DOI:
10.1039/D1SC03487A
(Edge Article)
Chem. Sci., 2021,
12, 11756-11761
Me3SiSiMe2(OnBu): a disilane reagent for the synthesis of diverse silacycles via Brook- and retro-Brook-type rearrangement†
Received
27th June 2021
, Accepted 31st July 2021
First published on 2nd August 2021
Abstract
Herein, a readily available disilane Me3SiSiMe2(OnBu) has been developed for the synthesis of diverse silacycles via Brook- and retro-Brook-type rearrangement. This protocol enables the incorporation of a silylene into different starting materials, including acrylamides, alkene-tethered 2-(2-iodophenyl)-1H-indoles, and 2-iodobiaryls, via the cleavage of Si–Si, Si–C, and Si–O bonds, leading to the formation of spirobenzosiloles, fused benzosiloles, and π-conjugated dibenzosiloles in moderate to good yields. Preliminary mechanistic studies indicate that this transformation is realized by successive palladium-catalyzed bis-silylation and Brook- and retro-Brook-type rearrangement of silane-tethered silanols.
Introduction
Silacycles have attracted increasing attention because they have shown unique physical, optoelectronic, and physiological properties in medicinal chemistry and materials science.1 In this context, considerable efforts have been devoted to the development of synthetic methods for silacycles, which is a prerequisite for fully discovering their application potentials.2,3 Among them, of particular interest to synthetic chemists is the synthesis of silacycles via the cleavage of C–Si bonds.3 Typical strategies include direct annulation of silicon-based frameworks3a–n and C–Si/C–C bond exchange reaction of small ring systems based on the existence of a high ring strain.3o–r However, these methods generally require transition-metal catalysts to assist the activation of C–Si bonds. Transition-metal-free catalyzed annulation to assemble silacycles by cleaving C–Si bonds is still elusive so far.
The Brook rearrangement enables an intramolecular migration of a silyl group from carbon to oxygen atoms via a hypervalent silicon species,4 which was initially introduced by Brook5 and was demonstrated to be a reversible process.6 Its reverse process, namely retro-Brook rearrangement, can, in turn, be achieved by the transfer of a silyl group from oxygen to carbon atoms (Scheme 1A).7 Obviously, the Brook and retro-Brook rearrangements allow the cleavage of a C–Si bond and the formation of a C–Si bond under transition-metal-free catalysis.8 On the other hand, the existing studies are limited to the translocation of a single silyl group from the starting materials. The migration of two different silyl groups in one event has not been reported so far. In this context, we want to explore the synthesis of silacycles via the migration of two different silyl groups in the Brook and retro-Brook rearrangement. The design and synthesis of silane-tethered silanols is undoubtedly the primary task and challenge to achieve this hypothesis.
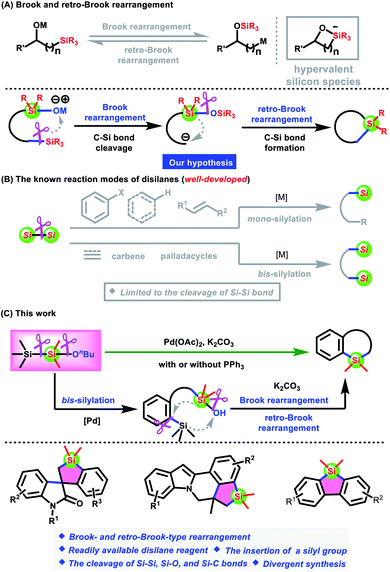 |
| Scheme 1 (A) Brook and retro-Brook rearrangement. (B) The Known reaction modes of disilanes. (C) Our work: a disilane reagent Me3SiSiMe2(OnBu) for the synthesis of diverse silacycles. | |
Disilanes have been among the most versatile silylation reagents in organic synthesis. Over the past few decades, a myriad of methods for accessing organosilanes from disilanes have focused on the activation of Si–Si bonds for the development of mono-silylation involving aryl halides or cyanides, alkenes, and C–H bonds and bis-silylation of alkynes, alkenes, carbenes, and palladacycles (Scheme 1B).9–13 Particularly, the pioneering work that realizes the bis-silylation of in situ generated palladacycles with hexamethyldisilane via the cleavage of Si–Si bonds has recently been reported by Zhang, Cheng, and us.12,13 These advances have inspired us to modify hexamethyldisilane, namely the replacement of the methyl group with an oxygen-containing group, for the synthesis of silane-tethered silanols, which were further converted into silacycles via Brook and retro-Brook-type rearrangement. Herein, we disclose a Brook and retro-Brook-type rearrangement strategy for the synthesis of diverse silacycles, including spirobenzosiloles, fused benzosiloles, and π-conjugated dibenzosiloles, by employing a readily available disilane reagent Me3SiSiMe2(OnBu) that could be prepared by a simple treatment of pentamethylchlorodisilane with n-butanol in the presence of NEt3 at room temperature (Scheme 1C). Notably, the cleavage of Si–Si, Si–C, and Si–O bonds is involved in the transformation.
Results and discussion
We initiated the studies by investigating the reaction of acrylamide 1a with 1-butoxy-1,1,2,2,2-pentamethyldisilane 2a. To our delight, the anticipated spirobenzosilole 3a was indeed afforded in 66% yield by using a simple catalytic system composed of Pd(OAc)2 and K2CO3 in DMF at 90 °C. Encouraged by these initial results, various parameters were screened, and the optimized reaction conditions are as follows: 1a (0.2 mmol), 2a (0.3 mmol), Pd(OAc)2 (10 mol%), PPh3 (20 mol%), and K2CO3 (0.6 mmol) in DMA (2 mL) at 90 °C under N2 for 6 h (see the ESI† for details). Next, several disilanes 2b–h were tested. As shown in Scheme 2, when the n-butyl group of disilane 2a was replaced by other functional groups such as n-hexyl, benzyl, cyclohexyl, and 2-oxopropyl, all of them could afford the desired product 3a, albeit in a lower yield. Unexpectedly, disilane 2f was unreactive. Finally, disiloxane 2g was found to produce product 3a in 58% yield.
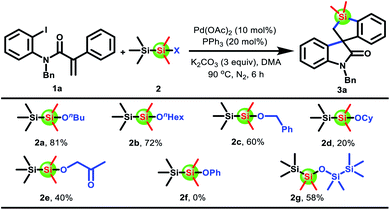 |
| Scheme 2 Screening of disilanes (2). | |
With the optimal reaction conditions and disilane reagent confirmed, the scope of acrylamides 1 was subsequently examined. Gratifyingly, this protocol was applicable to a large variety of acrylamides 1 to afford spiro[benzo[b]silole-3,3′-indolin]-2′-ones 3a–u in moderate to good yields (Scheme 3). Note that the replacement of iodine atoms with bromine atoms on the acrylamide showed good reactivity, delivering the product 3a in 66% yield. Moreover, the three substructures of acrylamides 1 were systematically investigated. Regarding different substituents on the nitrogen atom, methyl or ethyl group substituted acrylamides 1b and 1c were competent substrates, while acrylamide 1d with a Ts group could not give the target product 3d under the standard conditions. For the 2-iodoaniline fragment, a broad range of functional groups on the benzene ring, including electron-donating groups (Me and OMe), modifiable halogen groups (F and Cl), and even strong electron-withdrawing groups (CF3, CO2Me, and NO2), were well tolerated (3e–n). Meanwhile, the structure of 3g was unambiguously confirmed by X-ray crystallography. Their electronic properties seem to affect the reactivity, since substrates 1l–n with strong electron-withdrawing groups, especially CO2Me and NO2 groups, resulted in a diminished yield. Finally, the compatibility was further demonstrated by testing the key 2-phenylalkene moiety. Both the benzene ring containing ortho or para substituents and the naphthalene ring could survive, affording spirobenzosilole 3o–u in moderate to good yields. Nevertheless, a slight modification of the reaction conditions was required when substrates bearing F groups were used (3q and 3t). When the reaction was scaled up to 1 mmol, 70% spirocyclic product 3a could also be obtained.
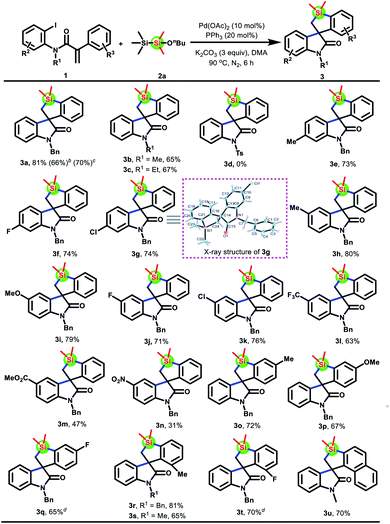 |
| Scheme 3 Variations of the acrylamides (1). aReaction conditions: 1 (0.2 mmol), 2a (0.3 mmol), Pd(OAc)2 (10 mol%), PPh3 (20 mol%), K2CO3 (3 equiv.), and DMA (2 mL) at 90 °C under a N2 atmosphere for 6 h. bN-Benzyl-N-(2-bromophenyl)-2-phenylacrylamide. c1a (1 mmol). d70 °C and 1 h. | |
To highlight the generality of this domino Heck/silacyclization, we envision that fused benzosiloles can be synthesized by a domino Heck/ortho C–H functionalization of aryl iodides. Therefore, 2-(2-iodophenyl)-1-(2-methylallyl)-1H-indole was employed to react with disilane 2a under the above reaction conditions. To our delight, indolo[2,1-a]silolo[4,3,2-de]isoquinolines 5a could smoothly be produced in 58% yield by the cleavage of Si–Si and Si–O bonds. Encouraged by these results, the scope of 2-(2-halophenyl)-1-(2-methylallyl)-1H-indoles was then explored (Scheme 4). Bromine atoms instead of iodine atoms on the substrate 4a′ were subjected to the standard conditions, which could give product 5a, albeit in a lower yield. Satisfactorily, substrates 4b–g being diversely substituted (Me, F, and Cl) on the indole ring were able to undergo this domino Heck/silacyclization with disilane 2a to provide the desired products 5b–g in moderate yields. Differently, using substrate 4h required relatively mild conditions.
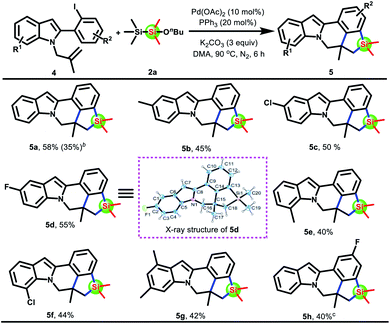 |
| Scheme 4 Variations of the 2-(2-iodophenyl)-1H-indoles chlorides (4). aReaction conditions: 4 (0.2 mmol), 2a (0.3 mmol), Pd(OAc)2 (10 mol%), PPh3 (20 mol%), K2CO3 (3 equiv.), and DMA (2 mL) at 90 °C under a N2 atmosphere for 6 h. b2-(2-Bromophenyl)-1-(2-methylallyl)-1H-indole. c70 °C and 1 h. | |
To emphasize the versatility of the disilane reagent Me3SiSiMe2(OnBu), we next attempted to synthesize π-conjugated dibenzo[b,d]siloles by performing the reaction of 2-iodo-1,1′-biphenyl with Me3SiSiMe2(OnBu) 2a. However, no anticipated product was observed under the above standard conditions. Subsequently, PPh3 was found to suppress the reaction, since dibenzo[b,d]siloles 7a could be afforded in 52% yield by the removal of PPh3. Encouraged by these results, the optimal reaction conditions that could furnish 64% of 7a were established by the screening of various parameters (see the ESI† and Scheme 5). Afterward, a series of substituted 2-iodobiphenyls were examined. Delightfully, the electron-donating group (Me and OMe) on the benzene ring could be tolerated for the silacyclization reaction with disilane 2a, thus delivering the desired products 7b–k in moderate yields. Unfortunately, this protocol was not applicable to substrates 6l–o bearing an electron-withdrawing group. The possible reason is that the protonation of palladacycles formed by substrates 6l–o is easier than bis-silylation (see the ESI†).
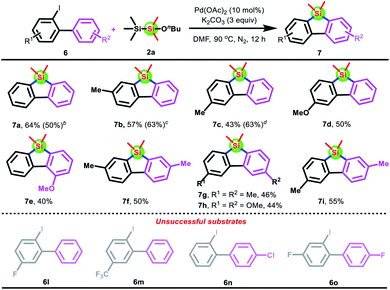 |
| Scheme 5 Variations of the 2-iodobiphenyls (6). aReaction conditions: 6 (0.2 mmol), 2a (0.24 mmol), Pd(OAc)2 (10 mol%), K2CO3 (3 equiv.), and DMF (2 mL) at 90 °C under a N2 atmosphere for 12 h. b2-Bromobiphenyl was used. c2-Iodo-4′-methyl-1,1′-biphenyl was used. d2-Iodo-3′-methyl-1,1′-biphenyl was used. | |
To gain insight into the reaction mechanism, a range of control experiments were performed (Scheme 6). The reaction of acrylamides 1a with disiloxane 2h or 2i could afford product 3a in 27% and 50% yields, respectively, under the optimal reaction conditions (eqn (1)†). Moreover, hexamethyldisiloxane (TMSOTMS) was detected by gas chromatography in the model reaction of 1a with 2a (eqn (2), see the ESI†). These results indicated that the silicon source of product 3a came from the dimethylsilyl group generated by disilane 2avia the cleavage of Si–Si and Si–O bonds. Interestingly, two disilylated products 8a and 8a′ as well as product 3a were isolated in 43%, 27%, and 16% yields when 1a and 2a were reacted under the standard conditions for 20 minutes (eqn (3)†). Therefore, we speculated that two disilylated products 8a and 8a′ were the reaction intermediates (the structure of 8a was absolutely confirmed by X-ray crystallography). Finally, a spiropalladacycle14 that could be prepared from acrylamides and stoichiometric Pd(PPh3)4 was employed for the reaction with disilane 2a (eqn (4)†). Unexpectedly, no product 3b was observed in the absence of Pd(OAc)2 and PPh3. Surprisingly, extra addition of Pd(OAc)2 and PPh3 could give product 3b in 60% yield. These results suggested that the spiropalladacycle as a reaction intermediate underwent transmetalation with intermediate G, rather than direct oxidative addition with disilane 2a, to furnish disilylated products 8a and 8a′, which were then converted into the desired product 3a.
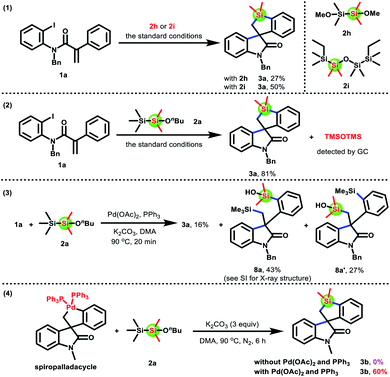 |
| Scheme 6 Mechanistic experiments. | |
To verify our hypothesis and propose the possible formation process of 3a from 8a or 8a′, we conducted several control experiments (Scheme 7). The silacyclization of disilylated products 8a and 8a′, respectively, was conducted in the absence of Pd(OAc)2 and PPh3, and the product 3a was obtained in 66% and 60% yields as expected (eqn (1)†). Besides, TMSOTMS was also detected (see the ESI†). These results demonstrated that the transformation of 8a and 8a′ into 3a involved the cleavage of the Me3Si–C bond, which did not require the assistance of palladium catalysts. On the basis of these results from eqn (1)† and previous work reported by Smith and Takeda,15 we speculated that 8a and 8a′ undergo a Brook- and retro-Brook-type rearrangement to afford 3a (path c and path d). To capture carbanion species F and F′, two common electrophilic reagents, such as iodomethane and benzyl bromide, were added for the silacyclization of 8a and 8a′ (eqn (2) and (3)†). However, the corresponding products F-1 and F′-2 were not observed. Therefore, these results are more favorable to this pathway involving synergetic Brook/retro-Brook-type rearrangement (path d).
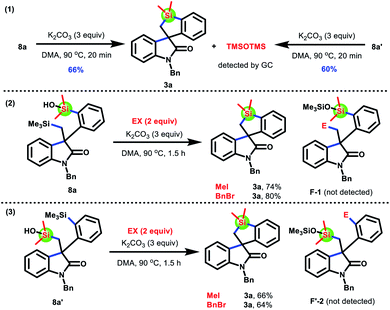 |
| Scheme 7 Mechanistic experiments. The possible process for the transformation of 8a and 8a′ into 3a. | |
Based on the results of mechanistic experiments as well as reported work,12,13,15 a plausible mechanism for the synthesis of silacycles was proposed (Scheme 8). Initially, oxidative addition followed by intramolecular Heck-cyclization of acrylamides 1a to Pd(0) species forms intermediate A, which then undergoes a C–H activation to afford spiropalladacycle B. Next, spiropalladacycle B produces disilylated products 8a/8a′ and regenerates Pd(0) by sequential transmetalation with intermediate G generated by disilane 2a, reductive elimination and further hydrolysis (path a). 8a and 8a′ then undergo a synergetic Brook/retro-Brook-type rearrangement to afford 3a and a trimethylsiloxy anion, which could be converted into TMSOTMS (path d).16 Notably, another possible pathway that synthesizes disilylated products 8a/8a′ by direct oxidative addition of spiropalladacycle B with disilane 2a is ruled out by the results of eqn (4)† (path b).
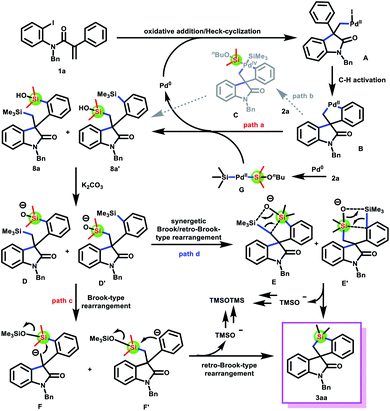 |
| Scheme 8 Possible reaction mechanism. | |
Conclusions
In conclusion, we have disclosed the first example of divergent synthesis of silacycles via a Brook- and retro-Brook-type rearrangement strategy by employing a readily accessible disilane reagent Me3SiSiMe2(OnBu). In this novel transformation, divergent silacycles, such as spirobenzosiloles, fused benzosiloles, and π-conjugated dibenzosiloles, can be produced in moderate to good yields by an unprecedented complex process composed of a bis-silylation of a palladacycle and a Brook- and retro-Brook-type rearrangement. Notably, mechanistic studies reveal that bis-silylation of the palladacycle is completed by a transmetalation process. Further applications of the disilane reagent Me3SiSiMe2(OnBu) and the rearrangement are still in progress in our laboratory.
Data availability
All experimental procedures and spectroscopic data can be found in the ESI.†
Author contributions
Y. X. designed and performed the experiments and analysed the data. W. X., X. C., X. L., H. L., M. Z., X. Y. and G. D. contributed to data analysis and scientific discussion. Y. Y. and Y. L. supervised the project, analyzed the results and wrote the manuscript.
Conflicts of interest
The authors declare no competing interests.
Acknowledgements
The authors thank the National Natural Science Foundation of China (21901071 and 21971061), the Natural Science Foundation of Hunan Province (2020JJ5350), the Scientific Research Fund of Hunan Provincial Education Department (18A002 and 19B359), and the Science and Technology Planning Project of Hunan Province (2018TP1017) for financial support. We thank Prof. Yuanhong Ma (Hunan Normal University) for helpful discussions and Dr Xiaoming Zhu (Hengyang Normal University) for the analysis of X-ray crystallography.
Notes and references
-
(a) A. K. Franz and S. O. Wilson, J. Med. Chem., 2013, 56, 388 CrossRef CAS
;
(b) J. B. Grimm, T. A. Brown, A. N. Tkachuk and L. D. Lavis, ACS Cent. Sci., 2017, 3, 975 CrossRef CAS PubMed
;
(c) R. Ramesh and D. S. Reddy, J. Med. Chem., 2018, 61, 3779 CrossRef CAS PubMed
;
(d) K. Umezawa, M. Kamiya and Y. Urano, Angew. Chem., Int. Ed., 2018, 57, 9346 CrossRef CAS
;
(e) S. J. Barraza and S. E. Denmark, J. Am. Chem. Soc., 2018, 140, 6668 CrossRef CAS PubMed
;
(f) K. L. Chan, M. J. McKiernan, C. R. Towns and A. B. Holmes, J. Am. Chem. Soc., 2005, 127, 7662 CrossRef CAS PubMed
;
(g) M. Akhtaruzzaman, Y. Seya, N. Asao, A. Islam, E. Kwon, A. Han, L. El-Shafei and Y. Yamamoto, J. Mater. Chem., 2012, 22, 10771 RSC
.
- For recent reviews, see:
(a) U. Sharma, R. Sharma, R. Kumar, I. Kumar and B. Singh, Synthesis, 2015, 47, 2347 CrossRef
;
(b) L. Li, Y. Zhang, L. Gao and Z. Song, Tetrahedron Lett., 2015, 56, 1466 CrossRef CAS
;
(c) Y. Yang and C. Wang, Sci. China: Chem., 2015, 58, 1266 CrossRef CAS
;
(d) Z. Xu, W.-S. Huang, J. Zhang and L.-W. Xu, Synthesis, 2015, 47, 3645 CrossRef CAS
;
(e) Q. W. Zhang, K. An and W. He, Synlett, 2015, 9, 1145 Search PubMed
;
(f) T. Komiyama, Y. Minami and T. Hiyama, ACS Catal., 2016, 7, 631 CrossRef
;
(g) W.-T. Zhao, F. Gao and D. Zhao, Synlett, 2018, 29, 2595 CrossRef CAS
;
(h) Q.-C. Mu, J. Chen, C.-G. Xia and L.-W. Xu, Coord. Chem. Rev., 2018, 374, 93 CrossRef CAS
;
(i) S. C. Richter and M. Oestreich, Trends Chem., 2020, 2, 13 CrossRef CAS
.
- For selected examples, see:
(a) T. Matsuda, S. Kadowaki, Y. Yamaguchi and M. Murakami, Chem. Commun., 2008, 2744 RSC
;
(b) M. Tobisu, M. Onoe, Y. Kita and N. Chatani, J. Am. Chem. Soc., 2009, 131, 7506 CrossRef CAS
;
(c) K. Mochida, M. Shimizu and T. Hiyama, J. Am. Chem. Soc., 2009, 131, 8350 CrossRef CAS PubMed
;
(d) E. Shirakawa, S. Masui, R. Narui, R. Watabe, D. Ikeda and T. Hayashi, Chem. Commun., 2011, 47, 9714 RSC
;
(e) Y. Liang, S. Zhang and Z. Xi, J. Am. Chem. Soc., 2011, 133, 9204 CrossRef CAS
;
(f) R. Shintani, K. Moriya and T. Hayashi, J. Am. Chem. Soc., 2011, 133, 16440 CrossRef CAS PubMed
;
(g) Y. Liang, W. Geng, J. Wei and Z. Xi, Angew. Chem., Int. Ed., 2012, 51, 1934 CrossRef CAS
;
(h) M. Onoe, K. Baba, Y. Kim, Y. Kita, M. Tobisu and N. Chatani, J. Am. Chem. Soc., 2012, 134, 19477 CrossRef CAS PubMed
;
(i) Q.-W. Zhang, K. An and W. He, Angew. Chem., Int. Ed., 2014, 53, 5667 CrossRef CAS
;
(j) N. Ishida, W. Ikemoto and M. Murakami, J. Am. Chem. Soc., 2014, 136, 5912 CrossRef CAS PubMed
;
(k) Q.-W. Zhang, K. An, L.-C. Liu, S. Guo, C. Jiang, H. Guo and W. He, Angew. Chem., Int. Ed., 2016, 55, 6319 CrossRef CAS
;
(l) Q.-W. Zhang, K. An, L.-C. Liu, Q. Zhang, H. Guo and W. He, Angew. Chem., Int. Ed., 2017, 56, 1125 CrossRef CAS
;
(m) S. Okumura, F. Sun, N. Ishida and M. Murakami, J. Am. Chem. Soc., 2017, 139, 12414 CrossRef CAS PubMed
;
(n) Q. Yang, L. Liu, Y. Chi, W. Hao, W.-X. Zhang and Z. Xi, Org. Chem. Front., 2018, 5, 860 RSC
;
(o) W. T. Zhao, F. Gao and D. Zhao, Angew. Chem., Int. Ed., 2018, 57, 6329 CrossRef CAS PubMed
;
(p) H. Chen, Y. Chen, X. Tang, S. Liu, R. Wang, T. Hu and Z. Song, Angew. Chem., Int. Ed., 2019, 58, 4695 CrossRef CAS PubMed
;
(q) X. B. Wang, Z. J. Zheng, J. L. Xie, X. W. Gu, Q. C. Mu, G. W. Yin, F. Ye, Z. Xu and L. W. Xu, Angew. Chem., Int. Ed., 2020, 59, 790 CrossRef CAS
;
(r) Y. Qin, J. L. Han, C. W. Ju and D. Zhao, Angew. Chem., Int. Ed., 2020, 59, 8481 CrossRef CAS
.
- For reviews, see:
(a)
A. G. Brook and A. R. Bassendale, in Rearrangements in Ground and Excited States, ed. P. de Mayo, Academic Press, New York, 1980, vol. 2, p. 149 Search PubMed
;
(b)
K. Tomooka, in The Chemistry of Organolithium Compounds, ed. Z. Rappoport and I. Marck, Wiley, Chichester, 2004, vol. 2, p. 749 Search PubMed
;
(c) A. G. Brook, Acc. Chem. Res., 1974, 7, 77 CrossRef CAS
;
(d) W. H. Moser, Tetrahedron, 2001, 57, 2065 CrossRef CAS
;
(e) E. Schaumann and A. Kirschning, Synlett, 2007, 18, 177 CrossRef
;
(f) A. B. Smith III and W. M. Wuest, Chem. Commun., 2008, 5883 RSC
;
(g) A. B. Smith III and C. M. Adams, Acc. Chem. Res., 2004, 37, 365 CrossRef
;
(h) Y. Deng and A. B. Smith III, Acc. Chem. Res., 2020, 53, 988 CrossRef CAS PubMed
.
-
(a) A. G. Brook, J. Am. Chem. Soc., 1958, 80, 1886 CrossRef CAS
;
(b) A. G. Brook, C. M. Warner and M. E. McGriskin, J. Am. Chem. Soc., 1959, 81, 981 CrossRef CAS
;
(c) A. G. Brook and N. V. Schwartz, J. Am. Chem. Soc., 1960, 82, 2435 CrossRef CAS
;
(d) A. G. Brook and B. Iachia, J. Am. Chem. Soc., 1961, 83, 827 CrossRef CAS
.
-
(a) R. West, R. Lowe, H. F. Stewart and A. Wright, J. Am. Chem. Soc., 1971, 93, 282 CrossRef CAS
;
(b) R. J. Linderman and A. Ghannam, J. Am. Chem. Soc., 1990, 112, 2392 CrossRef CAS
;
(c) X. Jiang and W. F. Bailey, Organometallics, 1995, 14, 5704 CrossRef CAS
;
(d) T. Kawashima, K. Naganuma and R. Okazaki, Organometallics, 1998, 17, 367 CrossRef CAS
;
(e) K. Naganuma, T. Kawashima and R. Okazaki, Chem. Lett., 1999, 28, 1139 CrossRef
.
- For selected examples, see:
(a) A. Wright and R. J. West, J. Am. Chem. Soc., 1974, 96, 3214 CrossRef CAS
;
(b) A. Wright and R. West, J. Am. Chem. Soc., 1974, 96, 3227 CrossRef CAS
;
(c) W. C. Still and T. Macdonald, J. Am. Chem. Soc., 1974, 96, 5561 CrossRef CAS
;
(d) R. J. Linderman and A. Ghannam, J. Am. Chem. Soc., 1990, 112, 2392 CrossRef CAS
;
(e) X. L. Jiang and W. F. Bailey, Organometallics, 1995, 14, 5704 CrossRef CAS
;
(f) Y. Mori, Y. Futamura and K. Horisaki, Angew. Chem., Int. Ed., 2008, 47, 1091 CrossRef CAS
.
-
(a) M. E. Jung and C. J. Nichols, J. Org. Chem., 1996, 61, 9065 CrossRef CAS PubMed
;
(b) E. Bures, P. G. Spinazze, G. Beese, I. R. Hunt, C. Rogers and B. A. Keay, J. Org. Chem., 1997, 62, 8741 CrossRef CAS
;
(c) J. Clayden, D. W. Watson and M. Chambers, Tetrahedron, 2005, 61, 3195 CrossRef CAS
.
- For reviews, see:
(a) K. A. Horn, Chem. Rev., 1995, 95, 1317 CrossRef CAS
;
(b) H. K. Sharma and K. H. Pannell, Chem. Rev., 1995, 95, 1351 CrossRef CAS
;
(c) M. Suginome and Y. Ito, J. Chem. Soc., Dalton Trans., 1998, 1925 RSC
;
(d) I. Beletskaya and C. Moberg, Chem. Rev., 1999, 99, 3435 CrossRef CAS PubMed
;
(e) M. Suginome and Y. Ito, Chem. Rev., 2000, 100, 3221 CrossRef CAS PubMed
;
(f) I. Beletskaya and C. Moberg, Chem. Rev., 2006, 106, 2320 CrossRef CAS
;
(g) C. Cheng and J. F. Hartwig, Chem. Rev., 2015, 115, 8946 CrossRef CAS
;
(h) P. Xiao, L. Gao and Z. Song, Chem, 2019, 25, 2407 CrossRef CAS
;
(i) Y. Zhang, B. Zhou and A. Lu, Synlett, 2018, 30, 685 CrossRef
.
- For recent representative examples of mono-silylation, see:
(a) Y. J. Liu, Y. H. Liu, Z. Z. Zhang, S. Y. Yan, K. Chen and B.-F. Shi, Angew. Chem., Int. Ed., 2016, 55, 13859 CrossRef CAS
;
(b) B.-B. Zhan, J. Fan, L. Jin and B.-F. Shi, ACS Catal., 2019, 9, 3298 CrossRef CAS
;
(c) A. Maji, S. Guin, S. Feng, A. Dahiya, V. K. Singh, P. Liu and D. Maiti, Angew. Chem., Int. Ed., 2017, 56, 14903 CrossRef CAS
;
(d) A. Deb, S. Singh, K. Seth, S. Pimparkar, B. Bhaskararao, S. Guin, R. B. Sunoj and D. Maiti, ACS Catal., 2017, 7, 8171 CrossRef CAS
;
(e) H. Saito, K. Nogi and H. Yorimitsu, Angew. Chem., Int. Ed., 2018, 57, 11030 CrossRef CAS PubMed
.
- For recent representative examples of bis-silylation, see:
(a) M. B. Ansell, D. E. Roberts, F. G. Cloke, O. Navarro and J. Spencer, Angew. Chem., Int. Ed., 2015, 54, 5578 CrossRef CAS
;
(b) Z. Liu, H. Tan, T. Fu, Y. Xia, D. Qiu, Y. Zhang and J. Wang, J. Am. Chem. Soc., 2015, 137, 12800 CrossRef CAS
;
(c) M. Ahmad, A. C. Gaumont, M. Durandett and J. Maddaluno, Angew. Chem., Int. Ed., 2017, 56, 2464 CrossRef CAS
;
(d) T. He, L. C. Liu, L. Guo, B. Li, Q. W. Zhang and W. He, Angew. Chem., Int. Ed., 2018, 57, 10868 CrossRef CAS
;
(e) P. H. Xiao, Y. J. Cao, Y. Y. Gui, L. Gao and Z. L. Song, Angew. Chem., Int. Ed., 2018, 57, 4769 CrossRef CAS
.
-
(a) A. Lu, X. Ji, B. Zhou, Z. Wu and Y. Zhang, Angew. Chem., Int. Ed., 2018, 57, 3233 CrossRef CAS
;
(b) X. Ji, F. Wei, B. Wan, C. Cheng and Y. Zhang, Chem. Commun., 2020, 56, 7801 RSC
;
(c) B. Zhou, A. Lu, C. Shao, X. Liang and Y. Zhang, Chem. Commun., 2018, 54, 10598 RSC
;
(d) X. Ma, A. Lu, X. Ji, G. Shi and Y. Zhang, Asian J. Org. Chem., 2018, 7, 1403 CrossRef CAS
;
(e) W. Lv, S. Wen, J. Yu and G. Cheng, Org. Lett., 2018, 20, 4984 CrossRef CAS
;
(f) W. Lv, J. Yu, B. Ge, S. Wen and G. Cheng, J. Org. Chem., 2018, 83, 12683 CrossRef CAS
;
(g) W. Lv, S. Liu, Y. Chen, S. Wen, Y. Lan and G. Cheng, ACS Catal., 2020, 10, 10516 CrossRef CAS
;
(h) M. Wollenburg, J. Bajohr, A. D. Marchese, A. Whyte, F. Glorius and M. Lautens, Org. Lett., 2020, 22, 3679 CrossRef CAS PubMed
.
-
(a) H. Lu, X. Yang, L. Zhou, W. Li, G. Deng, Y. Yang and Y. Liang, Org. Chem. Front., 2020, 7, 2016 RSC
;
(b) W. Li, C. Zhang, H. Lu, Y. Wang, G. Deng, Y. Liang and Y. Yang, Org. Chem. Front., 2020, 7, 2075 RSC
;
(c) W. Li, W. Chen, B. Zhou, Y. Xu, G. Deng, Y. Liang and Y. Yang, Org. Lett., 2019, 21, 2718 CrossRef CAS PubMed
;
(d) Y. Xu, X. Liu, W. Chen, G. Deng, Y. Liang and Y. Yang, J. Org. Chem., 2018, 83, 13930 CrossRef CAS PubMed
;
(e) G. Xiao, L. Chen, G. Deng, J. Liu and Y. Liang, Tetrahedron Lett., 2018, 59, 1836 CrossRef CAS
;
(f) W. Li, G. Xiao, G. Deng and Y. Liang, Org. Chem. Front., 2018, 5, 1488 RSC
.
- H. Yoon, A. Lossouarn, F. Landau and M. Lautens, Org. Lett., 2016, 18, 6324 CrossRef CAS
.
-
(a) A. B. Smith III, R. Tong, W.-S. Kim and W. A. Maio, Angew. Chem., Int. Ed., 2011, 50, 8904 CrossRef PubMed
;
(b) H. Taguchi, K. Tadaki, M. Ghoroku, A. Tsubouchi and T. Takeda, J. Org. Chem., 2002, 67, 8450 CrossRef CAS
;
(c) H. Taguchi, K. Takami, A. Tsubouchi and T. Takeda, Tetrahedron Lett., 2004, 45, 429 CrossRef CAS
.
-
(a) K. J. Shea and D. A. Loy, Acc. Chem. Res., 2001, 34, 707 CrossRef CAS PubMed
;
(b) F. Neumeyer and N. Auner, Chem.–Eur. J., 2016, 22, 1 CrossRef PubMed
;
(c) L. V. Zhilitskaya, N. O. Yarosh, L. G. Shagun, I. A. Dorofeev and L. I. Larina, Mendeleev
Commun., 2017, 27, 352 CrossRef CAS
.
Footnote |
† Electronic supplementary information (ESI) available. CCDC 2040657–2040659. For ESI and crystallographic data in CIF or other electronic format see DOI: 10.1039/d1sc03487a |
|
This journal is © The Royal Society of Chemistry 2021 |