DOI:
10.1039/D1SC04288B
(Edge Article)
Chem. Sci., 2021,
12, 15061-15066
Asymmetric hydrogenation for the synthesis of 2-substituted chiral morpholines†
Received
5th August 2021
, Accepted 26th October 2021
First published on 28th October 2021
Abstract
Asymmetric hydrogenation of unsaturated morpholines has been developed by using a bisphosphine-rhodium catalyst bearing a large bite angle. With this approach, a variety of 2-substituted chiral morpholines could be obtained in quantitative yields and with excellent enantioselectivities (up to 99% ee). The hydrogenated products could be transformed into key intermediates for bioactive compounds.
Introduction
Chiral N-heterocycles are widely used structural motifs present in a large number of valuable drug candidates and other bioactive compounds, in which chiral morpholine is one of the most important and attractive members (Fig. 1).1 Therefore it is no surprise that a variety of asymmetric synthetic approaches to afford chiral morpholines have been reported (Fig. 2).2–5 However, most of these methodologies require stoichiometric chiral starting materials or reagents,2 while relatively efficient and economical asymmetric catalytic methods have not been well studied.3–5 There are a few indirect catalytic examples which form the 2-stereocenter (the stereocenter adjacent to the O-atom) or 3-stereocenter (the stereocenter adjacent to the N-atom) before the cyclization of the morpholine ring (Fig. 2a).3 Other methods have been reported for the construction of the 2- or 3-stereocenter during the cyclization of the morpholine ring, such as the preparation of chiral 3-acylmethyl morpholines via organocatalyzed intramolecular aza-Michael addition and chiral 2-vinyl morpholines via metal-catalyzed allylic substitution (Fig. 2b).4 Furthermore, there are only a sporadic number of reports concerning the generation of the 3-stereocenter via enantioselective addition of the C
C or C
N bond after the cyclization of the unsaturated morpholine ring (Fig. 2c).5 No examples concerning the production of 2-substituted chiral morpholines via this way have been reported so far. Therefore, an efficient and universal catalytic method is highly desired to acquire such chiral morpholines, especially 2-substituted chiral compounds.
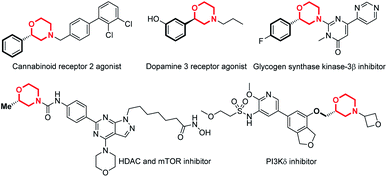 |
| Fig. 1 2-Substituted chiral morpholines as bioactive compounds. | |
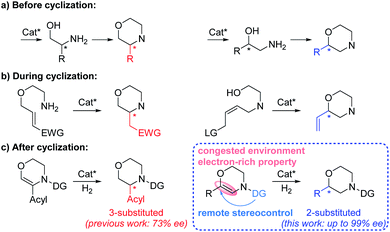 |
| Fig. 2 Asymmetric synthesis of 2- and 3-substituted chiral morpholines. (a) Form the stereocenter before cyclization; (b) form the stereocenter during cyclization; (c) form the stereocenter after cyclization. | |
The transition-metal-catalyzed asymmetric hydrogenation reaction is indisputably one of the most powerful methods for the acquisition of chiral molecules owing to its high efficiency, simple operation, and atom economy.6 It has been widely used as an “after cyclization” method for the efficient synthesis of various chiral N-heterocyclic compounds such as (benzo)piperidines and pyrrolidines.7 However, to the best of our knowledge, there are only two literature examples concerning asymmetric hydrogenation for the synthesis of 3-substituted chiral morpholines, and only 73% ee was obtained for the endocyclic alkenyl substrates.5e,f,8 In addition, an electron-withdrawing acyl substituent on the alkenyl was always required for the α-branched dehydromorpholines utilized in the asymmetric hydrogenation (Fig. 2c, left). Compared with 3-substituted chiral morpholines, asymmetric hydrogenation for the preparation of 2-substituted chiral morpholines with the stereocenter adjacent to the O-atom is considered to be more challenging and has not been reported at all. The main difficulty lies in the congested environment and electron-rich properties of the dehydromorpholine substrates, which results in very low reactivity. Introducing an N-acyl directing group is a universal strategy for the activation of enamine substrates.9 However, this is still insufficient and would bring about another difficulty to the untouched β-branched dehydromorpholines due to the challenge in the remote stereocontrol (Fig. 2c, right). Therefore, an efficient strategy needs to be developed and adopted to address the above problems.
In continuation of our long-term pursuit of highly efficient asymmetric catalytic hydrogenations,10 we have developed a weak interaction-promoted strategy for substrate activation and stereocontrol.10j,l–p In addition, we have realized highly efficient remote stereocontrol in the asymmetric hydrogenation of acyclic β-branched enamides/enol esters and γ-branched allylic amides by using a bisphosphine-Rh catalyst bearing a large bite angle.10h,i,s Therefore, we envisaged that the first efficient asymmetric hydrogenation of 2-substituted dehydromorpholines could be realized by using a similar catalytic system and control strategy. The approach is also expected to be applied to the asymmetric hydrogenation of other challenging substrates for the efficient synthesis of related chiral N-heterocycles.
Results and discussion
Based on the above assumptions, 6-phenyl-3,4-dihydro-2H-1,4-oxazines with different N-substituents (1a-R) were tested in the hydrogenation (Table 1, entries 1–5). The complex of (R,R,R)-SKP with [Rh(cod)2]SbF6, which has been successfully applied in the asymmetric hydrogenation of β-branched enol esters and γ-branched enamides in our previous studies,10h,s,11 was chosen as the catalyst. Dichloromethane (DCM), which is thought to have little coordinating ability, was chosen as the solvent. It was found that the substrate 1a bearing a N-Cbz group gave superior enantioselectivity compared to its analogue 1a-NO2 (entry 1 vs. 2). Other carbamate-substituted substrates 1a-COOiBu, and 1a-Boc also showed high reactivity but relatively lower enantioselectivity (entries 3–4). Changing the N-substituent to a Ts group failed to yield any product (entry 5). The dehydromorpholine 1a was then chosen as the model substrate for further condition optimization. Firstly, several representative chiral diphosphine ligands were evaluated under 50 atm hydrogen pressure at room temperature. Several classic diphosphine ligands possessing large bite angles, including SDP, f-Binaphane and JosiPhos gave positive results (entries 6–8), while others including DTBM-SegPhos, Me-DuPhos, Ph-BPE, and QuinoxP* showed no reactivities (entries 9–12). Secondly, some commonly used solvents were screened using the rhodium complex of (R,R,R)-SKP as catalyst (entries 13–18). Only the aprotic and less polar solvents AcOEt and toluene provided moderate conversions (entries 13–14), while dichloroethane (DCE), MeOH, tetrahydrofuran (THF) and 1,4-dioxane possessing certain coordinating abilities resulted in almost no reaction (entries 15–18). Finally, decreasing the hydrogen pressure reduces the reactivity (entries 19–21). Quantitative conversion with identical enantioselectivity could be obtained under 30 atm hydrogen pressure when the reaction time was prolonged to 24 hours (entry 20).
Table 1 Condition optimization
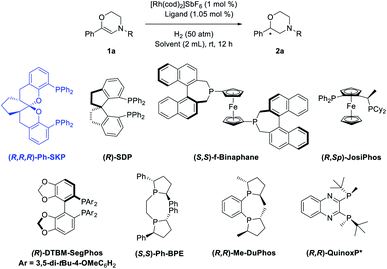
|
Entrya |
R |
Ligand |
Solvent |
Conv.b (%) |
eec (%) |
Conditions: 1a (0.2 mmol), [Rh(cod)2]SbF6 (1 mol%), ligand (1.05 mol%), H2 (50 atm), solvent (2 mL), rt, 12 h, unless otherwise noted.
Conversions were calculated from 1H NMR spectra.
The ee values of 2a were determined by HPLC using chiral columns.
30 atm, 12 h.
30 atm, 24 h.
10 atm, 24 h.
|
1 |
Cbz |
SKP
|
DCM |
>99 |
92 |
2 |
4-NO2-Cbz |
SKP
|
DCM |
>99 |
26 |
3 |
COOiBu |
SKP
|
DCM |
>99 |
89 |
4 |
Boc |
SKP
|
DCM |
>99 |
75 |
5 |
Ts |
SKP
|
DCM |
NR |
— |
6 |
Cbz |
SDP
|
DCM |
24 |
70 |
7 |
Cbz |
f-Binaphane
|
DCM |
>99 |
4 |
8 |
Cbz |
JosiPhos
|
DCM |
97 |
63 |
9 |
Cbz |
DTBM-SegPhos
|
DCM |
NR |
— |
10 |
Cbz |
Me-DuPhos
|
DCM |
NR |
— |
11 |
Cbz |
Ph-BPE
|
DCM |
NR |
— |
12 |
Cbz |
QuinoxP*
|
DCM |
NR |
— |
13 |
Cbz |
SKP
|
AcOEt |
32 |
79 |
14 |
Cbz |
SKP
|
Toluene |
42 |
91 |
15 |
Cbz |
SKP
|
DCE |
<10 |
— |
16 |
Cbz |
SKP
|
MeOH |
<10 |
— |
17 |
Cbz |
SKP
|
THF |
NR |
— |
18 |
Cbz |
SKP
|
Dioxane |
NR |
— |
19d |
Cbz |
SKP
|
DCM |
98 |
92 |
20e |
Cbz |
SKP
|
DCM |
>99 |
92 |
21f |
Cbz |
SKP
|
DCM |
56 |
92 |
Using the optimized reaction conditions of entry 20 in Table 1, a variety of substrates bearing different substituents were examined (Table 2). All the unsaturated morpholines were converted into their corresponding products in quantitative yields and with satisfactory enantioselectivities. Among the 4-substituted substrates 1b–h, the electron-withdrawing trifluoromethyl substituted substrate 1f gave the corresponding product 2f with the highest 94% ee. Among the 3-substituted substrates 1i–l, the electron-donating methoxy substituted substrate 1l gave the corresponding product 2l with the highest 94% ee. It is worth noting that substrates 1m–p bearing a substituent at the 2-position all gave excellent enantioselectivities (99% ee) for both electron-withdrawing and electron-donating species, which can be attributed to the steric effects of the aromatic ring substituents. A similar ortho-effect occurs in the SKP-catalyzed asymmetric hydrogenation of E-allylamides, which points to the same stereocontrol pattern.10s 3,4-Disubstituted unsaturated morpholines 1q and 1r were also subjected to the reaction conditions, affording the corresponding products in high yields with 94% and 88% ees, respectively. When the phenyl ring was changed to either a naphthalene (1s) or thiophene ring (1t), satisfactory yields were also observed, albeit with a comparatively low ee value for the thiophene species. The method also applies to alkyl-substituted substrates (1u–w) which gave the corresponding products with 81%, 58%, and 91% ees, respectively. The absolute configurations of products were assigned to be the same as 2b which was confirmed by single-crystal analysis (see the ESI† for details).
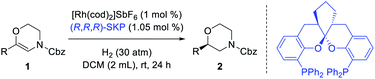
|
Conditions: 1 (0.2 mmol), (R,R,R)-SKP (1.05 mol%), [Rh(cod)2]SbF6 (1 mol%), H2 (30 atm), DCM (2 mL), rt, 24 h. Yields of isolated products are given. The ee values were determined by HPLC using chiral stationary phases.
|
|
To further evaluate the potential application of this methodology, the gram scale hydrogenation of 1a was performed, delivering the desired product 2a in 97% yield and 92% ee. Decreasing the catalyst amount from 1 to 0.2 mol% did not obviously affect the result if the reaction time and temperature were increased (Fig. 3a). Using Pd/C–H2, the Cbz group of the hydrogenated products 2 can be easily removed to obtain the free NH morpholines 3, which can be further applied to the synthesis of bioactive compounds. For instance, deprotection of 2b bearing the electron-withdrawing 4-fluoro substituent afforded the free NH morpholine 3b in 95% yield and 92% ee. Compound 3b can be transformed into 4bvia aromatic nucleophilic substitution, which is the enantiomer of a potent GSK-3β inhibitor (Fig. 3b).12 Another deprotected product 3l bearing an electron-donating 3-methoxy substituent can be further converted to a dopamine 3 receptor agonist 4lvia reductive amination to 3l′ in 75% yield and 88% ee and further demethylation (Fig. 3c).13
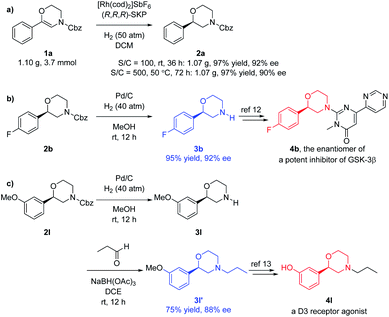 |
| Fig. 3 Scale-up and applications. (a) Gram scale hydrogenation; (b) for the synthesis of a potent GSK-3β inhibitor; (c) for the synthesis of a D3 receptor agonist. | |
A deuterium-labelling experiment was also conducted to reveal the mechanism of this reaction (Fig. 4). When H2 was replaced by D2, two deuterium atoms were substituted for both of the adjacent carbons, which indicates that the hydrogenation only occurs at the C
C bond of the enamide stage.
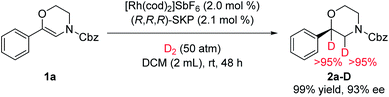 |
| Fig. 4 Deuterium labelling experiment. | |
Conclusions
In summary, we have developed the first asymmetric hydrogenation of 2-substituted dehydromorpholines catalyzed by the SKP–Rh complex bearing a large bite angle. With this method, a variety of 2-substituted chiral morpholines were obtained in quantitative yields and excellent enantioselectivities (up to 99% ee). The reaction can be carried out on a gram scale and the corresponding chiral products could subsequently be transformed into important intermediates required for the preparation of useful drug candidates.
Data availability
All experimental data associated with this work is available in the ESI.†
Author contributions
M. L. conducted most of the experiments and wrote the initial manuscript draft. J. Z. screened the initial reaction conditions. Y. Z. and F. Z. performed part of the experiments. Z. Z. conceived the project and finalized the manuscript. Z. Z. and W. Z. directed the project. All the authors co-wrote the paper. All authors discussed the results and commented on the manuscript.
Conflicts of interest
There are no conflicts to declare.
Acknowledgements
This work was supported by the National Key R&D Program of China (No. 2018YFE0126800), National Natural Science Foundation of China (No. 21620102003, 21831005, 91856106, 21991112, and 22071150), and Shanghai Municipal Education Commission (No. 201701070002E00030). We appreciate Prof. Kuiling Ding for providing SKP ligands. We also thank the Instrumental Analysis Center of Shanghai Jiao Tong University.
Notes and references
- Recent examples:
(a) M. A. Graham, H. Asjey, A. D. Campell, L. Chan, K. G. Cooper, Z. Cui, A. Dalgleish, D. Dave, G. Ensor, M. R. G. Espinosa, P. Hamilton, C. Heffernan, L. V. Jackson, D. Jing, M. F. Jones, P. Liu, K. R. Mulholland, M. Pervez, M. Popadynec, E. Randles, S. Tomasi and S. Wang, Org. Process Res. Dev., 2021, 25, 43–56 CrossRef CAS;
(b) Z. A. Henley, A. Amour, N. Barton, M. Bantscheff, G. Bergamini, S. M. Bertrand, M. Convery, K. Down, B. Dümpelfeld, C. D. Edwards, P. Grandi, P. M. Gore, S. Keeling, S. Livia, D. Mallett, A. Maxwell, M. Price, C. Rau, F. B. M. Reinhard, J. Rowedder, P. Rowland, J. A. Taylor, D. A. Thomas, E. M. Hessel and J. N. Hamblin, J. Med. Chem., 2020, 63, 638–655 CrossRef CAS PubMed;
(c) U. Lücking, L. Wortmann, A. M. Wengner, J. Lefranc, P. Lienau, H. Briem, G. Siemeister, U. Bömer, K. Denner, M. Schäfer, M. Koppitz, K. Eis, F. Bartels, B. Bader, W. Bone, D. Moosmayer, S. J. Holton, U. Eberspächer, J. Grudzinska-Goebel, C. Schatz, G. Deeg, D. Mumberg and F. von Nussbaum, J. Med. Chem., 2020, 63, 7293–7325 CrossRef PubMed;
(d) Y. Chen, X. Yuan, W. Zhang, M. Tang, L. Zheng, F. Wang, W. Yan, S. Yang, Y. Wei, J. He and L. Chen, J. Med. Chem., 2019, 62, 1577–1592 CrossRef CAS PubMed;
(e) S.-M. Yang, M. Yoshioka, J. W. Strovel, D. J. Urban, X. Hu, M. D. Hall, A. Jadhav and D. J. Maloney, Bioorg. Med. Chem. Lett., 2019, 29, 1220–1226 CrossRef CAS PubMed.
-
(a) W. Park, Y. Kim and Y. S. Park, Eur. J. Org. Chem., 2019, 2671–2674 CrossRef CAS;
(b) M. E. Kopach, P. C. Heath, R. B. Scherer, M. A. Pietz, B. A. Astleford, M. K. McCauley, U. K. Singh, S. W. Wong, D. M. Coppert, M. S. Kerr, P. G. Houghton, G. A. Rhodes and G. A. Tharp, Org. Process Res. Dev., 2015, 19, 543–550 CrossRef CAS;
(c) F. C. Sequeira and S. R. Chemler, Org. Lett., 2012, 14, 4482–4485 CrossRef CAS PubMed;
(d) T.-X. Métro, D. G. Pardo and J. Cossy, Chem. Eur. J., 2009, 15, 1064–1070 CrossRef PubMed;
(e) K. E. Henegar, J. Org. Chem., 2008, 73, 3662–3665 CrossRef CAS PubMed;
(f) T.-X. Métro, D. G. Pardo and J. Cossy, J. Org. Chem., 2008, 73, 707–710 CrossRef PubMed.
-
(a) R. Ma and M. C. White, J. Am. Chem. Soc., 2018, 140, 3202–3205 CrossRef CAS PubMed;
(b) W. Yang, Z. Wang and J. Sun, Angew. Chem., Int. Ed., 2016, 55, 6954–6958 CrossRef CAS PubMed;
(c) M. C. O'Reilly and C. W. Lindsley, Org. Lett., 2012, 14, 2910–2913 CrossRef PubMed;
(d) M. Yar, E. M. McGarrigle and V. K. Aggarwal, Org. Lett., 2009, 11, 257–260 CrossRef CAS PubMed.
- Allylic substitution:
(a) C. Qian and W. Tang, Org. Lett., 2020, 22, 4483–4488 CrossRef CAS PubMed;
(b) M. Bandini, M. Monari, A. Romaniello and M. Tragni, Chem. Eur. J., 2010, 16, 14272–14277 CrossRef CAS PubMed;
(c) M. C. Wilkinson, Tetrahedron Lett., 2005, 46, 4773–4775 CrossRef CAS;
(d) K. Ito, Y. Imahayashi, T. Kuroda, S. Eno, B. Saito and T. Katsuki, Tetrahedron Lett., 2004, 45, 7277–7281 CrossRef CAS;
(e) H. Nakano, J.-i. Yokoyama, R. Fujita and H. Hongo, Tetrahedron Lett., 2002, 43, 7761–7764 CrossRef CAS;
(f) A. Yamazaki and K. Achiwa, Tetrahedron: Asymmetry, 1995, 6, 1021–1024 CrossRef CAS;
(g) Y. Uozumi, A. Tanahashi and T. Hayashi, J. Org. Chem., 1993, 58, 6826–6832 CrossRef CAS; Michael addition:
(h) C. Mulet, M. Escolano, S. Llopis, S. Sanz, C. R. de Arellano, M. Sánchez-Roselló, S. Fustero and C. del Pozo, Adv. Synth. Catal., 2018, 360, 2885–2893 CrossRef CAS;
(i) X.-D. Zhai, Z.-D. Yang, Z. Luo and H.-T. Xu, Chin. Chem. Lett., 2017, 28, 1793–1797 CrossRef CAS;
(j) M. Sánchez-Roselló, C. Mulet, M. Guerola, C. del Pozo and S. Fustero, Chem. Eur. J., 2014, 20, 15697–15701 CrossRef PubMed;
(k) S. Fustero, D. Jiménez, J. Moscardó, S. Catalán and C. del Pozo, Org. Lett., 2007, 9, 5283–5286 CrossRef CAS PubMed; Others:
(l) A. Sen, K. Takenaka and H. Sasai, Org. Lett., 2018, 20, 6827–6831 CrossRef CAS PubMed;
(m) P. Zhou, Y. Cai, X. Zhong, W. Luo, T. Kang, J. Li, X. Liu, L. Lin and X. Feng, ACS Catal., 2016, 6, 7778–7783 CrossRef CAS;
(n) M. U. Luescher and J. W. Bode, Angew. Chem., Int. Ed., 2015, 54, 10884–10888 CrossRef CAS PubMed.
-
(a) Y.-P. He, H. Wu, Q. Wang and J. Zhu, J. Am. Chem. Soc., 2021, 143, 7320–7325 CrossRef CAS PubMed;
(b) V. G. Lisnyak, T. Lynch-Colameta and S. A. Snyder, Angew. Chem., Int. Ed., 2018, 57, 15162–15166 CrossRef CAS PubMed;
(c) Y. Y. Lau, H. Zhai and L. L. Schafer, J. Org. Chem., 2016, 81, 8696–8709 CrossRef CAS PubMed;
(d) H. Zhai, A. Borzenko, Y. Y. Lau, S. H. Ahn and L. L. Schafer, Angew. Chem., Int. Ed., 2012, 51, 12219–12223 CrossRef CAS PubMed;
(e) C. Pousset, R. Callens, A. Marinetti and M. Larchevêque, Synlett, 2004, 2766–2770 CrossRef CAS;
(f) R. Kuwano, D. Karube and Y. Ito, Tetrahedron Lett., 1999, 40, 9045–9049 CrossRef CAS.
- Reviews:
(a) J. Wen, F. Wang and X. Zhang, Chem. Soc. Rev., 2021, 50, 3211–3237 RSC;
(b) P. D. Parker, X. Hou and V. M. Dong, J. Am. Chem. Soc., 2021, 143, 6724–6745 CrossRef CAS PubMed;
(c) Z. Zhang, N. A. Butt, M. Zhou, D. Liu and W. Zhang, Chin. J. Chem., 2018, 36, 443–454 CrossRef CAS;
(d) Z. Zhang, N. A. Butt and W. Zhang, Chem. Rev., 2016, 116, 14769–14827 CrossRef CAS PubMed;
(e) D.-S. Wang, Q.-A. Chen, S.-M. Lu and Y.-G. Zhou, Chem. Rev., 2012, 112, 2557–2590 CrossRef CAS PubMed;
(f) J.-H. Xie, S.-F. Zhu and Q.-L. Zhou, Chem. Rev., 2011, 111, 1713–1760 CrossRef CAS PubMed; Recent representative examples:
(g) J. Mas-Roselló, T. Smejkal and N. Cramer, Science, 2020, 368, 1098–1102 CrossRef PubMed;
(h) F.-H. Zhang, F.-J. Zhang, M.-L. Li, J.-H. Xie and Q.-L. Zhou, Nat. Catal., 2020, 3, 621–627 CrossRef CAS;
(i) J. Wang, P.-L. Shao, X. Lin, B. Ma, J. Wen and X. Zhang, Angew. Chem., Int. Ed., 2020, 59, 18166–18171 CrossRef CAS PubMed;
(j) W. Li, T. Wagener, L. Hellmann, C. G. Daniliuc, C. Mück-Lichtenfeld, J. Neugebauer and F. Glorius, J. Am. Chem. Soc., 2020, 142, 7100–7107 CrossRef CAS PubMed;
(k) J. Zheng, J. Jongcharoenkamol, B. B. C. Peters, J. Guhl, S. Ponra, M. S. G. Ahlquist and P. G. Andersson, Nat. Catal., 2019, 2, 1093–1100 CrossRef CAS;
(l) L. Zhang, Y. Tang, Z. Han and K. Ding, Angew. Chem., Int. Ed., 2019, 58, 4973–4977 CrossRef CAS PubMed;
(m) Y. Ge, Z. Han, Z. Wang and K. Ding, J. Am. Chem. Soc., 2019, 141, 8981–8988 CrossRef CAS PubMed;
(n) Q. Yan, G. Xiao, Y. Wang, G. Zi, Z. Zhang and G. Hou, J. Am. Chem. Soc., 2019, 141, 1749–1756 CrossRef CAS PubMed;
(o) J. Chen and W. Zhang, Chin. J. Org. Chem., 2020, 40, 4372–4374 CrossRef CAS.
- Reviews:
(a) ref. 6d,e;
(b) A. Limuro, K. Higashida, H. Nagae and K. Mashima, Heterocycles, 2017, 95, 63–80 CrossRef; Representative examples:
(c) C. Liu, M. Wang, S. Liu, Y. Wang, Y. Peng, Y. Lan and Q. Liu, Angew. Chem., Int. Ed., 2021, 60, 5108–5113 CrossRef CAS PubMed;
(d) W. Li, H. Yang, R. Li, H. Lv and X. Zhang, ACS Catal., 2020, 10, 2603–2608 CrossRef CAS;
(e) Y. Chen, Y. Pan, Y.-M. He and Q.-H. Fan, Angew. Chem., Int. Ed., 2019, 58, 16831–16834 CrossRef CAS PubMed;
(f) Y. Chen, Y.-M. He, S. Zhang, T. Miao and Q.-H. Fan, Angew. Chem., Int. Ed., 2019, 58, 3809–3813 CrossRef CAS PubMed;
(g) T. Touge and T. Arai, J. Am. Chem. Soc., 2016, 138, 11299–11305 CrossRef CAS PubMed;
(h) C. Guo, D.-W. Sun, S. Yang, S.-J. Mao, X.-H. Xu, S.-F. Zhu and Q.-L. Zhou, J. Am. Chem. Soc., 2015, 137, 90–93 CrossRef CAS PubMed;
(i) Y. Duan, L. Li, M.-W. Chen, C.-B. Yu, H.-J. Fan and Y.-G. Zhou, J. Am. Chem. Soc., 2014, 136, 7688–7700 CrossRef CAS PubMed; Also via direct pyridine reduction:
(j) Z.-S. Ye, M.-W. Chen, Q.-A. Chen, L. Shi, Y. Duan and Y.-G. Zhou, Angew. Chem., Int. Ed., 2012, 51, 10181–10184 CrossRef CAS PubMed;
(k) M. Chang, Y. Huang, S. Liu, Y. Chen, S. W. Krska, I. W. Davies and X. Zhang, Angew. Chem., Int. Ed., 2014, 53, 12761–12764 CrossRef CAS PubMed;
(l) X. Wei, B. Qu, X. Zeng, J. Savoie, K. R. Fandrick, J.-N. Desrosiers, S. Tcyrulnikov, M. A. Marsini, F. G. Buono, Z. Li, B.-S. Yang, W. Tang, N. Haddad, O. Gutierrez, J. Wang, H. Lee, S. Ma, S. Campbell, J. C. Lorenz, M. Eckhardt, F. Himmelsbach, S. Peters, N. D. Patel, Z. Tan, N. K. Yee, J. J. Song, F. Roschangar, M. C. Kozlowski and C. H. Senanayake, J. Am. Chem. Soc., 2016, 138, 15473–15481 CrossRef CAS PubMed;
(m) B. Qu, H. P. R. Mangunuru, X. Wei, K. R. Fandrick, J.-N. Desrosiers, J. D. Sieber, D. Kurouski, N. Haddad, L. P. Samankumara, H. Lee, J. Savoie, S. Ma, N. Grinberg, M. Sarvestani, N. K. Yee, J. J. Song and C. H. Senanayake, Org. Lett., 2016, 18, 4920–4923 CrossRef CAS PubMed;
(n) B. Qu, H. P. R. Mangunuru, S. Tcyrulnikov, D. Rivalti, O. V. Zatolochnaya, D. Kurouski, S. Radomkit, S. Biswas, S. Karyakarte, K. R. Fandrick, J. D. Sieber, S. Rodriguez, J.-N. Desrosiers, N. Haddad, K. McKellop, S. Pennino, H. Lee, N. K. Yee, J. J. Song, M. C. Kozlowski and C. H. Senanayake, Org. Lett., 2018, 20, 1333–1337 CrossRef CAS PubMed.
- Other examples for asymmetric hydrogenative synthesis of chiral benzomorpholines with the 3-stereocenter:
(a) S. Wei, X. Feng and H. Du, Org. Biomol. Chem., 2016, 14, 8026–8029 RSC;
(b) J. Qin, F. Chen, Y.-M. He and Q.-H. Fan, Org. Chem. Front., 2014, 1, 952–955 RSC;
(c) J. L. Núñez-Rico and A. Vidal-Ferran, Org. Lett., 2013, 15, 2066–2069 CrossRef PubMed;
(d) N. Arai, Y. Saruwatari, K. Isobe and T. Ohkuma, Adv. Synth. Catal., 2013, 355, 2769–2774 CrossRef CAS;
(e) J. Hu, D. Wang, Z. Zheng and X. Hu, Chin. J. Chem., 2012, 30, 2664–2668 CrossRef CAS;
(f) K. Gao, C.-B. Yu, D.-S. Wang and Y.-G. Zhou, Adv. Synth. Catal., 2012, 354, 483–488 CrossRef CAS;
(g) Y.-G. Zhou, P.-Y. Yang and X.-W. Han, J. Org. Chem., 2005, 70, 1679–1683 CrossRef CAS PubMed; With the 2-stereocenter:
(h) X. Yin, Y. Huang, Z. Chen, Y. Hu, L. Tao, Q. Zhao, X.-Q. Dong and X. Zhang, Org. Lett., 2018, 20, 4173–4177 CrossRef CAS PubMed.
- Recent representative examples:
(a) Y. Lou, J. Wang, G. Gong, F. Guan, J. Lu, J. Wen and X. Zhang, Chem. Sci., 2020, 11, 851–855 RSC;
(b) C. Li, F. Wan, Y. Chen, H. Peng, W. Tang, S. Yu, J. C. McWilliams, J. Mustakis, L. Samp and R. J. Maguire, Angew. Chem., Int. Ed., 2019, 58, 13573–13583 CrossRef CAS PubMed;
(c) H. Zhong, M. R. Friedfeld and P. J. Chirik, Angew. Chem., Int. Ed., 2019, 58, 9194–9198 CrossRef CAS PubMed;
(d) Y.-Q. Guan, Z. Han, X. Li, C. You, X. Tan, H. Lv and X. Zhang, Chem. Sci., 2019, 10, 252–256 RSC;
(e) M. R. Friedfeld, H. Zhong, R. T. Ruck, M. Shevlin and P. J. Chirik, Science, 2018, 360, 888–893 CrossRef CAS PubMed.
- Selected examples:
(a) Y. Liu and W. Zhang, Angew. Chem., Int. Ed., 2013, 52, 2203–2206 CrossRef CAS PubMed;
(b) J. Chen, D. Liu, N. Butt, C. Li, D. Fan, Y. Liu and W. Zhang, Angew. Chem., Int. Ed., 2013, 52, 11632–11636 CrossRef CAS PubMed;
(c) Y. Liu, I. D. Gridnev and W. Zhang, Angew. Chem., Int. Ed., 2014, 53, 1901–1905 CrossRef CAS PubMed;
(d) Q. Hu, Z. Zhang, Y. Liu, T. Imamoto and W. Zhang, Angew. Chem., Int. Ed., 2015, 54, 2260–2264 CrossRef CAS PubMed;
(e) J. Chen, Z. Zhang, D. Liu and W. Zhang, Angew. Chem., Int. Ed., 2016, 55, 8444–8447 CrossRef CAS PubMed;
(f) Q. Hu, J. Chen, Z. Zhang, Y. Liu and W. Zhang, Org. Lett., 2016, 18, 1290–1293 CrossRef CAS PubMed;
(g) S. Chen, M. Zhou, Z. Zhang and W. Zhang, Res. Chem. Intermed., 2017, 43, 6293–6298 CrossRef CAS;
(h) C. Liu, J. Yuan, J. Zhang, Z. Wang, Z. Zhang and W. Zhang, Org. Lett., 2018, 20, 108–111 CrossRef CAS PubMed;
(i) J. Zhang, C. Liu, X. Wang, J. Chen, Z. Zhang and W. Zhang, Chem. Commun., 2018, 54, 6024–6027 RSC;
(j) J. Chen, Z. Zhang, B. Li, F. Li, Y. Wang, M. Zhao, I. D. Gridnev, T. Imamoto and W. Zhang, Nat. Commun., 2018, 9, 5000 CrossRef PubMed;
(k) D. Fan, Y. Liu, J. Jia, Z. Zhang, Y. Liu and W. Zhang, Org. Lett., 2019, 21, 1042–1045 CrossRef CAS PubMed;
(l) B. Li, J. Chen, Z. Zhang, I. D. Gridnev and W. Zhang, Angew. Chem., Int. Ed., 2019, 58, 7329–7334 CrossRef CAS PubMed;
(m) J. Zhang, J. Jia, X. Zeng, Y. Wang, Z. Zhang, I. D. Gridnev and W. Zhang, Angew. Chem., Int. Ed., 2019, 58, 11505–11512 CrossRef CAS PubMed;
(n) Y. Hu, Z. Zhang, J. Zhang, Y. Liu, I. D. Gridnev and W. Zhang, Angew. Chem., Int. Ed., 2019, 58, 15767–15771 CrossRef CAS PubMed;
(o) Y. Hu, J. Chen, B. Li, Z. Zhang, I. D. Gridnev and W. Zhang, Angew. Chem., Int. Ed., 2020, 59, 5371–5375 CrossRef CAS PubMed;
(p) D. Fan, J. Zhang, Y. Hu, Z. Zhang, I. D. Gridnev and W. Zhang, ACS Catal., 2020, 10, 3232–3240 CrossRef CAS;
(q) D. Liu, B. Li, J. Chen, I. D. Gridnev, D. Yan and W. Zhang, Nat. Commun., 2020, 11, 5935 CrossRef CAS PubMed;
(r) Y. Hu, Z. Zhang, Y. Liu and W. Zhang, Angew. Chem., Int. Ed., 2021, 60, 16989–16993 CrossRef CAS PubMed;
(s) J. Zhang, T. Chen, Y. Wang, F. Zhou, Z. Zhang, I. D. Gridnev and W. Zhang, Nat. Sci., 2021, 1, e10021 Search PubMed.
- Developed by Ding:
(a) X. Wang, Z. Han, Z. Wang and K. Ding, Angew. Chem., Int. Ed., 2012, 51, 936–940 CrossRef CAS PubMed;
(b) X. Wang, Z. Han, Z. Wang and K. Ding, Acc. Chem. Res., 2021, 54, 668–684 CrossRef CAS PubMed.
- K. Fukunaga, F. Uehara, K. Aritomo, A. Shoda, S. Hiki, M. Okuyama, Y. Usui, K. Watanabe, K. Yamakoshi, T. Kohara, T. Hanano, H. Tanaka, S. Tsuchiya, S. Sunada, K.-I. Saito, J.-i. Eguchi, S. Yuki, S. Asano, S. Tanaka, A. Mori, K. Yamagami, H. Baba, T. Horikawa and M. Fujimura, Bioorg. Med. Chem. Lett., 2013, 23, 6933–6937 CrossRef CAS PubMed.
- J. Blagg, C. M. N. Allerton, D. V. J. Batchelor, A. D. Baxter, D. J. Burring, C. L. Carr, A. S. Cook, C. L. Nichols, J. Phipps, V. G. Sanderson, H. Verrier and S. Wong, Bioorg. Med. Chem. Lett., 2007, 17, 6691–6696 CrossRef CAS PubMed.
Footnote |
† Electronic supplementary information (ESI) available. CCDC 2095617 and 2095806. For ESI and crystallographic data in CIF or other electronic format see DOI: 10.1039/d1sc04288b |
|
This journal is © The Royal Society of Chemistry 2021 |
Click here to see how this site uses Cookies. View our privacy policy here.