DOI:
10.1039/D1SC02682H
(Edge Article)
Chem. Sci., 2021,
12, 10092-10096
Electrooxidative dearomatization of biaryls: synthesis of tri- and difluoromethylated spiro[5.5]trienones†
Received
14th May 2021
, Accepted 24th June 2021
First published on 24th June 2021
Abstract
Radical spirocyclization via dearomatization has emerged as an attractive strategy for the rapid synthesis of structurally diverse spiro molecules. We report the use of electrochemistry to perform an oxidative dearomatization of biaryls leading to tri- and difluoromethylated spiro[5.5]trienones in a user friendly undivided cell set-up and a constant current mode. The catalyst- and chemical oxidant-free dearomatization procedure features ample scope, and employs electricity as the green and sole oxidant.
Introduction
The development of new approaches for the construction of CF3- or CF2H-containing target molecules is becoming increasingly important owing to the growing applications of organo-fluorinated compounds in inter alia pharmaceutical and agrochemical industries.1–3 Thus, the incorporation of fluoroalkyl groups into organic molecules can significantly improve their physical, chemical, and biological properties, such as permeability, bioavailability, and metabolic stability.4–6 Although a multitude of methods have been developed for the preparation of trifluoromethylated or difluoromethylated compounds by radical addition to unsaturated bonds,7 a protocol for directly introducing CF3 or CF2H onto spiro[5.5]trienone has thus far proven elusive, despite these motifs being prevalent in a number of natural products and bioactive molecules (Fig. 1).
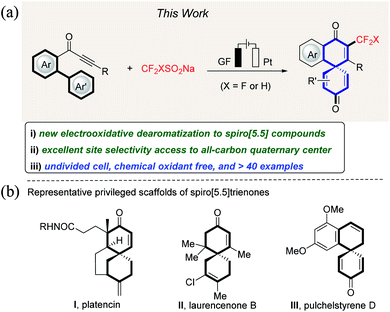 |
| Fig. 1 Electrooxidative biaryl dearomatization: (a) reaction design and (b) selected examples of bioactive spiro compounds. | |
Electrochemistry has surfaced as an attractive technique for the discovery of new modes of reactivity and transformations that are not readily accessible with chemical reagents.8 As radical spirocyclization by dearomatization of biaryls is an attractive strategy for the rapid construction of spiro[5.5] molecules,9,11b we questioned whether the efficiency and mildness noted in the CF3-radical formation could be translated into an electrooxidative biaryl dearomatization strategy. However, significant challenges would need to be overcome (Fig. 1). (1) Regioselectivity between 5-exo-trig, 6-exo-trig and 7-endo-trig cyclization needs to be controlled. (2) The low reactivity of alkynes towards fluoroalkyl radicals needs to be addressed. Compared to the radical addition onto alkenes,10 alkyne functionalization by radical addition is more challenging.11 Herein, we report an unprecedented dearomatization of biarylynones under green and operationally-simple electrochemical conditions. The salient features of our strategy comprise the following: (a) the first efficient dearomatization of biaryls to CF3-containing spiro[5.5]trienones, (b) a most user-friendly undivided cell set-up, (c) simple reaction conditions devoid of a redox mediator, (d) the absence of catalysts and chemical oxidants, and (e) high regioselectivity.
Results and discussion
Optimization of reaction conditions
We initiated our studies by probing various reaction conditions for the envisioned electrochemical dearomatization reaction of biarylynone 1a in a most user-friendly undivided cell set-up (Tables 1 and S2 in the ESI†), using the bench-stable and inexpensive Langlois' reagent (CF3SO2Na, 2a) as the CF3 source. After considerable preliminary experimentation, we observed that the desired trifluoromethylation/dearomatization was accomplished with a mixed solvent system consisting of DCE/MeCN (1
:
1) and Et4NClO4 as the electrolyte (entry 1). Different solvents and a series of supporting electrolytes were tested but found not to be beneficial (entries 2–7). Neither decreasing nor increasing the reaction temperature improved the yield of product 3a (entry 8). In contrast to previous literature reports,12–14 a catalytic redox mediator was not required for this transformation (entries 9 and 10). When the dearomative reaction was performed on a 1 mmol scale, the product 3a was isolated in 50% yield (entry 11). Control experiments confirmed the essential role of electricity for the electrooxidative dearomatization (entry 12). The structure of 3a was unambiguously confirmed by single-crystal X-ray analysis (Scheme 1).15a
Table 1 Optimization of the electrooxidative dearomatizationa
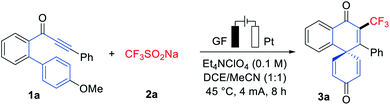
|
Entry |
Variation from standard conditions |
Yieldb/% |
Standard conditions: undivided cell, GF anode, Pt cathode, constant current (CCE) = 4 mA, 1a (0.3 mmol), 2a (0.6 mmol), Et4NClO4 (0.1 M, 0.4 mmol), DCE/MeCN (4 mL), under air, 8 h.
Yield of isolated products.
H2O (1 mL) was added.
30 W, blue LED. GF = graphite felt.
|
1 |
No change |
60 |
2 |
MeCN |
40 |
3 |
MeCN/H2O (2 : 1) |
15 |
4 |
In the absence of Et4NClO4 |
46 |
5 |
n-Bu4NBF4 instead of Et4NClO4 |
20 |
6 |
Et4NPF6 instead of Et4NClO4 |
18 |
7 |
Na2CO3 and H2O instead of Et4NClO4 |
55c |
8 |
At room temperature (25 °C) |
40 |
9 |
Addition of [Mes-Acr]ClO4 (10 mol%) |
50d |
10 |
Addition of Cp2Fe (10 mol%) |
45 |
11 |
1 mmol scale (4 mL solvent) |
50 |
12 |
No electricity |
0 |
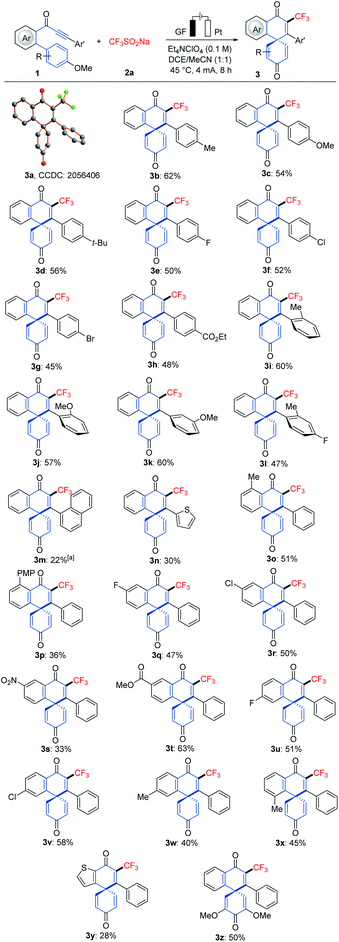 |
| Scheme 1 Scope of trifluoromethylated spiro[5.5]trienones 3. [a] NMR yield. | |
Robustness
With the optimized reaction conditions in hand, we explored the viable scope of the electrochemical transformation. Various biarylynones 1 were investigated and the results are shown in Scheme 1. Generally, the electronic effect of substituents on the arene Ar′ did not influence the reaction efficiency and synthetically useful yields of spiro[5.5]trienones were obtained (3a–3h). We were pleased to find that ortho- and meta-substituted arene Ar′ underwent this transformation efficiently despite a possible steric repulsion (3i–3l), even for polysubstituted substrates. A naphthyl substituent was also compatible (3m). Substitutions on the phenome scaffold were next examined. Substrates bearing either electron-withdrawing or electron-donating groups did not significantly alter the reaction outcome and the spiro compounds 3 were efficiently obtained (3o–3x). As depicted, the dearomatization approach was compatible with several functional groups, such as chloro, bromo, ether, nitro and ester. A heterocyclic substrate was also applicable in this electrooxidative transformation to afford the corresponding product 3y in a moderate yield. It is noteworthy that a substrate with a substituent at the ortho-position of OMe (1z) also formed the desired compound 3z.16
As the difluoromethyl group (CF2H) can serve as a hydrogen bond donor, it has been employed as a lipophilic isostere in drug design for functionalities such as amides, alcohols, thiols and hydroxamic acids.17 Thus, we turned our attention to electrochemical difluoromethylation/dearomatization with differently substituted biarylynones 1 and CF2HSO2Na (2b). Similarly, the electronic properties of arenes Ar and Ar′ in substrate 1 did not have distinct influence on the efficiency of the dearomatization, and moderate to good yields of the spiro[5.5]trienones 4 were obtained (Scheme 2). The structure of product 4o was unambiguously verified by X-ray crystallographic analysis.15b
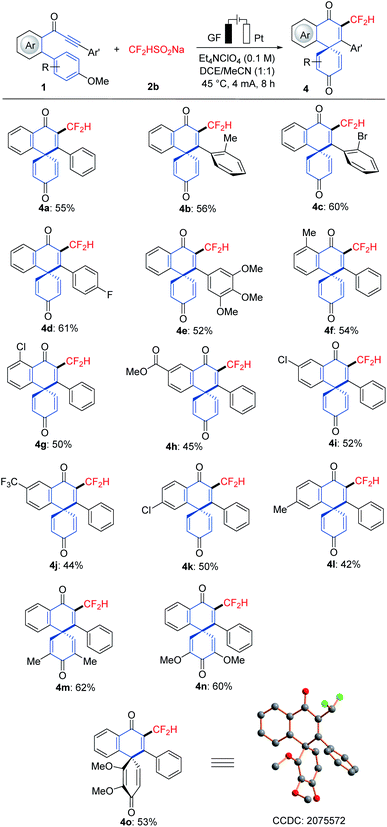 |
| Scheme 2 Scope of difluoromethylated spiro[5.5]trienones 4. | |
We further demonstrated the synthetic utility and showed that the trifluoromethylated spiro[5.5]trienones are a versatile framework that can be readily transformed into more value-added scaffolds in single step reactions (Scheme 3). The biologically relevant epoxyquinone 5 could be obtained in excellent yield via oxidation, while reduction of the ketone selectively furnished alcohol 6. Alcohol 7 was synthesized by selective addition of Grignard reagent, while alkene 8 could be prepared by a Wittig reaction.
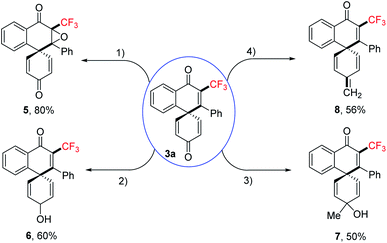 |
| Scheme 3 Reaction conditions: (1) H2O2 (1.5 equiv.), Na2CO3 (1.5 equiv.), EtOH, 45 °C, 2 h. (2) DIBAL (1.0 equiv.), THF, −78 °C, 3 h. (3) CH3MgCl (2.0 equiv.), THF, room temperature, 3 h. (4) Methyltriphenylphosphonium bromide (2.0 equiv.), t-BuOK (2.0 equiv.), THF, room temperature, 6 h. | |
To gain mechanistic insight into this electrochemical dearomatization reaction, the radical clock reaction using (1-cyclopropylvinyl)benzene (1′) as the radical-trapping reagent provided the known product 9 in 25% yield (Fig. 2a). Based on these results, a radical mechanism is proposed in Fig. 2c for this electrooxidative dearomatization reaction. First, radical CF3˙ is generated from 2a through anodic oxidation. Radical addition of CF3˙ to C–C triple bonds of 1a affords a vinyl radical A, which undergoes 6-exo-trig cyclization to the radical species B. Intermediate B is reoxidized to oxocarbenium ion C at the anode. Finally, the corresponding product 3a is formed after demethylation. The incorporation of the diflouro moiety proceeds via the same mechanism, but the oxidation peak of CF2HSO2Na was found much lower than that of CF3SO2Na by a cyclic voltammetry (CV) experiment.18 In addition, inspired by the pioneering work of Jiao for 1,2-dichloroethane (DCE) dehydrochlorination,19 we also found that when the model reaction between 1a and 2a was performed under the standard conditions, the reaction mixture clearly became acidic as shown by pH measurement (in ESI†). Hence, we propose that HCl was generated from the dehydrochlorination of DCE. Notably, the analogue of ion C could be trapped by water while changing the reaction solvent system with 1-(4′-(tert-butyl)-[1,1′-biphenyl]-2-yl)-3-phenylprop-2-yn-1-one 1f′. The trapping product 10 was here isolated in 30% yield (Fig. 2b).
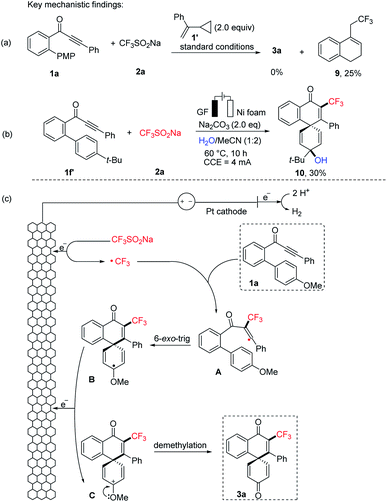 |
| Fig. 2 Mechanistic investigation of the electrooxidative biaryl dearomatization: (a) radical clock experiment, (b) trapping experiment for the key intermediate, and (c) proposed mechanism. | |
Conclusions
In summary, we have developed an efficient approach for the electrochemical dearomatization of biaryls under metal catalyst- and chemical oxidant-free conditions. This green and environmentally friendly strategy showed a broad scope and great compatibility with sensitive functional groups, affording a series of CF3- and CF2H-substituted spiro[5.5]trienones in moderate to good yields. It represents the first electrooxidative 6-exo-trig radical dearomative spirocyclization process, and a plausible mechanism was proposed.
Data availability
All experimental data, procedures for data analysis and pertinent data sets are provided in the ESI.
Author contributions
Y. Z. and L. A. conceived the project. Y. Z. and C. M. performed the experiments, Y. Z., J. F. and J. S. analyzed and interpreted the experimental data. Y. Z. and J. S. drafted the paper. G. Z., Y. Z. and L. A. supervised the project. All of the authors discussed the results and contributed to the preparation of the final manuscript.
Conflicts of interest
There are no conflicts to declare.
Acknowledgements
Generous support by the National Natural Science Foundation of China (No. 21702188) and by the DFG (Gottfried-Wilhelm-Leibniz award to LA) is gratefully acknowledged.
Notes and references
-
(a) K. Haranahalli, T. Honda and I. Ojima, J. Fluorine Chem., 2019, 217, 29–40 CrossRef CAS PubMed;
(b) S. Purser, P. R. Moore, S. Swallow and V. Gouverneur, Chem. Soc. Rev., 2008, 37, 320–330 RSC;
(c) K. Müller, C. Faeh and F. Diederich, Science, 2007, 317, 1881 CrossRef PubMed;
(d) M. Shimizu and T. Hiyama, Angew. Chem., Int. Ed., 2005, 44, 214–231 CrossRef CAS.
- J. Peter, ChemBioChem, 2004, 5, 570 CrossRef.
-
(a) M. Reichel and K. Karaghiosoff, Angew. Chem., Int. Ed., 2020, 59, 12268–12281 CrossRef CAS PubMed;
(b) A. Harsanyi and G. Sandford, Green Chem., 2015, 17, 2081–2086 RSC;
(c)
P. Kirsch, Modern Fluoroorganic Chemistry, Wiley-VCH, Weinheim, Germany, 2013 CrossRef;
(d)
J. P. Begue and D. Bonnet-Delpon, Bioorganic and Medicinal Chemistry of Fluorine, Wiley, Hoboken, 2008 CrossRef.
- J. A. Erickson and I. J. McLoughlin, J. Org. Chem., 1995, 60, 1626–1631 CrossRef CAS.
- C. A. Veale, P. R. Bernstein, C. M. Bohnert, F. J. Brown, C. Bryant, J. R. Damewood Jr, R. Earley, S. W. Feeney, P. D. Edwards, B. Gomes, J. M. Hulsizer, B. J. Kosmider, R. D. Krell, G. Moore, T. W. Salcedo, A. Shaw, D. S. Silberstein, G. B. Steelman, M. Stein, A. Strimpler, R. M. Thomas, E. P. Vacek, J. C. Williams, D. J. Wolanin and S. Woolson, J. Med. Chem., 1997, 40, 3173–3181 CrossRef CAS.
- N. A. Meanwell, J. Med. Chem., 2011, 54, 2529–2591 CrossRef CAS PubMed.
- For selected reviews, see:
(a) C. Alonso, E. M. De Marigorta, G. Rubiales and F. Palacios, Chem. Rev., 2015, 115, 1847 CrossRef CAS;
(b) L. Chu and F.-L. Qing, Acc. Chem. Res., 2014, 47, 1513 CrossRef CAS PubMed;
(c) S. Barata-Vallejo, B. Lantaño and A. Postigo, Chem.–Eur. J., 2014, 20, 16806 CrossRef CAS;
(d) H. Egami and M. Sodeoka, Angew. Chem., Int. Ed., 2014, 53, 8294 CrossRef CAS;
(e) E. Merino and C. Nevado, Chem. Soc. Rev., 2014, 43, 6598 RSC;
(f) P. Chen and G. Liu, Synthesis, 2013, 45, 2919 CrossRef CAS;
(g) A. Studer, Angew. Chem., Int. Ed., 2012, 51, 8950 CrossRef CAS PubMed.
- For reviews on electrochemical synthesis, see:
(a) C. Zhu, N. W. J. Ang, T. H. Meyer, Y. Qiu and L. Ackermann, ACS Cent. Sci., 2021, 7, 415–431 CrossRef CAS PubMed;
(b) J. C. Siu, N. Fu and S. Lin, Acc. Chem. Res., 2020, 53, 547–560 CrossRef CAS PubMed;
(c) L. Ackermann, Acc. Chem. Res., 2020, 53, 84–104 CrossRef CAS PubMed;
(d) G. M. Martins, G. C. Zimmer, S. R. Menders and N. Ahmed, Green Chem., 2020, 22, 4849–4870 RSC;
(e) P. Xiong and H.-C. Xu, Acc. Chem. Res., 2019, 52, 3339–3350 CrossRef CAS;
(f) Y. Jiang, K. Xu and C. Zeng, Chem. Rev., 2018, 118, 4485–4540 CrossRef CAS PubMed;
(g) Q.-L. Yang, P. Fang and T.-S. Mei, Chin. J. Chem., 2018, 36, 338–352 CrossRef CAS;
(h) M. D. Kärkäs, Chem. Soc. Rev., 2018, 47, 5786–5865 RSC;
(i) N. Sauermann, T. H. Meyer, Y. Qiu and L. Ackermann, ACS Catal., 2018, 8, 7086–7103 CrossRef CAS;
(j) K. D. Moeller, Chem. Rev., 2018, 118, 4817–4833 CrossRef CAS PubMed;
(k) M. Yan, Y. Kawamata and P. S. Baran, Chem. Rev., 2017, 117, 13230–13319 CrossRef CAS PubMed;
(l) A. Jutand, Chem. Rev., 2008, 108, 2300–2347 CrossRef CAS PubMed.
- Access to spiro[5.5] compounds through radical addition:
(a) T. Lanza, R. Leardini, M. Minozzi, D. Nanni, P. Spagnolo and G. Zanardi, Angew. Chem., Int. Ed., 2008, 47, 9439–9442 CrossRef CAS PubMed;
(b) F. G.-L. de Turiso and D. P. Curran, Org. Lett., 2005, 7, 151–154 CrossRef PubMed.
- For selected representative examples of radical addition to alkenes, see:
(a) T. Patra, P. Bellotti, F. Strieth-Kalthoff and F. Glorius, Angew. Chem., Int. Ed., 2020, 59, 3172–3177 CrossRef CAS;
(b) Y. Yang, C.-H. Xu, Z.-Q. Xiong and J.-H. Li, Chem. Commun., 2020, 56, 9549–9552 RSC;
(c) R. Wei, H. Xiong, C. Ye, Y. Li and H. Bao, Org. Lett., 2020, 22, 3195–3199 CrossRef CAS PubMed;
(d) L. Wu, F. Wang, P. Chen and G. Liu, J. Am. Chem. Soc., 2019, 141, 1887–1892 CrossRef CAS PubMed;
(e) N. Fu, L. Song, J. Liu, Y. Shen, J. C. Siu and S. Lin, J. Am. Chem. Soc., 2019, 141, 14480–14485 CrossRef CAS PubMed;
(f) M. A. Ashley, C. Yamauchi, J. C. K. Chu, S. Otsuka, H. Yorimitsu and T. Rovis, Angew. Chem., Int. Ed., 2019, 58, 4002–4006 CrossRef CAS;
(g) Y. Jiao, M.-F. Chiou, Y. Li and H. Bao, ACS Catal., 2019, 9, 5191–5197 CrossRef CAS;
(h) K.-Y. Ye, G. Pombar, N. Fu, G. S. Sauer, I. Keresztes and S. Lin, J. Am. Chem. Soc., 2018, 140, 2438–2441 CrossRef CAS PubMed;
(i) L. Fu, S. Zhou, X. Wan, P. Chen and G. Liu, J. Am. Chem. Soc., 2018, 140, 10965–10969 CrossRef CAS PubMed;
(j) A. Tlahuext-Aca, R. A. Garza-Sanchez, M. Schäfer and F. Glorius, Org. Lett., 2018, 20, 1546–1549 CrossRef CAS PubMed;
(k) L. Zhang, G. Zhang, P. Wang, Y. Li and A. Lei, Org. Lett., 2018, 20, 7396–7399 CrossRef CAS PubMed;
(l) N. Fu, G. S. Sauer, A. Saha, A. Loo and S. Lin, Science, 2017, 357, 575–579 CrossRef CAS PubMed;
(m) A. J. Musacchio, B. C. Lainhart, X. Zhang, S. G. Naguib, T. C. Sherwood and R. R. Knowles, Science, 2017, 355, 729–730 CrossRef PubMed;
(n) Z.-L. Li, X.-H. Li, N. Wang, N.-Y. Yang and X.-Y. Lin, Angew. Chem., Int. Ed., 2016, 55, 15100–15104 CrossRef CAS PubMed;
(o) L. Huang, L. Ye, X.-H. Li, Z.-L. Li, J.-S. Lin and X.-Y. Liu, Org. Lett., 2016, 18, 5384–5388 CrossRef PubMed;
(p) Z. Gu, H. Zhang, P. Xu, Y. Cheng and C. Zhu, Adv. Synth. Catal., 2015, 357, 3057–3063 CrossRef CAS;
(q) J.-Y. Wang, Y.-M. Su, F. Yin, Y. Bao, X. Zhang, Y.-M. Xu and X.-S. Wang, Chem. Commun., 2014, 50, 4108–4111 RSC;
(r) C. Feng and T.-P. Loh, Angew. Chem., Int. Ed., 2013, 52, 12414–12417 CrossRef CAS;
(s) C.-J. Wallentin, J. D. Nguyen, P. Finkbeiner and C. R. J. Stephenson, J. Am. Chem. Soc., 2012, 134, 8875–8884 CrossRef CAS PubMed;
(t) A. Studer, Angew. Chem., Int. Ed., 2012, 51, 8950–8958 CrossRef CAS PubMed.
- Selected examples of radical additions onto internal alkynes:
(a) J. Hua, Z. Fang, J. Xu, M. Bian, C. Liu, W. He, N. Zhu, Z. Yang and K. Guo, Green Chem., 2019, 21, 4706–4711 RSC;
(b) P. Xiong, H.-H. Xu, J. Song and H.-C. Xu, J. Am. Chem. Soc., 2018, 140, 2460–2464 CrossRef CAS PubMed;
(c) Y. Zhang, J. Zhang, B. Hu, M. Ji, S. Ye and G. Zhu, Org. Lett., 2018, 20, 2988–2992 CrossRef CAS PubMed;
(d) Y. Zhang, D. Guo, S. Ye, Z. Liu and G. Zhu, Org. Lett., 2017, 19, 1302–1305 CrossRef CAS PubMed;
(e) H.-L. Hua, B.-S. Zhang, Y.-T. He, Y.-F. Qiu, J.-Y. Hu, Y.-C. Yang and Y.-M. Liang, Chem. Commun., 2016, 52, 10396–10399 RSC;
(f) W. Fu, M. Zhu, G. Zou, C. Xu, Z. Wang and B. Ji, J. Org. Chem., 2015, 80, 4766–4770 CrossRef CAS PubMed;
(g) J. Xu, Y.-L. Wang, T.-J. Gong, B. Xiao and Y. Fu, Chem. Commun., 2014, 50, 12915–12918 RSC;
(h) G.-C. Ge, X.-J. Huang, C.-H. Ding, S.-L. Wan, L.-X. Dai and X.-H. Hou, Chem. Commun., 2014, 50, 3048–3051 RSC;
(i) P. Gao, Y.-W. Shen, R. Fang, X.-H. Hao, Z.-H. Qiu, F. Yang, X.-B. Yan, Q. Wang, X.-J. Gong, X.-Y. Liu and Y.-M. Liang, Angew. Chem., Int. Ed., 2014, 53, 7629–7633 CrossRef CAS PubMed;
(j) Y.-P. Xiong, M.-Y. Wu, X.-Y. Zhang, C.-L. Ma, L. Huang, L.-J. Zhao, B. Tan and X.-Y. Liu, Org. Lett., 2014, 16, 1000–1003 CrossRef CAS PubMed;
(k) Y.-L. Ji, J.-H. Lin, J.-C. Xiao and Y. C. Gu, Org. Chem. Front., 2014, 1, 1280–1284 RSC.
- L. Zhu, P. Xiong, Z.-Y. Mao, Y.-H. Wang, X. Yan, X. Lu and H.-C. Xu, Angew. Chem., Int. Ed., 2016, 55, 2226–2229 CrossRef CAS PubMed.
- Z. Zhang, L. Zhang, Y. Cao, F. Li, G. Bai, G. Liu, Y. Yang and F. Mo, Org. Lett., 2019, 21, 762–766 CrossRef CAS PubMed.
- Z. Ruan, Z. Huang, Z. Xu, G. Mo, X. Tian, X.-Y. Yu and L. Ackermann, Org. Lett., 2019, 21, 1237–1240 CrossRef CAS PubMed.
-
(a) CCDC 2056406.†;
(b) CCDC 2075572.†.
- Under otherwise identical reaction conditions, 1-(4′-methoxy-[1,1′-biphenyl]-2-yl)-4,4-dimethylpent-2-yn-1-one and 1-(4′-methoxy-[1,1′-biphenyl]-2-yl)hept-2-yn-1-one gave thus far less satisfactory results..
- Z. Zou, W. Zhang, Y. Wang, L. Kong, G. Karotsis, Y. Wang and Y. Pan, Org. Lett., 2019, 21, 1857–1862 CrossRef CAS.
-
(a) X. Wang, S. Niu, L. Xu, C. Zhang, L. Meng, X. Zhang and D. Ma, Org. Lett., 2017, 19, 246–249 CrossRef CAS PubMed;
(b) A. J. Reay, L. A. Hammarback, J. T. W. Bray, T. Sheridan, D. Turnbull, A. C. Whitwood and I. J. S. Fairlamb, ACS Catal., 2017, 7, 5174–5179 CrossRef CAS;
(c) T. J. Osberger, D. C. Rogness, J. T. Kohrt, A. F. Stepan and M. C. White, Nature, 2016, 537, 214–219 CrossRef CAS;
(d) B. Mondal, B. Roy and U. Kazmaier, J. Org. Chem., 2016, 81, 11646–11655 CrossRef CAS;
(e) B. V. S. Reddy, L. R. Reddy and E. J. Corey, Org. Lett., 2006, 8, 3391–3394 CrossRef CAS PubMed.
- Y. Liang, F. Lin, Y. Adeli, R. Jin and N. Jiao, Angew. Chem., Int. Ed., 2019, 58, 4566–4570 CrossRef CAS PubMed.
Footnote |
† Electronic supplementary information (ESI) available: Experimental details and characterization of all new compounds and details of DFT calculations. CCDC 2056406 and 2075572. For ESI and crystallographic data in CIF or other electronic format see DOI: 10.1039/d1sc02682h |
|
This journal is © The Royal Society of Chemistry 2021 |
Click here to see how this site uses Cookies. View our privacy policy here.