloramphenicol (CAP), testosterone (T) and pesticide chlorothalonil (CTN) in water (b) images of LFA strips for different concentrations of testosterone. Reproduced with permission.44 Copyright 2015, Elsevier. | |
4.2 Cortisol
Due to the recent implication of cortisol as a biomarker for stress, detection of this hormone has gained widespread interest. LFAs for the detection of cortisol in blood have been developed. Leung et al.45 described a one-step cortisol LFA which produced a test line signal that increased with increasing cortisol concentration, despite being a competitive LFA. The LFA utilised cortisol-BSA at the test line (termed immune-threshold capture analyte) which competed with cortisol in the sample for binding to the labelled IgG. This meant that, when present in the sample, cortisol would bind to the IgG and be immobilised at the test line, unlike the standard competitive format. Thus, the proposed LFA had an advantage of simpler analysis by the user. The test strips were evaluated using a stability test and accelerated stability test; it was confirmed that the strips could be kept at room temperature for up to 1 year without any significant loss of activity. Cross-reactivity was low, and no sample pre-treatment was required- both huge advantages for commercialisation. Later, Nara et al.59 described a competitive LFA which utilised colloidal gold nanoparticles conjugated to cortisol-3-carboxymethyloxime–adipic acid dihydrazide–bovine serum albumin (F-3-CMO–ADH–BSA) for competition with cortisol. This method also facilitated semi-quantitative results by use of a reference shade card. The LOD of 30 ng mL−1, although enabling a preliminary indication of cortisol levels, was not as sensitive as the previously reported LFA (3.5 ng mL−1 (ref. 45)) and would not be able to identify low cortisol concentrations. However, this LFA utilised in-house developed gold labelled antigens, rather than antibodies, reducing manufacture costs. One factor not considered thus far for cortisol detection by LFA is the presence of plasma proteins. In the blood, cortisol is largely bound to plasma proteins cortisol-binding globulin (CBG) and albumin.90 Thus, assay results may be misleading in patients with altered concentrations of these proteins. This should be studied extensively before the assays can be use by patients, since this could impact the validity of results obtained for sick patients.
The process of blood sample collection can lead to elevated cortisol levels due to stress, rendering serum or plasma cortisol LFAs inaccurate. Consequently, measurement of cortisol in saliva has gained considerable attention over the years, given that its concentration correlates well to unbound, serum free cortsiol.45 Salivary cortisol is difficult to measure since it is present in around one tenth of the blood concentration.45 However, it is less invasive which offers a significant advantage. In 2009, the first salivary cortisol LFA was proposed by Yamaguchi et al.80 The device utilised enzyme-labelled glucose oxidase (GOD)-cortisol conjugates which competed with cortisol, thus producing enhanced colour intensity as cortisol concentration decreased (typical competitive process). The LFA was able to accurately detect cortisol in saliva from 1–10 ng mL−1 and was thus able to detect low morning cortisol concentration and covered the normal range at night. Following from this, Yamaguchi et al.83 developed another competitive LFA for the quantitative detection of cortisol in saliva, without the need for sample pre-treatment and with a larger detection range (0.1–10 ng mL−1). The lower LOD meant that diseases such as adrenal insufficiency which led to low cortisol concentrations could be detected, and mildly high cortisol concentrations could be detected if measured at night. The system incorporated a vertical flow component in which the immune reaction occurred and a lateral flow component which worked to remove proteins and unreacted enzyme-labelled conjugate from the sample. However, this LFA took relatively long to complete analysis compared to other LFAs (35 minutes) and the range was still insufficient to cover cortisol excess and deficiency both during the day and at night. Furthermore, the CVs were >10%, indicating poor precision.
Zangheri et al.72 described an LFA strip, housed inside a cartridge, which was adapted as a smartphone accessory to utilise the smartphone camera (Fig. 5a–e). The LFA had a low detection limit of 0.3 ng mL−1 and a large detection range (0.3–60 ng mL−1). Furthermore, its rapid detection and ease of use make it useful for at-home monitoring by patients and the device showed good agreement with conventional ELISA (Fig. 5f). Compared to other methods, however, extra equipment such as 3D printers would be required, making this method relatively expensive. Based on a similar principle, Zangheri et al.74 later developed a biosensor for accurate and rapid monitoring of astronauts' health status during space missions. Importantly, the assay was made to withstand changes in gravity, mechanical stress and bubble formation. The LFA proved to be sensitive when employed on a space mission in Italy, detecting salivary cortisol down to 0.4 ng mL−1. The device only detected the normal range and so further optimisation would be required in order to detect high levels of stress.
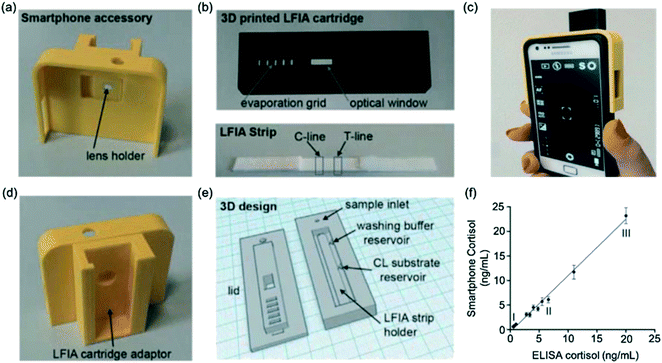 |
| Fig. 5 Smartphone-based LFA for cortisol detection. (a) Image of smartphone accessory with lens holder (b) images of 3D printed LFA cartridge (image above) to house LFA test strip (image below) comprising a test and control line (c) image of phone connected to the smartphone accessory (d) smartphone accessory with adaptor to insert the LFA cartridge for image capture (e) 3D design for LFA cartridge (f) agreement between measurements obtained from gold-standard ELISA and smartphone-assisted LFA. Reproduced with permission.72 Copyright 2015, Elsevier. | |
Gold nanoparticle labels coupled with silver enhancement have also been utilised in the development of a very sensitive cortisol LFA.62 The silver enhancer, previously reported to enhance gold nanoparticle signal,63 was added 2 minutes after the sample. A darker colour formed following enhancement (Fig. 6a–c) which led to a significantly sensitivity achieved (LOD of 0.5 ng mL−1). Furthermore, this LFA had the highest reported detection range of any researched LFA for the detection of cortisol, covering low, normal and high cortisol concentrations. Spike-recovery testing revealed that the matrix did not impact the results obtained. Nonetheless, as with previous cortisol LFA studies, the device was not utilised on patient cohorts with specific illnesses, thus there is a need to test and validate the device for diagnosis of disease. In 2018, Shin et al.73 proposed the smart fatigue phone: an LFA and smartphone-linked fluorescence signal reader for the quantitative detection of salivary cortisol. Since the cortisol would need to be measured below 1 ng mL−1 to detect fatigue, this sensor is highly sensitive (LOD: 0.1 ng mL−1). However, the study included a relatively small sample size, indicating that more research will be needed before the device can be used in POC settings.
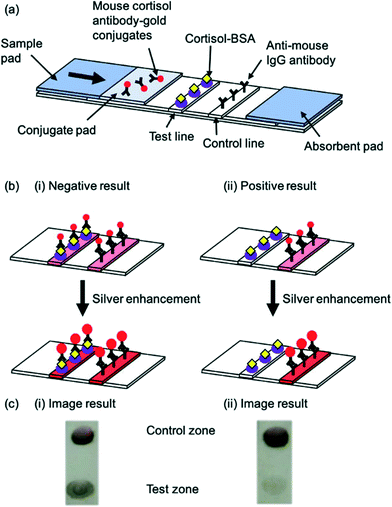 |
| Fig. 6 Schematic illustration of a competitive salivary cortisol LFA utilising silver enhancement. (a) Schematic of the basic LFA strip and components (b) principle of LFA before and after enhancement for (i) negative result and (ii) positive result (c) image result of the test and control lines for (i) negative result and (ii) positive result. Reproduced with permission.62 Copyright 2018, PMC. | |
Competitive LFAs are often limited due to the narrow measurable range, as seen with many of the previously developed cortisol LFAs. To overcome this limitation, Oh et al.77 described a trap lateral flow immunoassay (trapLFI) sensor with deletion and detection zones, instead of the generic LFA format. The detection zone enabled the capture of conjugates. Conjugates not bound to cortisol would be immobilised at the deletion zone. Enzyme-catalysed colour signals at the detection zone would therefore increase with increased cortisol concentration. Thus, the ratio of deletion zone: detection zone signal could be used to detect a large range (0.1–100 ng ml−1) of cortisol with significantly higher sensitivity (9.9 pg mL−1) and specificity than any previously described cortisol LFA. This LFA provided a good correlation with ELISA. TrapLFIs are a relatively novel development renowned for their ultra-sensitivity, due to the decreased deletion zone signal when there is an increase in detection zone signal, and ability to detect proteins with low molecular weights. TrapLFIs also show reduced false positive results since unreacted conjugates must be perfectly trapped in the deletion zone.
Most LFAs employed for the detection of cortisol rely on antibody recognition, as previously described in this review. Recently, aptamers conjugated to gold nanoparticles have been used for the recognition of the hormone in both sweat61 and saliva.60 The advantages of aptamers are that they are highly stable, cheaper and provide an overall simpler handling method than antibodies. Interestingly, these assays covered only the physiological range of cortisol: 8–140 ng mL−1 in sweat and 1–10 ng mL−1 in saliva. To quantify cortisol in deficient of excessive states, a user could dilute the sample appropriately, but this would increase assay duration and complicate its use. Thus, different techniques would be preferred, or further optimisation would be required.
Generally, cortisol is the most widely researched hormone for LFA detection over recent years. During these development stages, several issues have arisen, some of which have been met with novel solutions. Generally, competitive assays yield confusing results: enhanced signal for decreased analyte concentration. This can be confusing, especially for the implementation of devices for patient monitoring at home. New techniques such as trapLFIs improve upon this, enabling simpler results for untrained personnel. Furthermore, a major issue often encountered with competitive assays, and seen with many of the developed cortisol assays, is limited detection ranges. The use of enhancement methods can improve these ranges, as well as trapLFI development. The use of chemiluminescent and fluorescent nanoparticles as well as aptamers has enabled improved sensitivity for cortisol LFAs. Despite extensive research into LFAs for cortisol detection in saliva, as opposed to blood, sweat cortisol LFAs are a novel development, and further research should be undertaken in this field. Furthermore, it is likely that cortisol LFAs will progress to further testing and validation, which has yet to be undertaken for most tests. Pilot studies testing patient samples with specific cortisol-related diseases will validate the devices and provide vital information of their potential use in hospitals.
4.3 Thyroid stimulating hormone (TSH)
In 2012, Kosack et al.84 described a rapid immunochromatographic assay for the detection of TSH. The test had a low LOD (10 mlU L−1) and high sensitivity (100%) proving that this LFA could be useful in the management of thyroid disease. It was found that, whilst correctly identifying all sera with TSH ≥10 mlU L−1, the test lacked sufficient specificity (76.6%). Furthermore, given that the LFA relied on visual inspection, determination of TSH at extremely low concentrations (hyperthyroidism, 0.4 mlU L−1) was found to be inconclusive. Because of this, most TSH LFAs created around this time had mainly been focussed on the detection of hypothyroidism (high TSH levels).
Following from this, You et al.54 created a cell-phone-based TSH LFA using Mie scatter optimisation (Fig. 7a and b). This was the first LFA allowing for quantitative analysis of serum TSH. A much higher sensitivity was achieved (LOD = 0.31 mIU L−1) than in previous studies. Given that the system incorporated the use mobile devices (Fig. 7c and d), which are accessible for most populations around the world, there is a high potential for this device to be distributed and used widely. The error bars for all results obtained for this study were large and/or overlapping, which brings into question the true accuracy of the overall test. Choi et al.65 developed a surface-enhanced Raman scattering (SERS)-based LFA for the measurement of TSH in clinical fluids. Raman reporter-labelled gold nanoparticles were used as SERS nano-tags and the presence of TSH could be observed visually through a colour change at the test line or quantitatively analysed. This was even more sensitive than previously reported LFAs (LOD = 0.025 mlU L−1). However, the study showed considerably higher % CVs for lower TSH concentrations. Utilisation of Mie scatter54 or Raman scatter65 optimisation therefore prove a strong possibility for early hyperthyroidism diagnosis since they are able to detect concentrations of the hormone below 0.5 mlU L−1, although further optimisation of sensitivity may be required to diagnose severe cases.
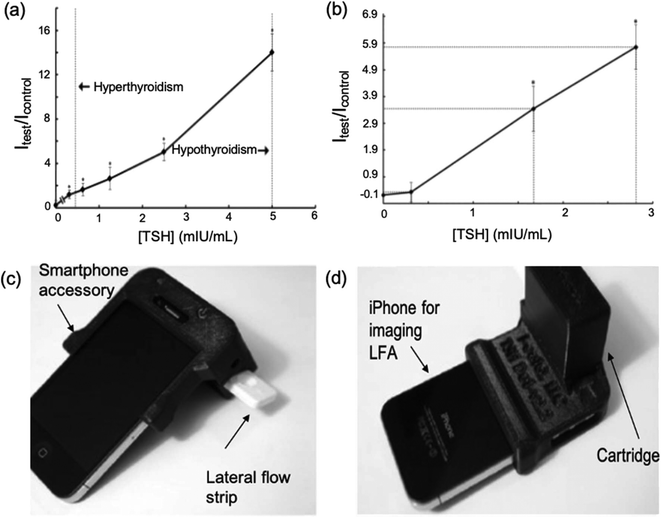 |
| Fig. 7 Cell-phone-based LFA systems for TSH detection. (a) A calibration curve for cell-phone-based TSH detection system developed using standard solutions (b) testing of serum samples using the TSH detection system (c and d) images of iPhone attached to the smartphone accessory which comprises a cartridge. Once the sample has been introduced to the LFA and results have been produced, the lateral flow strip is inserted into the cartridge. This creates a darkbox which allows the iPhone to obtain take an image of the test strip without being affected by external light. The photos can be used to obtain the concentration of TSH in the sample through analysis of the colour formed at the test line. Reproduced with permission.54 Copyright 2013, Elsevier. | |
Since the development of such highly specific TSH LFAs, a variety of nano-labels have been implemented. Preechakasedkit et al.53 synthesised hybrid nanocomposite particles comprising a gold core coated in a europium(III)-chelate fluorophore-doped silica shell. The fluorescence detection system could also be linked to smartphone and digital colour analysis for a lower LOD (0.1 μIU mL−1). More recently, the use of paramagnetic particles68 allowed for rapid (25 min), high-precision quantification of all clinically relevant concentrations of serum TSH with an even lower LOD of 0.017 mlU L−1, pivotal for treatment strategy. These provide examples of the potential for enhancing LFA sensitivity using novel labels, which will likely continue to be implemented in the future as new techniques emerge.
4.4 Parathyroid hormone-like hormone
LFAs have been implemented for detection of some cancer biomarkers, however this remains a field which lacks research. Chammorro-Garcia et al.51 developed a LFIA for the qualitative (by eye) and quantitative (using a simple colorimetric reader) of PTHLH, a hormone involved in the progression of several tumours, in lysates and culture media of different human cell lines. This method provided a cheap and rapid alternative to conventional methods for PTHLH detection, mostly radioimmunoassay (RIA) and immunoradiometric assay (IRMA) which are routinely used for cancer patients. However, the observed LOD was 1.42 ng mL−1. The hormone is typically present in the picomolar range, thus enhanced sensitivity will be a key focus for future development, perhaps utilising different formats or labels as seen with other successfully implemented hormone LFAs.
4.5 Insulin
Rapid glucose monitoring remains one of the most important and useful approaches for the monitoring of diabetes by patients at home. It seems logical that a similar device for the detection of insulin could work hand-in-hand with glucose monitoring in order to provide the best possible patient care. Recently, an LFA was described for the measurement of insulin and C-peptide for use in evaluating insulin resistance indices among diabetic patients. The SelexOn48 system by Osang Healthcare Inc. comprised a cartridge which detected the analytes in a sandwich immunoassay using capture antibodies conjugated with gold nanoparticles. The device contained a camera which detected the intensity of the gold nanoparticles allowing for densitometric analysis. However, during insulin resistance the body produces more insulin. The 2-hour postprandial insulin levels can rise to 1153 pmol L−1. The range for this LFA was found to be 218.1–275.72 pmol L−1. Thus, the test strips must be further optimised to assess larger ranges before being employed for clinical use. As well as sensitivity, was another issue encountered during the development of this assay was that the population consisted disproportionately of post-menopausal women. This would have affected the results obtained, especially the cut-off values, emphasising the importance of test standardisation.
4.6 Vitamin D
Vitamin D is difficult to measure due to the need for complex sample preparation including separation of the marker from its binding protein. In 2017, Vemulapati et al.66 described a rapid LFA for the detection of 25(OH)D3 from finger prick samples, in which no sample preparation was required. This was a competitive assay since 25(OH)D3 has a small size of approximately 350 Da. The test strips were used in conjunction with a smartphone-assisted portable imaging device which allowed for quantitation which was highly accurate (90.5%). This test allowed for reliable quantification of the hormone levels across the entire physiologically relevant range (0–150 nmol L−1). At higher concentrations, the % CVs were >10%, thus precision could be an issue for the device. In the future, novel methods could be utilised to further improve this test.
5. Commercialisation of hormone LFAs
LFAs for hormone detection are produced by several manufacturers globally. The move towards POC testing is desirable not only among clinicians and patients due its rapid results (reduction of approximately 46 minutes per patient91) and ease of use, but also because it has been estimated that the total savings due to decreased waiting time for results can be as high as €148 per patient.91 Thus, much effort has been made to research and manufacture novel LFAs (Fig. 8). For the detection of hormones, rapid testing will enable self-monitoring of disease for patients at home, further reducing costs and psychological impacts of disease.
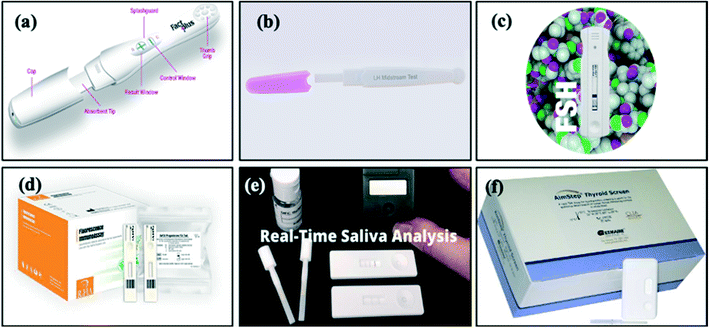 |
| Fig. 8 Images of commercially available LFAs: (a) FactPlus hCG pregnancy test,92 (b) BTNX LH midstream test,120 (c) Alfa Scientific Designs Instant-View FSH urine test,126 (d) CTK Biotech Prolactin,25 progesterone,130 testosterone,35 T3 (ref. 136) and T4 (ref. 137) FIA test, (e) SOMA Bioscience cortisol lateral flow device131 and (f) Germaine Laboratories rapid test kit AimStep thyroid screen TSH test.133 | |
We have summarised the key manufacturers of different hormone LFAs which can be used in clinics or by patients at home (Table 5). As shown in Table 5, the majority of LFAs that have been documented in research, such as hCG, LH and cortisol, are available commercially. The most commonly available LFAs are pregnancy tests. Despite being well-researched, very few cortisol LFAs exist commercially. This could be due to the fact that cortisol was only recently implicated as a biomarker for stress. Despite lacking research advances, T3 and T4 LFAs are available commercially. These could aid in diagnosis and monitoring of thyroid disease (alongside TSH LFAs) and be used to monitor thyroid replacement medication. Similarly, FSH and prolactin LFAs are commercially available, but research in the future to investigate alternative methods to enhance sensitivity could be useful, as well as testing of the devices in clinical settings.
Table 5 Analytical performance of commercially available hormone LFAs
Hormone |
Sample/matrix |
Company/product name |
LOD |
Sensitivity |
Specificity |
Ref. |
Beta-hCG |
Urine |
Fact Plus by Swiss Precision Diagnostics |
25 mIU mL−1 |
— |
— |
92
|
Clear Blue by Swiss Precision Diagnostics |
10 mIU mL−1 |
— |
— |
93
|
Abbott Alere |
25 mlU mL−1 |
>99% |
100% |
94
|
Siemens Clinitest |
At least 25 mlU mL−1 |
>99% |
— |
95
|
Stanbio QuPID |
20 mlU mL−1 for urine |
99% |
99% |
96
|
Quidel Sofia FIA |
20 mlU mL−1 |
>99% |
>99% |
97
|
Germaine Laboratories AimStrip |
20 mlU mL−1 |
— |
— |
98
|
Sekisui Diagnostics OSOM |
25 mlU mL−1 |
100% |
100% |
99
|
Clarity |
25 mlU mL−1 |
99% |
100% |
100
|
Consult diagnostics McKesson h |
20 mlU mL−1 |
>99% |
No cross-reactivity with LH, FSH or TSH |
101
|
CLIAwaived, Inc. |
25 mlU mL−1 |
— |
— |
102
|
BTNX Rapid Response |
25 mlU mL−1 |
>99.9% |
— |
103
|
SA Scientific |
25 mlU mL−1 |
>99% |
>99% |
104
|
Instant-View |
25 mlU mL−1 |
>99% |
>99% |
105
|
Urine or plasma |
Microgen Bioproducts |
Urine test strip: 25 mlU mL−1 |
— |
99.9% |
106
|
Urine/serum/plasma cassette: 10 mlU mL−1 |
Urine or serum |
Fisher HealthCare Sure-Vue |
Urine – 20 mlU mL−1 |
|
No cross-reactivity with LH, FSH or TSH |
107
|
Serum – 10 mlU mL−1 |
Meridian Bioscience ImmunoCard STAT! |
Urine – 20 mlU mL−1 |
>99% |
No cross-reactivity with LH, FSH or TSH |
108
|
Serum – 10 mlU mL−1 |
Polymedco Poly stat |
20 mlU mL−1 |
100% |
100% |
109
|
HemoCue America Beckman Coulter ICON 25 |
25 mlU mL−1 |
— |
>99% |
110
|
SA Scientific |
Urine – 20 mlU mL−1 |
>99% |
>99% no cross-reactivity with LH, TSH or FSH |
111
|
Serum – 10 mlU mL−1 |
Quidel one-step |
Urine – 20 mlU mL−1 |
>99% |
>99% |
112
|
Serum – 10 mlU mL−1 |
Sekisui Diagnostics OSOM |
25 mlU mL−1 |
100% urine |
100% urine |
113
|
99.5% serum |
99% serum |
Cen-Med Enterprises |
20 mlU mL−1 |
— |
— |
114
|
Germaine Laboratories AimStep |
20 mlU mL−1 |
— |
— |
115
|
Clarity |
25 mlU mL−1 |
99% |
100% |
116
|
Instant-View |
25 mlU mL−1 |
>99% |
>99% |
117
|
Whole blood, plasma or serum |
NOW Diagnostics ADEXUSDx |
10 mlU mL−1 |
>99% |
>99% |
118
|
Urine, serum, plasma or whole blood |
Veda Lab |
10 mIU mL−1 |
— |
— |
119
|
LH |
Urine |
BTNX |
— |
98.7% |
— |
120
|
Joysbio |
— |
— |
— |
121
|
Instant-View |
20 mlU mL−1 |
>99% |
— |
122
|
SA Scientific |
25 mlU mL−1 |
— |
— |
123
|
Germaine Aimstep |
40 mlU mL−1 |
— |
— |
124
|
Serum |
CTK Biotech FIA |
— |
— |
— |
125
|
Serum, plasma or whole blood |
Veda Lab |
— |
— |
— |
119
|
LH and FSH |
Urine |
Concile CrinoCheck |
— |
— |
— |
36
|
FSH |
Urine |
Instant-View |
40 mlU mL−1 |
>99% |
— |
126
|
SA Scientific |
25 mlU mL−1 |
— |
No cross-reactivity |
127
|
BTNX Rapid Response |
25 mlU mL−1 |
99% |
— |
128
|
Serum |
CTK Biotech FIA |
— |
— |
— |
129
|
Serum, plasma or whole blood |
Veda Lab |
— |
— |
— |
119
|
Prolactin |
Serum |
CTK Biotech FIA |
— |
— |
— |
25
|
Serum, plasma or whole blood |
Veda Lab |
— |
— |
— |
119
|
Progesterone |
— |
CTK Biotech FIA |
— |
— |
— |
130
|
Testosterone |
— |
CTK Biotech FIA |
— |
— |
— |
35
|
Concile CrinoCheck |
— |
— |
— |
36
|
Cortisol |
Saliva |
SOMA |
0.55 nM |
— |
— |
131
|
Whole blood, serum or plasma |
Veda Lab cortisol |
— |
— |
— |
119
|
TSH |
Whole blood |
Jant Pharmacal Corporation Accutest |
— |
— |
— |
132
|
Germaine Laboratories Aimstep |
— |
— |
— |
133
|
BTNX Rapid Response |
— |
— |
— |
134
|
Serum or plasma |
CTK Biotech FIA |
— |
— |
— |
135
|
Serum, plasma or whole blood |
Veda Lab |
— |
— |
— |
119
|
N/A |
Concile CrinoCheck |
— |
— |
— |
36
|
T3 |
Serum or plasma |
Veda Lab |
— |
— |
— |
119
|
N/A |
CTK Biotech FIA |
— |
— |
— |
136
|
T4 |
Serum or plasma |
Veda Lab |
— |
— |
— |
119
|
N/A |
CTK Biotech FIA |
— |
— |
— |
137
|
Vitamin D |
Serum |
Quidel Sofia FIA |
5.2 ng mL−1 |
— |
Some cross-reactivity |
138
|
N/A |
Concile CrinoCheck |
— |
— |
— |
36
|
5.1 Pregnancy and fertility
Today, rapid home pregnancy tests are the most popular and widely available lateral flow test. These are available commercially in many supermarkets and online stores, typically costing between £1 to £30 per test. LFAs for hCG detection have mainly been developed for urine, although those for serum, plasma and whole blood are also available, although they tend to have a slightly longer detection time of 5 minutes. Most pregnancy tests today have a sensitivity and specificity of over 99%. The LOD of the tests varies between companies, normally 20 mlU mL−1 or 25 mlU mL−1 and thus capable for detecting pregnancy from the day of the expected period. Interestingly, the hCG urine LFA produced by Veda Lab139 has an exceptionally low sensitivity of 10 mlU mL−1, often able to detect pregnancy up to 4 days prior to the expected period. Given that LH is structurally very similar to hCG, LH LFAs became available soon after pregnancy tests and are currently produced by a number of manufacturers including Germaine,124 SA Scientific123 and BTNX.120 To aid in ease of use, commercially available LFAs for the determination of LH produce qualitative results from urine samples121,124 in which the LH surge can be detected non-invasively. The lowest detection limit for urinary LH LFAs is 20 mIU mL−1 (ref. 122) with a 99% sensitivity level. Few LFAs exist for serum detection of LH, although those available enable quantitative detection of LH119,125 and therefore may be more widely used in clinical rather than home settings.
Urine FSH LFAs have also been commercialised, although these are not used widely in routine settings.126–129 LFAs also exist commercially for the detection of FSH in serum, whole blood and plasma.119 Some FSH tests allow for accurate, quantitative detection of the hormone36,119,129 which could be linked to a computer software for monitoring of patient fertility by gynaecologists. The availability of an LFA which is able to simultaneously detect LH and FSH from one sample is interesting, as it can be used at home and in clinics for detection of abnormal oestrous cycle, PCO-Syndrome, infertility and menopause.36 Similarly, few commercial LFAs exist for the detection of prolactin.25,119 Manufacturers have developed an FIA for quantitative serum prolactin detection within 20 minutes.25 This LFA offers an interestingly large detection range of 5–4000 mIU mL−1 and is thus able to detect elevated prolactin concentrations for the potential diagnosis of tumours and other diseases.25 Another similar device also detects the hormone in serum, as well plasma or whole blood, thus giving it a wider variety of applications and it is has a shorter assay time, thus producing accurate results more rapidly with a range of 20–350 ng mL−1, which is also able to detect elevated prolactin concentrations (>25 ng mL−1).119 The same manufacturers have developed a progesterone FIA.130 The test is quantitative, has a detection range of 0.3–80 ng mL−1 which enables the detection of both low and high concentrations of this hormone. This test was found to have low cross-reactivity (<0.3 ng mL−1) when tested with several potentially interfering hormones, including oestradiol, estrone and testosterone, which is extremely important. For testosterone, only a few LFAs have been developed.35,36 The widest detection range for a commercial testosterone LFA is 0.2–20 ng mL−1.35 Therefore, the device can be employed in clinics for rapid detection of infertility in men (low levels), polycystic ovarian syndrome in women (slightly elevated levels) or androgen-secreting tumours (extremely elevated concentrations). However, no simple qualitative LFA exist for testosterone measurement, which would facilitate the use of these commercially available devices for use by patients at home.
5.2 Cortisol
LFAs for cortisol detection are a recent development, and few have been brought to the market. SOMA Bioscience recently developed a cortisol LFA for the quantitation of salivary cortisol (taken using a mouth swab).131 The test takes only 10 minutes to produce results which were tested against ELISA in the range 1.25–40 mM. A lateral flow device (LFD) reader is required to obtain these results, thus increasing the cost and complexity of use. Another device is also commercially available, however for the detection of cortisol serum, plasma or whole blood (detection range: 25–250 ng mL−1).119 This LFA should be able to detect elevated concentrations of cortisol in the evening, and thus could aid in the diagnosis of diseases such as Cushing's or could indicate the presence of an adrenal carcinoma. These are currently the only commercially available cortisol LFAs.
5.3 Thyroid function
Commercially, there are many LFAs for thyroid function monitoring. Qualitative TSH LFAs132–134 produce visual results within 5–10 minutes whilst quantitative tests36,119,135 take between 15 and 20 minutes. One kit that is available is an FIA with a larges detection range of 0.4–100 μIU mL−1.135 Patients with hyperthyroidism have TSH levels of 0.4 μlU mL−1 or lower. Only two of the available commercial kits are sensitive enough to detect hyperthyroidism (LODs 0.3 (ref. 36 and 0.2 (ref. 119)). The LFAs available are likely to be more useful for the diagnosis of hypothyroidism. Several T3 and T4 LFAs are also available (ranges of 0.5–10 nmol L−1 (ref. 136) for T3 and 0.75–15 (ref. 119) or 10–320 nmol L−1 for T4 (ref. 137)). It should be noted that T3 and T4 are structurally very similar which is an issue for specificity of the tests.
5.4 Vitamins
There are a few commercially available vitamin D LFAs which have the purpose of detecting vitamin D deficiency. The assays produce results within 10 minutes (ref. 138) the range of 3–100 ng mL−1.36 The low LOD is useful as the device is able to detect more extreme deficiency in vitamin D, and could be potentially used to monitor supplementation, for appropriate dosing.
6. Future perspectives
Evidence from the literature suggests that several challenges remain in hormone detection by LFA, despite extensive research into this field, and this could explain the lack of commercialisation and more importantly the fact that, to this day, LFAs are not gold standard diagnostic techniques, except for pregnancy detection. Generally, hCG LFAs have been well researched, optimised and tested, leading to the development of several commercial products with high sensitivity, specificity, and precision. Current hCG LFAs show suitable detection ranges and are able to detect pregnancy at different weeks. The development of digital hCG LFAs has been more recent, but it well documented. It can be assumed that the future move for modern hCG assays will be incorporation of newly discovered nanoparticles, such as quantum dots, for further enhanced sensitivity. Moreover, several diseases may induce excessive hCG secretion, such as gestational trophoblastic disease or pituitary disease. Thus, multiplexing of LFAs in the future will enable patients and clinicians to differentiate between pregnancy and illness at POC. For many other fertility hormones, further tests are required. Many studies do not investigate parameters such as selectivity or test stability and have not been optimised in terms of sensitivity. In the future, several steps will likely be taken to enhance these methods. Firstly, the use of aptamers instead of antibodies as bioreceptors leads to significant improvements in test selectivity. This has been implemented for progesterone46 and cortisol61,140 but remains a new area for research for other hormones. Furthermore, many studies have only focussed on optimisation for clinical fluids; this is an issue since different matrices can have interfering substances. Thus, in the future, these studies will be important before bringing the devices to industry. Further, it is likely that in the future, there will be a move away from testing blood samples, and towards biofluids with less invasive collection protocols such as saliva or sweat. Hormones are relatively small molecules, and this many hormones such as oestrogen, testosterone and cortisol rely on competitive LFA formats for detection. Competitive assays yield the typical competitive inhibitor results: decreased signal intensity with increased analyte concentration. This can often be confusing for the reader This can be overcome by incorporating new formats such as trapLFIs, which produce signals directly proportional to the analyte concentration. It is likely that new methods to detect haptens in this way will be developed in the future. Also, competitive assays are often limited by small detection ranges, and thus the LFAs developed are unable to detect deficiency or excess of the hormones.
Another issue with hormone detection is developing devices which are sensitive enough for their detection. From the literature, it is clear that the implementation of carbon nanoparticles, fluorescent nanoparticles and magnetic nanoparticles cause a great improvement in test sensitivity. Recently, fluorescent labels have gained interest for enhanced sensitivity. In particular, quantum dots can be implemented for 500× increased sensitivity.141 However, as seen with hormone LFAs, enhanced sensitivity often comes at the cost of lengthier analysis times or the requirement for expensive strip readers. Traditional colorimetric techniques, which have been most widely implemented for hormone LFAs, require no extra equipment. Thus, the future move will be to develop and implement methods which can allow simple, rapid and affordable POC diagnosis. One major issue currently faced is difficulty in multiplexing LFAs. Few hormones have been researched in multiplexed format, and no commercial multiplexed hormone LFAs exist. Given the rise in interest in personalised medicine and targeted therapy, multiplexed devices have gained widespread interest. Multiplexed LFAs can enable accurate diagnosis of disease since they test for multiple biomarkers at once. It is likely that in the near future, multiplexed LFAs will become commercially available for hormone LFAs. An undeniable trend is the move towards digitalised LFAs, for examples smartphone-readout systems. This has been developed for fertility hormones such as hCG and LH, to track fertility cycles and to identify ovulation (fertile periods), however is will likely be incorporated for other hormones for disease monitoring by patients at home. It is also likely that we will begin to see more studies investigating the use of commercial LFAs for disease diagnosis, monitoring, and prognostication, including pilot studies on diseased cohorts.
7. Conclusion
LFAs have been extensively research and commercially produced over the last 3 decades. They are rapid and cheap portable devices which have enabled timely diagnosis and monitoring of disease.44 Monitoring of hormones has been a key part of patient care within the endocrine world. Excess or deficiency of hormones can be indicative of a plethora of diseases. For this reason, the detection of hormones has gained widespread interest and the NHS is investing heavily into this sector. LFAs have been developed for the detection of some hormones. Most LFAs utilise highly specific antibodies labelled with coloured nanoparticles, which bind to the analyte and immobilise the analyte in the sample, thus producing colour on the test strip. Most hormones can be detected using sandwich LFAs, such as hCG and LH, while others must utilise competitive formats as the molecules are too to be sandwiched between antibodies, such as cortisol. Many LFAs have been made with high sensitivity (>95%) and specificity (>95%) for the diagnosis of endocrine diseases. For many hormones, such as hCG and LH, these have proceeding past the research phase and are widely available commercially. Several methods for LFA enhancement have been trialled such as the use of superparamagnetic and gold nanoparticle enhancement techniques. This has allowed for improved sensitivity. In the laboratory, many LFAs have been linked to smartphone-readout systems;72,73,86,88,89 however, these are not yet used routinely by patients. The future move toward read-out systems and the use of digital devices will enable tracking of patient health at home by clinicians, as the systems can be linked to existing healthcare systems in the hospital. However, while LFAs have been produced in the lab for other hormones, they are yet to be commercially available such as insulin and PTHLH.
The development of more LFAs in the future will enable more rapid diagnosis of disease, as well as efficient patient monitoring. One of the key goals of the NHS long-term plan is to improve diagnosis of disease and develop rapid, point-of-care devices for disease diagnosis. In particular, the long-term plan for diabetes is to develop digital self-management support tools.142 The commercialisation of insulin LFAs,48 and potential linking to a smartphone-readout system, would help to meet these targets and therefore reduce the burden of diabetes on the NHS and worldwide, due to earlier detection of complications in patients through continuous simple monitoring. Furthermore, much research exists into LFAs for fertility and stress hormones. However, there is clear lack of research into others such as hypothalamic hormones, for examples gonadotrophin-releasing hormone and somatostatin, and pancreatic hormones. This suggests there is room for further future development. Moreover, multiplexed LFAs have been developed only for the detection of hCG and testosterone. Multiplexing is a newly emerging field which is of great interest currently within the medical world. Future research into hormone LFAs should focus on multiplexed hormone sensing, for the purpose of differential disease diagnosis at POC.
Conflicts of interest
The authors declare no competing financial interest.
References
-
K. Chou and J. Henderson, Endocrine system, Encyclopedia of Toxicology, 2nd edn, 2005, pp. 170–174 Search PubMed.
- S. Hiller-Sturmhöfel and A. Bartke, The endocrine system: an overview, Alcohol Health Res. World, 1998, 22(3), 153 Search PubMed.
-
Markets, F.
Global Endocrine Testing Market Is Expected to Reach USD 15.69 Billion by 2027, Fior Markets, https://www.globenewswire.com/news-release/2020/09/10/2091386/0/en/Global-Endocrine-Testing-Market-Is-Expected-to-Reach-USD-15-69-Billion-by-2027-Fior-Markets.html Search PubMed.
-
Research, G. V., Endocrine Testing Market Size, Share & Trends Analysis Report By Test Type, By Technology (Tandem Mass Spectrometry, Immunoassay), By End-use And Segment Forecasts, 2018–2024, https://www.grandviewresearch.com/industry-analysis/endocrine-testing-market Search PubMed.
-
Intelligence, M., Endocrine Testing Market – Growth, Trends, COVID-19 Impact, and Forecase (2021–2026), https://www.mordorintelligence.com/industry-reports/endocrine-testing-market Search PubMed.
- K. M. Koczula and A. Gallotta, Lateral flow assays, Essays Biochem., 2016, 60(1), 111–120 CrossRef PubMed.
-
T. Atlantic, Before There Were Hormone Pregnancy Tests, https://www.theatlantic.com/health/archive/2015/06/history-home-pregnancy-test/396077/ Search PubMed.
-
Laboratory, E. C., hCG (Human Chorionic Gonadotrophin), https://www.exeterlaboratory.com/test/hcg-human-chorionic-gonadotrophin/ Search PubMed.
-
D. Nedresky and G. Singh, Physiology, luteinizing hormone, 2019 Search PubMed.
- K. Tanabe, N. Susumu, K. Hand, K. Nishii, I. Ishikawa and S. Nozawa, Prediction of the potentially fertile period by urinary hormone measurements using a new home-use monitor: comparison with laboratory hormone analyses, Hum. Reprod., 2001, 16(8), 1619–1624 CrossRef CAS PubMed.
-
M. E. Ellis, Luteinizing Hormone (LH) Test: What It Is and Why It's Important, https://www.healthline.com/health/lh-blood-test Search PubMed.
- H. M. Behre, J. Kuhlage, C. Gaβner, B. Sonntag, C. Schem, H. P. Schneider and E. Nieschlag, Prediction of ovulation by urinary hormone measurements with the home use ClearPlan® Fertility Monitor: comparison with transvaginal ultrasound scans and serum hormone measurements, Hum. Reprod., 2000, 15(12), 2478–2482 CrossRef CAS PubMed.
-
M. Sinai, Follicle-stimulating hormone (FSH) blood test, https://www.mountsinai.org/health-library/tests/follicle-stimulating-hormone-fsh-blood-test#:~:text=Before%20puberty%20%2D%200%20to%204.0,25.8%20to%20134.8%20IU%2FL Search PubMed.
-
M. Eng, Estrone Test: High & Low Levels + Normal Range, https://labs.selfdecode.com/blog/estrone-test/ Search PubMed.
-
W. Stillwell, An introduction to biological membranes: composition, structure and function, Elsevier, 2016 Search PubMed.
-
J. Martel, Estradiol Test, https://www.healthline.com/health/estradiol-test Search PubMed.
-
B. Novkovic, Estriol Blood Test: High & Low Levels + Normal Range, https://labs.selfdecode.com/blog/estriol-blood-test-high-low-levels-normal-range/ Search PubMed.
-
S. Hale, LH Levels: Understanding Normal Ranges and The Benefit of Testing, https://www.miracare.com/blog/lh-levels/ Search PubMed.
- K. Henderson and J. Stewart, A dipstick immunoassay to rapidly measure serum oestrone sulfate concentrations in horses, Reprod., Fertil. Dev., 2000, 12(4), 183–189 CrossRef CAS PubMed.
-
G. W. Almond, Diagnosis of pregnancy, Current Therapy in Large Animal Theriogenology, 2007, pp. 765–773 Search PubMed.
-
Healthline Serum Progesterone Test: Purpose, Results, and Risks, https://www.healthline.com/health/serum-progesterone Search PubMed.
- A. A. Zaied, C. J. Bierschwal, R. G. Elmore, R. S. Youngquist, A. J. Sharp and H. A. Garverick, Concentrations of progesterone in milk as a monitor of early pregnancy diagnosis in dairy cows, Theriogenology, 1979, 12(1), 3–11 CrossRef CAS PubMed.
-
T. Rehagen, Keep Testosterone In Balance, https://www.webmd.com/men/features/keep-testosterone-in-balance#:~:text=Testosterone%20levels%20are%20measured%20through,t%20enough%20to%20warrant%20alarm Search PubMed.
-
Health, U., Prolactin blood test, https://www.ucsfhealth.org/medical-tests/prolactin-blood-test#:~:text=The%20normal%20values%20for%20prolactin,80%<?pdb_no 20to?>20to<?pdb END?>%20400%20%C2%B5g%2FL Search PubMed.
-
Biotech, C., PRL FIA Test, https://ctkbiotech.com/product/prl-fia-test/ Search PubMed.
-
G. T. Griffing, Serum cortisol, https://ctkbiotech.com/product/prl-fia-test/ Search PubMed.
-
Laboratory, E. C., Cortisol (Urine), https://exeterlaboratory.com/test/cortisol-urine/ Search PubMed.
- P. Pearlmutter, G. DeRose, C. Samson, N. Linehan, Y. Cen, L. Begdache, D. Won and A. Koh, Sweat and saliva cortisol response to stress and nutrition factors, Sci. Rep., 2020, 10(1), 19050 CrossRef CAS PubMed.
-
S. Buppajarntham, Insulin, https://emedicine.medscape.com/article/2089224-overview Search PubMed.
-
Pharmacy, U., Vitamin D and Chronic Pain: Promising Correlates, https://www.uspharmacist.com/article/vitamin-d-and-chronic-pain-promising-correlates Search PubMed.
-
Clinic, C., Thyroid Blood Tests, https://my.clevelandclinic.org/health/diagnostics/17556-thyroid-blood-tests Search PubMed.
-
L. M. Basile, What are T3, T4, and TSH, https://www.endocrineweb.com/thyroid-what-are-t3-t4-tsh#:~:text=A%20normal%20T3%20level%20might,deciliter%20(ng%2FdL) Search PubMed.
- H. Suwa, M. Hirano, K. Kawarada, M. Nagayama, M. Ehara, T. Muraki, H. Shisa, A. Sugiyama, M. Sugimoto, H. Hiai, M. Kitano and J. Tanuma, Pthlh, a promising cancer modifier gene in rat tongue carcinogenesis, Oncol. Rep., 2014, 31(1), 3–12 CrossRef CAS PubMed.
- G. A. Posthuma-Trumpie, J. Korf and A. van Amerongen, Development of a competitive lateral flow immunoassay for progesterone: influence of coating conjugates and buffer components, Anal. Bioanal. Chem., 2008, 392(6), 1215–1223 CrossRef CAS PubMed.
-
Biotech, C., Testosterone FIA Test, https://ctkbiotech.com/product/testosterone-fia-test/ Search PubMed.
-
Concile Quantitative Rapid Tests, https://www.concile.de/en/produkte/quantitative-rapid-tests/ Search PubMed.
-
L. Thau and S. Sharma, Physiology, cortisol, StatPearls, 2020 Search PubMed.
-
M. A. Shahid, M. A. Ashraf and S. Sharma, Physiology, thyroid hormone, 2018 Search PubMed.
- M. Yao, T. Murakami, K. Shioi, N. Mizuno, H. Ito, K. Kondo, H. Hasumi, F. Sano, K. Makiyama, N. Nakaigawa, T. Kishida, Y. Nagashima, S. Yamanaka and Y. Kubota, Tumor signatures of PTHLH overexpression, high serum calcium, and poor prognosis were observed exclusively in clear cell but not non clear cell renal carcinomas, Cancer Med., 2014, 3(4), 845–854 CrossRef CAS PubMed.
-
M. Wallace, Measurement of Hormones, Oxford University Press, 3rd edn, 2011 Search PubMed.
- J. Pum, A practical guide to validation and verification of analytical methods in the clinical laboratory, Adv. Clin. Chem., 2019, 90, 215–281 CAS.
- C. Parolo, A. Sena-Torralba, J. F. Bergua, E. Calucho, C. Fuentes-Chust, L. Hu, L. Rivas, R. Álvarez-Diduk, E. P. Nguyen and S. Cinti, Tutorial: design and fabrication of nanoparticle-based lateral-flow immunoassays, Nat. Protoc., 2020, 15(12), 3788–3816 CrossRef CAS PubMed.
-
Research, G. V., Lateral Flow Assay Market Size, Share & Trends Analysis Report By Product (Kits & Reagents, Lateral Flow Readers), By End-use, By Application (Clinical Testing, Veterinary Diagnostics), By Technique, By Region, And Segment Forecasts, 2019–2026, https://www.grandviewresearch.com/industry-analysis/lateral-flow-assay-market Search PubMed.
- E. B. Bahadır and M. K. Sezgintürk, Lateral flow assays: Principles, designs and labels, TrAC, Trends Anal. Chem., 2016, 82, 286–306 CrossRef.
- W. Leung, P. Chan, F. Bosgoed, K. Lehmann, I. Renneberg, M. Lehmann and R. Renneberg, One-step quantitative cortisol dipstick with proportional reading, J. Immunol. Methods, 2003, 281(1–2), 109–118 CrossRef CAS PubMed.
- R. Tanaka, T. Yuhi, N. Nagatani, T. Endo, K. Kerman, Y. Takamura and E. Tamiya, A novel enhancement assay for immunochromatographic test strips using gold nanoparticles, Anal. Bioanal. Chem., 2006, 385(8), 1414–1420 CrossRef CAS PubMed.
- M. P. Laitinen and M. Vuento, Immunochromatographic assay for quantitation of milk progesterone, Acta Chem. Scand., 1996, 50(2), 141–145 CrossRef CAS PubMed.
- D. W. Jekarl, H. Choi, E. S. Kim, S. Lee, H.-I. Park, M. Kim and Y. Kim, Analytical evaluation and clinical application of insulin and C-peptide by a whole blood, lateral flow, point of care (POC) assay system, Scand. J. Clin. Lab. Invest., 2019, 79(5), 347–353 CrossRef CAS PubMed.
- M. N. Alnajrani and O. A. Alsager, Lateral flow aptasensor for progesterone: Competitive target recognition and displacement of short complementary sequences, Anal. Biochem., 2019, 587, 113461 CrossRef CAS PubMed.
- V. Nayan, E. S. Sinha, S. K. Onteru and D. Singh, A proof-of-concept of lateral flow based luteinizing hormone detection in urine for ovulation prediction in buffaloes, Anal. Methods, 2020, 12(26), 3411–3424 RSC.
- A. Chamorro-Garcia, A. De La Escosura-Muñiz, M. Espinoza-Castañeda, C. J. Rodriguez-Hernandez, C. De Torres and A. Merkoçi, Detection of parathyroid hormone-like hormone in cancer cell cultures by gold nanoparticle-based lateral flow immunoassays, Nanomed.: Nanotechnol., Biol. Med., 2016, 12(1), 53–61 CrossRef CAS PubMed.
- C. Xing, L. Liu, S. Song, M. Feng, H. Kuang and C. Xu, Ultrasensitive immunochromatographic assay for the simultaneous detection of five chemicals in drinking water, Biosens. Bioelectron., 2015, 66, 445–453 CrossRef CAS PubMed.
- P. Preechakasedkit, K. Osada, Y. Katayama, N. Ruecha, K. Suzuki, O. Chailapakul and D. Citterio, Gold nanoparticle core–europium (iii) chelate fluorophore-doped silica shell hybrid nanocomposites for the lateral flow immunoassay of human thyroid stimulating hormone with a dual signal readout, Analyst, 2018, 143(2), 564–570 RSC.
- D. J. You, T. San Park and J.-Y. Yoon, Cell-phone-based measurement of TSH using Mie scatter optimized lateral flow assays, Biosens. Bioelectron., 2013, 40(1), 180–185 CrossRef CAS PubMed.
- V. Tripathi, S. Nara, K. Singh, H. Singh and T. G. Shrivastav, A competitive immunochromatographic strip assay for 17-α-hydroxy progesterone using colloidal gold nanoparticles, Clin. Chim. Acta, 2012, 413(1–2), 262–268 CrossRef CAS PubMed.
- F. Di Nardo, L. Anfossi, L. Ozella, A. Saccani, C. Giovannoli, G. Spano and C. Baggiani, Validation of a qualitative immunochromatographic test for the noninvasive assessment of stress in dogs, J. Chromatogr., B, 2016, 1028, 192–198 CrossRef CAS PubMed.
- Z. Wang, L. Guo, L. Liu, H. Kuang and C. Xu, Colloidal gold-based immunochromatographic strip assay for the rapid detection of three natural estrogens in milk, Food Chem., 2018, 259, 122–129 CrossRef CAS PubMed.
- J. Liu, Z. Kong, Y. Wang, Y. Fan, J. Luo, S. Xu, H. Jin and X. Cai, A rapid quantitative determination method of luteinizing hormone with gold immunochromatographic strip, Annu. Int. Conf. IEEE Eng. Med. Biol. Soc., 2017, 17–20 CAS.
- S. Nara, V. Tripathi, H. Singh and T. G. Shrivastav, Colloidal gold probe based rapid immunochromatographic strip assay for cortisol, Anal. Chim. Acta, 2010, 682(1–2), 66–71 CrossRef CAS PubMed.
- S. Dalirirad, D. Han and A. J. Steckl, Aptamer-Based Lateral Flow Biosensor for Rapid Detection of Salivary Cortisol, ACS Omega, 2020, 5(51), 32890–32898 CrossRef CAS PubMed.
- S. Dalirirad and A. J. Steckl, Aptamer-based lateral flow assay for point of care cortisol detection in sweat, Sens. Actuators, B, 2019, 283, 79–86 CrossRef CAS.
- A. Apilux, S. Rengpipat, W. Suwanjang and O. Chailapakul, Development of competitive lateral flow immunoassay coupled with silver enhancement for simple and sensitive salivary cortisol detection, EXCLI J., 2018, 17, 1198 Search PubMed.
- M. O. Rodríguez, L. B. Covián, A. C. García and M. C. Blanco-López, Silver and gold enhancement methods for lateral flow immunoassays, Talanta, 2016, 148, 272–278 CrossRef PubMed.
- C. Kim, Y. K. Yoo, S. I. Han, J. Lee, D. Lee, K. Lee, K. S. Hwang, K. H. Lee, S. Chung and J. H. Lee, Battery operated preconcentration-assisted lateral flow assay, Lab Chip, 2017, 17(14), 2451–2458 RSC.
- S. Choi, J. Hwang, S. Lee, D. W. Lim, H. Joo and J. Choo, Quantitative analysis of thyroid-stimulating hormone (TSH) using SERS-based lateral flow immunoassay, Sens. Actuators, B, 2017, 240, 358–364 CrossRef CAS.
- S. Vemulapati, E. Rey, D. O'dell, S. Mehta and D. Erickson, A quantitative point-of-need assay for the assessment of vitamin D 3 deficiency, Sci. Rep., 2017, 7(1), 1–8 CrossRef CAS PubMed.
- X.-D. Yang, F.-Y. Wang, C.-M. Song, S.-Y. Wu, G.-P. Zhang and X.-Y. Zeng, Establishment of a lateral flow colloidal gold immunoassay strip for the rapid detection of estradiol in milk samples, LWT–Food Sci. Technol., 2015, 64(1), 88–94 CrossRef CAS.
- S. L. Znoyko, A. V. Orlov, V. A. Bragina, M. P. Nikitin and P. I. Nikitin, Nanomagnetic lateral flow assay for high-precision quantification of diagnostically relevant concentrations of serum TSH, Talanta, 2020, 216, 120961 CrossRef CAS PubMed.
- C. Wang, D. Guan, C. Chen, S. He, X. Liu, C. Wang and H. Wu, Rapid detection of unconjugated estriol in the serum via superparamagnetic lateral flow immunochromatographic assay, Anal. Bioanal. Chem., 2018, 410(1), 123–130 CrossRef CAS PubMed.
- H. Yuan, F. Yan, L. Ma, F. Wu, J. Zhuang and W. Yang, Carboxyl-functionalized superparamagnetic Fe3O4/poly (St-co-MPS)/SiO2 composite particles for rapid and sensitive immunoassay, J. Nanosci. Nanotechnol., 2011, 11(3), 2232–2236 CrossRef CAS PubMed.
- M. Masello, Z. Lu, D. Erickson, J. Gavalchin and J. Giordano, A lateral flow-based portable platform for determination of reproductive status of cattle, J. Dairy Sci., 2020, 103(5), 4743–4753 CrossRef CAS PubMed.
- M. Zangheri, L. Cevenini, L. Anfossi, C. Baggiani, P. Simoni, F. Di Nardo and A. Roda, A simple and compact smartphone accessory for quantitative chemiluminescence-based lateral flow immunoassay for salivary cortisol detection, Biosens. Bioelectron., 2015, 64, 63–68 CrossRef CAS PubMed.
- J. Shin, S. Kim, T. Yoon, C. Joo and H.-I. Jung, Smart Fatigue Phone: Real-time estimation of driver fatigue using smartphone-based cortisol detection, Biosens. Bioelectron., 2019, 136, 106–111 CrossRef CAS PubMed.
- M. Zangheri, M. Mirasoli, M. Guardigli, F. Di Nardo, L. Anfossi, C. Baggiani, P. Simoni, M. Benassai and A. Roda, Chemiluminescence-based biosensor for monitoring astronauts' health status during space missions: results from the International Space Station, Biosens. Bioelectron., 2019, 129, 260–268 CrossRef CAS PubMed.
- J. Liu, Y. Wang, J. Luo, S. Xu, H. Jin and X. Cai, Study of Clinical Sample Detection for LH With Lateral Flow Immunochromatographic Strip Using Support Vector Regression, Annu. Int. Conf. IEEE Eng. Med. Biol. Soc., 2018, 4285–4288 Search PubMed.
- E. A. Shirtcliff, R. L. Buck, M. J. Laughlin, T. Hart, C. R. Cole and P. D. Slowey, Salivary cortisol results obtainable within minutes of sample collection correspond with traditional immunoassays, Clin. Ther., 2015, 37(3), 505–514 CrossRef CAS PubMed.
- H.-K. Oh, J.-W. Kim, J.-M. Kim and M.-G. Kim, High sensitive and broad-range detection of cortisol in human saliva using a trap lateral flow immunoassay (trapLFI) sensor, Analyst, 2018, 143(16), 3883–3889 RSC.
- J. Samsonova, V. Safronova and A. Osipov, Pretreatment-free lateral flow enzyme immunoassay for progesterone detection in whole cows' milk, Talanta, 2015, 132, 685–689 CrossRef CAS PubMed.
- H. Baker, M. Bangah, H. Burger, G. Kovacs, D. Summerbell and G. Warnes, Timing of ovulation by determination of the urinary luteinizing hormone surge with an enzyme-linked monoclonal antibody dipstick (OvuStick), Aust. N. Z. J. Obstet. Gynaecol., 1986, 26(1), 79–83 CrossRef CAS PubMed.
-
M. Yamaguchi, S. Yoshikawa, Y. Tahara, D. Niwa, Y. Imai and V. Shetty, in Point-of-use measurement of salivary cortisol levels, Sensors, 2009 IEEE, IEEE, 2009, pp. 343–346 Search PubMed.
- A. van Amerongen, D. van Loon, L. B. Berendsen and J. H. Wichers, Quantitative computer image analysis of a human chorionic gonadotropin colloidal carbon dipstick assay, Clin. Chim. Acta, 1994, 229(1–2), 67–75 CrossRef CAS.
- G. Osikowicz, M. Beggs, P. Brookhart, D. Caplan, S. Ching, P. Eck, J. Gordon, R. Richerson, S. Sampedro and D. Stimpson, One-step chromatographic immunoassay for qualitative determination of choriogonadotropin in urine, Clin. Chem., 1990, 36(9), 1586–1586 CrossRef CAS.
- M. Yamaguchi, Y. Matsuda, S. Sasaki, M. Sasaki, Y. Kadoma, Y. Imai, D. Niwa and V. Shetty, Immunosensor with fluid control mechanism for salivary cortisol analysis, Biosens. Bioelectron., 2013, 41, 186–191 CrossRef CAS PubMed.
- C. S. Kosack, A.-L. Page, L. T. Van Hulsteijn and E. G. Lentjes, TSH-CHECK-1 Test: Diagnostic Accuracy and Potential Application to Initiating Treatment for Hypothyroidism in Patients on Anti-Tuberculosis Drugs, PLoS One, 2012, 7(3), e33704 CrossRef CAS PubMed.
- E. M. Hamad, G. Hawamdeh, N. A. Jarrad, O. Yasin, S. I. Al-Gharabli and R. Shadfan, Detection of Human Chorionic Gonadotropin (hCG) Hormone using Digital Lateral Flow Immunoassay, Annu. Int. Conf. IEEE Eng. Med. Biol. Soc., 2018, 3845–3848 Search PubMed.
- S. Bu, K. Wang, C. Ju, Y. Han, Z. Li, P. Du, Z. Hao, C. Li, W. Liu and J. Wan, A pregnancy test strip for detection of pathogenic bacteria by using concanavalin A-human chorionic gonadotropin-Cu 3 (PO 4) 2 hybrid nanoflowers, magnetic separation, and smartphone readout, Microchim. Acta, 2018, 185(10), 1–7 Search PubMed.
- J. Bonnar, A. Flynn, G. Freundl, R. Kirkman, R. Royston and R. Snowden, Personal hormone monitoring for contraception, Br. J. Fam. Plann., 1999, 24(4), 128–134 CAS.
- R. Thakur, F. Akram, V. Rastogi, A. Mitra, R. Nawani, V. Av, S. K. Dubey and C. Shakher, Development of Smartphone-Based Lateral Flow Device for the Quantification of LH and E3G Hormones, IEEE Sens. J., 2020, 20(23), 14491–14500 CAS.
- P. Matías-García, J. Martinez-Hurtado, A. Beckley, M. Schmidmayr and V. Seifert-Klauss, Hormonal Smartphone Diagnostics, Methods Mol. Biol., 2018, 1735, 505–515 CrossRef PubMed.
- N. El-Farhan, D. A. Rees and C. Evans, Measuring cortisol in
serum, urine and saliva - are our assays good enough?, Ann. Clin. Biochem., 2017, 54(3), 308–322 CrossRef CAS PubMed.
-
Abbott Point of Care Testing, https://www.pointofcare.abbott/int/en/about-us/benefits-of-point-of-care-testing Search PubMed.
-
GmbH, S. P. D., Our products, https://www.swissprecisiondiagnostics.com/our-products/ Search PubMed.
-
Blue, C., Early Detection Pregnancy Test, https://www.clearblue.com/pregnancy-tests/early-detection Search PubMed.
-
Abbott Alere hCG Easy Pregnancy Test, https://www.globalpointofcare.abbott/en/product-details/alere-hcg-easy-pregnancy.html Search PubMed.
-
Healthineers, S., Clinitest hCG Pregnancy Test, https://www.siemens-healthineers.com/urinalysis-products/urinalysis-reagents/clinitest-hcg-pregnancy-test Search PubMed.
-
Diagnostics, E., QuPID hCG Pregnancy Test, https://www.ekfusa.com/womens_health/qupid-hcg-test/ Search PubMed.
-
Scientific, F., Quidel Sofia hCG FIA, https://www.fishersci.com/shop/products/quidel-sofia-hcg-fia-3/23043079 Search PubMed.
-
Scientific, F., Germaine Laboratories AimStrip Pregnancy Test, https://www.fishersci.com/shop/products/aimstep-pregnancy-test-1/23111296 Search PubMed.
-
Scientific, F., Sekisui Diagnostics OSOM hCG Urine Test Kit, https://www.fishersci.com/shop/products/sekisui-diagnostics-osom-hcg-urine-test-kit/22071063 Search PubMed.
-
Diagnostics, C., hCG Urine Strips, https://claritydiagnostics.com/hcg-urine-strips/ Search PubMed.
-
McKesson Rapid Test Kit McKesson Consult Fertility Test hCG Pregnancy Test Urine Sample 25 Tests, https://mms.mckesson.com/product/949871/McKesson-Brand-5001 Search PubMed.
- CLIAwaived CLIAwaived, Inc. Pregnancy Tests (50 Cassettes), https://cliawaived.com/cliawaived-inc-pregnancy-test-cassette.html.
-
BTNX hCG Pregnancy Test Strip, https://www.btnx.com/Product?id=1782 Search PubMed.
-
Scientific, S., Pregnancy (hCG) One-Step, https://www.sascientific.com/products/sas-rapid-diagnostic-tests/fertility-and-pregnancy/item/pregnancy-hcg-one-step Search PubMed.
-
CLIAwaived Instant-View Pregnancy Urine Dip Strips, https://cliawaived.com/instant-view-pregnancy-urine-dip-strip.html Search PubMed.
-
Bioproducts, M., Human Chorionic Gonadotropin (hCG) test for early detection of pregnancy, https://microgenbioproducts.com/hcg/ Search PubMed.
-
Scientific, F., Fisher HealthCare Sure-Vue STAT Serum/Urine hCG Test Kit, https://www.fishersci.com/shop/products/fisher-healthcare-sure-vue-stat-serum-urine-hcg-test-kit-2/p-3347614 Search PubMed.
-
Bioscience, M., Immunocard STAT! hCG, https://www.meridianbioscience.com/human-condition/other/pregnancy-2/immunocard-stat-hcg/ Search PubMed.
-
Scientific, F., Polymedco Poly stat hCG Pregnancy Test, https://www.fishersci.com/shop/products/polymedco-poly-stat-hcg-pregnancy-test/21000127 Search PubMed.
-
Scientific, F., HemoCue America Beckman Coulter ICON 25 hCG Test Kit, https://www.fishersci.com/shop/products/beckman-coulter-icon-25-hcg-test-kit/22247114 Search PubMed.
-
Scientific, S., Pregnancy (hCG) Ultimate Serum/Urine, https://www.sascientific.com/products/sas-rapid-diagnostic-tests/fertility-and-pregnancy/item/pregnancy-hcg-ultimate-serum-urine Search PubMed.
-
Scientific, F., Quidel hCG Quick Urine Control for QuickVue+ One-Step hCG Combo Lateral Flow Test Kit, https://www.fishersci.com/shop/products/quidel-quickvue-one-step-hcg-combo-lateral-flow-test-kit-1/22043045 Search PubMed.
-
Scientific, F., Sekisui Diagnostics OSOM Ultra hCG Combo Rapid Test, https://www.fishersci.com/shop/products/osom-ultra-hcg-combo-rapid-test/22321113 Search PubMed.
-
Scientific, F., Cen-Med Enterprises Sensitive Urine & Serum hCG Pregnancy Test Kit, https://www.fishersci.com/shop/products/cen-med-enterprises-sensitive-urine-serum-hcg-pregnancy-test-kit/21520106 Search PubMed.
-
Scientific, F., Germaine Laboratories AimStep Combo Pregnancy, https://www.fishersci.com/shop/products/aimstep-combo-pregnancy/23111299 Search PubMed.
-
Diagnostics, C., hCG Combo 20 (Urine/Serum), https://claritydiagnostics.com/hcg-combo-urineserum-2/ Search PubMed.
-
CLIAwaived Instant-View Combo (Urine/Serum) Dip Strips, https://cliawaived.com/instant-view-combo-urine-serum-dip-strip.html Search PubMed.
-
Scientific, F., NOW Diagnostics ADEXUSDx hCG Test, https://www.fishersci.com/shop/products/adexusdx-hcg-test-2/p-8481008 Search PubMed.
- Lab, V., Products: Hormones.
-
BTNX LH Midstream Test, https://www.btnx.com/Product?id=1710 Search PubMed.
-
Joysbio Luteinizing Hormone Test Kit (Colloidal Gold), https://en.joysbio.com/product/lh/ Search PubMed.
-
Designs, A. S.
Instant-view LH Ovulation Urine Tests, https://www.alfascientific.com/products/ovulation/ Search PubMed.
-
Scientific, S., Ovulation (LH), https://www.sascientific.com/products/sas-rapid-diagnostic-tests/fertility-and-pregnancy/item/ovulation-lh Search PubMed.
-
Laboratories, G., AimStep Ovulation, https://www.germainelabs.com/product/aimstep-ovulation-30-box/ Search PubMed.
-
Biotech, C., LH FIA Test, https://ctkbiotech.com/product/lh-fia-test/ Search PubMed.
-
Scientific, A., Instant-view FSH Urine Test, https://www.alfascientific.com/products/menopause/ Search PubMed.
-
Scientific, S., FSH (Follicle Stimulating Hormone), https://www.sascientific.com/products/sas-rapid-diagnostic-tests/fertility-and-pregnancy/item/fsh-follicle-stimulating-hormone Search PubMed.
-
BTNX FSH Menopause Test Cassette, https://www.btnx.com/Product?id=1897 Search PubMed.
-
Biotech, C., FSH FIA Test, https://ctkbiotech.com/product/fsh-fia-test/ Search PubMed.
-
Biotech, C., Progesterone FIA Test, https://ctkbiotech.com/product/progesterone-fia-test/ Search PubMed.
-
Bioscience, S., SOMA Cortisol Lateral Flow Device (LFD), https://somabioscience.com/wp-content/uploads/2017/02/SOMA-Cortisol-LFD-Product-Sheet.pdf Search PubMed.
-
McKesson Rapid Test Kit Accutest Thyroid/Metabolic Assay Thyroid Stimulating Hormone (TSH) Whole Blood Sample 20 Tests, https://mms.mckesson.com/product/743134/Jant-Pharmacal-Corporation-MD801 Search PubMed.
-
McKesson Rapid Test Kit AimStep Thyroid Screen Thyroid, https://mms.mckesson.com/product/909462/Germaine-Laboratories-Inc-76220 Search PubMed.
-
BTNX Thyroid Stimulating Hormone (TSH) Test Cassette, https://www.btnx.com/Product?id=1716 Search PubMed.
-
Biotech, C., TSH FIA Test, https://ctkbiotech.com/product/tsh-fia-test/ Search PubMed.
-
Biotech, C., T3 FIA Test, https://ctkbiotech.com/product/t3-fia-test/ Search PubMed.
-
Biotech, C., T4 FIA Test, https://ctkbiotech.com/product/t4-fia-test/ Search PubMed.
-
Quidel Sofia Quantitative Vitamin D FIA, https://www.quidel.com/immunoassays/rapid-vitamin-d-tests/sofia-quantitative-vitamin-d-fia Search PubMed.
-
Lab, V., Qualtiative rapid tests of reader, https://www.vedalab.com/product/qualitative-rapid-tests-for-reader/ Search PubMed.
- S. Dalirirad, D. Han and A. J. Steckl, Aptamer-Based Lateral Flow Biosensor for Rapid Detection of Salivary Cortisol, ACS Omega, 2020, 5(51), 32890–32898 CrossRef CAS PubMed.
- S. Jooken, Y. de Coene, O. Deschaume, D. Zámbó, T. Aubert, Z. Hens, D. Dorfs, T. Verbiest, K. Clays and G. Callewaert, Enhanced electric field sensitivity of quantum dot/rod two-photon fluorescence and its relevance for cell transmembrane voltage imaging, Nanophotonics, 2021, 10(9), 2407–2420 CrossRef CAS.
-
NHS NHS Long-term plan: Diabetes, https://www.longtermplan.nhs.uk/online-version/chapter-3-further-progress-on-care-quality-and-outcomes/better-care-for-major-health-conditions/diabetes/ Search PubMed.
|
This journal is © The Royal Society of Chemistry 2022 |
Click here to see how this site uses Cookies. View our privacy policy here.