DOI:
10.1039/D0SC05755J
(Edge Article)
Chem. Sci., 2021,
12, 1745-1749
Tri-insertion with dearomatization of terminal arylalkynes using a carborane based frustrated Lewis pair template†
Received
19th October 2020
, Accepted 27th November 2020
First published on 28th November 2020
Abstract
Intramolecular vicinal Frustrated Lewis Pairs (FLPs) have played a significant role in the activation of small molecules, and their stabilities and reactivities are found to strongly depend on the nature of the bridging units. This work reports a new carborane based FLP, 1-PPh2-2-BPh2-1,2-C2B10H10 (2), which reacts with an equimolar amount of p-R2NC6H4C
CH (R = Me, Et, Ph) at room temperature to give C
C triple bond addition products 1,2-[PPh2C(R2NC6H4)
CHBPh2]-1,2-C2B10H10 (3) in high yields. Compounds 3 react further with two equiv. of p-R2NC6H4C
CH (R = Me, Et) at 60–70 °C to give unprecedented stereoselective tri-insertion products, 3,3a,6,6a-tetrahydronaphtho[1,8a-b]borole tricycles (4), in which one of the aryl rings from arylacetylene moieties has been dearomatized with the formation of four stereocenters including one quaternary carbon center. It is noted that the phosphine unit functions as a catalyst during the reactions. After trapping and structural characterization of a key intermediate, a reaction mechanism is proposed, involving sequential alkyne insertion and 1,2-boryl migration.
Introduction
Frustrated Lewis Pairs (FLPs), in which Lewis acid/base adducts are not formed due to steric hindrance, can activate a variety of small molecules.1 After the first example of H2 activation by FLPs,2 this concept has been widely used and FLPs showed reactivity toward a series of unsaturated compounds such as alkenes and alkynes,3,4 carbonyl compounds,4,5 carbon oxides,6,7 nitrogen oxides,8 SO2,7,9etc. Among these, reactions of FLPs with alkynes were widely studied, which could be broadly classified into four types of reaction: C–H bond activation (type I),3c C
C bond addition (type II),3b–d 1,1-carboboration (type III)10 and 1,2-hydroboration (type IV)4b,11 (Scheme 1a). For type I and II reactions, FLPs became part of the final products, whereas only a Lewis acid was incorporated in the final products in type III and IV reactions.
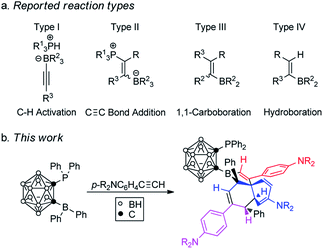 |
| Scheme 1 Reaction types of alkynes with FLP templates: (a) reported reaction types, (b) tri-insertion reaction described in this work. | |
In the aforementioned developments, intramolecular vicinal FLPs have played an important role, and their stabilities and reactivities were found to strongly depend on the nature of the bridging units.12–14 For example, the saturated FLP1 has been one of the most active metal-free dihydrogen activators,12 whereas the unsaturated otherwise closely related FLP2 is almost inactive towards dihydrogen splitting (Scheme 2).13 In contrast, the cyclohexadiene-derived FLP3 and aromatic phenylene bridged FLP4 are very active FLPs despite the presence of a pair of C(sp2) centers in their vicinal bridges.14 As a three-dimensional analog of benzene, o-carborane has two adjacent six-coordinate carbons with a C–C distance of ca 1.67 Å.15 Such a 3D bridging system differs significantly from FLP1-4 in terms of the bridging C–C distances, the hybridization and geometry of two bridging carbons and the bulkiness of the backbone, which offers an excellent model compound for comparison with the 2D system. The results showed that a carborane based FLP,16 1-PPh2-2-BPh2-1,2-C2B10H10 (2), reacted with p-R2NC6H4C
CH to afford unprecedented stereoselective tri-insertion products, 3,3a,6,6a-tetrahydronaphtho[1,8a-b]borole tricycles (4) accompanied by the dearomatization of one of the aryl rings from arylacetylene moieties (Scheme 1b). Such properties had not been observed in FLP chemistry before.1,17 These new results are reported in this article.
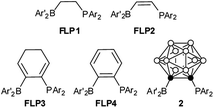 |
| Scheme 2 Intramolecular vicinal frustrated Lewis pairs. | |
Results and discussion
The FLP 1-PPh2-2-BPh2-1,2-C2B10H10 (2) was conveniently synthesized by treatment of 1-PPh2-1,2-C2B10H11 (1) with 1 equiv. of n-BuLi, followed by reaction with an equimolar amount of Ph2BCl in toluene. The 11B chemical shift of the BPh2 group was observed at 15.5 ppm. The 31P chemical shift of the PPh2 unit appeared at 27.9 ppm, which was slightly downfield-shifted compared to that of 26.0 ppm in 1. The P⋯B distance of 2.113(3) Å in 2 as determined by single-crystal X-ray analyses (Fig. S1 in the ESI†) is somewhat shorter than that of 2.203(6) Å in FLP4, and 2.182(3) Å in FLP3 (Scheme 2).14
Compound 2 exhibited weak FLP properties and did not split H2 under various reaction conditions (2 bar H2, toluene, 2 days, and T = RT to 100 °C) as indicated by 31P NMR spectra. However, it reacted readily with an equimolar amount of p-R2NC6H4C
CH in toluene at room temperature to afford C
C bond addition products 1,2-[Ph2PC(R2NC6H4)
C(H)BPh2]-1,2-C2B10H10 [R = Me (3-Me), Et (3-Et), Ph (3-Ph)] in 85–94% isolated yields (Scheme 3).
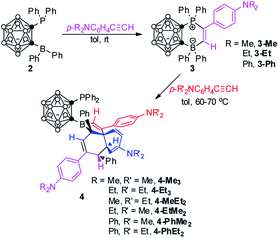 |
| Scheme 3 Insertion of terminal alkynes into the FLP template. | |
These compounds were characterized using various spectroscopic data as well as HRMS. The 11B chemical shift of the four-coordinate boron was observed at about −11 ppm, and the phosphorus signal appeared at ca. 12 ppm in the 31P NMR spectra. The olefinic proton signal was observed at about 9.3 ppm, and the corresponding olefinic carbons appeared at about 191 ppm. The molecular structures of 3-Me and 3-Ph were further confirmed by single-crystal X-ray diffraction studies. Fig. 1 shows the representative structure of 3-Me. It clearly indicates that the terminal alkyne carbon (C41) is bonded to B(13) due to the polarization of the C
C bond. The C(41)–C(42) bond distance of 1.338(3) Å falls in the range of 1.32 to 1.39 Å normally observed for C
C double bonds.3b,c,4b,d,10,11a,c,d
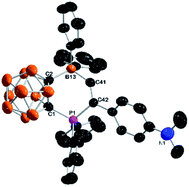 |
| Fig. 1 Molecular structure of 3-Me. All H atoms are omitted for clarity; thermal ellipsoids are drawn at the 50% probability level. Key bond distances (Å) and angles (°): B(13)–C(41) 1.632(4), B(13)–C(2) 1.691(4), C(41)–C(42) 1.338(3), P(1)–C(42) 1.787(3), P(1)–C(1) 1.844(3), C(1)–C(2) 1.690(4), C(2)–B(13)–C(41) 108.7(2), B(13)–C(41)–C(42) 133.9(2), C(41)–C(42)–P(1) 116.9(2), and C(42)–P(1)–C(1) 107.2(1). | |
Compounds 3-Me and 3-Et reacted slowly with 2 equiv. of p-R2NC6H4C
CH (R = Me, Et) in toluene at 70 °C to give unprecedented tri-insertion products 4-Me3 and 4-Et3 in 60% and 50% isolated yields, respectively (Scheme 3).
The molecular structures of both 4-Me3 and 4-Et3 were confirmed by single-crystal X-ray analyses. Fig. 2 shows the representative structure of 4-Me3. The B(13)⋯P distances of 3.303(5) Å in 4-Me3 and 3.305(4) Å in 4-Et3 are significantly longer than that of 2.113(3) Å in 2. Both P and B(13) atoms are three-coordinate, yet the coordination environment of the B(13) atom in 4 is completely different from that observed in 2. The sum of bond angles around the B(13) atom is 359.4° in 4-Me3 and 359.8° in 4-Et3, indicating that both B(13) atoms adopt a trigonal planar geometry. It is unambiguously confirmed that the insertion of three arylacetylenes into the FLP template 2 with the migration of the phenyl groups from the boron to two different carbons afforded 3,3a,6,6a-tetrahydronaphtho[1,8a-b]borole tricycles 4, leading to the construction of four stereocenters including one quaternary carbon. To the best of our knowledge, this type of transformation has not been observed before in FLP chemistry.
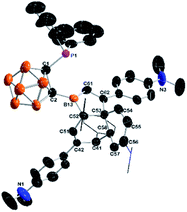 |
| Fig. 2 Molecular structure of 4-Me3. All H atoms are omitted, two phenyls and one Me2N group are shown in the wireframe format for clarity; thermal ellipsoids are drawn at the 50% probability level. Key bond distances (Å) and angles (°): B(13)–C(61) 1.495(6), B(13)–C(52) 1.586(6), B(13)–C(2) 1.618(6), C(61)–B(13)–C(52) 108.0(4), C(61)–B(13)–C(2) 125.1(4), and C(52)–B(13)–C(2) 126.3(4). | |
To gain some insight into the reaction path, crossover experiments were performed. Treatment of 3-Me with an excess amount of p-Et2NC6H4C
CH in toluene at 70 °C gave the tri-insertion product 4-MeEt2 in 51% isolated yield. In the same manner, the corresponding tri-insertion compounds 4-EtMe2, 4-PhMe2 and 4-PhEt2 were prepared in 51%, 81% and 77% isolated yields, respectively (Scheme 3).
Their NMR spectra shared the same features as those of 4-Me3 and 4-Et3, indicating that these compounds should have similar molecular skeletons. Subsequently, the molecular structures of 4-EtMe2 and 4-PhEt2 were unambiguously determined by single-crystal X-ray analyses. The representative structure of 4-EtMe2 is shown in Fig. 3. These crossover experimental results suggested that the insertion of arylacetylene into 2 to form 3 was an irreversible reaction, otherwise, the homo-triinsertion product 4-Me3 or 4-Et3 would be isolated.
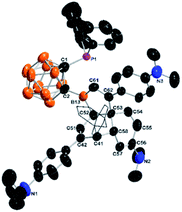 |
| Fig. 3 Molecular structure of 4-EtMe2. All H atoms are omitted, and two phenyl groups are shown in the wireframe format for clarity; thermal ellipsoids are drawn at the 50% probability level. Key bond distances (Å) and angles (°): B(13)–C(61) 1.513(5), B(13)–C(52) 1.603(6), B(13)–C(2) 1.609(6), C(61)–B(13)–C(52) 106.4(3), C(61)–B(13)–C(2) 125.5(4), and C(52)–B(13)–C(2) 127.7(3). | |
Another question then arises as to how the alkyne reacts with 3. After careful analyses of the molecular structures of 4, we speculated that the Ph group of the BPh2 unit in 3 may migrate to vinyl carbon, followed by a rearrangement to regenerate a new FLP template for the reaction with alkynes. Each migration of Ph from the B center would accompany an alkyne insertion, leading to the formation of the final product 4. However, many attempts to isolate the intermediates were not successful. We thought that a newly generated FLP species might be trapped by formaldehyde (CH2O)n.4,5 Accordingly, treatment of 3-Ph with an excess amount of (CH2O)n in C6D6 at 40 °C for 7 d afforded 1,2-{Ph2PCH2OB(Ph)[C(C6H4NPh2)
CHPh]}-1,2-C2B10H10 (5-Ph) as colorless crystals in 38% isolated yield (Scheme 4).
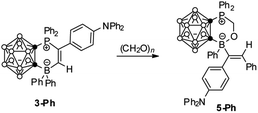 |
| Scheme 4 Reaction of 3-Ph with formaldehyde. | |
The proton chemical shifts of the CH2O unit were observed as two doublet of doublets at 5.06 and 4.84 ppm, and its 13C signal appeared at 61.2 ppm. The 31P resonance was observed at 3.7 ppm, while the exo-B signal was overlapped with cage B atoms in the 11B NMR spectrum. The molecular structure of 5-Ph was unambiguously confirmed by single-crystal X-ray analyses (Fig. 4). The C(41)–C(42) distance of 1.326(5) Å suggests that it is a typical C
C double bond, confirming that the borate and phenyl groups are in trans-positions in 5-Ph.
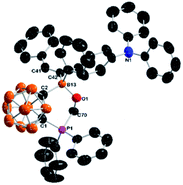 |
| Fig. 4 Molecular structure of 5-Ph. All H atoms are omitted for clarity; thermal ellipsoids are drawn at the 50% probability level. Key bond distances (Å) and angles (°): P(1)–C(70) 1.817(4), P(1)–C(1) 1.844(4), B(13)–O(1) 1.487(5), B(13)–C(2) 1.732(5), B(13)–C(42) 1.637(6), C(41)–C(42) 1.326(5), C(70)–O(1) 1.389(5), C(70)–P(1)–C(1) 102.9(2), and O(1)–B(13)–C(2) 106.7(3). | |
On the basis of the aforementioned results and literature work,3d,10,18,19 a plausible reaction mechanism is proposed for the formation of 4 (Scheme 5). 1,2-Migration of the phenyl group from the borate gives a phosphonium ylide A1. Another 1,2-boryl migration affords an intermediate A2, in which the boryl and Ph are in trans-positions due to steric reasons. A2 can be trapped by formaldehyde to form 5 (Scheme 4). A2 is a newly formed FLP template which is similar to that of 2. A second equiv. of alkyne addition yields B1, an analogue of 3. Similar 1,2-migration processes (from 3 to A1 to A2) repeat to generate B2 and B3. Electrocyclic ring closure of B3 gives a dearomatized intermediate B4 with the construction of two stereocenters in which two hydrogen atoms are trans to each other. B4 is again a newly generated FLP. A third equiv. of alkyne addition to B4, followed by rearrangement constructs stereoselectively a quaternary carbon center, giving a dearomatized intermediate C1. 1,2-Migration of the phenyl group from the borate affords the final product 4 with the generation of the fourth stereocenter.
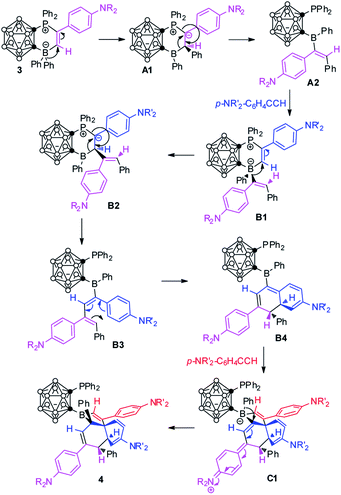 |
| Scheme 5 Proposed mechanism for the formation of 4. | |
Conclusions
In summary, a new FLP 1-PPh2-2-BPh2-1,2-C2B10H10 (2) having a carborane backbone was prepared and structurally characterized. Though it did not split H2, it reacted with p-R2NC6H4C
CH to give typical C
C bond insertion products 3. In the presence of an excess amount of p-R2NC6H4C
CH, unprecedented tri-insertion products 4 were isolated. The structural analyses confirmed that four stereocenters including one quaternary carbon had been created in a single process. After trapping and structural characterization of a key intermediate, a plausible mechanism was proposed, which involves sequential alkyne addition and 1,2-migration. These new properties enrich the chemistry of FLPs.
The results of this work indicate that the chemical properties of intramolecular vicinal FLPs (Scheme 2) are not only dependent on the P⋯B distance, the hybridization of the vicinal carbons, and electronic properties of the backbone, but also the steric hindrance of the bridging unit. As FLPs can be easily incorporated into an o-carborane cage,20 a new class of FLPs with various substituents would be accessible for the development of new FLP reactions.
Conflicts of interest
There are no conflicts to declare.
Acknowledgements
The work described in this paper was supported by grants from the Research Grants Council of the Hong Kong Special Administration Region (Project No. 14306519), and the NSFC/RGC Joint Research Scheme (Project No. N_CUHK402/18).
Notes and references
- For recent reviews, see:
(a) D. W. Stephan, Acc. Chem. Res., 2015, 48, 306–316 CrossRef CAS PubMed;
(b) D. W. Stephan and G. Erker, Angew. Chem., Int. Ed., 2015, 54, 6400–6441 CrossRef CAS PubMed;
(c) D. W. Stephan, J. Am. Chem. Soc., 2015, 137, 10018–10032 CrossRef CAS PubMed;
(d) A. J. P. Cardenas, Y. Hasegawa, G. Kehr, T. H. Warren and G. Erker, Coord. Chem. Rev., 2016, 306, 468–482 CrossRef CAS;
(e) D. W. Stephan, Science, 2016, 354, aaf7229 CrossRef PubMed;
(f) J. Paradies, Coord. Chem. Rev., 2019, 380, 170–183 CrossRef CAS;
(g) A. R. Jupp and D. W. Stephan, Trends Chem., 2019, 1, 25–48 Search PubMed.
- G. C. Welch, R. R. San Juan, J. D. Masuda and D. W. Stephan, Science, 2006, 314, 1124–1126 CrossRef CAS PubMed.
-
(a) J. S. J. McCahill, G. C. Welch and D. W. Stephan, Angew. Chem., Int. Ed., 2007, 46, 4968–4971 CrossRef CAS PubMed;
(b) A. Fukazawa, H. Yamada and S. Yamaguchi, Angew. Chem., Int. Ed., 2008, 47, 5582–5585 CrossRef CAS PubMed;
(c) M. A. Dureen and D. W. Stephan, J. Am. Chem. Soc., 2009, 131, 8396–8397 CrossRef CAS PubMed;
(d) S. Tamke, Z.-W. Qu, N. A. Sitte, U. Flörke, S. Grimme and J. Paradies, Angew. Chem., Int. Ed., 2016, 55, 4336–4339 CrossRef CAS PubMed.
-
(a) C. M. Mömming, S. Frömel, G. Kehr, R. Fröhlich, S. Grimme and G. Erker, J. Am. Chem. Soc., 2009, 131, 12l280–12289 CrossRef PubMed;
(b) C. Rosorius, G. Kehr, R. Fröhlich, S. Grimme and G. Erker, Organometallics, 2011, 30, 4211–4219 CrossRef CAS;
(c) X. Jie, C. G. Daniliuc, R. Knitsch, M. R. Hansen, H. Eckert, S. Ehlert, S. Grimme, G. Kehr and G. Erker, Angew. Chem., Int. Ed., 2019, 58, 882–886 CrossRef CAS PubMed;
(d) S. Dong, C. G. Daniliuc, G. Kehr and G. Erker, Chem.–Eur. J., 2020, 26, 745–753 CrossRef CAS PubMed.
-
(a) C. M. Mömming, G. Kehr, B. Wibbeling, R. Fröhlich and G. Erker, Dalton Trans., 2010, 39, 7556–7564 RSC;
(b) O. Ekkert, G. Kehr, C. G. Daniliuc, R. Fröhlich, B. Wibbeling, J. L. Petersen and G. Erker, Z. Anorg. Allg. Chem., 2013, 639, 2455–2462 CrossRef CAS;
(c) W. Uhl and C. Appelt, Organometallics, 2013, 32, 5008–5014 CrossRef CAS.
-
(a) C. M. Mömming, E. Otten, G. Kehr, R. Fröhlich, S. Grimme, D. W. Stephan and G. Erker, Angew. Chem., Int. Ed., 2009, 48, 6643–6646 CrossRef PubMed;
(b) G. Ménard and D. W. Stephan, J. Am. Chem. Soc., 2010, 132, 1796–1797 CrossRef PubMed;
(c) A. Berkefeld, W. E. Piers and M. Parvez, J. Am. Chem. Soc., 2010, 132, 10660–10661 CrossRef CAS PubMed;
(d) M. Sajid, L.-M. Elmer, C. Rororius, C. G. Daniliuc, S. Grimme, G. Kehr and G. Erker, Angew. Chem., Int. Ed., 2013, 52, 2243–2246 CrossRef CAS PubMed;
(e) M. Sajid, A. Lawzer, W. Dong, C. Rosorius, W. Sander, B. Schirmer, S. Grimme, C. G. Daniliuc, G. Kehr and G. Erker, J. Am. Chem. Soc., 2013, 135, 18567–18574 CrossRef CAS PubMed;
(f) L. Wang, S. Dong, C. G. Daniliuc, L. Liu, S. Grimme, R. Knitsch, H. Eckert, M. R. Hansen, G. Kehr and G. Erker, Chem. Sci., 2018, 9, 1544–1550 RSC.
-
(a) B. Waerder, M. Pieper, L. A. Körte, T. A. Kinder, A. Mix, B. Neumann, H.-G. Stammler and N. W. Mitzel, Angew. Chem., Int. Ed., 2015, 54, 13416–13419 CrossRef CAS PubMed;
(b) P. Holtkamp, F. Friedrich, E. Stratmann, A. Mix, B. Neumann, H.-G. Stammler and N. W. Mitzel, Angew. Chem., Int. Ed., 2019, 58, 5114–5118 CrossRef CAS PubMed;
(c) N. Aders, L. Keweloh, D. Pleschka, A. Hepp, M. Layh, F. Rogel and W. Uhl, Organometallics, 2019, 38, 2839–2852 CrossRef CAS.
-
(a) E. Otten, R. C. Neu and D. W. Stephan, J. Am. Chem. Soc., 2009, 131, 9918–9919 CrossRef CAS PubMed;
(b) R. C. Neu, E. Otten, A. Lough and D. W. Stephan, Chem. Sci., 2011, 2, 170–176 RSC;
(c) A. J. P. Cardenas, B. J. Culotta, T. H. Warren, S. Grimme, A. Stute, R. Fröhlich, G. Kehr and G. Erker, Angew. Chem., Int. Ed., 2011, 50, 7567–7571 CrossRef CAS PubMed;
(d) M. Sajid, A. Stute, A. J. P. Cardenas, B. J. Culotta, J. A. M. Hepperle, T. H. Warren, B. Schirmer, S. Grimme, A. Studer, C. G. Daniliuc, R. Fröhlich, J. L. Petersen, G. Kehr and G. Erker, J. Am. Chem. Soc., 2012, 134, 10156–10168 CrossRef CAS PubMed;
(e) G. Ménard, J. A. Hatnean, H. J Cowley, A. J. Lough, J. M. Rawson and D. W. Stephan, J. Am. Chem. Soc., 2013, 135, 6446–6449 CrossRef PubMed;
(f) J. C. M. Pereira, M. Sajid, G. Kehr, A. M. Wright, B. Schirmer, Z.-W. Qu, S. Grimme, G. Erker and P. C. Ford, J. Am. Chem. Soc., 2014, 136, 513–519 CrossRef CAS PubMed.
- M. Sajid, A. Klose, B. Birkmann, L. Liang, B. Schirmer, T. Wiegand, H. Eckert, A. J. Lough, R. Fröhlich, C. G. Daniliuc, S. Grimme, D. W. Stephan, G. Kehr and G. Erker, Chem. Sci., 2013, 4, 213–219 RSC.
-
(a) C. Chen, R. Fröhlich, G. Kehr and G. Erker, Chem. Commun., 2010, 46, 3580–3582 RSC;
(b) O. Ekkert, G. Kehr, R. Fröhlich and G. Erker, J. Am. Chem. Soc., 2011, 133, 4610–4616 CrossRef CAS PubMed;
(c) J. Möbus, Q. Bonnin, K. Ueda, R. Fröhlich, K. Itami, G. Kehr and G. Erker, Angew. Chem., Int. Ed., 2012, 51, 1954–1957 CrossRef PubMed;
(d) R. Liedtke, F. Scheidt, J. Ren, B. Schirmer, A. J. P. Cardenas, C. G. Daniliuc, H. Eckert, T. H. Warren, S. Grimme, G. Kehr and G. Erker, J. Am. Chem. Soc., 2014, 136, 9014–9027 CrossRef CAS PubMed;
(e) A. Feldmann, G. Kehr, C. G. Daniliuc, C. Mück-Lichtenfeld and G. Erker, Chem.–Eur. J., 2015, 21, 12456–12464 CrossRef CAS PubMed;
(f) J. Möricke, A. Ueno, B. Wibbeling, C. G. Daniliuc, G. Kehr and G. Erker, Eur. J. Inorg. Chem., 2019, 2912–2917 CrossRef.
-
(a) J. Yu, G. Kehr, C. G. Daniliuc and G. Erker, Eur. J. Inorg. Chem., 2013, 18, 3312–3315 CrossRefK. Chernichnko, Á. Madarász, I. Pápai, M. Nieger, M. Leskelä and T. Repo, Nat. Chem., 2013, 5, 718–723 CrossRef PubMed ; 46, 11715–11721
(b) Z. Jian, G. Kehr, C. G. Daniliuc, B. Wibbeling and G. Erker, Dalton Trans., 2017, 46, 11715–11721 RSC;
(c) L. Fan, A. R. Jupp and D. W. Stephan, J. Am. Chem. Soc., 2018, 140, 8119–8123 CrossRef CAS PubMed.
- P. Spies, G. Erker, G. Kehr, K. Bergander, R. Fröhlich, S. Grimme and D. W. Stephan, Chem. Commun., 2007, 47, 5072–5074 RSC.
- T. Wiegand, H. Eckert, O. Ekkert, R. Fröhlich, G. Kehr, G. Erker and S. Grimme, J. Am. Chem. Soc., 2012, 134, 4236–4249 CrossRef CAS PubMed.
- G.-Q. Chen, G. Kehr, C. G. Daniliuc, C. Mück-Lichtenfeld and G. Erker, Angew. Chem., Int. Ed., 2016, 55, 5526–5530 CrossRef CAS PubMed.
-
(a)
N. S. Hosmane, Boron Science: New Technologies and Application, CRC Press, Boca Raton, FL, 2012 Search PubMed;
(b)
R. N. Grimes, Carboranes, 3rd edn, Academic Press, Amsterdam, 2016 Search PubMed.
- For the preparation of 1-BO2C6H4-7-PPh2-closo-1,7-C2B10H10, see: A. Benton, J. D. Watson, S. M. Mansell, G. M. Rosair and A. J. Welch, J. Organomet. Chem., 2020, 907, 121057 CrossRef CAS.
-
(a) P. Vasko, L. A. Zulkifly, M. Á. Fuentes, Z. Mo, J. Hicks, P. C. J. Kämer and S. Aldridge, Chem.–Eur. J., 2018, 24, 10531–10540 CrossRef CAS PubMed;
(b) A. Bismuto, G. S. Nichol, F. Duarte, M. J. Cowley and S. T. Thomas, Angew. Chem., Int. Ed., 2020, 59, 12731–12735 CrossRef CAS PubMed.
- The 1,2-migratory reaction is a common reaction mode in organoborates, see:
(a)
A. Pelter; K. Smith and H. C. Brown, Borane Reagents, Academic Press Limited, London, 1988 Search PubMed;
(b)
S. E. Thomas, Organic Synthesis: The Roles of Boron and Silicon, Oxford University Press, Oxford, New York, 1991 Search PubMed.
-
(a) M. A. Dureen, C. C. Brown and D. W. Stephan, Organometallics, 2010, 29, 6422–6432 CrossRef CAS;
(b) B.-H. Xu, G. Kehr, R. Fröhlich and G. Erker, Organometallics, 2011, 30, 5080–5083 CrossRef CAS;
(c) B.-H. Xu, C. M. Mömming, R. Fröhlich, G. Kehr and G. Erker, Chem.–Eur. J., 2012, 18, 1826–1830 CrossRef CAS PubMed;
(d) M. M. Hansmann, R. L. Melen, F. Rominger, S. S. K. Hashmi and D. W. Stephan, J. Am. Chem. Soc., 2014, 136, 777–782 CrossRef CAS PubMed;
(e) M. M. Hansmann, R. L. Melen, M. Rudolph, F. Rominger, H. Wadepohl, D. W. Stephan and A. S. K. Hashmi, J. Am. Chem. Soc., 2015, 137, 15469–15477 CrossRef CAS PubMed;
(f) A. Bähr, L. C. Wilkins, K. Ollegott, B. M. Kariuki and R. L. Melen, Molecules, 2015, 20, 4530–4547 CrossRef PubMed.
-
(a) Z. Xie, Acc. Chem. Res., 2003, 36, 1–9 CrossRef CAS PubMed;
(b) D. Olid, R. Núñez, C. Viñas and F. Teixidor, Chem. Soc. Rev., 2013, 42, 3318–3336 RSC;
(c) R. M. Dziedzic and A. M. Spokoyny, Chem. Commun., 2019, 55, 430–442 RSC;
(d) M. Scholz and E. Hey-Hawkins, Chem. Rev., 2011, 111, 7035–7062 CrossRef CAS PubMed;
(e) Y. Quan and Z. Xie, Chem. Soc. Rev., 2019, 48, 3660–3673 RSC.
Footnote |
† Electronic supplementary information (ESI) available: Experimental and summary of crystal data collection and refinement. CCDC 2026881–2026888. For ESI and crystallographic data in CIF or other electronic format see DOI: 10.1039/d0sc05755j |
|
This journal is © The Royal Society of Chemistry 2021 |