DOI:
10.1039/D1RA05990D
(Paper)
RSC Adv., 2021,
11, 34160-34169
Impact of germination on the chemical profile of HomChaiya rice wort and beer
Received
7th August 2021
, Accepted 12th October 2021
First published on 20th October 2021
Abstract
HomChaiya rice is one of the important rice varieties in Thailand. However, the commercial value of this rice is very limited as it is not widely known and has limited availability. The present research aimed to produce an alcoholic beverage from malted HomChaiya rice, and various chemical profiles (physicochemical, phytochemicals, volatiles, and amino acids) were studied. HomChaiya rice was germinated for different periods (3, 5, and 7 days), kilned at 60 °C, and used to produce wort and beer. Physicochemical qualities such as pH, brix, titratable acidity, viscosity, soluble nitrogen, FAN, and extractability were higher in the wort, and the results were significantly influenced by the germination time. On the other hand, the color values were higher in the beer samples, and prolonged germination brightened the beer color. The total phenolic content, flavonoid content, and various antioxidant potentials (DPPH, ABTS, and reducing power) were significantly increased in the beer samples when made with rice malt germinated for 7 days compared to the wort under similar conditions. Wort samples had 13 identified volatiles, whereas the beer samples had 30 volatile compounds. Furthermore, 20 amino acids were identified, and most of them were found at a higher level in the wort samples compared to beer samples, and prolonged germination slightly increased the amino acid levels. The beer samples' sensory characteristics showed that the HomChaiya rice germination period had no significant effect.
1. Introduction
HomChaiya rice is an indigenous rice variety that is only cultivated in the Chaiya district of Surat Thani province in southern Thailand. HomChaiya rice grains are slightly fatter than those of jasmine rice, a very famous Thai rice worldwide.1 HomChaiya rice grains are pale brown, and upon optimum cooking conditions provide good texture and palatability and are mostly sold unpolished, and due to that, they contain lots of phytochemicals, so apart from being a staple food, it has numerous vitamins (A, E, B1, and B2), minerals (iron, zinc, and calcium), and gamma oryzanol and γ-aminobutyric acid (GABA).2 Besides, it also has antioxidant effects against reactive oxygen species. However, HomChaiya rice and its products are very limited, and consumers do not widely know it as it is mostly sold in the local market. Cereals have the propensity to germinate, and the germination process could modify the nutritional content in the rice to a more functional and nutraceutical direction.3,4
Germination and malting help hydrolyze complex substances into simpler forms, facilitating the metabolic availability of the rice.5 Isomalto-oligosaccharides and various di- and monosaccharides are produced during germination, and these can be potentially prebiotic components.6 Lekjing and Venkatachalam (2020)1 found that the HomChaiya rice that had undergone prolonged germination had increased levels of various hydrolase enzymes and a higher kilning temperature (50–60 °C) induced the production of monosaccharides as well as amino acids. Thus, germination and malting are the two major methods for processing cereals, particularly rice, to produce various commercial products.
Production of a beverage from rice is always secondary to making a snack, particularly an extruded product, or consumption as a staple food. Therefore, HomChaiya rice has not been used to produce any beverages. Beer is a versatile alcoholic beverage, which can be produced using various malted grains. It is the most popular alcoholic beverage worldwide, and Thailand is one of the topmost countries for beer consumption. Traditionally, beers are produced using malted wheat and barley, and the raw materials vary by country in which the beer is made. Generally, rice is rarely used in making beer and then only as a secondary component (up to 40%) instead of being the sole raw material, and the low level of rice usage is due to the formation of haze and off-odors in the beer.7,8 These drawbacks in beer mainly occur due to improper germination and malting of the rice.
Furthermore, insufficient worting also leads to poor quality of rice beer. Wort is a complex liquid medium that controls or enhances the growth of yeast. Wort comprises two major components: carbohydrates (90%) and nitrogenous compounds (5–10%). Various vitamins, minerals, lipids, organic acids, bioactive compounds, and nucleic acids are also observed in the wort.9 Rice is an excellent alternative for gluten-free beer, and it can be crafted to imitate the taste and flavor of traditional beer.10 At present, no alcoholic beverage has been made to explore the versatile nature of HomChaiya rice. The present research aimed to explore the impact of germination times on various chemical profiles including, physicochemical, phytochemical, volatile compounds, and amino acids in HomChaiya wort and beer.
2. Material and methods
2.1 Raw material, malting, kilning
The HomChaiya rice (Oryza sativa L. HomChaiya) was obtained from a local producer in the Chaiya district, Surat Thani province. The rice was thoroughly washed to remove any extraneous materials and was then subjected to malting.1 First, rice was soaked and covered with a muslin cloth and kept for 48 hours under ambient temperature and at every 8 hours, the old water was replaced with a newer one. After soaking, the water was drained, and the rice was washed thoroughly three times with plain water, and then it was allowed to germinate for various times (3, 5, or 7 days). After germination, the rice was collected and kilned at 55 °C until the final moisture was 8.4%. Then, the malted rice samples were collected and ground into coarse particles. The coarsely ground (∼0.5–0.8 μm) malted rice samples were packed in a vacuum package and stored in a dry place until the beer production (within two months).
2.2 Beer production
The mashing and fermentation were done on a pilot-scale following Mayer et al. (2016)11 with slight modifications. First, malted rice samples were mixed with brewing water in a ratio of 1
:
4, the pH of the mashing liquid was set to 5.6 using lactic acid. There were five combinations of mashing temperature and time ((i) at 55 °C for 30 min, (ii) at 65 °C for 60 min, (iii) at 74 °C for 10 min, and (iv) 78 °C for 10 min) applied in the mashing of malted HomChaiya rice. An iodine test was performed to observe the saccharification every 10 min when mashing reached 65 °C, and complete saccharification was achieved when the mashing temperature was maintained at 78 °C for 10 min. After the complete mashing, the wort liquid was lautering for 1 hour to filter the wort. Sparging was done at 78 °C twice, first with 4 L followed by 2 L. Then the sweet wort was boiled for an hour; at the beginning stage of the boil, hops pellets (Humulus lupulus, α acid 3–6%, from Hallertau Hersbrucker) were added to the wort liquid, and then hops were added again 10 min before the one-hour boil was complete. International bitterness unit (IBU) level of the wort was set to 15. After boiling, the wort was filtered to remove the hot tub, obtain a clear liquid, and keep it for cooling to 22 °C. Then the wort was aerated before pitching the yeast. Before the pitching of yeast, the wort was sampled for various quality tests. After that, the yeast (Saccharomyces cerevisiae from Fermentis, Safbrew T-58) was added to the wort (1 g L−1) after being rehydrated with sweet wort to achieve 107 cells per mL. The fermentation temperature was maintained at 20 °C for the optimum fermenting, and the fermentation lasted for 12 days. After fermentation, the beer was carefully collected and filtered using four layers of muslin cloth and stored in aseptic bottles at −18 °C for quality analysis.
2.3 Quality analysis
2.3.1 Determination of wort and beer quality. The quality analysis included determining extract of wort (°P) (European Brewery Convention (EBC)12 method no. 8.3); pH in wort and beer was measured using a pH meter (EBC method no. 8.17, 9.35); the color of wort and beer by using the EBC methods no. 8.5, 9.6; density by using a hydrometer; titratable acidity (%, lactic acid) by titration method of Association of Official Analytical Chemists (AOAC, 2000)13 (AOAC method no. 942.15); total soluble solids using a handheld refractometer (°Brix); viscosity using a viscometer (mPa s at 20 °C) (EBC method no. 8.4, 9.4); fermentability (%) or wort was measured using a rapid method (EBC method no. 8.6.2); total nitrogen (mg L−1) in wort and beer was measured by using Kjeldahl method (EBC method no. 8.9.1, 9.9.1); and free amino acids (mg L−1) using a spectrophotometer (EBC method no. 8.10, 9.10). Wort yield (%) was measured using the method of Mayer et al. (2016).11 Alcohol content (%) in the beer was measured using gas chromatography (EBC method 9.2.1). The bitterness (mg L−1) of the beer was measured by quantifying the iso-α-acids using a spectrophotometer (EBC method 9.8). The phytochemicals such as total phenolic content (TPC) and total flavonoid content (TFC) in wort and beer were measured using the methods of Singleton et al. (1999)14 and Zhishen et al. (1999),15 respectively. Antioxidant activities such as DPPH radical scavenging activity (%), ABTS radical cation scavenging activity (%), and ferric reducing/antioxidant power (mmol Fe2± per 100 mL) were measured in the wort and beer samples by following the methods of Brand-Williams et al. (1995),16 Re et al. (1998)17 and Benzie and Strain (1999),18 respectively.
2.3.2 Volatile compounds. The volatile compounds in the wort and beer samples were identified based on Rossi et al. (2013)19 with slight modifications. First, 5 mL of sample was placed in a 15 mL vial covered with a Teflon-lined cap, and then the vial was heated for derivatization at 50 °C for 26 min. Next, an SPME fiber (50/30 μm polydimethylsiloxane/divinylbenzene (PDMS/DVB) on 2 cm stable flex fiber) was inserted into the sample containing vial and allowed to absorb for 2 min at 50 °C. Helium was used as a carrier gas, and the flow rate was set to 1.1 mL min−1. The front inlet temperature was set to 200 °C, and the purge valve was set to 20 mL min−1. Initially, the oven temperature was at 60° and held for 5 min; after that, the temperature was raised at 3 °C min−1 to 210 °C and held for 5 min. The flame ionization detector (FID) was set to 280 °C, and the ionization energy was 70 eV. The detection and data collection were performed from 30 to 660 m/z in the scan mode. The data were analyzed using the MSD ChemStation Data Analysis Software, and the results are reported as percentages of relative concentrations.
2.3.3 Amino acid composition. Amino acid compositions in the wort and beer samples were measured according to Bonke et al. (2020).20 First, the samples were freeze-dried at 0.1 mbar for 3 hours at −30 °C with a freeze dryer. Then, the dried samples were hydrolyzed under vacuum conditions (65 millitorrs) for 2 min by using 200 μL of HCL (6 M)/1% phenol three times. After that, the samples were kept at 105 °C for 24 hours, and then, 50 μL of the sample was reconstituted with 75 μL of absolute ethanol and 75 μL of sodium borate (100 mM) to a 400 μL solution. A 50 μL aliquot of the reconstituted sample was added to a 25 μL internal standard (4.6 μmoL ml−1 of norvaline). After hydrolysis, the sample was derivatized using a 75 μL 1-fluoro-2,4-dinitrobenzene (70 mM), and after that, it was heated and dried. Finally, 20% methanol was used to reconstitute the samples. The samples were then subjected to High-Performance Capillary Electrophoresis (HPME) to identify the amino acid compositions. The method derivatized the sample at 50 °C using DNFB, the separation voltage was set to 18 kV, the injection time was set to 2 s, and the pressure was set to 50 mbar. The running time of the sample was 75 min, and the UV detection was at 360 nm. The results are reported as mg per 100 mL.GABA content in the wort and beer samples were measured in accordance with the method of Baranzelli et al. (2018).21 1 mL of sample mixed with 5 mL of methanol (90%, v/v) and then, the mixture was vortex for 10 min and then sonicated for 15 min at ambient temperature and centrifuged for 10 min at 4000 × g. After centrifugation, the supernatant was collected and filtered through 0.22 μm nylon syringe filter and then 10 μL of aliquot was tested for GABA in the LC/MS chromatograph/mass spectrometer. The results are reported as mg per 100 mL.
2.4 Sensory characteristics
The beer's sensory analysis was carried out using 50 untrained panelists aged between 25 and 50 years. The panelists were mostly from the university campus, and they were mostly routine beer drinkers. The guidelines for the sensory analysis were obtained from EBC method no. 13.10. The scores ranged from 0 to 9, in which 0 represents the absence of the sensory characteristic and 9 represents an extremely strong sensory characteristic. There were 12 sensory characteristics, namely fruity, color, acceptance, linger, body, astringent, sour, bitter, sweet, oxidized, malty, and alcoholic, tested in the beer. All the beer samples were labeled randomly before testing of sensory characteristics. The results are presented in a spider plot.
2.5 Statistical analysis
All experiments were conducted in triplicates, and the data are presented as mean ± standard deviation. Significant differences between the treatments were analyzed using analysis of variance (ANOVA) by SPSS software for Windows. In addition, Duncan's post hoc test was used at a threshold level of 5% to confirm the significant differences between means.
3. Results and discussion
3.1 Wort quality attributes
Various quality characteristics of wort made of HomChaiya rice malt produced with alternative germination times are presented in Table 1. The results show that prolonged germination increased the extractability of wort from the HomChaiya rice malt. Rice that was germinated for 7 days had better wort extractability than the other cases. An increase of wort extractability indicates the higher activity of the hydrolase enzymes and the amounts of soluble solids present in the wort.22 Prolonged germination could increase hydrolase activity and thus help the wort extractability. Lekjing and Venkatachalam (2020)1 found that increased wort extractability was mainly attributed to α- and β-amylase activities. Wort pH gradually decreased when utilizing malted HomChaiya rice with prolonged germination time (7 days). Though the changes are not large, they still fell in the optimum pH range of several hydrolase enzymes. Meyer et al. (2016)11 found increased protease, dextrinase, and amylase activities in wort samples, which had a pH range from 5.4 to 5.8.
Table 1 Quality attributes of wort and beer produced using HomChaiya rice malt with different germination times
Physiochemical characteristic |
Germination time (days) |
3 |
5 |
7 |
The results are presented as mean ± SD (n = 3). Different superscripts in a row indicate significant differences. |
Wort |
Extract of wort (%) |
42.75 ± 0.68a |
47.77 ± 0.99b |
50.44 ± 1.00c |
pH |
5.88 ± 0.51b |
5.67 ± 0.68b |
5.32 ± 0.56a |
EBC color |
7.58 ± 0.48b |
8.54 ± 0.82a |
9.46 ± 1.26c |
Density |
5.14 ± 0.03b |
6.18 ± 0.02ab |
6.82 ± 0.01a |
Titratable acidity (% lactic acid) |
4.41 ± 0.04a |
4.47 ± 0.04a |
4.53 ± 0.03a |
Total soluble solids (°Brix) |
6.89 ± 0.28b |
7.64 ± 0.03a |
8.82 ± 0.25a |
Viscosity (mPa s) |
2.53 ± 0.05b |
2.22 ± 0.09a |
2.21 ± 0.04a |
Total nitrogen (mg L−1) |
650.88 ± 1.08a |
646.67 ± 1.53b |
642.67 ± 2.08b |
Free amino acids (mg L−1) |
146.33 ± 2.08c |
148.67 ± 2.08b |
154.33 ± 3.06a |
Malt yield (%) |
61.33 ± 0.58c |
63.67 ± 0.58b |
65.67 ± 1.15a |
![[thin space (1/6-em)]](https://www.rsc.org/images/entities/char_2009.gif) |
Beer |
Original extract (%P) |
16.51 ± 0.02a |
17.57 ± 0.50b |
17.96 ± 0.12b |
Apparent attenuation (%) |
66.43 ± 0.50a |
71.77 ± 0.30b |
75.22 ± 0.73c |
Alcohol (%) |
4.12 ± 0.11a |
4.53 ± 0.11b |
4.97 ± 0.07c |
pH |
4.63 ± 0.50b |
4.58 ± 0.03a |
4.61 ± 0.01b |
EBC color |
8.89 ± 0.10a |
9.41 ± 0.51b |
9.62 ± 0.43c |
Total nitrogen (mg L−1) |
460.33 ± 2.08a |
466.33 ± 0.58b |
470.33 ± 1.53c |
Free amino acids (mg L−1) |
73.21 ± 1.04a |
74.20 ± 0.82b |
76.30 ± 0.98c |
Viscosity (mPa s) |
1.65 ± 0.10b |
1.26 ± 0.05a |
1.21 ± 0.04a |
Bitterness (mg L−1) |
16.03 ± 0.06a |
16.60 ± 0.10ab |
16.77 ± 0.06ab |
Titratable acidity (% lactic acid) |
2.03 ± 0.06a |
2.27 ± 0.12b |
2.60 ± 0.00c |
Total soluble solids (°Brix) |
2.13 ± 0.05a |
2.55 ± 0.05b |
2.57 ± 0.07b |
Density |
1.01 ± 0.01a |
1.08 ± 0.03a |
1.18 ± 0.01b |
The color of the malted HomChaiya rice wort was brownish-yellow. Malted rice color was significantly affected when the germination time was prolonged. The darkness of the wort liquid gradually increased with germination time. The quality of wort liquid determines the color of the wort as well as kilning of the malt. Wort color is mainly contributed by the mashing process, in which the hydrolase enzyme breaks complex molecules such as proteins and starch into simpler forms, and these are further converted into various color compounds by Maillard reactions.23 On the other hand, the titratable acidity of the samples gradually turned acidic (P < 0.05). Total soluble solids tended to increase in the wort samples with extended germination, possibly because of amylase enzymes. The density of wort also tended to increase with germination time. Conversely, the viscosity decreased with increased germination time, although the differences in wort viscosity were minimal. The germination period significantly affected the total nitrogen and free amino acids in the wort due to increased protease and hydrolase activities during germination and mashing. Furthermore, the malt yield of HomChaiya rice was higher in the samples that underwent prolonged germination.
In addition, the quality characteristics of beer produced using malted HomChaiya rice that had undergone prolonged germination are also presented in Table 1. Overall, the results of beer quality attributes exhibited significant differences by germination time. Among the different samples, the original extract of the beer was higher when using malted rice that was germinated for 7 days. Similarly, the apparent attenuation of the beer samples was higher with the germination time. The alcohol content of the rice beer was not much influenced by the germination time; however, a slight increase in alcohol level was observed in the malted rice beer when the rice was germinated for a longer time. pH and titratable acidity of malted rice beer samples were stable and did not significantly differ by germination time. The color of malted HomChaiya rice beer samples was slightly affected by the germination time. Prolonged rice germination increased the beer's bright yellowish-brown color. The beer samples' density and viscosity were smaller than those of the wort samples, but these gradually increased in the beer with germination time. Total soluble solids were very low in the beer samples compared to the wort, possibly due to the fermentation process in which the yeast consumed all of the free sugar and converted it into alcohol. Titratable acidity slightly increased with germination time in the beer samples produced using malted rice. Hops contributed to the bitterness in the wort during beer production, but bitterness did not significantly differ among the beer samples. Total nitrogen and free amino acids in the beer samples were significantly affected by germination time in the malted rice.
3.2 Phytochemicals and antioxidants
Phytochemicals and antioxidant activities of wort and beer from HomChaiya rice malt produced with alternative germination times are presented in Table 2. TPC in the wort was not significantly influenced by the germination time, even though it slightly increased. On the other hand, a significantly higher level of TPC was noticed in the beer, and similar to the wort, the TPC did not differ much by germination time. The TFC levels in wort and beer samples did not differ much. A slight increase in TFC was observed in beer samples. HomChaiya rice germinated for 7 days had slightly increased phytochemical levels. Phattayakorn et al. (2016)24 found that not germinated rice held more TPC and TFC than germinated rice. Polyphenols are the key source of antioxidant activities in wort and beer.25 The main source of polyphenols in wort and beer is the malted grains (70–80%) and hops (30–20%).26 A change in the polyphenol content could be caused by chemical changes during wort production.27 Polyphenols can readily interact with the beer constituents, particularly with ions and proteins, contributing to the overall flavor, color, foam, and stability.28 Koren et al. (2019)29 reported that the lower polyphenols in the wort could be due to soluble phenols that evaporated in the hot tub. Though the phytochemical contents in the wort and beer were not high, the antioxidant activities were significantly high in all samples. Sun et al. (2016)30 reported that the formation of 5-hydroxymethylfurfural (HMF) and melanoidins through the Maillard reaction could also contribute to the antioxidant activities. Martinez-Gomez et al. (2020)31 reported that the beer-making process is critical, including germination, kilning, mashing, and wort boiling. As a result, the phenolic compounds and melanoidin levels are increased. The DPPH radical scavenging potency was slightly higher in the beer as compared to wort samples. Besides, the ABTS radical scavenging activity was higher compared with DPPH scavenging activity, and the beer samples held more scavenging potency than the wort samples. The beer samples had significantly high reducing power that increased germination time, while the wort was on a lesser stable level with no differences by germination time. The different germination times significantly influenced the beer antioxidant activities, whereas the antioxidant activity was lesser, with only small differences among the wort samples.
Table 2 Phytochemicals and antioxidant activities of wort and beer produced using HomChaiya rice malt from different germination times
Quality characteristic |
Germination time (days) |
3 |
5 |
7 |
The results are presented as mean ± SD (n = 3). Different superscripts in a row indicate significant differences. |
Phytochemicals and antioxidant activities |
Wort |
TPC (μg mL−1 gallic acid equivalents) |
6.40 ± 0.03a |
6.42 ± 0.02a |
6.45 ± 0.01a |
TFC (μg mL−1 quercetin equivalents) |
3.47 ± 0.02a |
3.79 ± 0.03a |
4.01 ± 0.02b |
DPPH radical scavenging activity (%) |
85.40 ± 0.25a |
87.03 ± 0.23b |
87.78 ± 0.30b |
ABTS radical scavenging activity (%) |
88.73 ± 0.23a |
91.19 ± 0.17b |
92.20 ± 0.03b |
Reducing power (mmol Fe2± per 100 mL) |
14.67 ± 0.68a |
15.70 ± 1.22b |
15.71 ± 1.16b |
![[thin space (1/6-em)]](https://www.rsc.org/images/entities/char_2009.gif) |
Beer |
TPC (μg mL−1 gallic acid equivalents) |
9.23 ± 0.18a |
9.31 ± 1.11b |
9.39 ± 0.89b |
TFC (μg mL−1 gallic acid equivalents) |
3.76 ± 0.45a |
3.88 ± 0.9b |
4.11 ± 0.50c |
DPPH radical scavenging activity (%) |
88.32 ± 2.82a |
89.15 ± 1.45a |
90.56 ± 0.15b |
ABTS radical scavenging activity (%) |
92.15 ± 0.80a |
94.56 ± 0.50b |
95.16 ± 0.70c |
Reducing power (mmol Fe2± per 100 mL) |
23.13 ± 0.05a |
24.56 ± 0.23b |
26.51 ± 0.49c |
3.3 Wort and beer volatile compounds
The volatile compounds in wort and beer produced using malted HomChaiya rice from different germination times are presented in Tables 3 and 4. There were 13 volatile compounds identified in the wort samples (Table 3). All the volatile compounds in the wort had an increasing trend with the germination time of the HomChaiya rice. Among them, the 2,2′,4-tris(trifluoromethyl)biphenyl, 2-butanone, 3-hydroxy-, benzaldehyde, pentanoic acid, and 1-(5-(4-isopropylthiazol-2-yl)-2,2-dimethyl-1,3,4-oxadiazol-3(2H)-yl)ethenone were the dominant volatile compounds found in the wort. Conversely, butanal, 3-methyl- and octanoic acid were the least volatile compounds in the wort samples. Furthermore, butanoic acid and hexanoic acid in the wort were minimally affected by the germination time compared to the other compounds. Overall, the results showed that alcohol, ketone, aldehyde, and fatty acids were the major volatile compounds in the wort. It is well known that the level of volatile compounds in the wort mainly comes from the mashing process, especially through enzymatic hydrolysis and Maillard reactions. Furthermore, the addition of hops in the wort contributes to the flavor. Normally, the wort contains fewer volatile compounds than beer, as it loses a lot of the volatiles by evaporation during the boiling. Schutter et al. (2008)32 found the volatile differences between wort and vapor condensate of the wort, and the results showed that the wort vapor condensate contains an abundant level of flavors compared to the wort. Several studies have reported that rice wort has fewer volatile compounds than barley malt.33 This study found that the volatile fatty acids are abundant in the wort compared to other groups, and besides, the germination time affected the fatty acid content in the wort. An increase of volatile fatty acids in wort is due to higher lipase expression in the germinated rice. Schwarz et al. (2002)34 studied the lipase activity during the mashing of rice and found that increasing mashing time did not completely inactivate the lipase enzyme, which liberated free fatty acids and hydroperoxides. Therefore, free fatty acids could cause off-flavor to the wort as well as to beer.
Table 3 Volatile compounds in the wort produced using HomChaiya rice malt from different germination times
Volatile compounds in wort (%, relative concentration) |
Germination time (days) |
3 |
5 |
7 |
The results are presented as mean ± SD (n = 3). Different superscripts in a row indicate significant differences. |
2-Propanone |
0.92 ± 0.01a |
1.07 ± 0.03b |
1.22 ± 0.01c |
Butanal, 3-methyl- |
0.46 ± 0.05a |
0.56 ± 0.01b |
0.59 ± 0.01c |
2,2′,4-tris(Trifluoromethyl)biphenyl |
4.44 ± 0.50a |
4.47 ± 0.09a |
4.59 ± 0.17b |
2-Butanone, 3-hydroxy- |
34.58 ± 0.18a |
39.45 ± 0.80b |
40.19 ± 0.77c |
1-(5-(4-Isopropylthiazol-2-yl)-2,2-dimethyl-1,3,4-oxadiazol-3(2H)-yl)ethanone |
1.72 ± 0.08a |
1.79 ± 0.01ab |
1.84 ± 0.05b |
Benzaldehyde |
12.62 ± 0.14a |
13.56 ± 0.03b |
13.89 ± 0.07b |
Butanoic acid |
0.72 ± 0.03a |
0.77 ± 0.00ab |
0.81 ± 0.07b |
Pentanoic acid |
5.36 ± 0.50a |
5.37 ± 0.34a |
5.41 ± 0.61b |
Cyclononasiloxane, octadecamethyl- |
0.52 ± 0.01a |
0.55 ± 0.02ab |
0.57 ± 0.01b |
Hexanoic acid |
0.64 ± 0.07a |
0.61 ± 0.04a |
0.63 ± 0.01a |
Hexanoic acid, 2-ethyl- |
0.81 ± 0.06a |
0.82 ± 0.04a |
0.91 ± 0.03b |
Octanoic acid |
0.41 ± 0.01a |
0.49 ± 0.00b |
0.54 ± 0.01c |
Nonanoic acid |
0.61 ± 0.00a |
0.71 ± 0.00b |
0.75 ± 0.00c |
Table 4 Volatile compounds in beer produced using HomChaiya rice malt from different germination times,
Volatile compound in beer (%, relative concentration) |
Germination time (days) |
3 |
5 |
7 |
The results are presented as mean ± SD (n = 3). Different superscripts in a row indicate significant differences. (*) indicates no detection. |
Borane-methyl sulfide complex |
0.15 ± 0.00a |
0.17 ± 0.00b |
0.21 ± 0.00c |
Acetic acid ethyl ester |
3.06 ± 0.05a |
3.17 ± 0.01b |
3.22 ± 0.02c |
Butanoic acid, ethyl ester |
0.55 ± 0.05b |
0.45 ± 0.03ab |
0.41 ± 0.01a |
Isobutylalcohol |
2.44 ± 0.07a |
2.77 ± 0.05b |
3.18 ± 0.08c |
1-Butanol, 3-methyl-, acetate |
0.48 ± 0.02a |
0.52 ± 0.01b |
0.53 ± 0.03b |
1-Butanol |
0.29 ± 0.01b |
0.27 ± 0.04ab |
0.25 ± 0.01a |
1-Butanol, 3-methyl- |
34.47 ± 0.05a |
38.89 ± 1.13b |
39.05 ± 0.34c |
Benzene, ethenyl- |
1.08 ± 0.01a |
1.09 ± 0.05ab |
1.11 ± 0.04b |
Benzene, 1,2-bis(iodomethyl)- |
0.73 ± 0.02a |
0.77 ± 0.04b |
0.77 ± 0.00b |
2-Butanone, 3-hydroxy- |
0.13 ± 0.00a |
0.16 ± 0.01b |
0.21 ± 0.00c |
Cyclopropane, (1-methylethyl)- |
0.19 ± 0.00c |
0.16 ± 0.00b |
0.11 ± 0.00a |
Linalool |
0.24 ± 0.07c |
0.15 ± 0.00b |
0.09 ± 0.00a |
Formic acid, octyl ester |
3.8 ± 0.03a |
4.1 ± 0.12b |
4.4 ± 0.09b |
Propanedioic acid, dimethyl- |
0.16 ± 0.01a |
0.55 ± 0.03b |
0.88 ± 0.00c |
Butanoic acid, 3-methyl- |
0.47 ± 0.05c |
0.41 ± 0.01b |
0.39 ± 0.00a |
Cyclohexene, 4-[(1E)-1,5-dimethyl-1,4-hexadien-1-yl]-1-methyl- |
0.95 ± 0.00a |
1.05 ± 0.04b |
1.08 ± 0.04b |
Naphthalene, decahydro-4a-methyl-1-methylene-7-(1-methylethenyl)-, [4aR-(4a.alpha.,7.alpha.,8a.beta.)]- |
0.14 ± 0.00b |
0.09 ± 0.00a |
* |
.alpha.-Selinene |
0.21 ± 0.00a |
0.24 ± 0.04ab |
0.27 ± 0.02b |
Eudesma-3,7(11)-diene |
0.06 ± 0.00a |
* |
* |
Hexanoic acid |
0.16 ± 0.00a |
0.19 ± 0.00b |
0.24 ± 0.01c |
2,5-Di-tert-Butyl-1,4-benzoquinone |
2.81 ± 0.05a |
3.22 ± 0.07b |
3.41 ± 0.09c |
Phenol |
0.35 ± 0.01a |
0.39 ± 0.01b |
0.41 ± 0.02c |
.beta.-Selinene |
0.12 ± 0.01a |
0.15 ± 0.01b |
0.19 ± 0.00c |
Octanoic acid |
0.26 ± 0.02c |
0.21 ± 0.01b |
0.15 ± 0.00a |
(1R,3E,7E,11R)-1,5,5,8-Tetramethyl-12-oxabicyclo[9.1.0]dodeca-3,7-diene |
0.14 ± 0.04b |
0.08 ± 0.00a |
* |
1,1,4,7-Tetramethyldecahydro-1h-cyclopropa[e]azulen-4-ol |
0.09 ± 0.00a |
* |
* |
2-Methoxy-4-vinylphenol |
0.41 ± 0.02ns |
0.44 ± 0.00ns |
0.49 ± 0.04ns |
2-Naphthalenemethanol, 1,2,3,4,4a,5,6,8a-octahydro-.alpha.,.alpha.,4a,8-tetramethyl-, [2R-(2.alpha.,4a.alpha.,8a.beta.)]- |
0.15 ± 0.01b |
0.14 ± 0.00b |
0.09 ± 0.00a |
.beta.-Eudesmol |
0.15 ± 0.00a |
* |
* |
Imidazo(1,5-a)pyrimidine |
0.61 ± 0.02a |
0.77 ± 0.00b |
0.81 ± 0.04b |
On the other hand, the malted HomChaiya rice beer had an abundant level of volatile compounds (30 compounds) (Table 4). Acetic acid ethyl ester, isobutylalcohol, 1-butanol, 3-methyl-, benzene, ethenyl-, formic acid, octyl ester, and 2,5-di-tert-butyl-1,4-benzoquinone were the dominant volatile compounds in the beer, and these compounds gradually increased with the germination time of the malted rice. Furthermore, borane-methyl sulfide complex, 1-butanol, 3-methyl-, acetate, benzene, 1,2-bis(iodomethyl)-, 2-butanone, 3-hydroxy-, propanedioic acid, dimethyl-, cyclohexene, 4-[(1E)-1,5-dimethyl-1,4-hexadien-1-yl]-1-methyl-, .alpha.-selinene, .beta.-selinene, hexanoic acid, phenol, 2-methoxy-4-vinylphenol, and imidazole(1,5-a) pyrimidine were observed at low levels in the beer, and the levels gradually increased with germination time. Normally, ethyl esters are common in craft beer, and it is well known that these compounds provide floral, fruit, and herbal characteristics to the beer.35,36 Furthermore, the extended germination could increase the lipid content in the malted rice, and while mashing the malted rice, the lipase enzyme could hydrolyze the lipids and convert them to volatile fatty acids that are then converted to esters. However, the abundant volatile fatty acids could cause off-flavor in the beer. Humia et al. (2019)35 reported that wort's higher gravity could adversely affect ester production. Kongkaew (2010)37 found that extended rice germination significantly increased the ester compounds in the beer. The flavor compounds in the beer have increased with the germination time of the malted HomChaiya rice, which could be due to enzymatic reactions during fermentation.38 Another important flavor compound in the beer is linalool, which is developed from the added hops, and this compound is recognized as the most active and stable (against heat) aromatic compound.39 Witrick et al. (2020)40 mentioned that esters, acids, sulfurs, higher alcohols, diacetyl, aldehydes, and phenols are the major contributors to beer flavor. Octanoic acid, responsible for the cheesy aroma and waxy taste, decreased in the beer when the rice had had extended germination. Rossi et al. (2013)19 reported that fermentation (top, bottom, and spontaneous), yeast type, and hops play crucial roles in the flavor, more so than the malted rice.
3.4 Amino acid profile
Amino acids in the beer are important contributors to the beer's flavor. Normally, the source of amino acids in fermented beverages, particularly in beer, is mainly the proteins in the cereals broken down by the proteolytic enzymes from rice, along with hops as well as yeast.41–43 The nitrogenous compounds (amino acids, peptides, polypeptides, proteins, nucleic acids, and their degradation products) are distinct, and the wort contains 3–5% of nitrogenous compounds.44 The amino acid content in the wort and beer produced using malted HomChaiya rice with various germination times is presented in Table 5. In total, 20 amino acids were identified (including 10 essential amino acids), with significant differences in the quantities by germination time that strongly influenced the amino acid contents (P < 0.05). Among the different amino acids, asparagine, glutamic acid, histidine, arginine, alanine, valine, leucine, lysine, and aspartic acids were the dominant amino acids observed in the wort of malted HomChaiya rice. Sircar and Dastidar (1962)45 reported on amino acid changes in malted rice that were due to extended germination, and the hydrolysis of endospermic protein dominated the first 72 hours of germination. After that, the second phase of embryo activity synthesizes protein from the production of translocation. This could be why extended germination decreased glutamic acid, threonine, lysine, arginine, alanine, and tyrosine in the HomChaiya rice. Serine, glycine, threonine, methionine, phenylalanine, glycine, and tryptophan were also noticed in the wort samples; however, their levels were minimal, and extended germination also significantly influenced the levels of those amino acids. On the other hand, the results showed that fermentation adversely affected amino acid levels. Compared with the wort samples, the amino acids found in the beer were at significantly lower levels in most cases, and among them, it can be seen that the serine, glycine, phenylalanine, tryptophan, and methionine were severely affected by the fermentation process. These were initially found at higher levels in the wort samples but were strongly decreased after fermentation. Ferrerira and Guido (2018)44 mentioned that brewer's yeast could assimilate around 50% of the wort's amino nitrogen. The malting did not affect the beer's amino acid content much. Tyrosine and arginine were the two dominant amino acids that increased in the beer during fermentation. The rice beer's amino acid contents vary widely with the process and the rice species. Zhang et al. (2019)42 observed proline, alanine, glutamic acid, and valine as the dominant amino acids in the rice beer, and this finding contrasts with the present study. Amino acid contents are the crucial sources of nutrition to yeast.46 A decrease in the amino acid content in the beer samples could cause the yeast action, which could utilize the amino acids as a substrate to metabolize it.43,47 Hammond (1993)48 reported that the yeast in beer production requires more than one type of amino acid, which could be associated with the differences in the beers' amino acids in this study. Santos et al. (2020)49 reported that GABA level is high in the brown malted rice as compared with other types. The GABA content in wort was significantly affected by the germination time. A continuous decrement in the GABA level was found in HomChaiya rice when germinated for a prolonged period. Normally, rice contains relatively low level of GABA and however, when it undergone for germination process could increase the GABA content by various biochemical process. Karladee and Suriyong (2012)50 found a similar trend in GABA level of varieties of Thai rice, when they were malted for prolonged period had significantly lower the level of GABA. Brown and Shelp (1997)51 reported that glutamic acid plays a key role in the production of GABA in the malted rice through decarboxylation process by the glutamate decarboxylase enzyme. The present study showed that glutamic acid in the HomChaiya rice under prolonged germination had decreased and this also could be reason for lower GABA in the wort samples. Similarly, a continuous decrease in the GABA level was also noted in the HomChaiya beer. Sahab et al. (2020)52 reported, a lower level of GABA in the beer could be utilized as a nitrogen source by the yeast.
Table 5 Amino acid contents in the wort and beer produced using HomChaiya rice malt from different germination times
Amino acid profile (mg per 100 mL) |
Germination time (days) |
3 |
5 |
7 |
Wort |
Beer |
Wort |
Beer |
Wort |
Beer |
The results are presented as mean ± SD (n = 3). Different superscripts in a row indicate significant differences. |
Asparagine |
11.70 ± 0.70a |
4.82 ± 0.21a |
12.51 ± 0.82a |
5.70 ± 0.40b |
15.41 ± 0.41b |
6.13 ± 0.63c |
Glutamic acid |
11.54 ± 0.81c |
3.27 ± 0.17c |
5.91 ± 0.71b |
2.56 ± 0.05a |
3.41 ± 0.14b |
1.20 ± 0.16a |
Serine |
7.30 ± 0.01a |
1.21 ± 0.05a |
7.85 ± 0.11b |
1.42 ± 0.05b |
8.13 ± 0.84c |
1.75 ± 0.01c |
Histidine |
15.42 ± 0.91a |
9.70 ± 0.50a |
17.81 ± 0.90b |
13.42 ± 0.57b |
18.64 ± 0.82c |
15.61 ± 0.63c |
Arginine |
14.30 ± 0.31a |
5.14 ± 0.17a |
18.12 ± 1.23b |
6.35 ± 0.41b |
20.11 ± 1.01c |
7.86 ± 0.56c |
Glycine |
5.61 ± 0.00a |
4.13 ± 0.11a |
6.24 ± 0.31b |
4.40 ± 0.14b |
7.31 ± 0.63c |
4.97 ± 0.51c |
Threonine |
5.13 ± 0.05c |
3.71 ± 0.13c |
4.82 ± 0.04b |
2.50 ± 0.03b |
3.57 ± 0.11a |
1.73 ± 0.08a |
Alanine |
64.52 ± 0.21a |
44.29 ± 0.12c |
55.81 ± 0.61b |
37.61 ± 2.14b |
48.97 ± 1.11c |
31.17 ± 1.23a |
Tyrosine |
41.71 ± 0.71c |
55.40 ± 0.52a |
34.18 ± 0.51b |
57.92 ± 0.93b |
28.91 ± 1.11a |
62.63 ± 1.17c |
Methionine |
3.60 ± 0.71a |
1.42 ± 0.14a |
4.71 ± 0.11b |
1.73 ± 0.13b |
5.46 ± 0.71c |
2.23 ± 0.44c |
Valine |
19.52 ± 0.13a |
9.10 ± 0.11a |
25.63 ± 0.40b |
9.81 ± 0.17ab |
27.84 ± 0.52c |
11.72 ± 0.13b |
Phenylalanine |
6.17 ± 0.13c |
2.23 ± 0.11a |
6.57 ± 0.14b |
2.74 ± 0.11ab |
5.31 ± 0.42a |
2.90 ± 0.27ab |
Leucine |
41.14 ± 1.13a |
8.71 ± 0.51a |
47.37 ± 0.20b |
9.25 ± 0.41ab |
49.80 ± 0.92c |
9.73 ± 0.64b |
Isoleucine |
10.21 ± 0.61a |
8.93 ± 0.10a |
10.87 ± 0.12b |
9.10 ± 0.27ab |
11.18 ± 0.13c |
9.64 ± 0.91ab |
Lysine |
117.24 ± 2.62c |
91.27 ± 1.10c |
97.82 ± 1.57b |
85.74 ± 0.63b |
85.91 ± 1.12a |
71.56 ± 2.71a |
Aspartic acid |
54.11 ± 0.53a |
17.84 ± 0.51a |
55.49 ± 0.92ab |
21.42 ± 0.71b |
59.12 ± 1.10b |
22.81 ± 0.40b |
Arginine |
20.54 ± 0.92c |
31.51 ± 1.13a |
18.83 ± 1.47b |
37.57 ± 0.62b |
16.73 ± 0.81a |
41.58 ± 0.90c |
Glycine |
3.41 ± 0.22a |
1.70 ± 0.11a |
3.72 ± 0.15b |
1.97 ± 0.13ab |
3.91 ± 0.42c |
2.15 ± 0.11b |
Tryptophan |
7.92 ± 0.61a |
2.11 ± 0.10a |
8.27 ± 0.72b |
2.74 ± 0.17b |
8.71 ± 0.31b |
3.18 ± 0.46c |
GABA |
12.89 ± 0.35b |
6.41 ± 0.92b |
5.87 ± 1.09c |
2.59 ± 0.21c |
3.26 ± 0.89a |
1.58 ± 0.69a |
3.5 Sensory characteristics
The sensory characteristics of beer made using malted HomChaiya rice from different germination times are shown in Fig. 1. Overall, the beer received good consumer scores for its sensorial characteristics. There were few differences among the beer samples produced using the malted HomChaiya, processed with different germination times. However, beer from rice malt that had 5–7 days of germination received a higher score for alcohol than the case with 3 days. Similarly, malted rice from extended germination had increased color and bitterness scores for the beer. Furthermore, the other sensory characteristics such as malty, fruity, sweetness, linger, body, astringent, sour, and acceptance of the beer samples obtained similar scores from the panelists, with no significant differences by germination time (P > 0.05). However, the panelists gave high overall scores to the sensory characteristics of the HomChaiya rice beer, indicating that this type of beer could be further developed into a more refined product. The lower sensory scores of rice beer could predominantly be due to its poor recognition and lack of standards. Mayer et al. (2016)11 had also observed more fruity and malty flavors in rice beer. Sensory analysis is a complex measurement, and the important sensory attributes of the beer include its aftertaste, which is felt after discarding the product from the mouth.53,54 Liu et al. (2012)55 found that sensory analysis is prone to emphasize bitter taste more than the beer's other characteristics. The present study is in accordance with Liu et al. (2012),55 as the bitter taste score was high compared to its other scores. However, the bitterness solely depends on the raw materials and processing conditions of the beer. Ripari et al. (2018)56 reported that oxidation of ingredients plays a crucial role in the bitterness of taste. On the other hand, a very low score for ‘oxidized’ in the sensory analysis indicates good freshness and lack of off-flavors in the HomChaiya rice beer.
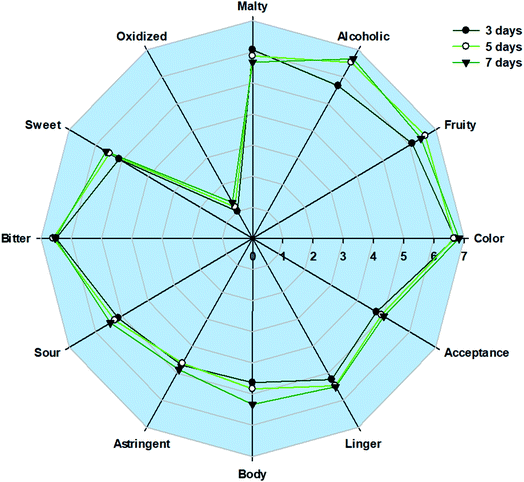 |
| Fig. 1 Sensory characteristics of rice beer produced using HomChaiya rice malt from different germination times. | |
4. Conclusion
The present study found that the germination time of rice has a substantial impact on the wort and beer quality. Furthermore, the physicochemical characteristics of the beer have been influenced by the fermenting process. The prolonged germination period had significantly influenced the physicochemical attributes particularly total nitrogen, free amino acids and malting yield were high in the sample that germinated for 7 days. Consequently, it improved the yeast performance in the beer production and improved the apparent attenuation, flavor, color, and alcoholic contents. Furthermore, the prolonged germination process did not significantly affect the phytochemical contents in the wort samples and however, the fermentation process had increased the phytochemicals and antioxidant activities in the beer. In addition, the volatile contents were high in the beer samples than wort and the prolonged germination time had slightly improved the flavor compounds in the samples. The majority of amino acids in this study was high in the wort samples and the different germination time had not influenced the amino acid much in the samples and however, the fermentation process had significantly affected them, and the majority of the amino acids were high in the wort as compared with beer. Overall, 7 days germinated malted rice positively affected various qualities compared with 3 and 5 days of germination for HomChaiya malted rice and appeared highly suitable for making beer products.
Conflicts of interest
There are no conflicts to declare.
Acknowledgements
This research was financially supported by Prince of Songkla University, Surat Thani Campus Collaborative Research Fund (Grant no. SIT6203022S). In addition, the authors would like to express their gratitude to the Food Innovation and Product Development Laboratory for providing them with research space and equipment.
References
- S. Lekjing and K. Venkatachalam, RSC Adv., 2020, 10, 16254–16265 RSC.
- S. Saetan, Chaiya Rice, 2008, available: available at http://www.rakbankerd.com/agriculture/page/phpid=277%26s=tblrice, accessed on 12 May 2012 Search PubMed.
- F. Wu, H. Chen, N. Yang, J. Wang, X. Duan and Z. Jin, J. Cereal Sci., 2013, 58(2), 263–271 CrossRef CAS.
- P. Saman, A. J. Vazquez and S. S. Pandiella, Process Biochem., 2008, 43, 1377–1382 CrossRef CAS.
- D. Kalita, B. Sarma and B. Srivastava, Food Chem., 2017, 220, 67–75 CrossRef CAS PubMed.
- H. Mizubuchi, T. Yajima, N. Aoi and T. Tomita, Nat. Immunol., 2005, 135, 2857–2861 CAS.
- Y. Teramoto, S. Yoshida and U. Seinosuke, World J. Microbiol. Biotechnol., 2002, 18, 813–816 CrossRef CAS.
- V. M. L. Van, P. Strehaiano, D. C. Nguyen and P. Taillandier, J. Am. Soc. Brew. Chem., 2001, 59(1), 10–16 CrossRef.
- P. Bogdan and E. Kordialik-Bogacka, Trends Food Sci. Technol., 2017, 65, 1–9 CrossRef CAS.
- D. N. Prestes, A. Spessato, A. Talhamento, A. M. Gularte, A. M. Schirmer, L. N. Vanier and V. C. Rombaldi, LWT – Food Sci. Technol., 2019, 112, 108262 CrossRef CAS.
- H. Mayer, D. Ceccaroni, O. Marconi, V. Sileoni, G. Perrertti and P. Fantozzi, LWT – Food Sci. Technol., 2016, 67, 67–73 CrossRef CAS.
- European Brewery Convention (EBC), Analytica, Fachverlag Hans Carl, D-Nürnberg, Germany, 5th edn, 1998 Search PubMed.
- Association of Official Agricultural Chemists (AOAC), Official Method of Analysis, The Association Analytical Chemist, Washington D.C., 17th edn, 2000 Search PubMed.
- L. V. Singleton, R. Orthofer and R. Lamuela, Methods Enzymol., 1999, 299, 152–178 Search PubMed.
- J. Zhishen, T. Mengcheng and W. Jianming, Food Chem., 1999, 64, 555–559 CrossRef CAS.
- W. Brand-Williams, M. E. Cuvelier and C. Berset, LWT – Food Sci. Technol., 1995, 28(1), 25–30 CrossRef CAS.
- R. Re, N. Pellegrini, A. Proteggente, A. Pannala and M. Yang, Free Radical Biol. Med., 1998, 72, 1231–1237 Search PubMed.
- I. F. F. Benzie and J. J. Strain, Methods Enzymol., 1999, 299, 15–27 CAS.
- S. Rossi, V. Sileoni, G. Perretti and O. Marconi, J. Sci. Food Agric., 2013, 94, 919–928 CrossRef PubMed.
- A. Bonke, S. Sieuwerts and L. I. Petersen, Foods, 2020, 9, 429 CrossRef CAS PubMed.
- J. Baranzelli, D. H. Kringel, R. Colussi, F. F. Aranha, M. Z. Miranda, E. R. de Zavareze and A. R. G. Diaz, LWT, 2018, 90, 483–490 CrossRef CAS.
- V. Farzaneh and I. S. Carvalho, J. Appl. Res. Med. Aromat. Plants., 2017, 6, 92–100 Search PubMed.
- Y. Huang, J. Tippmann and T. Becker, Eur. Food Res. Technol., 2017, 243, 1485–1495 CrossRef CAS.
- K. Phattayakorn, P. Pajanyor, S. Wongtecha, A. Prommakool and W. Saveboworn, Int. Food Res. J., 2016, 23(1), 406–409 CAS.
- P. M. Aron, T. H. Shellhammer and J. I. Brew, J. Inst. Brew., 2011, 116, 369–380 CrossRef.
- A. Martinez-Gomez, I. Caballero and A. C. Blanco, Biomolecules, 2020, 10, 400 CrossRef CAS PubMed.
- J. Gasior, J. Kawa-Rygielska and Z. A. Kucharska, Molecules, 2020, 25, 3882 CrossRef CAS PubMed.
- J. Wannenmacher, M. Gastl and T. Becker, Compr. Rev. Food Sci. Food Saf., 2018, 17, 953–988 CrossRef CAS PubMed.
- D. Koren, S. Kun, B. Vecseri Hegyesne and G. Kun-Farkas, J. Food Sci. Technol., 2019, 56, 3801–3809 CrossRef CAS PubMed.
- X. Sun, J. Tang, J. Wang, B. A. Rasco, K. Lai and Y. Huang, Meat Sci., 2016, 116, 1–7 CrossRef CAS PubMed.
- A. Martinez-Gomez, I. Caballero and A. C. Blanco, Biomolecules, 2020, 10, 400 CrossRef CAS PubMed.
- P. D. Schutter, D. Saison, F. Delvaux, G. Derdelinckx, M. J. Rock, H. Neven and R. F. Delvaux, J. Agric. Food Chem., 2008, 56, 246–254 CrossRef PubMed.
- O. Marconi, V. Sileoni, D. Ceccaroni and G. Perretti, The use of rice in brewing, in Advances in International Rice Research, IntechOpen, UK, 2017, pp. 49–66 Search PubMed.
- P. Schwarz, S. Patriciz and S. Sean, J. Am. Soc. Brew. Chem., 2002, 60(3), 107–109 CrossRef CAS.
- B. V. Humia, K. S. Santos, A. M. Barbosa, M. Sawata, M. da Costa Medonca and F. F. Padilha, Molecules, 2019, 24, 1568–1574 CrossRef CAS PubMed.
- H. M. Bettenhausen, B. Amanda, F. Scott, H. Dustin, H. Javier, J. Lim, Q. H. Sue, H. T. Shellhammer, V. Veronica, Y. Linxing, H. L. Adam and H. M. Patrick, J. Am. Soc. Brew. Chem., 2020, 78(2), 136–152 CrossRef CAS.
- A. Kongkaew, Optimization of beer production from rice malt based using response surface methodology, MSc thesis, Suranaree University of Technology, Bangkok, 2010.
- D. W. K. Toh, J. Y. Chua and S. Q. Liu, LWT – Food Sci. Technol., 2018, 91, 26–33 CrossRef CAS.
- K. Takoi, K. Koie, Y. Itoga, Y. Katayama, M. Shimase, Y. Nakayama and J. Watari, J. Agric. Food Chem., 2010, 58, 5050–5058 CrossRef CAS PubMed.
- K. Witrick, R. E. Pitts and F. S. O'Keefe, Beverages, 2020, 6, 31 CrossRef CAS.
- J. M. Poveda, Food Control, 2019, 96, 227–233 CrossRef CAS.
- T. Zhang, H. Zhang, Z. Yang, Y. Wang and H. Li, Food Sci. Nutr., 2019, 7, 3664–3674 CrossRef CAS PubMed.
- K. Venkatachalam, C. Techanknon and S. Thitithanakul, ACS Omega, 2018, 3, 6710–6718 CrossRef CAS PubMed.
- M. I. Ferrerira and F. L. Guido, Fermentation, 2018, 4, 23 CrossRef.
- S. M. Sircar and G. A. Dastidar, Physiol. Plant., 1962, 15, 206–210 CrossRef CAS.
- C. Nie, C. Wang, G. Zhou, F. Dou and M. Huang, Afr. J. Biotechnol., 2010, 9(53), 9018–9025 CAS.
- C. Lucie, N. Thibault, S. Isabelle, D. Sylvie and C. Carole, Appl.
Environ. Microbiol., 2012, 78(22), 8102–8111 CrossRef PubMed.
- J. R. M. Hammond, Brewer's yeasts, in The Yeast, Academic Press, London, 1993, pp. 7–67 Search PubMed.
- J. P. Santos, T. S. Acunha, D. N. Prestes, C. V. Rombaldi, S. L. M. E. Halal and N. L. Vanier, Cereal Chem., 2020, 97(6), 1148–1157 CrossRef.
- D. Karladee and S. Suriyong, ScienceAsia, 2012, 38, 13–17 CAS.
- A. W. Brown and B. J. Shelp, Plant Physiol., 1997, 4(11), 1–5 Search PubMed.
- N. R. M. Sahab, E. Subroto, R. L. Balia and G. L. Utama, Heliyon, 2020, 6, e05526 CrossRef PubMed.
- K. Deans, A. Pinder, B. J. Catley and J. A. Hodgson, Proceedings of the European Brewing Convention Congress, IRL Press: Oxford, Maastricht, 1997 Search PubMed.
- A. S. Langstaff, X. J. Guinard and J. M. Lewis, J. Inst. Brew., 1991, 97(6), 427–433 CrossRef.
- C. Liu, J. Dong, J. Wang, X. Yin and Q. Li, J. Inst. Brew., 2012, 118, 325–333 CrossRef CAS.
- V. Ripari, M. Tomassetti, T. Cecchi and B. Enrico, Eur. Food Res. Technol., 2018, 244, 2027–2040 CrossRef CAS.
|
This journal is © The Royal Society of Chemistry 2021 |