DOI:
10.1039/D1SC01891D
(Edge Article)
Chem. Sci., 2021,
12, 8778-8783
Carbene-catalyzed enantioselective annulation of dinucleophilic hydrazones and bromoenals for access to aryl-dihydropyridazinones and related drugs†
Received
6th April 2021
, Accepted 17th May 2021
First published on 17th May 2021
Abstract
4,5-Dihydropyridazinones bearing an aryl substituent at the C6-position are important motifs in drug molecules. Herein, we developed an efficient protocol to access aryl-dihydropyridazinone molecules via carbene-catalyzed asymmetric annulation between dinucleophilic arylidene hydrazones and bromoenals. Key steps in this reaction include polarity-inversion of aryl aldehyde-derived hydrazones followed by chemo-selective reaction with enal-derived α,β-unsaturated acyl azolium intermediates. The aryl-dihydropyridazinone products accessed by our protocol can be readily transformed into drugs and bioactive molecules.
1. Introduction
4,5-Dihydropyridazinones and their derivatives, especially those bearing aryl substituents at the 6-position, display a broad range of pharmacological activities.1 Some members of this family have already made their way into the market, such as Levosimendan2 and Pimobendan3 which are used for the treatment of heart disease of humans and animals respectively (Fig. 1a). Other members of this family, such as (−)-KCA 1490 (bonchodilatory and anti-inflammatory activity),4 Meribendan (potent PDE III inhibitor),5 and (R)-DNMDP (cancer cytotoxic modulator),6 have shown promising activities in biological studies (Fig. 1a). Traditionally, these structural motifs can be accessed by condensation of substituted γ-keto carboxylic acids or their derivatives with hydrazines (Fig. 1b), whereas asymmetric access relies on either chiral resolution of the products4,5,7 or the use of optically enriched starting materials.8 It is worth noting that access to chiral starting materials (1,4-dicarbonyl compounds) used in these syntheses remains challenging in organic chemistry.9 Another approach to construct these heterocyclic moieties involves formal [4 + 2] cycloaddition between in situ generated 3-aryl azoalkenes and two carbon synthons (Fig. 1b).10 One asymmetric example of these formal [4 + 2] reactions was demonstrated using isothiourea as the organic catalyst to control enantioselectivity at the C4-position.10a There is no success in catalytic asymmetric access to C5 stereogenic center (Fig. 1a) of these 4,5-dihydropyridazinones.
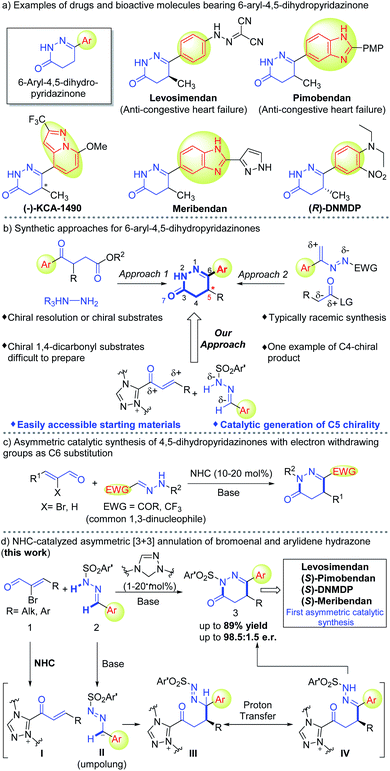 |
| Fig. 1 Strategies to access 6-aryl-4,5-dihydropyridazinones. | |
We're interested in designing N-heterocyclic carbene-catalyzed reactions for the preparation or modification of bioactive molecules for medicinal and agriculture uses.11 Herein, we disclose carbene catalyzed asymmetric annulation between N-monosubstituted arylidene hydrazones (precursor of 1,3-dinucleophile) and bromoenals12 (common 1,3-dinucleophilic hydrazones from trifluoroacetaldehyde and glyoxal derivatives13 have been explored in NHC-catalyzed reactions13c,d as demonstrated in Fig. 1c) to afford highly enantiopure 6-aryl-4,5-dihydropyridazinones (Fig. 1d). N-Monosubstituted hydrazones containing an electron-withdrawing group (e.g., –COR, –CF3)13 at azomethine carbon have been used widely as 1,3-dinucleophiles after the pioneering study by Vicario14 in 2012. In contrast, N-monosubstituted hydrazones from aryl aldehydes rarely showed such character as 1,3-dinucleophiles. This is probably because of the comparatively less stable anionic character at azomethine carbon and an unfordable tendency for proton transfer to regenerate a N-centered nucleophile (Fig. 1d). Therefore, aryl aldehyde-derived N-monosubstituted hydrazones were barely used as precursors of effective 1,3-dinucleophiles in asymmetric catalysis.13g,15 Key steps in our reaction involve the Umpolung of arylidene hydrazone (2) to form intermediate II followed by chemo-selective 1,4-addition to NHC-bound α,β-unsaturated acyl azolium I to form intermediate III. Intermediate III upon intramolecular proton transfer leads to intermediate IV that undergoes cyclization to form 6-aryl-4,5-dihydropyridazinone products with good yields and high enantioselectivities. A broad range of hydrazones from aryl and heteroaryl aldehydes were well tolerated under our reaction conditions. Straightforward transformations of our products lead to clinically approved drugs (Levosimendan and Pimobendan) and several other bioactive molecules without any erosion of the e.r. values (Scheme 3). It is worth mentioning that previously reported procedures to access these drugs and bioactive molecules comprised longer steps and used chiral resolutions or chiral starting materials.4–6,7c–f,8e
2. Results and discussion
We initiated our studies using readily available α-bromo cinnamaldehyde 1a and p-nosyl protected hydrazone 2a as the model substrates to search for suitable precatalysts and reaction conditions, with key results briefed in Table 1 (and also see ESI†). The aminoindanol-derived precatalyst with an N-phenyl substituent (A)16 led to the formation of the desired product 3a in moderate yield and enantioselectivity (Table 1, entry 1). Installing a nitro (–NO2) group in the aminoindanol moiety of precatalyst A (to get precatalyst B)17 improved the enantioselectivity, albeit with poor yield (Table 1, entry 2). Replacing the N-phenyl substituent of precatalyst A with an electron-rich and bulky N-mesityl substituent (to get precatalyst C)18 gave the product in improved yield (74%), although the e.r. value dropped down to 71
:
29 (Table 1, entry 3). As expected, switching to more sterically hindered NHC precatalyst D19 with a bromo atom on the aminoindanol motif further boosted the e.r. value, although a diminishing yield was obtained (Table 1, entry 4). Ultimately, we found out that the use of nitro (–NO2) substituted aminoindanol scaffold containing NHC precatalyst E19 could give the desired product in 72% yield and 95
:
5 e.r (Table 1, entry 5). In the hope for further improvement, we replaced the mesityl group of E with the bulky 1,3,5-triisopropyl phenyl group (to get precatalyst F).19 However, poorer enantioselectivity and yield were detected (Table 1, entry 6). Screening of other solvents showed no additional encouraging improvement in the enantioselectivity of our reaction (Table 1, entry 7 and see ESI†). Both organic and inorganic bases were efficient in providing the desired product (Table 1, entries 8–10 and also see ESI†). After further screening of the precatalyst loading parameter, we established that the optimal conditions consist of ethyl acetate as the solvent and TMEDA as the base in the presence of 10 mol% precatalyst loading, providing the corresponding product without any change in the reaction outcomes (comparing entry 5 and entry 11). Further decrease in the precatalyst loading (5 mol%) resulted in low yield without affecting the enantioselectivity (Table 1, entry 12).
Table 1 Reaction condition optimizationa
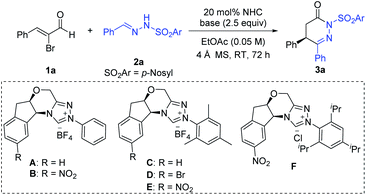
|
Entry |
Conditions |
Yieldb (%) |
e.r.c |
Reaction conditions: 1a (0.1 mmol.), 2a (0.05 mmol), NHC precat. (20 mol%), base (2.5 equiv.), solvent (0.05 M), 4Å MS (100 mg ml−1) at RT for 72 h.
Yields determined by 1H NMR analysis with 1,3,5-trimethoxybenzene as the internal standard.
The e.r. value was determined via chiral-phase HPLC analysis.
See ESI.
Isolated yield was given in parentheses.
10 mol% precatalyst and 0.075 mmol of 1a were used.
5 mol% precatalyst was used. MS = molecular sieves, TMEDA = tetramethylethylenediamine.
|
1 |
A, TMEDA |
44 |
78.5 : 21.5 |
2 |
B, TMEDA |
21 |
90 : 10 |
3 |
C, TMEDA |
74 |
71 : 29 |
4 |
D, TMEDA |
64 |
87 : 13 |
5 |
E, TMEDA |
72 |
95 : 5 |
6 |
F, TMEDA |
48 |
89.5 : 10.5 |
7 |
E, TMEDA, solventsd |
32–77 |
92.5 : 7.5–94 : 6 |
8 |
E, K2CO3 |
72 |
94 : 6 |
9 |
E, K3PO4 |
67 |
93.5 : 6.5 |
10 |
E, Cs2CO3 |
90 |
93 : 7 |
11 |
E, TMEDA |
72(71)e,f |
95 : 5
|
12 |
E, TMEDA |
57g |
95 : 5 |
With the optimized reaction conditions in hand (Table 1, entry 11), we moved to evaluate the generality of this reaction. The scope of various hydrazones was examined first with α-bromo cinnamaldehyde (1a) as the model substrate and the results are shown in Scheme 1. Different kinds of substituted phenyl rings [such as halogens, –OAc, –N(Boc)2 and methyl groups at the ortho, meta, and para positions] at the azomethine carbon of hydrazones were all tolerated and gave the desired products in good yields and excellent e.r. values (3a–h). Interestingly, gram-scale synthesis of 3b with 1 mol% precatalyst loading could furnish the product in 74% yield and 96
:
4 e.r. The phenyl ring of 2a could also be replaced with 1-napthyl and 2-napthyl without affecting the yield and e.r. value of the products (3i–j) significantly. A diverse set of heteroaryls such as 2-benzothiophenyl, 2-thiophenyl, 3-thiophenyl and 3-pyridyl at the azomethine carbon of hydrazones afforded the desired products in excellent yield and e.r. (3k–3n). The absolute configuration of 3n was confirmed by X-ray analysis.20 Most of the time p-nosyl (p-Ns) protected hydrazones gave our desired products in excellent outcomes; unfortunately, similar hydrazones bearing a highly electron-deficient phenyl ring at the azomethine position were degraded under our catalytic conditions. Thus, tosyl protected hydrazones (2o–2p) were used in our reaction, giving the products (3o–3p) in good yields and excellent enantioselectivities. Surprisingly, vinyl substituted hydrazone 2q was also compatible to provide the corresponding product (3q) in 75% yield and 98.5
:
1.5 e.r.
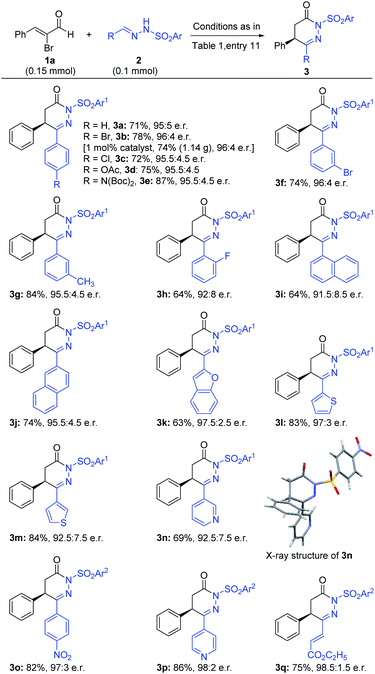 |
| Scheme 1 Scope of hydrazone substrates.aReaction conditions as in Table 1, entry 11. Yields (after silica gel chromatography purification) based on 2. Reaction time 72–84 h. The e.r. value was determined via chiral-phase HPLC analysis. SO2Ar1 = p-nosyl, SO2Ar2 = tosyl. | |
We next examined the scope of bromoenals, and the results are summarized in Scheme 2. Different kinds of electron-donating and withdrawing substituents at the β-phenyl ring (such as methyl, halogens, and methoxyl at the ortho, meta, and para positions) of the bromoenal worked well under our reaction conditions and gave the annulated products in good to excellent yields and high enantioselectivities (4a–4g). The β-phenyl ring of the bromoenal could also be replaced with the 2-napthyl ring without affecting the result significantly (4h). As a 5-methyl substituent in 6-aryl-4,5-dihydropyridazinone is crucial from the viewpoint of existing drugs and bioactive molecules2–6 (Fig. 1a), we further examined the reaction of α-bromocrotonaldehyde with some hydrazones. For example, hydrazone containing 2-fluoro phenyl for azomethine substitution was coupled with α-bromocrotonaldehyde to obtain the product 4i in moderate yield and excellent enantioselectivity. With the aim to prepare precursors of clinical drugs and bioactive molecules utilizing our methodology, products 4j and 4k were obtained in moderate to good yields and excellent enantioselectivities by coupling corresponding hydrazones with α-bromocrotonaldehyde. Notably, in the case of 4jo-nosyl protected hydrazone was used over p-nosyl protected hydrazone due to its instability under our reaction conditions and the reagents were added inside a glove box, as we found the hydrazone decomposed under our reaction conditions and hence resulted in lower yield, when the reagents were added outside. Higher homologues of α-bromocrotonaldehyde, for example, β-ethyl substituted bromoenal have also been used to couple with hydrazone derived from 4-nitrobenzaldehyde to give product 4l in good yield and excellent enantioselectivity. Notably, in the case of 4l tosyl protected hydrazone instead of nosyl protected hydrazone was used, to avoid the decomposition of the hydrazone under the reaction conditions.
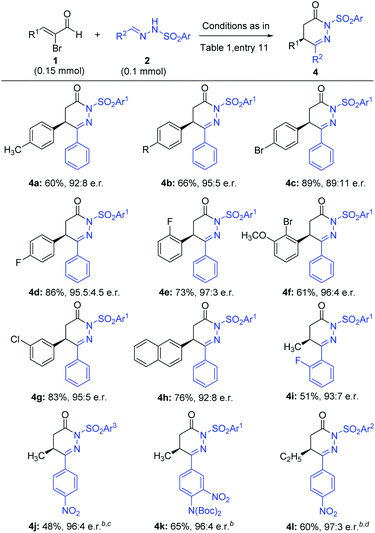 |
| Scheme 2 Scope of α-bromoenal substrates.aReaction conditions as in Table 1, entry 11. Yields (after silica gel chromatography purification) based on 2. Reaction time 72–84 h. The e.r. value was determined via chiral-phase HPLC analysis. SO2Ar1 = p-nosyl, SO2Ar2 = tosyl, SO2Ar3 = o-nosyl. bNaOAc and THF were used as the base and solvent, respectively. cReagents were added in a glovebox. The reaction was carried out at 0 °C for 24 h with 20 mol% precatalyst and 0.2 mmol bromoenal. d0.2 mmol bromoenal was used. | |
A rationale for the reaction stereoselectivity is illustrated in Fig. 2 for product 3n based on the absolute configuration of 3n. The Si-face of the NHC-bound α,β-unsaturated acyl azolium is blocked by the catalyst and specifically the presence of the nitro (–NO2) group on the catalyst makes the Si-face less available for the nucleophile. So, it is more favourable to intercept the NHC-bound α,β-unsaturated acyl azolium from the Re-face resulting in the formation of the (R)-3a stereoselectively.
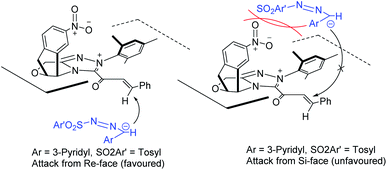 |
| Fig. 2 Proposed TS model for stereoselectivity. | |
The chiral 4,5-dihydropyridazinone molecules from our catalytic reaction were readily transformed to marketed drugs and other bioactive molecules (Scheme 3). For example, intermediate 5b, the precursor of Levosimendan (clinical drug), was obtained without any degradation of the e.r. value from optically enriched 4j (96
:
4 e.r.) through thiophenol mediated nosyl deprotection, followed by Pd/C-catalyzed hydrogenation (Scheme 3a).7c Successive deprotection of the p-nosyl group and two Boc groups of the product 4k (96
:
4 e.r.) gave the intermediate 6b in high yield without any erosion of the e.r. value (Scheme 3b). Intermediate 6b is the precursor for cancer cytotoxic modulator DNMDP6, drug Pimobendan8e,21 and bioactive Meribendan5,21 (Scheme 3b).
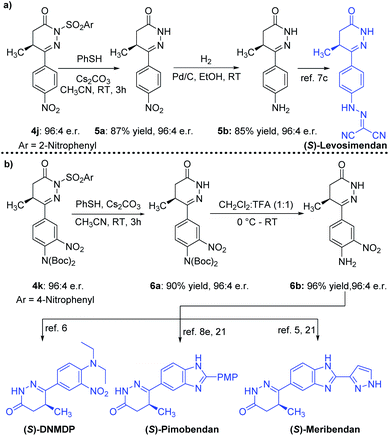 |
| Scheme 3 Synthesis of marketed-drugs and bioactive molecules. | |
3. Conclusions
In summary, we have developed a carbene-catalyzed enantioselective formal [3 + 3] annulation strategy for the construction of 6-aryl-4,5-dihydropyridazinones. The reaction proceeds via the Umpolung of aryl aldehyde-derived hydrazones followed by chemoselective 1,4-addition to the NHC-bound acyl azolium intermediate. A broad scope of functional groups is well tolerated on both of the bromoenal and hydrazone substrates, with all the corresponding products afforded in good to excellent yields and enantioselectivities. Scalable synthesis with low precatalyst loading (1 mol%) could also give the annulated products in good results. Applications of our reaction products allow enantiomeric access to marketed drugs and bioactive molecules.
Author contributions
B. Mondal conducted most of the experiments and wrote the initial manuscript draft. R. Maiti, X. Yang, J. Xu, and J.-L. Yan performed part of the experiments. W. Tian and X. Li contributed to product design regarding potential bioactivities. Y. R. Chi conceptualized and directed the project and finalized the manuscript draft. All authors contributed to discussions.
Conflicts of interest
There are no conflicts to declare.
Acknowledgements
We thank Dr Yongxin Li (NTU) for assistance with X-ray structure analysis. We acknowledge financial support from the Singapore National Research Foundation under its NRF Investigatorship (NRF-NRFI2016-06) and Competitive Research Program (NRF-CRP22-2019-0002); the Ministry of Education, Singapore, under its MOE AcRF Tier 1 Award (RG108/16, RG5/19, RG1/18), MOE AcRF Tier 2 (MOE2019-T2-2-117), MOE AcRF Tier 3 Award (MOE2018-T3-1-003); the Agency for Science, Technology and Research (A*STAR) under its A*STAR AME IRG Award (A1783c0008, A1783c0010); GSK-EDB Trust Fund; Nanyang Research Award Grant, Nanyang Technological University; the National Natural Science Foundation of China (21772029, 21801051, 21807019, 21961006, 22071036, 22061007, 82360589, 81360589), Frontiers Science Center for Asymmetric Synthesis and Medicinal Molecules, Department of Education, Guizhou Province [Qianjiaohe KY number (2020)004]; The 10 Talent Plan (Shicengci) of Guizhou Province ([2016]5649); the Science and Technology Department of Guizhou Province ([2018]2802, [2019]1020); the National Natural Science Foundation of China (No. 31960546); the Program of Introducing Talents of Discipline to Universities of China (111 Program, D20023) at Guizhou University; the Guizhou Province First-Class Disciplines Project [(Yiliu Xueke Jianshe Xiangmu)-GNYL(2017)008], NSFC Grant (81360589), Guizhou University of Traditional Chinese Medicine (China); and Guizhou University.
Notes and references
- For selected reviews, see:
(a) R. Bansal and S. Thota, Med. Chem. Res., 2013, 22, 2539–2552 CrossRef CAS;
(b) S. Dubey and P. A. Bhosle, Med. Chem. Res., 2015, 24, 3579–3598 CrossRef CAS; for selected examples, see:
(c) R. Bansal, D. Kumar, R. Carron and C. de la Calle, Eur. J. Med. Chem., 2009, 44, 4441–4447 CrossRef CAS PubMed;
(d) M. Thyes, H. D. Lehmann, J. Gries, H. Koenig, R. Kretzschmar, J. Kunze, R. Lebkuecher and D. Lenke, J. Med. Chem., 1983, 26, 800–807 CrossRef CAS PubMed;
(e) S. Demirayak, A. C. Karaburun, I. Kayagil, K. Erol and B. Sirmagul, Arch. Pharmacal Res., 2004, 27, 13–18 CrossRef CAS PubMed;
(f) S. Ovais, K. Javed, S. Yaseen, R. Bashir, P. Rathore, R. Yaseen, A. D. Hameed and M. Samim, Eur. J. Med. Chem., 2013, 67, 352–358 CrossRef CAS PubMed;
(g) J. Singh, V. Saini, A. Kumar and R. Bansal, Bioorg. Chem., 2017, 71, 201–210 CrossRef CAS PubMed;
(h) D. Sharma and R. Bansal, Med. Chem. Res., 2016, 25, 1574–1589 CrossRef CAS.
- For selected examples, see:
(a) Z. Papp, I. Édes, S. Fruhwald, S. G. De Hert, M. Salmenperä, H. Leppikangas, A. Mebazaa, G. Landoni, E. Grossini, P. Caimmi, A. Morelli, F. Guarracino, R. H. G. Schwinger, S. Meyer, L. Algotsson, B. G. Wikström, K. Jörgensen, G. Filippatos, J. T. Parissis, M. J. G. González, A. Parkhomenko, M. B. Yilmaz, M. Kivikko, P. Pollesello and F. Follath, Int. J. Cardiol., 2012, 159, 82–87 CrossRef PubMed;
(b) M. S. Nieminen, S. Fruhwald, L. M. A. Heunks, P. K. Suominen, A. C. Gordon, M. Kivikko and P. Pollesello, Heart Lung Vessel, 2013, 5, 227–245 CAS;
(c) A. Mebazaa, M. S. Nieminen, M. Packer, A. Cohen-Solal, F. X. Kleber, S. J. Pocock, R. Thakkar, R. J. Padley, P. Põder, M. Kivikko and f. t. SURVIVE Investigators, JAMA, 2007, 297, 1883–1891 CrossRef CAS PubMed.
- For selected examples, see:
(a) J. Brett, C. Wylie and J. Brown, Clin. Toxicol., 2020, 58, 146–148 CrossRef PubMed;
(b) M. Baron Toaldo, M. Pollesel and A. Diana, J. Vet. Cardiol., 2020, 28, 37–47 CrossRef CAS PubMed.
- K. Ochiai, N. Ando, K. Iwase, T. Kishi, K. Fukuchi, A. Ohinata, H. Zushi, T. Yasue, D. R. Adams and Y. Kohno, Bioorg. Med. Chem. Lett., 2011, 21, 5451–5456 CrossRef CAS PubMed.
- R. Jonas, M. Klockow, I. Lues, H. Prücher, H. J. Schliep and H. Wurziger, Eur. J. Med. Chem., 1993, 28, 129–140 CrossRef CAS.
- L. de Waal, T. A. Lewis, M. G. Rees, A. Tsherniak, X. Wu, P. S. Choi, L. Gechijian, C. Hartigan, P. W. Faloon, M. J. Hickey, N. Tolliday, S. A. Carr, P. A. Clemons, B. Munoz, B. K. Wagner, A. F. Shamji, A. N. Koehler, M. Schenone, A. B. Burgin, S. L. Schreiber, H. Greulich and M. Meyerson, Nat. Chem. Biol., 2016, 12, 102–108 CrossRef CAS PubMed.
- For selected examples, see:
(a) R. L. Hudkins, A. L. Zulli, R. Dandu, M. Tao, K. A. Josef, L. D. Aimone, R. C. Haltiwanger, Z. Huang, J. A. Lyons, J. R. Mathiasen, R. Raddatz and J. A. Gruner, Bioorg. Med. Chem. Lett., 2012, 22, 1504–1509 CrossRef CAS PubMed;
(b) S. Jakkaraj, V. G. Young Jr. and G. I. Georg, Tetrahedron, 2018, 74, 2769–2774 CrossRef CAS PubMed;
(c)
H. Singhania, EP3424908A1, 2019;
(d)
M. Harjula, I. Larma, S. Antila and L. Lehtonen, WO 99/16443, 1999;
(e)
W. Yan, X. Qi, S. Zhao and N. Liu, CN104418810A, 2015;
(f) L. Cheng, J. Cai, Q. Fu and Y. Ke, J. Sep. Sci., 2019, 42, 2482–2490 CrossRef CAS PubMed.
- For selected examples, see:
(a) Y. Nomoto, H. Takai, T. Ohno, K. Nagashima, K. Yao, K. Yamada, K. Kubo, M. Ichimura, A. Mihara and H. Kase, J. Med. Chem., 1996, 39, 297–303 CrossRef CAS PubMed;
(b) T. Seki, A. Kanada, T. Nakao, M. Shiraiwa, H. Asano, K. Miyazawa, T. Ishimori, N. Minami, K. Shibata and K. Yasuda, Chem. Pharm. Bull., 1998, 46, 84–96 CrossRef CAS PubMed;
(c) A. Kojima and Y. Kohno, Tetrahedron, 2013, 69, 1658–1662 CrossRef CAS;
(d) B. A. Provencher, K. J. Bartelson, Y. Liu, B. M. Foxman and L. Deng, Angew. Chem., Int. Ed., 2011, 50, 10565–10569 CrossRef CAS PubMed;
(e)
S. Li, CN107344933A, 2017;
(f) F. F. Owings, M. Fox, C. J. Kowalski and N. H. Baine, J. Org. Chem., 1991, 56, 1963–1966 CrossRef CAS.
- For selected examples, see:
(a) Y. Kuang, K. Wang, X. Shi, X. Huang, E. Meggers and J. Wu, Angew. Chem., Int. Ed., 2019, 58, 16859–16863 CrossRef CAS PubMed;
(b) M. Z. Liang and S. J. Meek, Angew. Chem., Int. Ed., 2019, 58, 14234–14239 CrossRef CAS PubMed;
(c) D. Kaldre, I. Klose and N. Maulide, Science, 2018, 361, 664–667 CrossRef CAS PubMed;
(d) S. Huang, L. Kötzner, C. K. De and B. List, J. Am. Chem. Soc., 2015, 137, 3446–3449 CrossRef CAS PubMed.
-
(a) X. Li, K. Gai, Z. Yuan, J. Wu, A. Lin and H. Yao, Adv. Synth. Catal., 2015, 357, 3479–3484 CrossRef CAS;
(b) W. C. Yuan, B. X. Quan, J. Q. Zhao, Y. You, Z. H. Wang and M. Q. Zhou, J. Org. Chem., 2020, 85, 11812–11821 CrossRef CAS PubMed;
(c) W. Yin, L. Fang, Z. Wang, F. Gao, Z. Li and Z. Wang, Org. Lett., 2019, 21, 7361–7364 CrossRef CAS PubMed;
(d) L. Duan, X. Wang, Y. Gu, Y. Hou and P. Gong, Org. Chem. Front., 2020, 7, 2307–2312 RSC.
-
(a) B. Liu, R. Song, J. Xu, P. K. Majhi, X. Yang, S. Yang, Z. Jin and Y. R. Chi, Org. Lett., 2020, 22, 3335–3338 CrossRef CAS PubMed;
(b) J. Sun, F. He, Z. Wang, D. Pan, P. Zheng, C. Mou, Z. Jin and Y. R. Chi, Chem. Commun., 2018, 54, 6040–6043 RSC;
(c) Y. Liu, Q. Chen, C. Mou, L. Pan, X. Duan, X. Chen, H. Chen, Y. Zhao, Y. Lu, Z. Jin and Y. R. Chi, Nat. Commun., 2019, 10, 1675 CrossRef PubMed;
(d) Y. Liu, P. K. Majhi, R. Song, C. Mou, L. Hao, H. Chai, Z. Jin and Y. R. Chi, Angew. Chem., Int. Ed., 2020, 59, 3859–3863 CrossRef CAS PubMed.
- For reviews, see:
(a) D. Enders, O. Niemeier and A. Henseler, Chem. Rev., 2007, 107, 5606–5655 CrossRef CAS;
(b) D. M. Flanigan, F. Romanov-Michailidis, N. A. White and T. Rovis, Chem. Rev., 2015, 115, 9307–9387 CrossRef CAS;
(c) X. Chen, H. Wang, Z. Jin and Y. R. Chi, Chin. J. Chem., 2020, 38, 1167–1202 CrossRef CAS; for selected examples, see:
(d) J. Guin, S. De Sarkar, S. Grimme and A. Studer, Angew. Chem., Int. Ed., 2008, 47, 8727–8730 CrossRef CAS;
(e) S. De Sarkar and A. Studer, Angew. Chem., Int. Ed., 2010, 49, 9266–9269 CrossRef CAS PubMed;
(f) S. De Sarkar, S. Grimme and A. Studer, J. Am. Chem. Soc., 2010, 132, 1190–1191 CrossRef CAS PubMed;
(g) A. Levens, A. Ametovski and D. W. Lupton, Angew. Chem., Int. Ed., 2016, 55, 16136–16140 CrossRef CAS PubMed;
(h) X. Y. Chen, Z. H. Gao and S. Ye, Acc. Chem. Res., 2020, 53, 690–702 CrossRef CAS PubMed;
(i) A. Ghosh and A. T. Biju, Angew. Chem., Int. Ed., 2021, 60, 2–15 CrossRef;
(j) H. Ohmiya, ACS Catal., 2020, 10, 6862–6869 CrossRef CAS;
(k) S. Mondal, S. R. Yetra, S. Mukherjee and A. T. Biju, Acc. Chem. Res., 2019, 52, 425–436 CrossRef CAS PubMed;
(l) T. K. Das and A. T. K. Biju, Chem. Commun., 2020, 56, 8537–8552 RSC;
(m) X. Wu, B. Liu, Y. Zhang, M. Jeret, H. Wang, P. Zheng, S. Yang, B. A. Song and Y. R. Chi, Angew. Chem., Int. Ed., 2016, 55, 12280–12284 CrossRef CAS;
(n) X. Wu, L. Zhou, R. Maiti, C. Mou, L. Pan and Y. R. Chi, Angew. Chem., Int. Ed., 2019, 58, 477–481 CrossRef CAS PubMed.
-
(a) A. Das, C. M. R. Volla, I. Atodiresei, W. Bettray and M. Rueping, Angew. Chem., Int. Ed., 2013, 52, 8008–8011 CrossRef CAS PubMed;
(b) C. M. R. Volla, A. Das, I. Atodiresei and M. Rueping, Chem. Commun., 2014, 50, 7889–7892 RSC;
(c) J.-H. Mao, Z.-T. Wang, Z.-Y. Wang and Y. Cheng, J. Org. Chem., 2015, 80, 6350–6359 CrossRef CAS PubMed;
(d) C.-L. Zhang, D.-L. Wang, K.-Q. Chen and S. Ye, Org. Biomol. Chem., 2015, 13, 11255–11262 RSC;
(e) X. Xu, P. Y. Zavalij, W. Hu and M. P. Doyle, J. Org. Chem., 2013, 78, 1583–1588 CrossRef CAS PubMed;
(f) J.-N. Zhu, W.-K. Wang, J. Zheng, H.-P. Lin, Y.-X. Deng and S.-Y. Zhao, J. Org. Chem., 2019, 84, 11032–11041 CrossRef CAS PubMed;
(g) W. Wu, X. Yuan, J. Hu, X. Wu, Y. Wei, Z. Liu, J. Lu and J. Ye, Org. Lett., 2013, 15, 4524–4527 CrossRef CAS PubMed.
- M. Fernández, U. Uria, J. L. Vicario, E. Reyes and L. Carrillo, J. Am. Chem. Soc., 2012, 134, 11872–11875 CrossRef PubMed.
-
(a) A. C. Mantovani, T. A. C. Goulart, D. F. Back and G. Zeni, Chem.–Eur. J., 2014, 20, 12663–12668 CrossRef CAS;
(b) I. R. Siddiqui, Rahila, P. Rai, H. Sagir and M. A. Waseem, RSC Adv., 2015, 5, 52355–52360 RSC.
- C. D. Campbell, C. Concellón and A. D. Smith, Tetrahedron: Asymmetry, 2011, 22, 797–811 CrossRef CAS.
- S. Kuwano, S. Harada, B. Kang, R. Oriez, Y. Yamaoka, K. Takasu and K. Yamada, J. Am. Chem. Soc., 2013, 135, 11485–11488 CrossRef CAS PubMed.
- M. He, J. R. Struble and J. W. Bode, J. Am. Chem. Soc., 2006, 128, 8418–8420 CrossRef CAS PubMed.
- C. Zhao, F. Li and J. Wang, Angew. Chem., Int. Ed., 2016, 55, 1820–1824 CrossRef CAS.
- CCDC 2062761 (3n) contains the supplementary crystallographic data for this paper.†.
-
Q. Tong, J. Chen, X. Cui, Z. Gong and B. Zhang, CN107522663A, 2017.
Footnote |
† Electronic supplementary information (ESI) available. CCDC 2062761. For ESI and crystallographic data in CIF or other electronic format see DOI: 10.1039/d1sc01891d |
|
This journal is © The Royal Society of Chemistry 2021 |
Click here to see how this site uses Cookies. View our privacy policy here.