DOI:
10.1039/D1RA06363D
(Paper)
RSC Adv., 2021,
11, 32419-32424
Development of highly gas-permeable polymers by metathesis copolymerization of 1-(p-trimethylsilyl)phenyl-1-propyne with tert-butyl and silyl group-containing diphenylacetylenes†
Received
23rd August 2021
, Accepted 27th September 2021
First published on 1st October 2021
Abstract
The development of highly gas-permeable membranes is required for gas separation applications. In this study, 1-(p-trimethylsilyl)phenyl-1-propyne (SPP) was copolymerized with diphenylacetylenes bearing tert-butyl (BDPA) and SiMe3 (SDPA) groups at various feed ratios to obtain poly(SPP-co-BDPA) and poly(SPP-co-SDPA) copolymers, respectively. Free-standing membranes were fabricated from toluene solutions of the copolymers, the gas permeability of which increased as the SPP ratio decreased (PO2: 550–2100 barrers). Interestingly, poly(SPP-co-BDPA) and poly(SPP-co-SDPA) at a 1
:
4 ratio of SPP:BDPA and SPP:SDPA, respectively, showed higher permeabilities than the respective homopolymers. Desilylation of the poly(SPP-co-BDPA) membrane increased the gas permeability, whereas desilylation of the poly(SPP-co-SDPA) membrane had the opposite result.
Introduction
The development of highly gas-permeable membranes is critical for the industrial application of gas-separation membranes.1 Polymeric membranes have been extensively developed owing to their accessibility and low cost.2–4 As an important category of polymeric membranes, disubstituted acetylene polymers have been studied extensively because of their extremely high gas permeabilities.5–9 As typical examples, poly[4-(tert-butyl)diphenylacetylene] [poly(BDPA)] and poly[4-(trimethylsilyl)diphenylacetylene] [poly(SDPA)] have high oxygen permeability coefficients (PO2) of 1100 and 1500 barrers, respectively (1 barrer = 10−10 cm3 (STP) cm cm−2 s−1 cmHg−1).10–13
Recently, we reported that the copolymerization of diphenylacetylenes improved their gas permeability.14–19 For example, the copolymer of 4-(tert-butyl)diphenylacetylene (BDPA) and 4-(trimethylsilyl)diphenylacetylene (SDPA) exhibited a higher PO2 of 2700 barrers than those of the homopolymers [poly(BDPA): 1100 barrers; poly(SDPA): 1500 barrers].14 The side groups in the copolymers are more diverse than those in the homopolymers, resulting in more irregular arrangement of the polymer chains and thus a higher gas permeability. In this regard, a copolymer composed of disubstituted acetylenes with different degrees of bulkiness is expected to show a high gas permeability. Herein, we describe the design of copolymers of 1-(p-trimethylsilyl)phenyl-1-propyne (SPP) with BDPA and SDPA (Scheme 1).
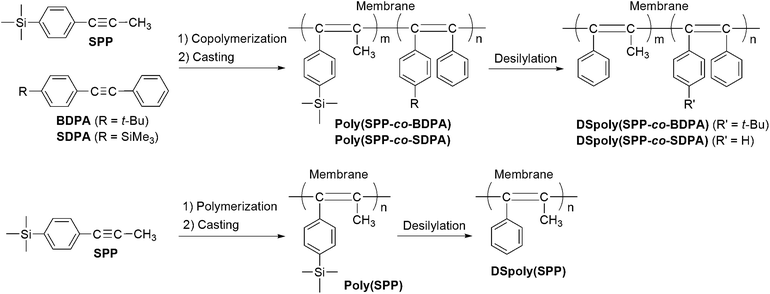 |
| Scheme 1 Preparation of disubstituted polyacetylenes. | |
The technique of desilylation is commonly applied to substituted polyacetylenes to allow for polymers with low polymerizability or poor solubility to be fabricated into membranes. This reaction can remove the silyl groups in the membrane state to generate molecular-scale voids, thereby changing the gas permeability.13,20 For example, while poly(diphenylacetylene) membranes [DSpoly(SDPA)] cannot be obtained by solution casting, they can be fabricated by the desilylation of poly(SDPA); however, desilylation decreases PO2 from 1500 to 900 barrers.13,20 On the contrary, it has been reported that the gas permeability of copolymer membranes containing tert-butyl groups can be improved by desilylation because the bulky side groups hinder chain packing.14,15,17
As described above, the copolymerization of SPP with diphenylacetylene and desilylation are promising for obtaining highly gas-permeable membranes. Herein, we report the copolymerization of SPP with BDPA and SDPA at varying feed ratios, the fabrication of poly(SPP-co-BDPA) and poly(SPP-co-SDPA) membranes, and their conversion to DSpoly(SPP-co-BDPA) and DSpoly(SPP-co-SDPA) membranes by desilylation. The gas permeability and general properties of these membranes are also described.
Experimental
Materials
Bis(triphenylphosphine)palladium(II) dichloride, triphenylphosphine, copper(I) iodide, 1-bromo-4-tert-butylbenzene, 1,4-dibromobenzene, sodium hydride, iodomethane, 2-methyl-3-butyn-2-ol, tetrahydrofuran (THF), triethylamine, N,N-dimethylformamide (DMF), chloroform (CHCl3), methanol (MeOH), trifluoroacetic acid (TFA), dimethyl sulfoxide (DMSO) (Fujifilm Wako Pure Chemical (Wako)), chlorotrimethylsilane (Tokyo Chemical Industry), n-butyllithium (Kanto Chemical), tantalum(V) chloride, and phenylacetylene (Sigma-Aldrich) were used without additional purification. n-Bu4Sn (cocatalyst for polymerization, Wako) and toluene (solvent for polymerization, Wako) were distilled over CaH2 (Wako) prior to use. 1-(p-Trimethylsilyl)phenyl-1-propyne (SPP),21 4-(tert-butyl)diphenylacetylene (BDPA),10 and 4-(trimethylsilyl)diphenylacetylene (SDPA)11 were prepared according to the literature.
Measurements
Gel permeation chromatography (Shimadzu LC-10AD chromatograph equipped with three polystyrene gel columns (Shodex A-80M × 2 and KF-802.5) and a Shimadzu RID-6A refractive index detector) was used to determine the molecular weight (eluent: THF, flow rate: 1.0 mL min−1, 40 °C, polystyrene calibration). An NMR spectrometer (JEOL ECX-500, Japan) was used to determine the composition ratio of the copolymers and characterize the monomers (solvent: CDCl3). An FT-IR spectrometer (Thermo Fisher Scientific Nicolet iS5) was used to determine the completion of the desilylation reaction. Field-emission scanning electron microscopy (Seiko Instruments Co. Ltd., Chiba, Japan; Zeiss Co. Ltd., Oberkochen, Germany) was used to record images of the membranes. Thermogravimetric analysis (Rigaku TG-DTA 8078G1 analyzer, Japan) was used to determine the thermal stability (heating rate: 10 °C min−1, nitrogen atmosphere). A gas permeation instrument (Tsukuba Rikaseiki K-315-N) was used to measure the gas permeability coefficients (P) of the membranes, which were calculated based on the slope of the steady-state region of the time–pressure curves. The gas diffusion coefficients (D) were calculated from the equation D = l2/(6θ), where l is the membrane thickness and θ is the time lag. The gas solubility coefficients (S) were calculated using the equation S = P/D.
Copolymerization
Two copolymers, poly(SPP-co-BDPA) and poly(SPP-co-SDPA), were synthesized by copolymerization of the corresponding monomers at a variety of feed ratios. Copolymerization was performed at the following reagent concentrations: [SPP + BDPA/SDPA]0 = 0.20 M, [TaCl5] = 20 mM, [n-Bu4Sn] = 40 mM. The detailed procedure for the copolymerization of SPP with BDPA at a 1
:
4 ratio was as follows. SPP (0.080 g), BDPA (0.38 g), and toluene (5.0 mL) were placed in a Schlenk tube equipped with a three-way stopcock under dry nitrogen. TaCl5 (71 mg), n-Bu4Sn (0.13 mL), and toluene (4.9 mL) were placed in another tube under a nitrogen atmosphere. The catalyst solution was heated for 10 min at 80 °C. The catalyst solution was then added to the monomer solution, and the mixed solution was heated to 80 °C for 24 h. After copolymerization, MeOH (∼1.0 mL) was added to the solution to deactivate the catalyst, after which the solution was diluted in toluene and poured in MeOH (∼1.0 L). The desired copolymer was obtained as a precipitate, and the yield was determined using the gravimetric method.
Membrane fabrication and desilylation
Poly(SPP-co-BDPA), poly(SPP-co-SDPA), and poly(SPP) membranes were prepared by casting the corresponding copolymer solutions (solvent: toluene, concentration: ∼1.0 wt%) on Petri dishes. After the evaporation of toluene, the membranes were peeled off, immersed in MeOH for 24 h, and dried to a constant weight under atmospheric pressure.
Desilylated copolymer membranes, DSpoly(SPP-co-BDPA) and DSpoly(SPP-co-SDPA), were prepared by desilylation of the poly(SPP-co-BDPA) and poly(SPP-co-SDPA) membranes, respectively. The membranes were immersed in a TFA/hexane solution (1
:
1 v/v) for 24 h. Then, the membranes were washed with MeOH, immersed in MeOH for 24 h, and finally dried to a constant weight under atmospheric pressure.
A desilylated homopolymer membrane, DSpoly(SPP), was prepared by the desilylation of poly(SPP) using the same procedure as for the desilylated copolymer membranes using TFA instead of a TFA/hexane mixture (Scheme 1).
Results and discussion
Copolymerization
Poly(SPP-co-BDPA) and poly(SPP-co-SDPA) were synthesized by copolymerization of the corresponding monomers at various feed ratios. The results are shown in Table 1. The composition ratios of the obtained copolymers were estimated from the 1H-NMR spectra (Fig. S1–S10†) as approximately equal to the feed ratios. Poly(SPP-co-BDPA)(1:4) with a SPP
:
BDPA feed ratio of 1
:
4 exhibited a high molecular weight (Mw = 1
670
000) and high yield (81%). With increasing SPP content, the molecular weight and yield of the obtained copolymers decreased (Mw = 253
000–774
000, yields = 53–71%). Similarly, poly(SPP-co-SDPA)(1:4) exhibited a high molecular weight (Mw = 1
890
000) and high yield (83%), whereas poly(SPP-co-SDPA) with higher SPP contents exhibited lower molecular weights and yields (Mw = 250
000–938
000, yields = 56–77%). It has been reported that the homopolymerization of SPP using TaCl5/n-Bu4Sn afforded a polymer with an Mw of 156
000,21 which is much lower than those of the homopolymers of BDPA (Mw = 3
600
000)10 and SDPA (Mw = 2
200
000)11 obtained under the same conditions. This suggests that the active species derived from SPP induce termination reactions more frequently than BDPA and SDPA. Therefore, copolymerization at a high feed ratio of SPP provides a low-molecular-weight polymer.
Table 1 Copolymerization of SPP with BDPA and SDPAa
Copolymer b |
Feed ratio |
Composition ratio c |
Yield (%) |
Mwd |
Mw/Mnd |
SPP : BDPA |
SPP : BDPA |
Copolymerization at 80 °C for 24 h; [SPP + BDPA/SDPA]0 = 0.20 M, [TaCl5] = 20 mM, [n-Bu4Sn] = 40 mM. MeOH-insoluble product. Estimated from 1H-NMR spectra (Fig. S1–S10). Determined by GPC eluted with THF. |
Poly(SPP-co-BDPA) |
1 : 4 |
1 : 3.93 |
81 |
1 670 000 |
6.78 |
1 : 2 |
1 : 2.07 |
71 |
387 000 |
5.77 |
1 : 1 |
1 : 1.08 |
67 |
774 000 |
5.62 |
2 : 1 |
2.08 : 1 |
53 |
371 000 |
4.49 |
4 : 1 |
4.09 : 1 |
55 |
253 000 |
2.46 |
Copolymer b |
Feed ratio |
Composition ratio c |
Yield (%) |
Mwd |
Mw/Mnd |
SPP : SDPA |
SPP : SDPA |
Poly(SPP-co-SDPA) |
1 : 4 |
1 : 4.15 |
83 |
1 890 000 |
7.71 |
1 : 2 |
1 : 1.91 |
77 |
938 000 |
7.15 |
1 : 1 |
1 : 0.95 |
63 |
813 000 |
6.39 |
2 : 1 |
2.00 : 1 |
64 |
250 000 |
3.38 |
4 : 1 |
3.98 : 1 |
56 |
573 000 |
5.29 |
Membrane fabrication and desilylation
Poly(SPP-co-BDPA) and poly(SPP-co-SDPA) membranes were fabricated using the casting method. Desilylation of the membranes was carried out using a TFA/hexane mixture to obtain DSpoly(SPP-co-BDPA) and DSpoly(SPP-co-SDPA) membranes, respectively. The FT-IR spectra of the membranes were measured to verify the desilylation conversion; the spectra of the polymer membranes prepared at a feed ratio of 4
:
1 are shown in Fig. 1. Absorption bands at 1120 and 1250 cm−1 were clearly observed in the FT-IR spectra of both poly(SPP-co-BDPA)(4:1) and poly(SPP-co-SDPA)(4:1), which originate from the stretching of Si–C bonds and vibration of SiC–H bonds, respectively. However, these two absorptions were not observed in the spectra of DSpoly(SPP-co-BDPA)(4:1) and DSpoly(SPP-co-SDPA)(4:1), indicating complete desilylation. The same results were observed in the FT-IR spectra of the other membranes (Fig. S11–S15†). Further, SEM images of membranes were shown in Fig. S16 and S17.† The morphologies of membranes did not change through desilylation, and the mapping data of silicon atom for membrane cross-section supported to completion of desilylation. On the other hand, the color of membranes changed to orange from yellow though desilylation (Fig. S18†).
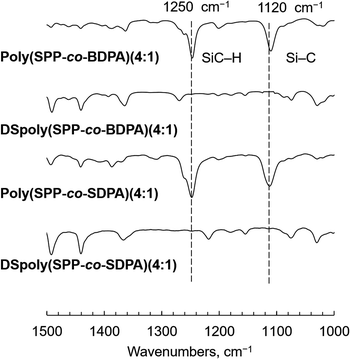 |
| Fig. 1 FT-IR spectra of poly(SPP-co-BDPA)(4:1), poly(SPP-co-SDPA)(4:1), DSpoly(SPP-co-BDPA)(4:1), and DSpoly(SPP-co-SDPA)(4:1). | |
The solvent solubilities of the copolymers are shown in Table 2. Poly(SPP-co-BDPA), poly(SPP-co-SDPA), and DSpoly(SPP-co-BDPA) exhibited the same solubility and were soluble in toluene, CHCl3, and THF but insoluble in MeOH, DMF, and DMSO. In contrast, DSpoly(SPP-co-SDPA) was insoluble in all the tested solvents. Poly(SPP-co-SDPA)s exhibited high thermal stability, and the onset temperatures were around 400 °C (Fig. S19†). The temperatures increased as the feed ratio of SDPA increased.
Table 2 Solvent solubility of copolymersa
Toluene |
CHCl3 |
THF |
MeOH |
DMF |
DMSO |
Symbols: +, soluble; −, insoluble. |
Poly(SPP-co-BDPA), Poly(SPP-co-SDPA), DSpoly(SPP-co-BDPA) |
+ |
+ |
+ |
− |
− |
− |
![[thin space (1/6-em)]](https://www.rsc.org/images/entities/char_2009.gif) |
DSpoly(SPP-co-SDPA) |
− |
− |
− |
− |
− |
− |
Gas permeability
Fig. 2 plots PO2 of the copolymers as a function of the ratio of SPP, and Table 3 summarizes the detailed gas permeability data. The PO2 values of the poly(SPP-co-BDPA) membranes were in the range of 550–1600 barrers, which increased with decreasing SPP ratio and were much higher than that of poly(SPP) (240 barrers). Poly(SPP-co-BDPA)(1:4) showed the highest PO2 (1600 barrers) among the poly(SPP-co-BDPA) samples, which was also higher than that of poly(BDPA) (1100 barrers). Similarly, the gas permeabilities of the poly(SPP-co-SDPA) samples were higher than that of poly(SPP) (PO2: 600–2100 vs. 240 barrers, respectively), and the gas permeability of poly(SPP-co-SDPA)(1:4) was higher than that of poly(SDPA) (PO2: 2100 vs. 1500 barrers, respectively). These results are consistent with our previous studies, which showed that copolymerization enhances the gas permeability of the materials.14–19 This can be explained by the fact that the copolymers have more abundant substituents than the homopolymers, resulting in more irregular chain packing. Comparing poly(SPP-co-BDPA) and poly(SPP-co-SDPA) at the same SPP ratio, poly(SPP-co-SDPA) showed a somewhat higher gas permeability, which is consistent with the higher gas permeability of poly(SDPA) than poly(BDPA).
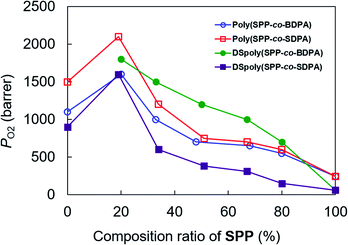 |
| Fig. 2 Relationship between PO2 and SPP ratio of copolymers. | |
Table 3 Gas permeability of copolymer and homopolymer membranesa
Membrane |
Feed ratio |
PO2 |
PCO2 |
PN2 |
PO2/PN2 |
Ref. |
Measured at 25 °C, P (barrer), 1 barrer = 10−10 cm3 (STP) cm cm−2 s−1 cmHg−1. |
SPP : BDPA |
Poly(SPP-co-BDPA) |
1 : 4 |
1600 |
6000 |
750 |
2.1 |
This work |
1 : 2 |
1000 |
4000 |
400 |
2.5 |
1 : 1 |
700 |
3200 |
250 |
2.8 |
2 : 1 |
650 |
3000 |
230 |
2.8 |
4 : 1 |
550 |
2400 |
180 |
3.1 |
DSpoly(SPP-co-BDPA) |
1 : 4 |
1800 |
6500 |
770 |
2.3 |
1 : 2 |
1500 |
5500 |
650 |
2.3 |
1 : 1 |
1200 |
4800 |
500 |
2.4 |
2 : 1 |
1000 |
4200 |
350 |
2.9 |
4 : 1 |
700 |
3500 |
200 |
3.5 |
![[thin space (1/6-em)]](https://www.rsc.org/images/entities/char_2009.gif) |
SPP : SDPA |
Poly(SPP-co-SDPA) |
1 : 4 |
2100 |
8400 |
950 |
2.2 |
This work |
1 : 2 |
1200 |
4300 |
480 |
2.5 |
1 : 1 |
750 |
3300 |
280 |
2.7 |
2 : 1 |
700 |
3000 |
220 |
3.2 |
4 : 1 |
600 |
2500 |
180 |
3.3 |
DSpoly(SPP-co-SDPA) |
1 : 4 |
1600 |
5500 |
700 |
2.3 |
1 : 2 |
600 |
2300 |
170 |
3.5 |
1 : 1 |
380 |
1600 |
100 |
3.8 |
2 : 1 |
310 |
1500 |
80 |
3.9 |
4 : 1 |
150 |
1000 |
38 |
3.9 |
Poly(SPP) |
|
240 |
1300 |
100 |
2.4 |
21 and 22 |
DSpoly(SPP) |
|
60 |
310 |
15 |
4.0 |
This work |
Poly(BDPA) |
|
1100 |
4800 |
500 |
2.2 |
10 |
Poly(SDPA) |
|
1500 |
4900 |
680 |
2.2 |
11 and 13 |
DSpoly(SDPA) |
|
900 |
|
410 |
2.2 |
11 and 13 |
Matrimid5218 |
|
2.12 |
|
0.32 |
6.6 |
23 |
Polysulfone |
|
1.4 |
|
0.24 |
5.8 |
23 |
6FDA-DAM |
|
55 |
|
17 |
3.3 |
24 |
The desilylated DSpoly(SPP-co-BDPA) membranes showed higher gas permeabilities (PO2: 700–1800 barrers) than the corresponding poly(SPP-co-BDPA) membranes. This is because the tert-butyl substituents hinder chain packing and maintain the microscale voids generated by the removal of silyl groups during desilylation. Similar to poly(SPP-co-BDPA), the gas permeabilities of DSpoly(SPP-co-BDPA) were much higher than those of DSpoly(SPP) [formed by the desilylation of poly(SPP)], and DSpoly(SPP-co-BDPA)(1:4) showed the highest gas permeability. Interestingly, although the desilylation of poly(SPP) decreased the gas permeability, the desilylation of poly(SPP-co-BDPA) increased the gas permeability, even at high SPP ratios.
In contrast, the desilylation of poly(SPP-co-SDPA) decreased the gas permeability, similar to the trend observed with poly(SDPA) and poly(SPP). Therefore, bulky substituents such as tert-butyl groups play an important role in enhancing the gas permeability of polymer membranes by desilylation. Nevertheless, the gas permeability of DSpoly(SPP-co-SDPA) was still high; especially, PO2 of DSpoly(SPP-co-SDPA)(1:4) was 1600 barrers, which is higher than that of DSpoly(SDPA) (900 barrers).
The gas permeability of copolymer membranes in this study were much higher than that of conventional glassy polymer membranes. For example, the commercially available Matrimid 5218 and polysulfone exhibit the PO2 less than 10 barrers,23 and relatively high gas permeable polyimide, 6FDA-DAM, shows 55 barrers.24 The PO2 of poly(SPP-co-SDPA)(1:4) was 38 times higher than that of 6FDA-DAM.
Gas diffusivity and solubility
The gas diffusion and solubility coefficients of the membranes are listed in Table 4. The diffusivity and solubility of all the membranes decreased with increasing SPP ratio. The D and S values of DSpoly(SPP-co-BDPA) were higher than those of poly(SPP-co-BDPA), which is consistent with the gas permeability trend. This increase in D and S may be attributable to desilylation increasing the free volume. In contrast, D and S of DSpoly(SPP-co-SDPA) were lower than those of poly(SPP-co-SDPA). As discussed above, the increase in gas permeability for poly(SPP-co-BDPA) and decrease in gas permeability for poly(SPP-co-SDPA) originated from variations in both the diffusivity and solubility.
Table 4 Gas diffusivity and solubility of copolymer membranes
Membrane |
Feed ratio |
Da |
Sb |
O2 |
CO2 |
N2 |
O2 |
CO2 |
N2 |
Diffusion coefficient (10−7 cm2 s−1). Solubility coefficient [10−3 cm3 (STP) cm−3 cmHg−1]. |
SPP : BDPA |
Poly(SPP-co-BDPA) |
1 : 4 |
41 |
35 |
38 |
39 |
170 |
20 |
1 : 2 |
38 |
33 |
37 |
26 |
120 |
11 |
1 : 1 |
26 |
28 |
24 |
26 |
114 |
10 |
2 : 1 |
25 |
27 |
23 |
26 |
110 |
10 |
4 : 1 |
23 |
24 |
19 |
24 |
100 |
9.5 |
DSpoly(SPP-co-BDPA) |
1 : 4 |
45 |
37 |
39 |
40 |
176 |
20 |
1 : 2 |
42 |
35 |
38 |
36 |
157 |
17 |
1 : 1 |
40 |
33 |
33 |
30 |
145 |
15 |
2 : 1 |
37 |
30 |
25 |
27 |
140 |
14 |
4 : 1 |
27 |
27 |
20 |
26 |
130 |
10 |
![[thin space (1/6-em)]](https://www.rsc.org/images/entities/char_2009.gif) |
SPP : SDPA |
Poly(SPP-co-SDPA) |
1 : 4 |
100 |
80 |
70 |
21 |
105 |
14 |
1 : 2 |
70 |
60 |
50 |
17 |
72 |
9.6 |
1 : 1 |
50 |
50 |
32 |
15 |
66 |
8.8 |
2 : 1 |
48 |
47 |
30 |
14 |
64 |
7.3 |
4 : 1 |
44 |
40 |
28 |
13 |
62 |
6.4 |
DSpoly(SPP-co-SDPA) |
1 : 4 |
80 |
60 |
65 |
20 |
83 |
10 |
1 : 2 |
40 |
33 |
30 |
15 |
70 |
5.7 |
1 : 1 |
35 |
30 |
20 |
11 |
54 |
5.0 |
2 : 1 |
30 |
28 |
17 |
10 |
53 |
4.7 |
4 : 1 |
20 |
23 |
10 |
7.5 |
43 |
3.8 |
Conclusions
Disubstituted polyacetylene membranes with high gas permeabilities were developed in this work by copolymerizing 1-(p-trimethylsilyl)phenyl-1-propyne (SPP) with diphenylacetylenes bearing tert-butyl (BDPA) and SiMe3 (SDPA) groups at various feed ratios. Poly(SPP-co-BDPA) and poly(SPP-co-SDPA) with a 20% SPP content exhibited higher gas permeabilities than the homopolymers, poly(BDPA) and poly(SDPA), respectively, despite the much lower permeability of poly(SPP). Desilylation of poly(SPP-co-BDPA) increased the gas permeability, whereas desilylation of poly(SPP-co-SDPA) reduced the gas permeability. These findings reveal that various disubstituted acetylene monomers can be applied to copolymerization to improve the gas permeability of the resulting membranes even if the corresponding homopolymers exhibit low gas permeabilities. Therefore, copolymerization is a promising method for the development of highly gas-permeable membranes.
Conflicts of interest
There are no conflicts to declare.
Acknowledgements
This work was supported by JSPS KAKENHI Grant Number JP20K05600.
Notes and references
- P. M. Budd and N. B. McKeown, Highly permeable polymers for gas separation membranes, Polym. Chem., 2010, 1, 63–68 RSC.
- D. F. Sanders, Z. P. Smith, R. Guo, L. M. Robeson, J. E. McGrath, D. R. Paul and B. D. Freeman, Energy-efficient polymeric gas separation membranes for a sustainable future: A review, Polymer, 2013, 54, 4729–4761 CrossRef CAS.
- W. J. Koros and G. K. Fleming, Membrane-based gas separation, J. Membr. Sci., 1993, 83, 1–80 CrossRef CAS.
- P. Pandey and R. S. Chauhan, Membranes for gas separation, Prog. Polym. Sci., 2001, 26, 853–893 CrossRef CAS.
- K. Nagai, T. Masuda, T. Nakagawa, B. D. Freeman and I. Pinnau, Poly[1-(trimethylsilyl)-1-propyne] and related polymers: Synthesis, properties and functions, Prog. Polym. Sci., 2001, 26, 721–798 CrossRef CAS.
- J. W. Y. Lam and B. Z. Tang, Functional polyacetylenes, Acc. Chem. Res., 2005, 38, 745–754 CrossRef CAS PubMed.
- T. Masuda, Substituted polyacetylenes, J. Polym. Sci., Part A: Polym. Chem., 2007, 45, 165–180 CrossRef CAS.
- M. Shiotsuki, F. Sanda and T. Masuda, Polymerization of substituted acetylenes and features of the formed polymers, Polym. Chem., 2011, 2, 1044–1058 RSC.
- T. Sakaguchi, Y. Hu and T. Masuda, Substituted polyacetylenes, in Membranes Materials for Gas and Vapor Separation: Synthesis and Application of Silicon-Containing Polymers, ed. Y. Yampolskii and E. Finkelshtein, Wiley, Chichester, 2017, pp. 107–136 Search PubMed.
- H. Kouzai, T. Masuda and T. Higashimura, Synthesis and properties of poly (diphenylacetylenes) having aliphatic para-substituents, J. Polym. Sci., Part A: Polym. Chem., 1994, 32, 2523–2530 CrossRef CAS.
- K. Tsuchihara, T. Masuda and T. Higashimura, Polymerization of silicon-containing diphenylacetylenes and high gas permeability of the product polymers, Macromolecules, 1992, 25, 5816–5820 CrossRef CAS.
- L. G. Toy, K. Nagai, B. D. Freeman, I. Pinnau, Z. He, T. Masuda, M. Teraguchi and Y. P. Yampolskii, Pure-gas and vapor permeation and sorption properties of poly[1-phenyl-2-[p-(trimethylsilyl)phenyl]acetylene] (PTMSDPA), Macromolecules, 2000, 33, 2516–2524 CrossRef CAS.
- T. Sakaguchi, K. Yumoto, M. Shiotsuki, F. Sanda, M. Yoshikawa and T. Masuda, Synthesis of poly(diphenylacetylene) membranes by desilylation of various precursor polymers and their properties, Macromolecules, 2005, 38, 2704–2709 CrossRef CAS.
- T. Sakaguchi, Y. Lin and T. Hashimoto, Enhancement of oxygen permeability by copolymerization of silyl group-containing diphenylacetylenes with tert-butyl group-containing diphenylacetylene and desilylation of copolymer membranes, RSC Adv., 2017, 7, 30949–30955 RSC.
- Y. Lin, T. Sakaguchi and T. Hashimoto, Desilylation of copolymer membranes composed of poly[1-(p-trimethylsilyl)phenyl-2-(p-trimethylsilyl)phenylacetylene] for improved gas permeability, RSC Adv., 2020, 10, 14637–14643 RSC.
- Y. Lin, T. Sakaguchi and T. Hashimoto, Excellent permselective membranes of diphenylacetylene copolymers with hydroxy groups, Polymer, 2020, 207, 122926 CrossRef CAS.
- Y. Lin, T. Sakaguchi and T. Hashimoto, Extremely high gas permeability of naphthyl group-containing diarylacetylene copolymers, Polymer, 2021, 212, 123305 CrossRef CAS.
- Y. Lin, T. Sakaguchi and T. Hashimoto, Ultrahigh oxygen permeability of the desilylated membranes of halogen-containing diphenylacetylene copolymers, J. Membr. Sci., 2021, 628, 119272 CrossRef CAS.
- Y. Lin, T. Sakaguchi and T. Hashimoto, Imidazolium-based diphenylacetylene copolymers with excellent carbon dioxide/nitrogen and oxygen/nitrogen gas separation performance, J. Membr. Sci., 2021, 637, 119638 CrossRef CAS.
- M. Teraguchi and T. Masuda, Poly(diphenylacetylene) membranes with high gas permeability and remarkable chiral memory, Macromolecules, 2002, 35, 1149–1151 CrossRef CAS.
- A. C. Savoca, A. D. Surnamer and C. F. Tien, Gas transport in poly(silylpropynes): The chemical structure point of view, Macromolecules, 1993, 26, 6211–6216 CrossRef CAS.
- L. M. Robeson, W. F. Burgoyne, M. Langsam, A. C. Savoca and C. F. Tien, High performance polymers for membrane separation, Polymer, 1994, 35, 4970–4978 CrossRef CAS.
- B. W. Rowe, B. D. Freeman and D. R. Paul, Physical aging of ultrathin glassy polymer films tracked by gas permeability, Polymer, 2009, 50, 5565–5575 CrossRef CAS.
- J. H. Kim, W. J. Koros and D. R. Paul, Physical aging of thin 6FDA-based polyimide membranes containing carboxyl acid groups. Part I. Transport properties, Polymer, 2006, 47, 3094–3103 CrossRef CAS.
Footnote |
† Electronic supplementary information (ESI) available: 1H-NMR spectra of poly(SPP-co-BDPA) and poly(SPP-co-SDPA); FT-IR spectra, SEM images, photographs and TGA curves of the membranes. See DOI: 10.1039/d1ra06363d |
|
This journal is © The Royal Society of Chemistry 2021 |
Click here to see how this site uses Cookies. View our privacy policy here.