DOI:
10.1039/D0SC05269H
(Edge Article)
Chem. Sci., 2021,
12, 1445-1450
Construction of chiral α-tert-amine scaffolds via amine-catalyzed asymmetric Mannich reactions of alkyl-substituted ketimines†
Received
24th September 2020
, Accepted 26th November 2020
First published on 27th November 2020
Abstract
Stereoselective Mannich reactions of aldehydes with ketimines provide chiral β-amino aldehydes that bear an α-tert-amine moiety. However, the structural variation of the ketimines is limited due to the formation of inseparable E/Z isomers, low reactivity, and other synthetic difficulties. In this study, a highly diastereodivergent synthesis of hitherto difficult-to-access β-amino aldehydes that bear a chiral α-tert-amine moiety was achieved using the amine-catalyzed Mannich reactions of aldehydes with less-activated Z-ketimines that bear both alkyl and alkynyl groups.
Introduction
The asymmetric Mannich reaction is one of the most basic and useful methods for the synthesis of chiral β-amino carbonyl compounds,1 which are fundamental and frequent motifs in bioactive compounds, as well as versatile building blocks for the synthesis of structurally complex molecules.2 In particular, the use of ketimines provides an efficient access to β-amino carbonyl compounds that bear an α-tert-amine moiety, and a wide variety of Mannich reactions with ketimines have been developed to date.3–8 During the face-selective nucleophilic addition to ketimines, the geometry of the N-substituent of the ketimine significantly affects the enantioselectivity because the chiral catalyst interacts with the N-substituent or the lone pair of the imine nitrogen atom. Therefore, dialkyl-substituted ketimines, which exist as an inseparable mixture of nearly equal amounts of the E- and Z-ketimine,9 are rarely used in asymmetric synthesis compared to ketimines that exist as single geometric isomers.3–7
Recently, we found that the Z isomer of ketimines can be obtained selectively by replacing one alkyl substituent with a less sterically hindered alkynyl group.10 This type of ketimine can be considered a synthetic equivalent of the dialkyl-substituted ketimine, since the alkynyl group can be converted to an alkyl group by simple hydrogenation. Via the introduction of a chiral auxiliary to the alkynyl-substituted ketimines, the asymmetric synthesis of α-tert-amine derivatives has been realized (Scheme 1a).11 However, stoichiometric quantities of the chiral auxiliary are required, as well as highly reactive reagents to achieve the nucleophilic addition to the less-reactive ketimines. We thus became interested in developing a catalytic asymmetric Mannich reaction using both alkyl and alkynyl-substituted ketimines under mild conditions.
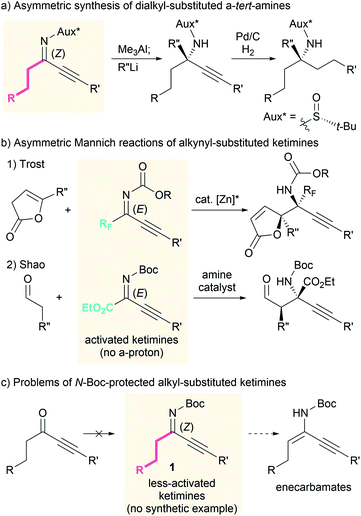 |
| Scheme 1 Alkynyl-substituted ketimines for asymmetric reactions. | |
Some alkynyl-substituted ketimines have been used for asymmetric reactions12 including Mannich reactions, although the applicable ketimines are limited to those activated by an electron-withdrawing perfluoroalkyl or ester group (Scheme 1b).8 To the best of our knowledge, examples of catalytic asymmetric reactions of alkynyl-substituted ketimines that bear an electron-donating alkyl group on the imine carbon have not yet been reported. This can be attributed in part to the limited number of available alkyl- and alkynyl-substituted ketimines11,13 and difficulties associated with their synthesis. For example, N-Boc-protected ketimines 1 cannot be synthesized from the corresponding ketones (Scheme 1c, left). Furthermore, unlike ketimines that bear a perfluoroalkyl or ester group, alkyl-substituted ketimines 1 can isomerize to the corresponding enecarbamates due to the presence of the ketimine α-protons (Scheme 1c, right). Herein, we report amine-catalyzed asymmetric Mannich reactions of less-activated alkyl- and alkynyl-substituted ketimines.14 The use of newly synthesized N-Boc-protected ketimines 1 and appropriate amine catalysts provides an effective solution to the aforementioned problems.
Results and discussion
Our initial investigation focused on the installation of a protecting group on the alkyl- and alkynyl-substituted ketimines to facilitate their amine-catalyzed Mannich reaction with an aldehyde (Scheme 2). The resulting product was treated in situ with sodium borohydride and converted into the corresponding alcohol to facilitate its isolation and avoid product decomposition. First, the Mannich reaction of an N-sulfinyl-protected ketimine, which was prepared following a known procedure,11 with 3-phenylpropanal in the presence of L-proline was examined; however, the desired Mannich adduct was not obtained probably due to the low reactivity of the ketimine. Next, an N-Ts-protected ketimine, which was synthesized by the oxidation of the N-sulfinyl-ketimine (for details, see the ESI†), was used in the Mannich reaction. Although this ketimine is considered to be more reactive than the N-sulfinyl-ketimine, the desired product was not observed, probably due to the deactivation of the catalyst through its addition to the ketimine. We then decided to use a ketimine activated by a Boc protecting group. However, the N-Boc-protected ketimine was unfortunately not accessible via common approaches, such as dehydrative condensation11,13d or an aza-Wittig reaction with the corresponding ketone.8 This may be due to the instability of N-Boc-ketimines at high reaction temperatures.15 In seeking to develop new methods for the synthesis of such ketimines, we found that the nucleophilic substitution of amidines that bear a benzimidazole moiety with organolithium reagents could provide the desired ketimines at low temperature (Scheme 3a). We also found that these Z-ketimines could be prepared by the oxidation of N-Boc-protected amines (Scheme 3b).16 The Mannich reaction of the thus obtained ketimine furnished the desired adduct 2a with high diastereo- and enantioselectivity, albeit in low yield due to undesired side reactions such as homoaldol condensation, hydrolysis of the ketimine and isomerization to the enecarbamate.
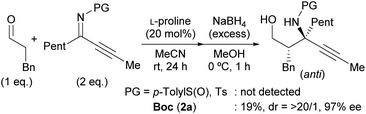 |
| Scheme 2 Amine-catalyzed asymmetric Mannich reaction of Z-ketimines. | |
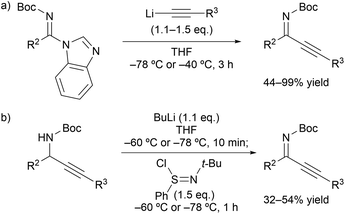 |
| Scheme 3 Synthesis of N-Boc-protected Z-ketimines. | |
Encouraged by this promising result, the reaction conditions were optimized to suppress these side reactions (Table 1). Solvent screening revealed that the Mannich reaction in chloroform proceeded to give 2a in moderate yield with high anti- and enantioselectivity (entry 3). When diphenylprolinol (S)-3 was used as the catalyst instead of proline, the reaction did not proceed (entry 4). This result indicates that the present Mannich reaction requires the activation of the ketimine by a catalyst with an acidic functionality, such as a carboxylic acid. Catalyst (S)-4 with a tetrazole moiety, which is a bioisostere of carboxylic acid, gave the Mannich adduct in 45% yield with lower anti-selectivity (entry 5). When the TBS-protected hydroxyproline (S,R)-5 was used to improve the solubility of the catalyst, anti-2a was obtained in low yield (entry 6).
Table 1 Asymmetric Mannich reaction of 3-phenylpropanal with 1aa
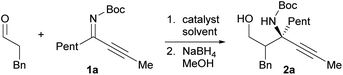
|
Entry |
Catalyst (mol%) |
Solvent |
Yieldb (%) |
anti/sync |
eed (%) |
Performed using 3-phenylpropanal (0.1 mmol) and 1a (0.2 mmol) in the specified solvent (100 μL) for 24 h at room temperature.
Isolated yield.
Determined by 1H NMR spectroscopy.
The ee of the major diastereomer was determined via HPLC using a chiral column.
Performed for 48 h using 3-phenylpropanal (0.2 mmol) and 1a (0.1 mmol).
Performed at 5 °C. n.d. = not detected.
|
1 |
L-Proline (20) |
MeCN |
19 |
>20/1 |
97 |
2 |
L-Proline (20) |
DMSO |
37 |
>20/1 |
95 |
3 |
L-Proline (20) |
CHCl3 |
67 |
>20/1 |
97 |
4 |
(S)-3 (20) |
CHCl3 |
n.d. |
— |
— |
5 |
(S)-4 (20) |
CHCl3 |
45 |
10/1 |
99 |
6 |
(S,R)-5 (20) |
CHCl3 |
9 |
>20/1 |
99 |
7e |
(S)-6 (10) |
MeCN |
31 |
1/4 |
99 |
8e |
(S,R)-7 (10) |
MeCN |
33 |
1/6 |
98 |
9e,f |
(S,R)-7 (10) |
MeCN |
84 |
1/13 |
99 |
|
We have previously developed the axially chiral amino sulfonamide catalyst (S)-617 and the chiral phenylcyclopropane-based amine catalyst (S,R)-7.18 In the Mannich reaction with aldimines, (S)-6 and (S,R)-7 show a diastereoselectivity opposite to proline and related catalysts. Fortunately, the binaphthyl-based amine catalyst (S)-6 promoted the reaction of 3-phenylpropanal with 1a to preferentially afford syn-2a, albeit with low syn-selectivity (entry 7). Use of the recently developed catalyst (S,R)-7 improved the syn-selectivity, although the yield remained low due to the isomerization and hydrolysis of 1a (entry 8). Further investigations revealed that 2a was obtained in good yield with high syn- and enantioselectivity when the reaction was performed at 5 °C (entry 9).
Using the optimal reaction conditions, the anti-selective Mannich reaction of several aldehydes with Z-ketimines was examined in the presence of L-proline as the catalyst (Table 2). Both aliphatic aldehydes and functionalized aldehydes bearing a protected hydroxy or amino group were tolerated under the applied reaction conditions (entries 1–6). However, when propanal was used as the nucleophile, the reaction furnished the desired adduct with slightly lower anti-selectivity (entry 2). Other alkyl-substituted ketimines (R2 = methyl or isobutyl) were also applicable to this reaction (entries 7 and 8). Replacing the R3 (Me) group on Z-ketimine 1a with a butyl, phenyl, or TIPS group, did not affect the anti- and enantioselectivity (entries 9–11).
Table 2
anti-Selective Mannich reaction catalyzed by L-prolinea
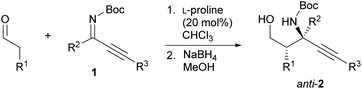
|
Entry |
R1 |
R2 |
R3 |
Yieldb (%) |
anti/sync |
eed (%) |
Performed using an aldehyde (0.1 mmol) and 1 (0.2 mmol) in CHCl3 (100 μL) for 24 h at room temperature.
Isolated yield.
Determined by 1H NMR spectroscopy.
The ee of the major diastereomer was determined via HPLC using a chiral column.
The ee was determined after benzoylation.
|
1 |
Bn |
Pent |
Me |
2a
|
67 |
>20/1 |
97 |
2e |
Me |
Pent |
Me |
2b
|
49 |
8/1 |
99 |
3e |
Bu |
Pent |
Me |
2c
|
73 |
>20/1 |
98 |
4e |
Allyl |
Pent |
Me |
2d
|
45 |
>20/1 |
99 |
5 |
(CH2)2OBn |
Pent |
Me |
2e
|
45 |
>20/1 |
96 |
6e |
(CH2)2NHCOCF3 |
Pent |
Me |
2f
|
61 |
17/1 |
99 |
7 |
Bn |
Me |
Me |
2g
|
61 |
13/1 |
98 |
8 |
Bn |
i-Bu |
Me |
2h
|
64 |
>20/1 |
96 |
9 |
Bn |
Pent |
Bu |
2i
|
62 |
>20/1 |
97 |
10 |
Bn |
Pent |
Ph |
2j
|
77 |
>20/1 |
99 |
11 |
Bn |
Pent |
TIPS |
2k
|
42 |
>20/1 |
96 |
Next, we examined the syn-selective Mannich reaction catalyzed by (S,R)-7 (Table 3). The reactions between various aldehydes and Z-ketimines furnished Mannich products with high syn- and enantioselectivity. While the use of aldehydes that bear a protected amino group, an isobutyl-substituted ketimine, or a phenylethynyl-substituted ketimine resulted in poor syn-selectivity (syn/anti = <10/1), lowering the reaction temperature increased the syn-selectivity (entries 6, 8, and 10).
Table 3
syn-Selective Mannich reaction catalyzed by (S,R)-7a
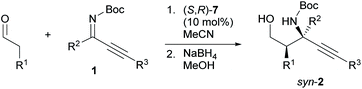
|
Entry |
R1 |
R2 |
R3 |
Yieldb (%) |
syn/antic |
eed (%) |
Performed using an aldehyde (0.2 mmol) and 1 (0.1 mmol) in MeCN (100 μL) for 48 h at 5 °C.
Isolated yield.
Determined by 1H NMR spectroscopy.
The ee of the major diastereomer was determined via HPLC using a chiral column.
The ee was determined after benzoylation.
Performed at 0 °C.
|
1 |
Bn |
Pent |
Me |
2a
|
84 |
13/1 |
99 |
2e |
Me |
Pent |
Me |
2b
|
84 |
>20/1 |
99 |
3e |
Bu |
Pent |
Me |
2c
|
81 |
>20/1 |
99 |
4e |
Allyl |
Pent |
Me |
2d
|
77 |
>20/1 |
99 |
5 |
(CH2)2OBn |
Pent |
Me |
2e
|
68 |
>20/1 |
99 |
6e,f |
(CH2)2NHCOCF3 |
Pent |
Me |
2f
|
61 |
>20/1 |
99 |
7 |
Bn |
Me |
Me |
2g
|
63 |
10/1 |
99 |
8f |
Bn |
i-Bu |
Me |
2h
|
60 |
>20/1 |
99 |
9 |
Bn |
Pent |
Bu |
2i
|
76 |
16/1 |
98 |
10f |
Bn |
Pent |
Ph |
2j
|
71 |
15/1 |
99 |
11 |
Bn |
Pent |
TIPS |
2k
|
80 |
20/1 |
99 |
A structural analysis of syn-2k using X-ray crystallography provided clear proof that the Mannich reaction catalyzed by (S,R)-7 gave syn-products with a 2R,3R configuration (Fig. 1). The absolute configuration of anti-2a, which was obtained from the reaction catalyzed by L-proline, was determined to be 2S,3R by comparison of the HPLC retention times of syn-2a ((2R,3R)-2a) and the epimer of anti-2a (for details, see the ESI†).
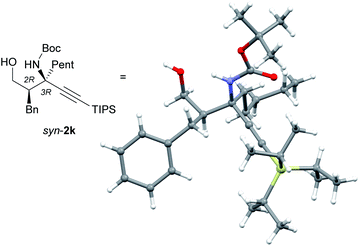 |
| Fig. 1 X-ray crystal structure of syn-2k. | |
Based on the absolute configuration of the products, we would like to propose transition state models TS2 and TS3 (Fig. 2). Since ketimines generally exist in an equilibrium between the E and Z isomers,19 a reaction of aldehydes with in situ-generated E-ketimines might occur. In transition state TS1, the E-ketimine approaches the s-trans-enamine, wherein the steric repulsion between the enamine moiety derived from the aldehyde and the carboxy group derived from L-proline is minimized, to give (2S,3S)-2. On the other hand, the Mannich product (2S,3R)-2 is formed from the Z-ketimine via transition state TS2. An analysis of the observed stereochemistry shows that the dominant Z-ketimine reacts with the s-trans-enamine (TS2). In the reaction catalyzed by (S,R)-7, both the s-trans-enamine and the s-cis-enamine can be formed, as the distal triflamide of (S,R)-7 does not cause steric repulsion with the enamine moiety. However, the reaction site of the s-trans-enamine is too far from the Z-ketimine activated by the triflimide group, and only the s-cis-enamine can react with the distant Z-ketimine to give predominantly syn-isomer (2R,3R)-2 (TS3).17,18 A computational study of the Mannich reaction of a ketimine using proline and a similar catalyst by Fu is consistent with the proposed transition state models TS2 and TS3.20
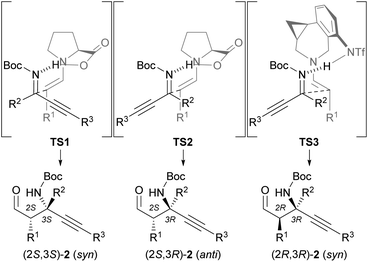 |
| Fig. 2 Transition state models for asymmetric Mannich reactions catalyzed by L-proline (TS2) or (S,R)-7 (TS3). | |
The synthetic utility of this method has been demonstrated via the transformation of the obtained Mannich product into previously less-accessible chiral α-tert-amine derivatives. Pinnick oxidation of the anti-Mannich product and methylation with trimethylsilyldiazomethane (TMSCHN2) provided the corresponding β-amino ester 8 without affecting the enantiomeric excess (Scheme 4a). Hydrogenation of anti-2a gave the chiral γ-amino alcohol derivative 9 with two similar alkyl groups (propyl and pentyl groups) (Scheme 4b). The chiral γ-amino alcohol 10, which bears a cis-olefin moiety, was obtained from the semi-hydrogenation of anti-2a with Lindlar catalyst (Scheme 4c).
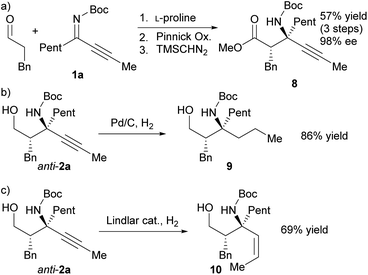 |
| Scheme 4 Transformations of the Mannich product anti-2a. | |
Conclusions
We have developed a diastereodivergent Mannich reaction of aldehydes with alkyl- and alkynyl-substituted Z-ketimines catalyzed by L-proline or the chiral phenylcyclopropane-based amine (S,R)-7. The newly synthesized N-Boc-protected ketimines, which cannot be accessed via conventional method, are effective for the present Mannich reaction. Hydrogenation of the obtained Mannich product provides access to less-accessible chiral α-tert-amine with two similar carbon chains. We believe that these results will find a broad range of applications in organic synthesis, enabling the preparation of valuable molecules for biological and pharmaceutical research.
Conflicts of interest
There are no conflicts to declare.
Acknowledgements
This work was supported by JSPS KAKENHI grants JP26220803, JP18H01975 and JP20H04815 (Hybrid Catalysis). The authors are grateful to Mr Y. Omatsu for performing X-ray crystallographic analyses.
Notes and references
- For review, see:
(a) S. Kobayashi and H. Ishitani, Chem. Rev., 1999, 99, 1069–1094 CrossRef CAS;
(b) A. E. Taggi, A. M. Hafez and T. Lectka, Acc. Chem. Res., 2003, 36, 10–19 CrossRef CAS;
(c) A. Córdova, Acc. Chem. Res., 2004, 37, 102–112 CrossRef;
(d) W. Notz, F. Tanaka and C. F. Barbas III, Acc. Chem. Res., 2004, 37, 580–591 CrossRef CAS;
(e) M. M. B. Marques, Angew. Chem., Int. Ed., 2006, 45, 348–352 CrossRef CAS;
(f) S. Mukherjee, J. W. Yang, S. Hoffmann and B. List, Chem. Rev., 2007, 107, 5471–5569 CrossRef CAS;
(g) A. Ting and S. E. Schaus, Eur. J. Org. Chem., 2007, 5797–5815 CrossRef CAS;
(h) G. K. Friestad and A. K. Mathies, Tetrahedron, 2007, 63, 2541–2569 CrossRef CAS;
(i) J. M. M. Verkade and L. J. C. van Hemert, Chem. Soc. Rev., 2008, 37, 29–41 RSC;
(j) P. Melchiorre, M. Marigo, A. Carlone and G. Bartoli, Angew. Chem., Int. Ed., 2008, 47, 6138–6171 CrossRef CAS;
(k) R. Gómez Arrayás and J. C. Carretero, Chem. Soc. Rev., 2009, 38, 1940–1948 RSC;
(l) B. Karimi, D. Enders and E. Jafari, Synthesis, 2013, 45, 2769–2812 CrossRef CAS.
- For review, see:
(a) G. Cardillo and C. Tomasini, Chem. Soc. Rev., 1996, 25, 117–128 RSC;
(b) M. Liu and M. P. Sibi, Tetrahedron, 2002, 58, 7991–8035 CrossRef CAS;
(c) D. Seebach, A. K. Beck, S. Capone, G. Deniau, U. Grošelj and E. Zass, Synthesis, 2009, 1–32 CrossRef CAS;
(d) B. Weiner, W. Szymański, D. B. Janssen, A. J. Minnaard and B. L. Feringa, Chem. Soc. Rev., 2010, 39, 1656–1691 RSC.
- For representative Mannich reactions using cyclic ketimines, see:
(a) S. Saaby, K. Nakama, M. A. Lie, R. G. Hazell and K. A. Jørgensen, Chem.–Eur. J., 2003, 9, 6145–6454 CrossRef CAS;
(b) W. Zhuang, S. Saaby and K. A. Jørgensen, Angew. Chem., Int. Ed., 2004, 43, 4476–4478 CrossRef;
(c) B. Jiang, J. J. Dong, Y. G. Si, X. L. Zhao, Z. G. Huang and M. Xua, Adv. Synth. Catal., 2008, 350, 1360–1366 CrossRef CAS;
(d) L. Li, M. Ham, M. Xiao and Z. Xie, Synlett, 2011, 1727–1730 CAS;
(e) T. Kano, S. Song, Y. Kubota and K. Maruoka, Angew. Chem., Int. Ed., 2012, 51, 1191–1194 CrossRef CAS;
(f) M. Rueping, R. Rasappan and S. Raja, Helv. Chim. Acta, 2012, 95, 2296–2303 CrossRef CAS;
(g) Y.-Q. Wang, Y. Zhang, K. Pan, J. You and J. Zhao, Adv. Synth. Catal., 2013, 355, 3381–3386 CrossRef CAS;
(h) Y.-Q. Wang, X.-Y. Cui, Y.-Y. Ren and Y. Zhang, Org. Biomol. Chem., 2014, 12, 9101–9104 RSC;
(i) S. Zhang, L. Li, Y. Hu, Z. Zha, Z. Wang and T.-P. Loh, Org. Lett., 2015, 17, 1050–1053 CrossRef CAS;
(j) B. Qiao, Y.-J. Huang, J. Nie and J.-A. Ma, Org. Lett., 2015, 17, 4608–4611 CrossRef CAS;
(k) Y. Luo, K. X. Xie, D. F. Yue, X. M. Zhang, X. Y. Xu and W. C. Yuan, Org. Biomol. Chem., 2018, 16, 3372–3375 RSC;
(l) H. Hu, J. Xu, W. Liu, S. Dong, L. Lin and X. Feng, Org. Lett., 2018, 20, 5601–5605 CrossRef CAS;
(m) Q. Shao, L. Wu, J. Chen, I. D. Gridnev, G. Yang, F. Xie and W. Zhang, Adv. Synth. Catal., 2018, 360, 4625–4633 CrossRef CAS;
(n) K. N. Reddy, M. V. K. Rao, B. Sridhar and B. V. S. Reddy, Chem.–Asian J., 2019, 14, 2958–2965 CrossRef CAS.
- For representative examples of sequential reactions with cyclic ketimines, see:
(a) M.-X. Zhao, H.-L. Bi, R.-H. Jiang, X.-W. Xu and M. Shi, Org. Lett., 2014, 16, 4566–4569 CrossRef CAS;
(b) M.-X. Zhao, Z.-W. Dong, G.-Y. Zhu, X.-L. Zhao and M. Shi, Org. Biomol. Chem., 2018, 16, 4641–4649 RSC;
(c) H.-J. Zhang, Y.-C. Xie and L. Yin, Nat. Commun., 2019, 10, 1699 CrossRef;
(d) X. Yuan, X. Wu, P. Zhang, F. Peng, C. Liu, H. Yang, C. Zhu and H. Fu, Org. Lett., 2019, 21, 2498–2503 CrossRef CAS;
(e) X. Liu, X. Yan, J.-H. Yu, Y.-D. Tang, K. Wang and H. Zhang, Org. Lett., 2019, 21, 5626–5629 CrossRef CAS;
(f) Z. Xu, X.-G. Wang, Y.-H. Wei, K.-L. Ji, J.-F. Zheng, J.-L. Ye and P.-Q. Huang, Org. Lett., 2019, 21, 7587–7591 CrossRef CAS.
- For representative Mannich reactions with non-cyclic ketimines, see:
(a) Y. Suto, M. Kanai and M. Shibasaki, J. Am. Chem. Soc., 2007, 129, 500–501 CrossRef CAS;
(b) R. Yazaki, T. Nitabaru, N. Kumagai and M. Shibasaki, J. Am. Chem. Soc., 2008, 130, 14477–14479 CrossRef CAS;
(c) Y. Du, L.-W. Xu, Y. Shimizu, K. Oisaki, M. Kanai and M. Shibasaki, J. Am. Chem. Soc., 2008, 130, 16146–16147 CrossRef CAS;
(d) V. A. Sukach, N. M. Golovach, V. V. Pirozhenko, E. B. Rusanov and M. V. Vovk, Tetrahedron: Asymmetry, 2008, 19, 761–764 CrossRef CAS;
(e) L. C. Wieland, E. M. Vieira, M. L. Snapper and A. H. Hoveyda, J. Am. Chem. Soc., 2009, 131, 570–576 CrossRef CAS;
(f) G. Lu, T. Yoshino, H. Morimoto, S. Matsunaga and M. Shibasaki, Angew. Chem., Int. Ed., 2011, 50, 4382–4385 CrossRef CAS;
(g) N. Hara, R. Tamura, Y. Funahashi and S. Nakamura, Org. Lett., 2011, 13, 1662–1665 CrossRef CAS;
(h) M. Hayashi, M. Sano, Y. Funahashi and S. Nakamura, Angew. Chem., Int. Ed., 2013, 52, 5557–5560 CrossRef CAS;
(i) L. Yin, H. Takada, N. Kumagai and M. Shibasaki, Angew. Chem., Int. Ed., 2013, 52, 7310–7313 CrossRef CAS;
(j) M. Hayashi, M. Iwanaga, N. Shiomi, D. Nakane, H. Masuda and S. Nakamura, Angew. Chem., Int. Ed., 2014, 53, 8411–8415 CrossRef CAS;
(k) S. Nakamura, R. Yamaji and M. Hayashi, Chem.–Eur. J., 2015, 21, 9615–9618 CrossRef CAS;
(l) S. Nakamura, R. Yamaji and M. Iwanaga, Chem. Commun., 2016, 52, 7462–7465 RSC;
(m) R. de la Campa, A. D. Gammack Yamagata, I. Ortín, A. Franchino, A. L. Thompson, B. Odell and D. J. Dixon, Chem. Commun., 2016, 52, 10632–10635 RSC;
(n) A. Franchino, J. Chapman, I. Funes-Ardoiz, R. S. Paton and D. J. Dixon, Chem.–Eur. J., 2018, 24, 17660–17664 CrossRef CAS.
- For representative Mannich reactions with isatin-derived ketimines, see:
(a) J. Kaur and S. S. Chimni, Org. Biomol. Chem., 2018, 16, 3328–3347 RSC;
(b) W. Yan, D. Wang, J. Feng, P. Li, D. Zhao and R. Wang, Org. Lett., 2012, 14, 2512–2515 CrossRef CAS;
(c) T.-Z. Li, X.-B. Wang, F. Sha and X.-Y. Wu, J. Org. Chem., 2014, 79, 4332–4339 CrossRef CAS;
(d) Z. Tang, Y. Shi, H. Mao, X. Zhu, W. Li, Y. Cheng, W.-H. Zheng and C. Zhu, Org. Biomol. Chem., 2014, 12, 6085–6088 RSC;
(e) J. Zhao, B. Fang, W. Luo, X. Hao, X. Liu, L. Lin and X. Feng, Angew. Chem., Int. Ed., 2015, 54, 241–244 CrossRef CAS;
(f) D. Engl, S. P. Fritz and H. Wennemers, Angew. Chem., Int. Ed., 2015, 54, 8193–8197 CrossRef;
(g) J. Dai, D. Xiong, T. Yuan, J. Liu, T. Chen and Z. Shao, Angew. Chem., Int. Ed., 2017, 56, 12697–12701 CrossRef CAS;
(h) Q. Huang, Y. Cheng, H. Yuan, X. Chang, P. Li and W. Li, Org. Chem. Front., 2018, 5, 3226–3230 RSC;
(i) Y. Liu, J. Wang, Z. Wei, J. Cao, D. Liang, Y. Lin and H. Duan, Org. Biomol. Chem., 2018, 16, 8927–8932 RSC;
(j) S. Yu, H. Noda, N. Kumagai and M. Shibasaki, Synlett, 2019, 30, 488–492 CrossRef;
(k) R. Ding, Z. A. De Los Santos and C. Wolf, ACS Catal., 2019, 9, 2169–2176 CrossRef CAS;
(l) J. Lu, Y. Fan, F. Sha, Q. Li and X. Y. Wu, Org. Chem. Front., 2019, 6, 2687–2691 RSC;
(m) P. Rodríguez-Ferrer, M. Sanz-Novo, A. Maestro, J. M. Andrés and R. Pedrosa, Adv. Synth. Catal., 2019, 361, 3645–3655 CrossRef;
(n) Z. Chang, C. Ye, J. Fu, P. Chigumbu, X. Zeng, Y. Wang, C. Jiang and X. Han, Adv. Synth. Catal., 2019, 361, 5516–5520 CrossRef CAS;
(o) M. Franc, M. Urban, I. Císařová and J. Veselý, Org. Biomol. Chem., 2019, 17, 7309–7314 RSC;
(p) Y. Fan, J. Lu, F. Sha, Q. Li and X. Y. Wu, J. Org. Chem., 2019, 84, 11639–11648 CrossRef CAS;
(q) S. Nakamura, K. Matsuzaka, T. Hatanaka and Y. Funahashi, Org. Lett., 2020, 22, 2868–2872 CrossRef CAS.
- For representative Mannich reactions with ketimines bearing a carbonyl moiety, see:
(a) S. Lin, N. Kumagai and M. Shibasaki, Chem.–Eur. J., 2016, 22, 3296–3299 CrossRef CAS;
(b) M. Sawa, K. Morisaki, Y. Kondo, H. Morimoto and T. Ohshima, Chem.–Eur. J., 2017, 23, 17022–17028 CrossRef CAS;
(c) Y. Zhou, Y. You, Z.-H. Wang, X.-M. Zhang, X.-Y. Xu and W.-C. Yuan, Eur. J. Org. Chem., 2019, 3112–3116 CrossRef CAS.
-
(a) B. M. Trost, C.-I. J. Hung and M. J. Scharf, Angew. Chem., Int. Ed., 2018, 57, 11408–11412 CrossRef CAS;
(b) J. Dai, Z. Wang, Y. Deng, L. Zhu, F. Peng, Y. Lan and Z. Shao, Nat. Commun., 2019, 10, 5182 CrossRef.
- To the best of our knowledge, there are only two examples of asymmetric reaction of ketimines with two similar alkyl groups, see:
(a) N. Kato, M. Suzuki, M. Kanai and M. Shibasaki, Tetrahedron Lett., 2004, 45, 3147–3151 CrossRef CAS;
(b) S. Lin, Y. Kawato, N. Kumagai and M. Shibasaki, Angew. Chem., Int. Ed., 2015, 54, 5183–5186 CrossRef CAS.
- T. Kano, R. Kobayashi and K. Maruoka, Org. Lett., 2016, 18, 276–279 CrossRef CAS.
- T. Kano, Y. Aota and K. Maruoka, Angew. Chem., Int. Ed., 2017, 56, 16293–16296 CrossRef CAS.
- Asymmetric reactions of alkynyl-substituted ketimines, see:
(a) Y. Yao, J.-L. Li, Q.-Q. Zhou, L. Dong and Y.-C. Chen, Chem.–Eur. J., 2013, 19, 9447–9451 CrossRef CAS;
(b) M.-W. Chen, B. Wu, Z.-P. Chen and Y. Zhou, Org. Lett., 2016, 18, 4650–4653 CrossRef CAS;
(c) M. Hatano, H. Okamoto, T. Kawakami, K. Toh, H. Nakatsuji, A. Sakakura and K. Ishihara, Chem. Sci., 2018, 9, 6361–6367 RSC.
- For representative examples of both alkyl and alkynyl-substituted ketimines, see:
(a) T. Kobayashi, T. Sakakura and M. Tanaka, Tetrahedron Lett., 1985, 26, 3463–3466 CrossRef CAS;
(b) Y. Ito, M. Inouye and M. Murakami, Chem. Lett., 1989, 18, 1261–1264 CrossRef;
(c) W. M. David and S. M. Kerwin, J. Am. Chem. Soc., 1997, 119, 1464–1465 CrossRef CAS;
(d) A. V. Kel’in, A. W. Sromek and V. Gevorgyan, J. Am. Chem. Soc., 2001, 123, 2074–2075 CrossRef;
(e) B. Van den Hoven and H. Alper, J. Am. Chem. Soc., 2001, 123, 10214–10220 CrossRef CAS.
- For representative stereoselective or diastereodivergent reactions using amine catalysts, see:
(a) B. List, J. Am. Chem. Soc., 2000, 122, 9336–9337 CrossRef CAS;
(b) B. List, P. Pojarliev, W. T. Biller and H. J. Martin, J. Am. Chem. Soc., 2002, 124, 827–833 CrossRef CAS;
(c) A. Córdova, W. Notz, G. Zhong, J. M. Betancort and C. F. Barbas III, J. Am. Chem. Soc., 2002, 124, 1842–1843 CrossRef;
(d) A. Córdova, S. Watanabe, F. Tanaka, W. Notz and C. F. Barbas III, J. Am. Chem. Soc., 2002, 124, 1866–1867 CrossRef;
(e) A. Córdova and C. F. Barbas III, Tetrahedron Lett., 2002, 43, 7749–7752 CrossRef;
(f) Y. Hayashi, W. Tsuboi, I. Ashimine, T. Urushima, M. Shoji and K. Sakai, Angew. Chem., Int. Ed., 2003, 42, 3677–3680 CrossRef CAS;
(g) A. Córdova, Chem.–Eur. J., 2004, 10, 1987–1997 CrossRef;
(h) J. Franzén, M. Marigo, D. Fielenbach, T. C. Wabnitz, A. Kjærsgaard and K. A. Jørgensen, J. Am. Chem. Soc., 2005, 127, 18296–18304 CrossRef;
(i) B. Westermann and C. Neuhaus, Angew. Chem., Int. Ed., 2005, 44, 4077–4079 CrossRef CAS;
(j) D. Enders, C. Grondal, M. Vrettou and G. Raabe, Angew. Chem., Int. Ed., 2005, 44, 4079–4083 CrossRef CAS;
(k) A. J. A. Cobb, D. M. Shaw, D. A. Longbottom, J. B. Gold and S. V. Ley, Org. Biomol. Chem., 2005, 3, 84–96 RSC;
(l) S. Mitsumori, H. Zhang, P. H.-Y. Cheong, K. N. Houk, F. Tanaka and C. F. Barbas III, J. Am. Chem. Soc., 2006, 128, 1040–1041 CrossRef CAS;
(m) J. W. Yang, M. Stadler and B. List, Angew. Chem., Int. Ed., 2007, 46, 609–611 CrossRef CAS;
(n) V. Bisai, R. A. Unhale, A. Suneja, S. Dhanasekaran and V. K. Singh, Org. Lett., 2015, 17, 2102–2105 CrossRef CAS.
- The reaction between the ketone (1.0 eq.) and N-Boc-imino-(triphenyl)phosphorene (1.1 eq.) in toluene at 80 °C furnished only the enecarbamate.
- To confirm the stereochemistry, ketimine 1, which bears pentyl and 1-hexynyl groups, was synthesized by a known method that affords Z-ketimines. Although the ketimine could not be isolated due to the simultaneous formation of inseparable byproducts, the observed stereoisomer was identical to the one prepared using our method. See also: B. M. Trost and B. Biannic, Org. Lett., 2015, 17, 1433–1436 CrossRef CAS.
-
(a) T. Kano, Y. Yamaguchi, O. Tokuda and K. Maruoka, J. Am. Chem. Soc., 2005, 127, 16408–16409 CrossRef CAS;
(b) T. Kano, Y. Yamaguchi and K. Maruoka, Angew. Chem., Int. Ed., 2009, 48, 1838–1840 CrossRef CAS;
(c) T. Kano, Y. Yamaguchi and K. Maruoka, Chem.–Eur. J., 2009, 15, 6678–6687 CrossRef CAS . See also ref 3e.
- A. Takeshima, T. Kano and K. Maruoka, Org. Lett., 2019, 21, 8071–8074 CrossRef CAS.
-
(a)
C. G. McCarty, in The Chemistry of the Carbon-Nitrogen Double Bond, ed. S. Patai, Interscience, London, 1969, pp 363–464 Search PubMed;
(b) J. Bjørgo, D. R. Boyd, C. G. Watson and W. B. Jennings, J. Chem. Soc., Perkin Trans. 2, 1974, 757–762 RSC.
- C. Zhao, A. Fu, H. Li, C. Tian, Z. Wang, S. Yuan and Y. Duan, Comput. Theor. Chem., 2013, 1018, 77–84 CrossRef CAS.
Footnote |
† Electronic supplementary information (ESI) available. CCDC 1979814. For ESI and crystallographic data in CIF or other electronic format see DOI: 10.1039/d0sc05269h |
|
This journal is © The Royal Society of Chemistry 2021 |
Click here to see how this site uses Cookies. View our privacy policy here.