DOI:
10.1039/D1SC04403F
(Edge Article)
Chem. Sci., 2021,
12, 14570-14576
Chiral exciplex dyes showing circularly polarized luminescence: extension of the excimer chirality rule†
Received
11th August 2021
, Accepted 18th October 2021
First published on 18th October 2021
Abstract
A series of axially chiral binaphthyls and quaternaphthyls possessing two kinds of aromatic fluorophores, such as pyrenyl, perylenyl, and 4-(dimethylamino)phenyl groups, arranged alternately were synthesized by a divergent method. In the excited state, the fluorophores selectively formed a unidirectionally twisted exciplex (excited heterodimer) by a cumulative steric effect and exhibited circularly polarized luminescence (CPL). They are the first examples of a monomolecular exciplex CPL dye. This versatile method for producing exciplex CPL dyes also improved fluorescence intensity, and the CPL properties were not very sensitive to the solvent or to the temperature owing to the conformationally rigid exciplex. This systematic study allowed us to confirm that the excimer chirality rule can be applied to the exciplex dyes: left- and right-handed exciplexes with a twist angle of less than 90° exhibit (−)- and (+)-CPL, respectively.
Introduction
Circularly polarized luminescence (CPL) organic dyes are recognized to be next-generation materials,1 and their development has been a recent focus of research.2 To develop CPL dyes, connecting two of the same aromatic luminophores, which can form an excimer (excited homodimer), to a chiral unit is one of the main approaches because these luminophores often emit with high quantum yields and large Stokes shifts. For example, CPL-active pyrene,3,4 perylene,4b,5 anthracene,6 carbazole,7 and naphthalimide5c,8 excimers have been reported. In contrast, exciplex (excited heterodimer) CPL dyes are still rare, although exciplex fluorescence (FL) dyes are often used in the field of materials and biological sciences.9 Exciplex CPL dyes will widen the range of application significantly due to the infinite number of combinations of two luminophores. Exciplex CPL dyes will widen the range of application significantly due to the infinite number of combinations of two luminophores; CPL dyes having desired absorption and emission wavelength ranges can be provided by the combination of different luminophores. In addition, information obtained from simple exciplex CPL dyes may be useful for the investigation of the conformation of complex (supra)molecules in the excited state. Yamaguchi–Meijer group has recently reported supramolecular aggregates including O-bridged triphenyl-borane and triphenylamine moieties as the sole example of an exciplex CPL dye, although the aggregation requires a specific temperature and solvent, and the fluorophore versatility is unknown.10 A new and versatile method for creating exciplex CPL dyes that are not affected by conditions is needed. Rigid and monomolecular chiral dyes are expected to reach this goal.
In the past decade, several axially chiral binaphthyl-based CPL dyes have been reported, demonstrating that the binaphthyl is one of the most suitable skeletons for the development of CPL dyes.11 We have also developed several axially chiral CPL dyes.3,12 Among them, tetrapyrenyl-appended binaphthyl (R)-1 displayed pyrene-excimer CPL with a glum value13 of +1.3 × 10−2 and a typical FL quantum yield (Φfl) of 0.24 (Fig. 1a).3a The quaternaphthyl analogs showed slightly greater CPL performance.3b The CPL was based on the locally chiral intramolecular excimer, which allowed us to discover the excimer chirality rule: left- and right-handed twisted excimers exhibit (−)- and (+)-CPL, respectively. We postulated that the binaphthyl- and quaternaphthyl-based strategy might be applied to creating exciplex CPL dyes. Herein, we synthesized binaphthyls (R)-2–6 and quaternaphthyl (R,R,R)-7 possessing two kinds of well-known fluorophores alternately arranged, such as pyrenyl, perylenyl, and 4-(dimethylamino)phenyl groups,9 as the first examples of monomolecular exciplex CPL dyes in addition to CPL-active pyrene–perylene and pyrene–4-(dimethylamino)phenyl exciplexes (Fig. 1b). The experimental and computational studies gave us an extended rule, named the exciplex chirality rule: left- and right-handed exciplexes exhibit (−)- and (+)-CPL, respectively.
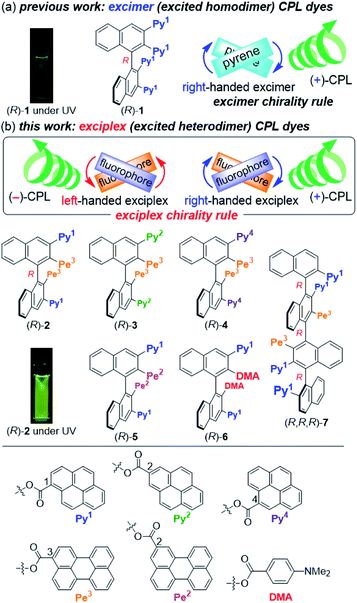 |
| Fig. 1 Chemical structures of 1–7 and the exciplex chirality rule. Conditions of photographs: CH2Cl2, 5.0 × 10−7 M, rt. Φfl values are 0.21 for 1 and 0.83 for 2. | |
Results and discussion
Synthesis of 2–7
The synthetic routes to (R)-2 and (R,R,R)-7 are shown in Scheme 1. The condensation of bis-MOM-protected binaphthyl-tetraol (R)-8 (ref. 14) with 1-pyrenecarboxylic acid (9) gave di-pyrenyl-appended binaphthyl (R)-10. Subsequent deprotection and condensation with 3-perylenecarboxylic acid (11) afforded (R)-2 in moderate yield. Similarly, condensation of quaternaphthyl-diol (R,R,R)-12 (ref. 12b) with 11 gave (R,R,R)-13, and then deprotection and condensation with 9 afforded (R,R,R)-7. Compounds (R)-3–6 were prepared by a similar method using common intermediates (Schemes S1 and S2†).15
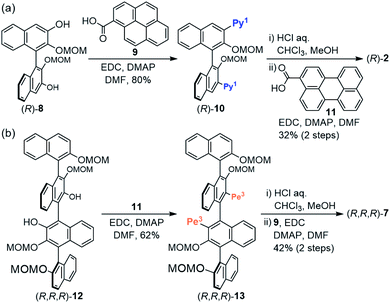 |
| Scheme 1 Synthesis of (R)-2 and (R,R,R)-7. | |
Absorption and fluorescence properties of 2–7 and reference compounds
We next analyzed the optical properties of 2–7 (Fig. 2 and S7†). These absorption spectral shapes comprised the sum of the fluorophores16 and the binaphthyl or quaternaphthyl spectra,3b,17 suggesting that in the ground state, each fluorophore is electronically isolated (Fig. 2b). The binaphthyl and quaternaphthyl skeletons absorbed light below the 350 nm wavelength; therefore, the strong CD signals with the |Δε| of over 25 were detected at around 450 nm, which indicates that the fluorophores are located in asymmetric environments (Fig. 2a). A binaphthyl (R)-17 possessing only two perylene rings, a reference compound, showed almost the same CD signals as those of (R)-2–4 (Fig. 2a and S6†), which indicates that the CD signals of (R)-2–4 at around 450 nm were mainly derived from the exciton coupling between the perylene rings (gabs = ca. −8.0 × 10−4).
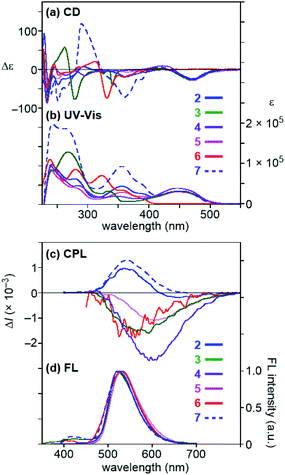 |
| Fig. 2 (a) CD, (b) UV-Vis, (c) CPL, and (d) FL spectra of (R)-2–6 and (R,R,R)-7. Conditions: CH2Cl2, 1.0 × 10−5 M for 2–6 and 6.7 × 10−6 M for 7, 20 °C, l = 1 cm, λex = 355 nm, baseline-corrected. | |
The FL spectra of 2–7 are shown in Fig. 2d. All the FL were identical with those of the typical exciplexes with the λex,max of ca. 530 nm.18 The Φfl values were 0.16–0.99, which are unexpectedly high for exciplexes, probably because the movement and vibration were suppressed by the contiguous four or six large fluorophores (Table 1). For example, the Φfl of 2 (0.83), 3 (0.95), 4 (0.99), 5 (0.63), and 6 (0.16) were equal or higher than those of the corresponding half model compounds 2′ (0.53), 3′ (0.92), 4′ (0.99), 5′ (0.51), and 6′ (0.06), respectively (Fig. S2†). To gain a deeper insight into excited-state characteristics, FL spectra of reference compounds, 3-methyl perylenecarboxylate (14) and two fluorophore-appended naphthalenes and binaphthyls 2′ and 15–17 were measured and compared with the spectra of 2 and 7 (Fig. 3 and S3†). The FL of 14, 2′, and 15 can be regarded as perylene-monomer, pyrene–perylene-exciplex, and perylene-excimer FL, respectively. The FL of 2 and 7 completely coincided with that of 2′, which indicates that the FL spectra of 2 and 7 are classified as exciplex FL that is completely free from the monomer and excimer FL. The fluorescence lifetimes of (R)-2 (16.3 ns) and (R)-6 (25.0 ns) were close to the reported lifetimes of pyrene–perylene exciplexes and pyrene–4-(dimethylamino)phenyl exciplexes, respectively.18 In addition, we measured the excitation spectra of (R)-2 and (R)-6, and the signals appeared in the absorption region of pyrene and perylene rings for (R)-2 and that of a pyrene ring and a 4-(dimethylamino)phenyl group for (R)-6 (Fig. S5†). These results support our assignment that they are exciplex fluorescence. In contrast, binaphthyl 16 exhibited the only perylene-monomer FL, which suggested that the two fluorophores located across the axis could not form an exciplex and that the energy transfer from the pyrene ring to the perylene ring was very fast. Binaphthyl 17 also exhibited the monomer FL without forming an excimer. These results clearly indicate that only a pair of fluorophores on the same naphthalene ring can form an exciplex efficiently and selectively. Quaternaphthyl 7 exhibited only exciplex FL due to the fast energy transfer from the outer pyrene rings to the inner pyrene rings.
Table 1 Luminescence and conformational properties of 2–7
Compd |
Φ
fl
,
|
Experimental gluma (10−3) |
Theoretical glumc (10−3) |
Twist of exciplexc |
Conditions: CH2Cl2, 1.0 × 10−5 M for 2−6 and 6.7 × 10−6 M for 7, 20 °C, l = 1 cm.
Absolute fluorescence quantum yield.
TD DFT calculations were carried out at the CAM-B3LYP/6-31G(d,p) level.
|
(R)-2 |
0.83 |
+1.0 |
+0.49 |
Right-handed |
(R)-3 |
0.95 |
−1.6 |
−0.91 |
Left-handed |
(R)-4 |
0.99 |
−2.7 |
−0.93 |
Left-handed |
(R)-5 |
0.63 |
−1.1 |
−2.7 |
Left-handed |
(R)-6 |
0.16 |
−1.8 |
−3.6 |
Left-handed |
(R,R,R)-7 |
0.89 |
+1.3 |
+0.32 |
Right-handed |
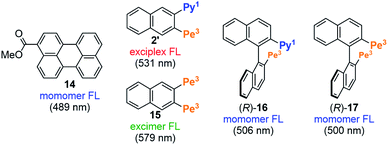 |
| Fig. 3 FL properties of reference compounds 2′ and 14–17. The λex,max values are given in parentheses. | |
CPL properties
To our delight, compounds 2–7 displayed clear CPL with |glum| values between 1.0 × 10−3 and 2.7 × 10−3 (Fig. 2c and Table 1).19 This result suggests that the binaphthyl-based strategy is a versatile method for providing exciplex CPL dyes. Interestingly, compounds (R)-2 and (R,R,R)-7 exhibited (+)-CPL and (R)-3–6 exhibited (−)-CPL, as the variation in the CPL sign has previously been observed for excimer CPL dyes (vide infra).3b Among compounds 2–7, compound 4 exhibited the highest |glum| value (2.7 × 10−3) in addition to the Φfl value (0.99). The CPL properties were not very sensitive to the solvent or to the temperature (10 to 90 °C) owing to the conformationally rigid exciplex, which is one of the main advantages of monomolecular dyes (Fig. 4). Both the |glum| and Φfl values of quaternaphthyl 7 (|glum| of 1.3 × 10−3 and Φfl of 0.89) were slightly higher than those of corresponding binaphthyl 2 (|glum| of 1.0 × 10−3 and Φfl of 0.83). Zinna group has recently proposed a new quantity for evaluating the overall efficiency of CPL, named CPL brightness (BCPL), which is defined as ε × Φfl × glum/2.2j,20 Among compounds 2–7, compound 7 showed the highest BCPL value of 58, and the value is the highest degree for organic dyes. However, considering the complexity of the syntheses, the binaphthyl as a chiral skeleton is also a good choice.
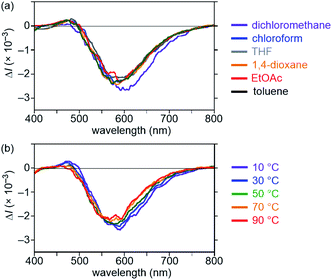 |
| Fig. 4 (a) CPL spectra of (R)-4 in various solutions at 20 °C and (b) VT CPL spectra of (R)-4 in toluene. Conditions: 1.0 × 10−5 M, l = 1 cm, λex = 355 nm. | |
DFT-optimized structures in the ground and excited states and the exciplex chirality rule
To clarify the correlation between conformation and CPL properties, DFT and time-dependent (TD) DFT calculations21 of compounds 2–7 were carried out using the Coulomb-attenuating method (CAM),22 which is suitable for polycyclic aromatic compounds (Fig. 5 and S9–S16†). We confirmed the optimized structures corresponded to an energy minimum by frequency analysis. The optimized ground- and excited-state structures of representatives 2 and 3 are shown in Fig. 5. For the most stable ground-state structures, the adjacent carbonyl groups were oriented in opposite directions from one another, and the adjacent fluorophores were apart from one another, perhaps due to the steric repulsion (Fig. 5a and c). Other metastable conformers, in which the direction of one or more carbonyl groups was flipped from the most stable structures, were significantly unstable (ΔE of 2.5–7.0 kcal mol−1 for 2 and 2.8–5.4 kcal mol−1 for 3, Fig. S11 and S12†). These characteristics were also seen in 4 (2.9–5.9 kcal mol−1), 5 (1.5–5.4 kcal mol−1), 6 (1.5–5.6 kcal mol−1), and 7 (4.1–19.4 kcal mol−1) (Fig. S13–S16†), suggesting that the most stable structures are conformationally-rigid. The especially large ΔE values of 7 support that higher rigidity leads to a higher |glum| value. In contrast, the ΔE value of two conformers of naphthalene 2′, whose carbonyl groups were oriented in the same and opposite directions, was small (0.8 kcal mol−1, Fig. S17†). These results suggest that the local chirality of fluorophores in 2–7 are induced by the following mechanisms, (1) adjacent carbonyl groups are oriented in the opposite directions due to steric and dipole repulsions, (2) adjacent fluorophores are directed away from each other via steric repulsion, and (3) the cumulative steric and dipole repulsions lead to the conformationally rigid structure, which is reminiscent of the sergeants-and-soldiers effect.23 Prior to analyzing the excited-state structures directly concerned with CPL properties, the orientations of the lowest energy transition dipole moment of each fluorophore moiety were theoretically determined using the corresponding methyl esters (Fig. S8†). The ester group affected the alignment of the transition moment slightly, and therefore this tilt was used in the following analyses. The excited-state structures of 2 and 3 were optimized, and the pyrene–perylene exciplexes were surely obtained (Fig. 5b and d). It is noteworthy that the exciplex moieties of 2 and 3 adopted a right- and left-handed chirality, respectively. Theoretical CPL signs of the exciplexes are (+) for 2 and (−) for 3, which are identical with the experimental signs (Table 1). This relationship between the twist direction and CPL sign was also found to be applicable to 4–7. These results allowed us to find an empirical rule named the exciplex chirality rule: left- and right-handed exciplexes exhibit (−)- and (+)-CPL, respectively (Fig. 1b). That is, importantly, the excimer chirality rule can be extended to exciplexes.
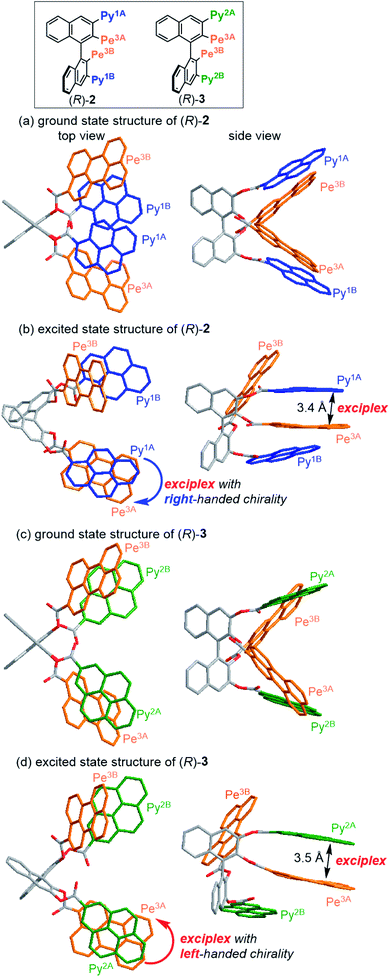 |
| Fig. 5 (TD) DFT-optimized ground- and excited-state structures of (a and b) (R)-2 and (c and d) (R)-3 at the CAM-B3LYP/6-31G(d,p) level. | |
Relationship between the twist angle and CPL sign of exciplexes
Finally, we investigated the relationship between the CPL sign and the twist pattern/angle of exciplexes in more detail. The sign of CPL of the model exciplex consisting of 1-methyl pyrenecaboxylate and 3-methyl perylenecaboxylate was calculated for every 15° change in the twist angle (Fig. 6a and Table S1†). As a result, interestingly, the sign was clearly inverted every 90°: (+)-CPL for 0–90° and 180–270° and (−)-CPL for 90–180° and 270–360°. The model pyrene–perylene exciplex regioisomers and pyrene–4-(dimethylamino)phenyl exciplex showed almost the same tendency (Tables S3–S6†).24 Moreover, an unsubstituted pyrene–perylene exciplex had the same result, which indicates generality (Fig. 6b and Table S2†).
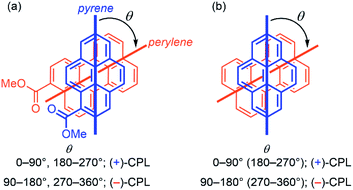 |
| Fig. 6 Relationship between the twist angle and theoretical sign of CPL of the pyrene–perylene exciplexes. TD DFT calculations were performed at the CAM-B3LYP/6-311+G(2d,p) level. | |
Conclusions
We have developed CPL-active pyrene–perylene and pyrene–4-(dimethylamino)phenyl exciplexes using the axially chiral binaphthyl or quaternaphthyl skeletons as the first example of a monomolecular exciplex CPL dye. The CPL were insensitive to conditions such as solvent and temperature. The method improved FL intensity as a bonus. We found that the left- and right-handed exciplexes with a twisted narrow angle exhibited (−)- and (+)-CPL, respectively. CPL based on this relationship may be a powerful tool for the determination of absolute configurations and conformations of multiple aromatics in the excited state. CPL-active exciplexes will enable new applications and promote chiroptical sciences. Development of other exciplex CPL dyes using this method is being studied in our laboratory.
Data availability
All experimental supporting data and procedures are available in the ESI.†
Author contributions
K. T. and T. E. planned and supervised the project. S. M. and K. I. synthesized and characterized the compounds and measured their optical spectra. K. T. conducted the DFT calculations. K. T. and T. E. wrote the initial draft of the manuscript, and all authors discussed the results and commented on the manuscript.
Conflicts of interest
There are no conflicts to declare.
Acknowledgements
This work was supported by JSPS KAKENHI Grant No. 21K05022, the Kyoto Technoscience Center, the Okayama Foundation for Science and Technology, and the Konica Minolta Science and Technology Foundation. The computations were performed using RCCS, Okazaki, Japan.
Notes and references
- For applications of CPL dyes:
(a) C. Wagenknecht, C.-M. Li, A. Reingruber, X.-H. Bao, A. Goebel, Y.-A. Chen, Q. Zhang, K. Chen and J.-W. Pan, Nat. Photonics, 2010, 4, 549–552 CrossRef CAS;
(b) R. Carr, N. H. Evans and D. Parker, Chem. Soc. Rev., 2012, 41, 7673–7686 RSC;
(c) R. Singh, K. N. N. Unni, A. Solanki and Deepak, Opt. Mater., 2012, 34, 716–723 CrossRef;
(d) M. C. Heffern, L. M. Matosziuk and T. J. Meade, Chem. Rev., 2014, 114, 4496–4539 CrossRef CAS PubMed;
(e) A. T. Frawley, H. V. Linford, M. Starck, R. Pal and D. Parker, Chem. Sci., 2018, 9, 1042–1049 RSC;
(f) M. Li, S.-H. Li, D. Zhang, M. Cai, L. Duan, M.-K. Fung and C.-F. Chen, Angew. Chem., Int. Ed., 2018, 57, 2889–2893 CrossRef CAS PubMed;
(g) D.-W. Zhang, M. Li and C.-F. Chen, Chem. Soc. Rev., 2020, 49, 1331–1343 RSC;
(h) Z. Jiang, J. Wang, T. Gao, J. Ma, Z. Liu and R. Chen, ACS Appl. Mater. Interfaces, 2020, 12, 9520–9527 CrossRef CAS PubMed;
(i) G. Albano, G. Pescitelli and L. Di Bari, Chem. Rev., 2020, 120, 10145–10243 CrossRef CAS PubMed;
(j) Z.-L. Tu, Z.-P. Yan, X. Liang, L. Chen, Z.-G. Wu, Y. Wang, Y.-X. Zheng, J.-L. Zuo and Y. Pan, Adv. Sci., 2020, 7, 2000804 CrossRef CAS PubMed;
(k) L. E. MacKenzie and R. Pal, Nat. Chem. Rev., 2021, 5, 109–124 CrossRef CAS;
(l) K. Dhbaibi, L. Abella, S. Meunier-Della-Gatta, T. Roisnel, N. Vanthuyne, B. Jamoussi, G. Pieters, B. Racine, E. Quesnel, J. Autschbach, J. Crassous and L. Favereau, Chem. Sci., 2021, 12, 5522–5533 RSC.
- For reviews on organic CPL dyes:
(a) H. Maeda and Y. Bando, Pure Appl. Chem., 2013, 85, 1967–1978 CAS;
(b) E. M. Sánchez-Carnerero, A. R. Agarrabeitia, F. Moreno, B. L. Maroto, G. Muller, M. J. Ortiz and S. de la Moya, Chem.–Eur. J., 2015, 21, 13488–13500 CrossRef PubMed;
(c) J. Kumar, T. Nakashima and T. Kawai, J. Phys. Chem. Lett., 2015, 6, 3445–3452 CrossRef CAS PubMed;
(d) G. Longhi, E. Castiglioni, J. Koshoubu, G. Mazzeo and S. Abbate, Chirality, 2016, 28, 696–707 CrossRef CAS PubMed;
(e) H. Tanaka, Y. Inoue and T. Mori, ChemPhotoChem, 2018, 2, 386–402 CrossRef CAS;
(f) F. Pop, N. Zigon and N. Avarvari, Chem. Rev., 2019, 119, 8435–8478 CrossRef CAS PubMed;
(g) J.-L. Ma, Q. Peng and C.-H. Zhao, Chem.–Eur. J., 2019, 25, 15441–15454 CrossRef CAS PubMed;
(h) Y. Ohishi and M. Inouye, Tetrahedron Lett., 2019, 60, 151232 CrossRef CAS;
(i) A. Homberg and J. Lacour, Chem. Sci., 2020, 11, 6362–6369 RSC;
(j) L. Arrico, L. Di Bari and F. Zinna, Chem.–Eur. J., 2021, 27, 2920–2934 CrossRef CAS PubMed;
(k) J. L. Greenfield, J. Wade, J. R. Brandt, X. Shi, T. J. Penfoldd and M. J. Fuchter, Chem. Sci., 2021, 12, 8589–8602 RSC.
-
(a) K. Takaishi, R. Takehana and T. Ema, Chem. Commun., 2018, 54, 1149–1452 RSC;
(b) K. Takaishi, K. Iwachido, R. Takehana, M. Uchiyama and T. Ema, J. Am. Chem. Soc., 2019, 141, 6185–6190 CrossRef CAS PubMed.
-
(a) M. Inouye, K. Hayashi, Y. Yonenaga, T. Itou, K. Fujimoto, T. Uchida, M. Iwamura and K. Nozaki, Angew. Chem., Int. Ed., 2014, 53, 14392–14396 CrossRef CAS PubMed;
(b) M. Nakamura, J. Suzuki, F. Ota, T. Takada, K. Akagi and K. Yamana, Chem.–Eur. J., 2016, 22, 9121–9124 CrossRef CAS PubMed;
(c) Y. Hashimoto, Y. Nakashima, D. Shimizu and T. Kawai, Chem. Commun., 2016, 52, 5171–5174 RSC;
(d) T. Ikai, Y. Kojima, K. Shinohara, K. Maeda and S. Kanoh, Polymer, 2017, 117, 220–224 CrossRef CAS;
(e) S. Ito, K. Ikeda, S. Nakanishi, Y. Imai and M. Asami, Chem. Commun., 2017, 53, 6323–6326 RSC;
(f) S. Koga, S. Ueki, M. Shimada, R. Ishii, Y. Kurihara, Y. Yamanoi, J. Yuasa, T. Kawai, T. Uchida, M. Iwamura, K. Nozaki and H. Nishihara, J. Org. Chem., 2017, 82, 6108–6117 CrossRef CAS PubMed;
(g) Y. Mimura, S. Kitamura, M. Shizuma, M. Kitamatsu and Y. Imai, Org. Biomol. Chem., 2018, 16, 8273–8279 RSC;
(h) P. Reiné, J. Justica, S. P. Morcillo, S. Abbate, B. Vaz, M. Ribagorda, Á. Orte, L. Á. de Cienfuegos, G. Longhi, A. G. Campaña, D. Miguel and J. M. Cuerva, J. Org. Chem., 2018, 83, 4455–4463 CrossRef PubMed;
(i) Y. Wang, X. Li, F. Li, W.-Y. Sun, C. Zhu and Y. Cheng, Chem. Commun., 2018, 53, 7505–7508 RSC;
(j) H. Anetai, T. Takeda, N. Hoshino, Y. Araki, T. Wada, S. Yamamoto, M. Mitsuishi, H. Tsuchida, T. Ogoshi and T. Akutagawa, J. Phys. Chem. C, 2018, 122, 6323–6331 CrossRef CAS;
(k) D. Niu, Y. Jiang, L. Ji, G. Ouyang and M. Liu, Angew. Chem., Int. Ed., 2019, 58, 5946–5950 CrossRef CAS PubMed;
(l) N. Hara, M. Shizuma, T. Harada and Y. Imai, RSC Adv., 2020, 10, 11335–11338 RSC.
-
(a) J. Li, C. Yang, X. Peng, Q. Qi, Y. Li, W.-Y. Lai and W. Huang, Org. Biomol. Chem., 2017, 15, 8463–8470 RSC;
(b) K. Hayashi, Y. Miyaoka, Y. Oishi, T. Uchida, M. Iwamura, K. Nozaki and M. Inouye, Chem.–Eur. J., 2018, 24, 14613–14616 CrossRef CAS PubMed;
(c) A. Homberg, E. Brun, F. Zinna, S. Pascal, M. Górecki, L. Monnier, C. Besnard, G. Pescitelli, L. Di Bari and J. Lacour, Chem. Sci., 2018, 9, 7043–7052 RSC.
-
(a) K. Miki, T. Noda, M. Gon, K. Tanaka, Y. Chujo, Y. Mizuhata, N. Tokitoh and K. Ohe, Chem.–Eur. J., 2019, 25, 9211–9216 CrossRef CAS PubMed;
(b) H. Nian, L. Cheng, L. Wang, H. Zhang, P. Wang, Y. Li and L. Cao, Angew. Chem., Int. Ed., 2021, 60, 15354–15358 CrossRef CAS PubMed.
- K. Tani, R. Imafuku, K. Miyanaga, M. E. Masaki, H. Kato, K. Hori, K. Kubono, M. Tanega, T. Harada, K. Goto, F. Tani and T. Mori, J. Phys. Chem. A, 2020, 124, 2057–2063 CrossRef CAS PubMed.
- Y. Sheng, J. Ma, S. Liu, Y. Wang, C. Zhu and Y. Cheng, Chem.–Eur. J., 2016, 22, 9519–9522 CrossRef CAS PubMed.
- For books and a review of exciplex dyes:
(a) N. Mataga and H. Miyasaka, Adv. Chem. Phys., 1999, 107, 431–496 CAS;
(b)
B. Valeur, Molecular Fluorescence: Principles and Applications, Wiley-VCH, Weinheim, Germany, 2002 Search PubMed;
(c) Y. N. Teo and E. T. Kool, Chem. Rev., 2012, 112, 4221–4245 CrossRef CAS PubMed.
- B. Adelizzi, P. Chidchob, N. Tanaka, B. A. G. Lamers, S. C. J. Meskers, S. Ogi, A. R. A. Palmans, S. Yamaguchi and E. W. Meijer, J. Am. Chem. Soc., 2020, 142, 16681–16689 CrossRef CAS PubMed.
- For selected examples:
(a) H. Maeda, Y. Bando, K. Simonura, I. Yamada, M. Naito, K. Nobusawa, H. Tsumatori and T. Kawai, J. Am. Chem. Soc., 2011, 133, 9266–9269 CrossRef CAS PubMed;
(b) H. Langhals, A. Hofer, S. Bernhard, J. S. Siegel and P. Mayer, J. Org. Chem., 2011, 76, 990–992 CrossRef CAS PubMed;
(c) E. M. Sánchez-Carnerero, F. Moreno, B. L. Maroto, A. R. Agarrabeita, M. J. Ortiz, B. G. Vo, G. Muller and S. de la Moya, J. Am. Chem. Soc., 2014, 136, 3346–3349 CrossRef PubMed;
(d) C. Katakami, S. Kamo, A. Torii, N. Hara, Y. Imai, T. Taniguchi, K. Monde, Y. Okabayashi, T. Hosokai, K. Kuramochi and K. Tsubaki, J. Org. Chem., 2018, 83, 14610–14616 CrossRef CAS PubMed;
(e) X. Yang, J. Han, Y. Wang and P. Duan, Chem. Sci., 2019, 10, 172–178 RSC;
(f) Z.-B. Sun, J.-K. Liu, D.-F. Yuan, Z.-H. Zhao, X.-Z. Zhu, D.-H. Liu, Q. Peng and C.-H. Zhao, Angew. Chem., Int. Ed., 2019, 58, 4840–4846 CrossRef CAS PubMed;
(g) R. Takishima, Y. Nishii and M. Miura, Org. Lett., 2021, 23, 1349–1354 CrossRef CAS PubMed;
(h) Y. Nojima, M. Hasegawa, N. Hara, Y. Imai and Y. Mazaki, Chem.–Eur. J., 2021, 27, 5923–5929 CrossRef CAS PubMed;
(i) J. Hong, S. Kim, G. Park, Y. Lee, H. Kim, S. Kim, T.-W. Lee, C. Kim and Y. You, Chem. Sci., 2021, 12, 8668–8681 RSC.
- For selected papers:
(a) K. Takaishi, M. Yasui and T. Ema, J. Am. Chem. Soc., 2018, 140, 5334–5338 CrossRef CAS PubMed;
(b) K. Takaishi, S. Hinoide, T. Matsumoto and T. Ema, J. Am. Chem. Soc., 2019, 141, 11852–11857 CrossRef CAS PubMed;
(c) K. Takaishi, K. Iwachido and T. Ema, J. Am. Chem. Soc., 2020, 142, 1774–1779 CrossRef CAS PubMed;
(d) K. Takaishi, T. Matsumoto, M. Kawataka and T. Ema, Angew. Chem., Int. Ed., 2021, 60, 9968–9972 CrossRef CAS PubMed.
- The dissymmetry factor glum is defined as 2(IL − IR)/(IL + IR); IL and IR represent the intensity of the left and right CPL, respectively..
- A. Yanagisawa, T. Satou, A. Izumiseki, Y. Tanaka, M. Miyagi, T. Arai, K. Yoshida, S. T. Chau, J. P. Lutz, K. Wu and A. G. Doyle, Chem.–Eur. J., 2009, 15, 11450–11453 CrossRef CAS PubMed.
-
1H NMR signals of some compounds were broadened probably due to the slow movement of the contiguous large fluorophores. The purities of these compounds were confirmed by recycle HPLC analysis (Fig. S1†).
-
(a) C.-H. Zhang, Z.-B. Chen and Y.-B. Jiang, Spectrochim. Acta, Part A, 2004, 60, 2729–2732 CrossRef PubMed;
(b) M. Aleksiejew and J. R. Heldt, J. Lumin., 2007, 126, 665–676 CrossRef CAS.
- K. Tsubaki, H. Tanaka, K. Takaishi, M. Miura, H. Morikawa, T. Furuta, K. Tanaka, K. Fuji, T. Sasamori, N. Tokitoh and T. Kawabata, J. Org. Chem., 2006, 71, 6579–6587 CrossRef CAS PubMed.
-
(a) C. D. Borsarelli, J. J. Cosa and C. M. Previtali, Langmuir, 1993, 9, 2895–2901 CrossRef CAS;
(b) H. Kashida, M. Komiyama and H. Asamura, Chem. Lett., 2006, 35, 934–935 CrossRef CAS;
(c) J. N. Wilson, J. Gao and E. T. Kool, Tetrahedron, 2007, 63, 3427–3433 CrossRef CAS PubMed;
(d) J. Chiba, S. Takeshima, K. Mishima, H. Maeda, Y. Nanai, K. Mizuno and M. Inouye, Chem.–Eur. J., 2007, 13, 8124–8130 CrossRef CAS PubMed.
- (S)-2 and (R)-2 showed clear mirror images, which indicate that the effects of linearly polarized emission could be ignored (Fig. S4†).
- Mori group has proposed a related quantity value named circular polarization luminosity index, ΛCPL, which is defined as f × Φfl × glum/2: Y. Nagata and T. Mori, Front. Chem., 2020, 8, 448 CrossRef CAS PubMed.
- DFT calculations were performed using Gaussian16, revision C.01, M. J. Frisch, et al., Gaussian, Inc., Wallingford CT, 2019, for the full citations, see ESI.†.
- A. G. Crawford, A. D. Dwyer, Z. Liu, A. Steffen, A. Beeby, L.-O. Pålsson, D. J. Tozer and T. B. Marder, J. Am. Chem. Soc., 2011, 133, 13349–13362 CrossRef CAS PubMed.
- M. M. Green, M. P. Reidy, R. J. Johnson, G. Darling, D. J. O'Leary and G. Wilsin, J. Am. Chem. Soc., 1989, 111, 6452–6454 CrossRef.
- The CPL sign of the exciplex including 4-methyl pyrenecarboxylate with a θ of 225–255° was minus, possibly because the transition dipole moment deviates from the long axis of the pyrene ring due to the ester group attached on an orthogonal side (Fig. S8†).
Footnote |
† Electronic supplementary information (ESI) available: Synthesis, spectra, and computational details. See DOI: 10.1039/d1sc04403f |
|
This journal is © The Royal Society of Chemistry 2021 |
Click here to see how this site uses Cookies. View our privacy policy here.