DOI:
10.1039/D1RA07086J
(Paper)
RSC Adv., 2021,
11, 35156-35160
Sodium iodide-mediated synthesis of vinyl sulfides and vinyl sulfones with solvent-controlled chemical selectivity†
Received
22nd September 2021
, Accepted 13th October 2021
First published on 31st October 2021
Abstract
Vinyl sulfides and vinyl sulfones are ubiquitous structures in organic chemistry because of their presence in natural and biologically active compounds and are very frequently encountered structural motifs in organic synthesis. Herein we report an efficient synthesis of vinyl sulfides and vinyl sulfones via transition metal-free sodium iodide-mediated sulfenylation of alcohols and sulfinic acids with solvent-controlled selectivity.
Introduction
Functionalized olefins are valuable intermediates in organic synthesis, because of their ability to serve as convenient substrates in transition metal-catalyzed cross-coupling reactions and their presence in natural and biologically active compounds.1 In this respect, sulfur-containing olefins as important building units or intermediates have been applied in many different fields, such as total synthesis, functional materials science and medicinal chemistry.2 For example, vinyl sulfides are employed as precursors for enol substituents,3 Michael acceptors,4 in cycloaddition reactions,5 in olefin metathesis,6 as reagents in cross-coupling,7 in Heck reactions,8 as equivalents of enolate anions,9 and in aldehyde/ketone formation.10 Vinyl sulfones and their derivatives have been reported to be potent inhibitors of enzymes such as sortase,11 protein tyrosine phosphatases,12 and cysteine proteases.13 In view of their significance, developing more practical and efficient synthetic approaches to construct these compounds is of great interest.14 Two common methods are frequently utilized for the synthesis of vinyl sulfides, including the copper-catalyzed S-vinylation of thiols with vinyl halides and the addition of thiols to alkynes.15 Recently, Zhang and co-workers reported a copper-mediated stereospecific C–H oxidative sulfenylation of terminal alkenes with disulfides.14 For the synthesis of vinyl sulfones, Wittig reaction of α-sulfinyl phosphonium ylides, β-elimination of halosulfones, condensation of aldehydes with sulfonylacetic, and the oxidation of the corresponding sulfides are perhaps the most common synthesis methods.16 Recently, Zhan's group reported a copper(II)-catalyzed chemo- and stereocontrolled synthesis of vinyl sulfones from terminal alkynes and arylsulfonyl hydrazides.2 Kuhakarn and co-workers discovered an efficient method for the synthesis of (E)-vinyl sulfones via decarboxylative sulfonylation of arylpropiolic acids with sulfinic acids.14 In spite of the great success achieved so far in this area, there are still certain limitations, such as difficulties in controlling stereoselectivities and chemoselectivities, expensive substrates, moderate scope and functional group tolerance. Therefore, a new general, flexible, high stereoselective and chemoselective approach for the synthesis of vinyl sulfides and vinyl sulfones is still necessary.
Sulfinic acids are readily accessible and have been widely used as sulfur sources in medicinal chemistry.17 Moreover, sulfinic acids can be reduced to disulfides, which are active intermediates that can be used as sulfenylating agents. So we envisioned that sulfinic acids might be served as potential sulfur sources to react with alcohols under certain reaction conditions. To our knowledge, there are no examples describing the reaction of sulfinic acids and alcohols. Herein we report an efficient synthesis of vinyl sulfides and vinyl sulfones via transition metal-free sodium iodide-mediated sulfenylation of alcohols with sulfinic acids.
Results and discussion
To obtain the optimized reaction conditions, several acids (10 mol%) were examined in the model reaction of alcohol 1a with benzenesulfinic acid 2a using tetrabutylammonium iodide as an additive in 1,2-dichloroethane at 80 °C (Table 1, entries 1–4). To our delight, TsOH·H2O was identified as the acid of choice, which promoted the formation of vinyl sulfide 3a in 61% yield (Table 1, entry 1). Iodine and several iodides such as NaI, KI were investigated to improve the reaction efficiency (Table 1, entries 5–7). Among the above iodides examined, NaI was found to be the most effective additive to give the target product 3a in 70% yield (Table 1, entry 5). Further solvent screening showed interesting results: (1) we observed the generation of vinyl sulfone 4a in MeCN (Table 1, entry 8). (2) MeNO2 was the best solvent for the formation of 4a, and improved the yield to 59% yield (Table 1, entry 9). (3) DCE was the best solvent for the formation of 3a. These results demonstrate that solvent plays an important role in controlling the chemoselectivity of the reaction, which could be explained by the fact that MeNO2 is beneficial to the production of free radical intermediates. A high yield was obtained when the loading of sodium iodide was increased to 1.5 equivalents (Table 1, entry 15). Further efforts to increase the yield of vinyl sulfide 3a by increasing the loading of TsOH·H2O to 0.2 equivalent resulted in a higher yield of 90% (Table 1, entry 16). Similarly, the yield of 4a was increased to 86% in the presence of 1.5 equivalents of NaI and 20 mol% TsOH·H2O in MeNO2.
Table 1 Optimization of reaction conditionsa

|
Entry |
Solvent |
Additive (eq.) |
Acid (eq.) |
Yieldb (%) 3a |
Yieldb (%) 4a |
Reaction conditions: alcohol 1a (0.20 mmol), benzenesulfinic acid 2a (0.30 mmol), additive, acid, solvent (1.0 mL), 80 °C, 24 h. Isolated yield. |
1 |
DCE |
n-Bu4NI (1.0) |
TsOH·H2O (0.1) |
61 |
0 |
2 |
DCE |
n-Bu4NI (1.0) |
HCl (0.1) |
57 |
0 |
3 |
DCE |
n-Bu4NI (1.0) |
TfOH (0.1) |
53 |
0 |
4 |
DCE |
n-Bu4NI (1.0) |
H2SO4 (0.1) |
58 |
0 |
5 |
DCE |
NaI (1.0) |
TsOH·H2O (0.1) |
70 |
0 |
6 |
DCE |
KI (1.0) |
TsOH·H2O (0.1) |
63 |
0 |
7 |
DCE |
I2 (1.0) |
TsOH·H2O (0.1) |
0 |
0 |
8 |
MeCN |
NaI (1.0) |
TsOH·H2O (0.1) |
18 |
44 |
9 |
MeNO2 |
NaI (1.0) |
TsOH·H2O (0.1) |
Trace |
59 |
10 |
Toluene |
NaI (1.0) |
TsOH·H2O (0.1) |
42 |
30 |
11 |
DMSO |
NaI (1.0) |
TsOH·H2O (0.1) |
17 |
21 |
12 |
DMF |
NaI (1.0) |
TsOH·H2O (0.1) |
Trace |
15 |
13 |
Dioxane |
NaI (1.0) |
TsOH·H2O (0.1) |
30 |
0 |
14 |
EtOH |
NaI (1.0) |
TsOH·H2O (0.1) |
Trace |
39 |
15 |
DCE |
NaI (1.5) |
TsOH·H2O (0.1) |
78 |
Trace |
16 |
DCE |
NaI (1.5) |
TsOH·H2O (0.2) |
90 |
Trace |
17 |
MeNO2 |
NaI (1.5) |
TsOH·H2O (0.2) |
Trace |
86 |
Encouraged by our preliminary findings, the substrate scope of alcohols and sulfinic acids for the synthesis of vinyl sulfides was explored, and the results were shown in Table 2. In the presence of 1.5 equivalents of NaI, 20 mol% TsOH·H2O and DCE as solvent, a broad range of bisbenzylic, monobenzylic and naphthyl alcohols smoothly underwent this kind of transformation, generating structurally diverse vinyl sulfide 3 in moderate to excellent yields with extremely high stereoselectivity (Table 2, entries 1–11). The reactivity of monoaryl alcohols is lower than diaryl alcohol. Notably, this protocol proved useful for the construction of vinyl sulfides containing polycycles such as 3d–f (Table 2, entries 4–6). However, this protocol was not applicable to less reactive alkyl alcohols (Table 2, entry 9) substrate scope is also broad with respect to the sulfinic acids. Various arylsulfinic acids smoothly reacted with alcohol 1a to give the corresponding vinyl sulfides in good to excellent yields (Table 2, entries 12–22). It is noteworthy that both electron-withdrawing and electron-donating groups were introduced into the vinyl sulfides by employing arylsulfinic acid bearing such groups on the aromatic ring. Furthermore, alkylsulfinic acids could also smoothly react with alcohol 1a to give the corresponding vinyl sulfides (Table 2, entries 23–25). But the yield is lower than arylsulfinic acids, this may be due to the low activity of alkylsulfinic acids.
Table 2 Sulfenylation of alcohols with sulfinic acidsa
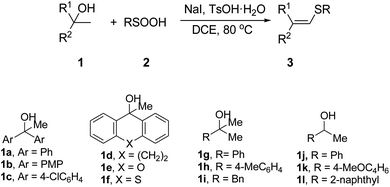
|
Entry |
1 |
2 |
Product 3 |
Yieldb (%) |
Reaction conditions: alcohol 1 (0.20 mmol), sulfinic acid 2 (0.30 mmol), NaI (0.30 mmol), TsOH·H2O (0.040 mmol), DCE (1.0 mL), 80 °C, 24 h. Isolated yield. |
1 |
1a |
2a |
 |
3aa, Ar = Ph |
90 |
2 |
1b |
2a |
3ba, Ar = PMP |
83 |
3 |
1c |
2a |
3ca, Ar = 4-ClC6H4 |
89 |
4 |
1d |
2a |
 |
3da, X = (CH2)2 |
91 |
5 |
1e |
2a |
3ea, X = O |
81 |
6 |
1f |
2a |
3fa, X = S |
77 |
7 |
1g |
2a |
 |
3ga, R = Ph |
65 |
8 |
1h |
2a |
3ha, R = 4-MeC6H4 |
69 |
9 |
1i |
2a |
3ia, R = Bn |
0 |
10 |
1j |
2a |
RCH = CHSPh |
3ja, R = Ph |
43 |
11 |
1k |
2a |
3ka, R = 2-naphthyl |
56 |
12 |
1a |
2b |
 |
3ab, R = 4-MeC6H4 |
91 |
13 |
1a |
2c |
3ac, R = 4-tBuC6H4 |
88 |
14 |
1a |
2d |
3ad, R = 4-MeOC6H4 |
85 |
15 |
1a |
2e |
3ae, R = 4-FC6H4 |
93 |
16 |
1a |
2f |
3af, R = 4-ClC6H4 |
89 |
17 |
1a |
2g |
3ag, R = 4-BrC6H4 |
86 |
18 |
1a |
2h |
3ah, R = 4-IC6H4 |
81 |
19 |
1a |
2i |
3ai, R = 3-O2NC6H4 |
90 |
20 |
1a |
2j |
3aj, R = 2,4-Cl2C6H4 |
83 |
21 |
1a |
2k |
3ak, 1-naphthyl |
98 |
22 |
1a |
2l |
3al, 2-naphthyl |
99 |
23 |
1a |
2m |
3am, Me(CH2)7 |
72 |
24 |
1a |
2n |
3an, Bn |
76 |
25 |
1a |
2o |
3ao, CH3 |
67 |
Next, the substrate scope of alcohols and sulfinic acids for the synthesis of vinyl sulfones was explored, and the results were shown in Table 3. In the presence of 1.5 equivalents of NaI, 20 mol% TsOH·H2O and MeNO2 as solvent, a broad range of bisbenzylic and monobenzylic alcohols were found to react well with benzenesulfinic acids to yield structurally diverse vinyl sulfones derivatives in good to excellent yields with extremely high stereoselectivity (Table 3, entries 1–7). The results represented in Table 3 show that the scope of sulfinic acids is also very general in the sulfonylation of alcohols. A number of arylsulfinic acids bearing either electron-donating groups or electron-withdrawing groups on the aromatic rings were transformed into their corresponding vinyl sulfones at 80 °C in good to excellent yields (Table 3, entries 8–14). Moreover, alkylsulfinic acids could also smoothly react with alcohol 1a to give the corresponding vinyl sulfones (Table 3, entry 15).
Table 3 Sulfonylation of alcohols with sulfinic acidsab
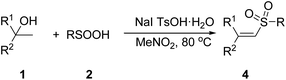
|
Entry |
1 |
2 |
Product |
Yieldb (%) |
Reaction conditions: alcohol 1 (0.20 mmol), sulfinic acid 2 (0.30 mmol), NaI (0.30 mmol), TsOH·H2O (0.040 mmol), MeNO2 (1.0 mL), 80 °C, 24 h. Isolated yield. |
1 |
1a |
2a |
 |
4aa, Ar = Ph |
86 |
2 |
1b |
2a |
4ba, Ar = PMP |
93 |
3 |
1c |
2a |
4ca, Ar = 4-ClC6H4 |
88 |
4 |
1d |
2a |
 |
4da, X = (CH2)2 |
90 |
5 |
1f |
2a |
4fa, X = S |
81 |
6 |
1g |
2a |
 |
4ga |
75 |
7 |
1j |
2a |
 |
4ja |
67 |
8 |
1a |
2b |
 |
4ab, R = 4-MeC6H4 |
91 |
9 |
1a |
2c |
4ac, R = 4-tBuC6H4 |
85 |
10 |
1a |
2d |
4ad, R = 4-MeOC6H4 |
82 |
11 |
1a |
2e |
4ae, R = 4-FC6H4 |
73 |
12 |
1a |
2f |
4af, R = 4-ClC6H4 |
87 |
13 |
1a |
2k |
4ak, 1-naphthyl |
90 |
14 |
1a |
2l |
4al, 2-naphthyl |
95 |
15 |
1a |
2m |
4am, Me(CH2)7 |
63 |
In order to gain further insight into the reaction mechanism, four control experiments were set up under various reaction conditions. Benzenesulfinic acid was reduced to diphenyldisulfane 2a′ in 94% yield in the presence of 1.5 equivalents of NaI, 20 mol% TsOH·H2O and DCE as solvent (Scheme 1a). Under the 1.0 equivalents of iodine, diphenyldisulfane 2a′ smoothly reacted with alcohol 1a to give the corresponding vinyl sulfide 3ab in 91% yield (Scheme 1b). Under the standard reaction conditions, alcohol 1a and diphenyldisulfane 2a′ don't react (Scheme 1c). In the presence of 1.5 equivalents of NaI, 20 mol% TsOH and DCE as solvent, alkene 6a was found to react smoothly with benzenesulfinic acids to give vinyl sulfide 3ab in 93% yields (Scheme 1d).
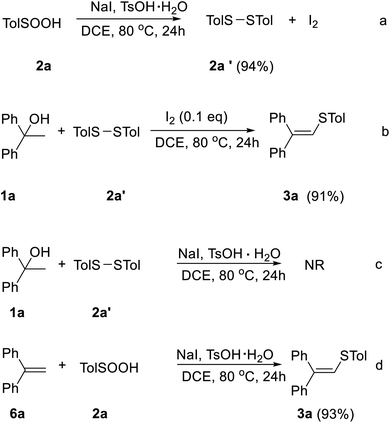 |
| Scheme 1 Control experiments (a–d). | |
On the basis of the above experimental results and previous relevant mechanism studies,14,18 we proposed the reaction pathway depicted in Scheme 2. Initially, alcohol 1 undergo elimination reaction to form alkene 6 under TsOH and heating conditions. Meanwhile, 1,2-diphenyldisulfane (2a′) is generated from sulfinic acid 2 in the presence of NaI and TsOH. Then 2a′ reacts with I2 to give the sulfenyl iodide 5, which is attacked by 6 to give intermediate 7. Finally, elimination of HI from 7 affords the corresponding vinyl sulfide 3.
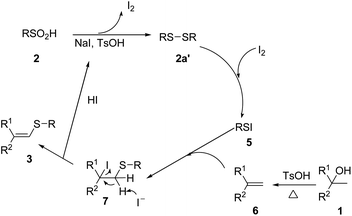 |
| Scheme 2 Proposed reaction pathway for the synthesis of vinyl sulfides. | |
We performed a relevant control experiment for vinyl sulfones (Scheme 3). When a stoichiometric amount of TEMPO (2,2,6,6-tetramethyl-1-piperidinyloxy, a well known radical-capturing species) was added to this reaction mixture, the formation of 4a was completely inhibited in the reaction. Therefore, we reason that a radical process should be involved in this reaction.
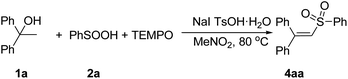 |
| Scheme 3 Control experiment for the generation of vinyl sulfone. | |
On the basis of the preliminary result above and previous relevant mechanism studies,14 we proposed a plausible mechanism for the generation of vinyl sulfones in Scheme 4. First, alcohol 1 undergo elimination reaction to form alkene 6 under TsOH and heating conditions. Meanwhile, sulfonyl iodide 8 was generated from benzenesulfinic acid in the presence of NaI under air. Then, 8 experienced homolytic cleavage to produce a sulfonyl radical 9, which interacted with alkene 6 to give intermediate 10. Next, intermediate 10 reacts with an iodine radical to give intermediate 11. Finally, elimination of HI from 11 produces the corresponding vinyl sulfone 4.
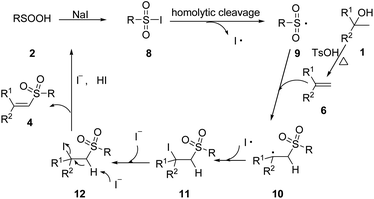 |
| Scheme 4 Proposed reaction pathway for the synthesis of vinyl sulfones. | |
Conclusions
In conclusion, we have developed a NaI mediated elimination/coupling reaction of alcohols and sulfinic acids, constructing structurally diverse vinyl sulfides and vinyl sulfones. Solvent is crucial for the chemical selectivity. Solvent-controlled selectivity of the elimination/coupling reaction was realized for divergent synthesis of vinyl sulfides and vinyl sulfones. The described protocol is more convenient for the preparation of vinyl sulfide than vinyl sulfone related skeletons and the starting materials are readily available. Current efforts are directed toward further methodological refinement and synthetic applications.
Experimental
General procedure for the synthesis of vinyl sulfides (Table 2)
To a solution of alcohol 1 (0.20 mmol) in DCE (1.0 mL) under an air atmosphere at room temperature were added sulfinic acid 2 (0.30 mmol), NaI (45.0 mg, 0.30 mmol) and TsOH·H2O (7.6 mg, 0.040 mmol). The mixture was stirred at 80 °C for 24 h, cooled to room temperature, and directly purified by preparative thin layer chromatography on silica gel, developing with petroleum ether/ethyl acetate (100
:
0 to 20
:
1), to give compound 3.
General procedure for the synthesis of vinyl sulfones (Table 3)
To a solution of alcohol 1 (0.20 mmol) in MeNO2 (1.0 mL) under an air atmosphere at room temperature were added sulfinic acid 2 (0.30 mmol), NaI (45.0 mg, 0.30 mmol) and TsOH·H2O (7.6 mg, 0.040 mmol). The mixture was stirred at 80 °C for 24 h, cooled to room temperature, and directly purified by preparative thin layer chromatography on silica gel, developing with petroleum ether/ethyl acetate (20
:
1 to 5
:
1), to give compound 4.
Conflicts of interest
There are no conflicts to declare.
Acknowledgements
We are grateful for the financial support from the National Natural Science Foundation of China (No. 21502182, 21202154), Key Laboratory of Watershed Geographic Sciences, Nanjing Institute of Geography and Limnology, Chinese Academy of Sciences (WSGS2017005), the Cooperation Fund of Energy Research Institute, Nanjing Institute of Technology (CXY201927).
Notes and references
-
(a) S. E. Denmark and J. Amburgey, J. Am. Chem. Soc., 1993, 115, 10386 CrossRef CAS;
(b) J. D. White and M. S. Jensen, Tetrahedron, 1995, 51, 5743 CrossRef CAS;
(c) I. Creton, I. Marek and J. F. Normant, Synthesis, 1996, 12, 1499 CrossRef;
(d) S. D. Brown and R. W. Armstrong, J. Am. Chem. Soc., 1996, 118, 6331 CrossRef CAS;
(e) J. Fauvarque, Pure Appl. Chem., 1996, 68, 1713 CAS;
(f) M. G. Organ, J. T. Cooper, L. R. Rogers, F. Soleymanzade and T. Paul, J. Org. Chem., 2000, 65, 7959 CrossRef CAS PubMed;
(g) D. Zhang and J. M. Ready, Org. Lett., 2005, 7, 5681 CrossRef CAS PubMed;
(h) J. R. Debergh, K. M. Spivey and J. M. Ready, J. Am. Chem. Soc., 2008, 130, 7828 CrossRef CAS PubMed;
(i) C. Wang, Z. Q. Xu, T. Tobrman and E. Negishi, Adv. Synth. Catal., 2010, 352, 627 CrossRef CAS PubMed;
(j) C. R. Liu, Y. B. Xue, L. H. ding, H. Y. Zhang and F. L. Yang, Eur. J. Org. Chem., 2018, 6537 CrossRef CAS.
-
(a) S. V. Ley and A. W. Thomas, Angew. Chem., Int. Ed., 2003, 42, 5400 CrossRef CAS PubMed;
(b) T. Kondo and T. Mitsudo, Chem. Rev., 2000, 100, 3205 CrossRef CAS PubMed;
(c) D. Enders, S. F. Muller, G. Raabe and J. Runsink, Eur. J. Org. Chem., 2000, 879 CrossRef CAS;
(d) M. E. Lebrun, P. L. Marquand and C. Berthelette, J. Org. Chem., 2006, 71, 2009 CrossRef CAS PubMed;
(e) P. Mauleon, I. Alonso, M. R. Rivero and J. C. Carretero, J. Org. Chem., 2007, 72, 9924 CrossRef CAS PubMed;
(f) I. P. Beletskaya and V. P. Ananikov, Chem. Rev., 2011, 111, 1596 CrossRef CAS PubMed;
(g) D. V. Partyka, Chem. Rev., 2011, 111, 1529 CrossRef CAS PubMed;
(h) X. T. Liu, Z. C. Ding, S. X. Xu and Z. P. Zhan, Synthesis, 2017, 49, 1575 CrossRef CAS.
- B. M. Trost and A. C. Lavoie, J. Am. Chem. Soc., 1983, 105, 5075 CrossRef CAS.
- R. D. Miller and R. Hassig, Tetrahedron Lett., 1985, 26, 2395 CrossRef CAS.
-
(a) P. P. Singh, A. K. Yadav, H. Ita and H. Junjappa, J. Org. Chem., 2009, 74, 5496 CrossRef CAS PubMed;
(b) S. Serra, C. Fugnti and A. Moro, J. Org. Chem., 2001, 66, 7883 CrossRef CAS PubMed;
(c) F. E. Mcdoald, S. A. Burova and L. G. Huffman, Synthesis, 2000, 970 CrossRef;
(d) S. Yamazaki, Synth. Org. Chem., 2000, 58, 50 CrossRef CAS;
(e) J. Adrio and J. C. Carretero, J. Am. Chem. Soc., 1999, 121, 7411 CrossRef CAS;
(f) R. Bruckner and R. Huisgen, Tetrahedron Lett., 1990, 31, 2561 CrossRef;
(g) D. Singleton and K. M. Church, J. Org. Chem., 1990, 55, 4780 CrossRef CAS;
(h) R. B. Gupta, R. W. Franck, K. D. Onan and C. E. Soll, J. Org. Chem., 1989, 54, 1097 CrossRef CAS.
-
(a) Z. Liu and J. D. Rainier, Org. Lett., 2005, 7, 131 CrossRef CAS PubMed;
(b) M. L. Macnaughtan, J. B. Gary, D. L. Gerlach, M. J. A. Johnson and J. W. Kampf, Organometallics, 2009, 28, 2880 CrossRef CAS.
-
(a) A. Sabarre and J. Love, Org. Lett., 2008, 10, 3941 CrossRef CAS PubMed;
(b) E. Wenkert, M. E. Shepard and A. T. Mcphail, J. Chem. Soc., Chem. Commun., 1986, 1390 RSC;
(c) V. Fiandanese, G. Harchese, F. Naso and L. Ronsini, Chem. Commun., 1982, 647 RSC;
(d) E. Venkert, T. W. Ferreira and E. L. Michelotti, Chem. Commun., 1979, 637 RSC.
-
(a) N. Muraoka, M. Mineno, K. Itami and J. Yoshida, J. Org. Chem., 2005, 70, 6933 CrossRef CAS PubMed;
(b) K. Itami, M. Mineno, N. Muraoka and J. Yoshida, J. Am. Chem. Soc., 2004, 126, 11778 CrossRef CAS PubMed;
(c) P. Mauleon, A. A. Nunez, J. Alonso and J. C. Carretero, Chem.–Eur. J., 2003, 9, 1511 CrossRef CAS PubMed;
(d) B. M. Trost and Y. Tanigawa, J. Am. Chem. Soc., 1979, 101, 4743 CrossRef CAS.
- B. M. Trost and A. C. Lavoie, J. Am. Chem. Soc., 1983, 105, 5075 CrossRef CAS.
- B. A. Trofimov and B. A. Shainyna, in The Chemistry of Sulfur-Containing Functional Groups, ed. S. Patai and Z. Rappoport, John Wiley and Sons, Chichester, 1993, p. 659 Search PubMed.
-
(a) B. A. Frankel, M. Bentley, R. G. Kruger and D. G. Mccafferty, J. Am. Chem. Soc., 2004, 126, 3404 CrossRef CAS PubMed;
(b) K. V. Kudryavtsev, M. L. Bentley and D. G. Mccafferty, Bioorg. Med. Chem., 2009, 17, 2886 CrossRef CAS PubMed.
- S. Liu, B. Zhou, H. Yang, Y. He, Z.-X. Jiang, S. Kumar, L. Wu and Z. Y. Zhang, J. Am. Chem. Soc., 2008, 130, 8251 CrossRef CAS PubMed.
-
(a) A. Singh and P. J. Rosenthal, J. Biol. Chem., 2004, 279, 35236 CrossRef CAS PubMed;
(b) W. R. Roush, S. L. Gwaltney, J. Cheng, K. A. Scheidt, J. H. Mckerrow and E. Hansell, J. Am. Chem. Soc., 1998, 120, 10994 CrossRef CAS.
-
(a) Y. Yatsumonji, O. Okada, A. Tsubouchi and T. Takeda, Tetrahedron, 2006, 62, 9981 CrossRef CAS;
(b) H. L. Kao and C. F. Lee, Org. Lett., 2011, 13, 5204 CrossRef CAS PubMed;
(c) I. G. Trostyanskaya and I. P. Beletskaya, Synlett, 2012, 23, 535 CrossRef CAS;
(d) H. Y. Tu, B. L. Hu, C. L. Deng and X. G. Zhang, Chem. Commun., 2015, 51, 15558 RSC;
(e) N. Zhang, D. S. Yang, W. Wei, L. Yuan, Y. J. Cao and H. Wang, RSC Adv., 2015, 5, 37013 RSC;
(f) J. Meesin, P. Katrun, V. Reutrakul, M. Pohmakotr, D. Soorukram and C. Kuhakarn, Tetrahedron, 2016, 72, 1440 CrossRef CAS;
(g) D. Y. Wang, R. X. Zhang, W. Ning, Z. H. Yan and S. Lin, Org. Biomol. Chem., 2016, 14, 5136 RSC.
-
(a) C. G. Bates, P. Saejueng, M. Q. Doherty and D. Venkataraman, Org. Lett., 2004, 6, 5005 CrossRef CAS PubMed;
(b) I. P. Beletskaya and V. P. Ananikov, Chem. Rev., 2011, 111, 1596 CrossRef CAS PubMed;
(c) C. C. Eichman and J. P. Stambuli, Molecules, 2011, 16, 590 CrossRef CAS PubMed;
(d) B. W. Wang, K. Jiang, J. X. Li, S. H. Luo, Z. Y. Wang and H. F. Jiang, Angew. Chem., Int. Ed., 2020, 59, 2338 CrossRef CAS PubMed.
-
(a) J. H. van Steenis, J. J. G. S. van Es and A. van der Gen, Eur. J. Org. Chem., 2000, 2787 CrossRef CAS;
(b) A. A. Linden, L. Kruger and J. Bäckvall, J. Org. Chem., 2003, 68, 5890 CrossRef CAS PubMed;
(c) Q. Xue, Z. Mao, Y. Shi, H. Mao, Y. Cheng and C. Zhu, Tetrahedron Lett., 2012, 53, 1851 CrossRef CAS.
-
(a) C. J. Dinsmore, T. M. Williams, T. J. O'Neill, D. Liu, E. Rands, J. C. Culberson, R. B. Lobell, K. S. Koblan, N. E. Kohl, J. B. Gibbs, A. I. Oliff, S. L. Graham and G. D. Hartman, Bioorg. Med. Chem. Lett., 1999, 9, 3301 CrossRef CAS PubMed;
(b) Z.-Y. Sun, E. Botros, A.-D. Su, Y. Kim, E. Wang, N. Z. Baturay and C.-H. Kwon, J. Med. Chem., 2000, 43, 4160 CrossRef CAS PubMed;
(c) C.-R. Liu, M.-B. Li, D.-J. Cheng, C.-F. Yang and S.-K. Tian, Org. Lett., 2009, 11, 2543 CrossRef CAS PubMed;
(d) C.-R. Liu and M.-B. Li, Chin. J. Chem., 2013, 31, 1274 CrossRef CAS;
(e) C.-R. Liu, F.-L. Yang and T.-T. Wang, Chin. J. Chem., 2014, 32, 387 CrossRef CAS.
- C. R. Liu and L. H. Ding, Org. Biomol. Chem., 2015, 13, 2251 RSC.
Footnote |
† Electronic supplementary information (ESI) available. See DOI: 10.1039/d1ra07086j |
|
This journal is © The Royal Society of Chemistry 2021 |