DOI:
10.1039/D1RA05405H
(Paper)
RSC Adv., 2021,
11, 28530-28534
Selective oxidation of alcohol-d1 to aldehyde-d1 using MnO2†
Received
14th July 2021
, Accepted 16th August 2021
First published on 24th August 2021
Abstract
The selective oxidation of alcohol-d1 to prepare aldehyde-d1 was newly developed by means of NaBD4 reduction/activated MnO2 oxidation. Various aldehyde-d1 derivatives including aromatic and unsaturated aldehyde-d1 can be prepared with a high deuterium incorporation ratio (up to 98% D). Halogens (chloride, bromide, and iodide), alkene, alkyne, ester, nitro, and cyano groups in the substrates are tolerated under the mild conditions.
1. Introduction
Deuterium (2H, d) is a stable, non-radioactive, and safe isotope of hydrogen (1H). Since its discovery,1 d has been widely utilized in organic chemistry, biochemistry, analytical chemistry, pharmaceutical science, and drug discovery.2,3 Because of the high demand for d-labelled molecules in the scientific research fields, many efforts have been devoted to developing a new method for the synthesis of d-labelled molecules.
Aldehyde-d1 2 has received significant attention as a synthetic target due to the facts that aldehyde 1 is a useful feedstock in organic synthesis. Various methods have been performed in the synthesis of alkyl and aryl aldehyde-d1. For example, more than 40 syntheses (25 different reaction conditions) of benzaldehyde-d1 (PhCDO) were conducted even since 2018 in the studies to develop new d-incorporation method or reaction mechanism using PhCDO.4–8
The previous synthetic approaches to access d-labelled molecules are classified into 5 types; (A) addition of D− followed by oxidation, (B) carbonyl Umpolung approach, (C) radical reaction, (D) transition metal-catalysed reaction, and (E) others. Recently, mild, one-step, and catalytic syntheses of aldehyde-d1 2 have been achieved by deuteration of the Breslow intermediates,9 deuteration of acyl radicals,6,10 and transition metal-catalysed deuterium incorporation.11 However, the previous synthetic methods including the modern direct syntheses often suffered from drawbacks such as over-deuteration, requirements of harsh conditions (high and low temperature, and strong base and acids), and the use of expensive catalysts. Moreover, the synthetic examples of substituted acrolein and propynal-d1 are much less than those of alkyl and aryl aldehyde-d1,12,13 though recently developed NHC-catalysed H–D exchange reactions allowed access to various substituted acrolein-d1 derivatives.9 In this context, development of a new d-incorporation method which allows flexible synthesis of aromatic and unsaturated aldehyde-d1 2 remains to be a challenging synthetic task (Scheme 1).
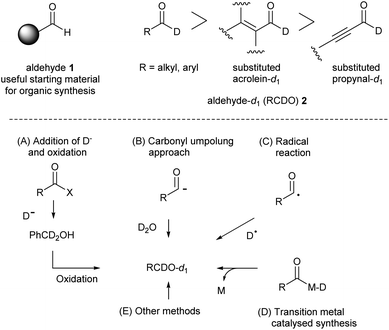 |
| Scheme 1 Synthesis of aldehyde-d1 derivatives. (A) reduction of the formyl with D− and oxidation, (B) carbonyl Umpolung approach, (C) radical H–D exchange, (D) transition metal-catalysed H–D exchange, and (E) others. | |
Method A using D− as a deuterium source has been recognized as a robust and conventional synthetic method to prepare aldehyde-d1 2 (Scheme 2). The synthesis is typically performed in two steps; (i) reduction of carboxylic acid derivatives using LiAlD4 to provide alcohol-d2 3 and (ii) oxidation to aldehyde-d1 2 (eqn (1)).14 In this approach, the deuterium incorporation ratio (%D) of the commercially available D− sources such as LiAlD4 (>98 atom) is reliably transferred into the product. On the other hand, the use of highly reactive LiAlD4 often limits the synthetic scope. Under the conditions, various functional groups such as nitro, nitrile, ester, and acid moieties, and alkene and alkyne with electron-withdrawing group(s) are not tolerated. To overcome the limitation, we emerged selective oxidation of alcohol-d1 derivatives 4 (Scheme 2(eqn (2))). It is expected that various alcohol-d1 4 can be prepared by the mild NaBD4 reduction. The next selective oxidation of D (H/D selectivity) is the key to this approach. Recently, oxidation of benzyl alcohol-d1 (PhCDHOH) with PCC or PDC was conducted to prepare PhCDO with ∼85%D.4a,4e,4q On the other hand, further efforts to improve the selectivity (%D) in the selective oxidation have not been well-examined. Herein, we would like to report that NaBD4 reduction followed by activated MnO2 oxidation (NaBD4/MnO2 system). The simple and mild protocol allows expansion of the synthetic range of aldehyde-d1 2 including not only aromatic aldehyde-d1 derivatives but also substituted acrolein-d1 and propynal-d1 derivatives with high %D (up to 98%).
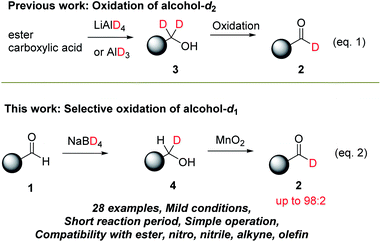 |
| Scheme 2 Oxidation of deuterated alcohols. Eqn (1): oxidation of alcohol-d2, eqn (2): selective oxidation of alcohol-d1. | |
2. Results and discussion
In a similar manner to the previous synthetic examples of NaBH4 reduction of aldehyde 1, the reduction with NaBD4 gave the corresponding alcohol-d1 derivatives 4 with excellent functional group compatibility and yields (Scheme 3). Chloride, bromide, iodide, methoxy, ethoxy, or methylene acetal, nitrile, ester, nitro, and alkyne groups on the aromatic ring of 4c–4q were tolerated under the conditions. Substituted acrolein and propynal 1r–1aa also underwent smooth NaBD4 reduction to provide 4r–4aa without loss of the alkyne and alkene moieties, and tetrahydropyanyl (THP), benzoyl (Bz), and tert-butyldimethylsilyl (TBS) protecting groups.
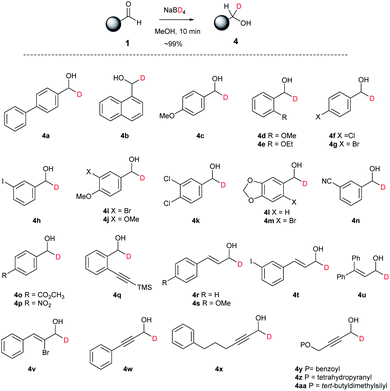 |
| Scheme 3 Reduction of aldehyde 1 with NaBD4. | |
We next examined the key oxidation of alcohol-d1 4 using 4-phenylbenzyl alcohol-d1 (4a) (Table 1). As a result, activated MnO2 was found to be superior to other general oxidation reagents (entry 1, Table 1). Treatment of 4a with 23 eq. of MnO2 in CH2Cl2 gave aldehyde-d1 2a with 92% D in 2 h. The use of pyridinium dichlorochromate (PDC) gave 2a in good selectivity (88% D).4e However, the isolate yield was moderate (entry 2). Dess–Martin periodinane oxidation, 2,2,6,6-tetramethylpiperidine 1-oxyl (TEMPO) oxidation in the presence of PhI(OAc)2, and Parikh–Doering oxidation (sulfur trioxide–pyridine complex in dimethyl sulfoxide (DMSO)) resulted in lower selectivities (74, 76 and 66%D, entries 3–5).
Table 1 Oxidation of alcohol-d1 4aa
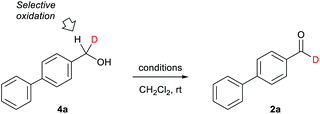
|
Entry |
Conditions |
Yieldb (%) |
%Dc |
0.5 mmol scale. Isolated yield. %D for 2a is calculated based on the integration ratios of aldehyde and aromatic proton. MnO2 = activated manganese dioxide, PDC = pyridinium dichlorochromate, MS4A = molecular sieves 4A, TEMPO = 2,2,6,6-tetramethylpiperidine 1-oxyl, DMSO = dimethyl sulfoxide. |
1 |
MnO2 (23 eq.), 1 h |
92 |
92 |
2 |
PDC (1.2 eq.), MS4A, 2 h |
51 |
88 |
3 |
Dess–Martin periodinane (1.5 eq.), 5 min |
84 |
74 |
4 |
TEMPO (0.01 eq.), Bu4NHSO4 (0.05 eq.), NaOCl (1.2 eq.), 1 h |
96 |
76 |
5 |
DMSO (10 eq.), SO3–pyridine (4 eq.), iPr2NEt (5 eq.), 1.5 h |
75 |
66 |
Activated MnO2 oxidation was successfully expanded to the synthesis of various aldehyde-d1 2a–2aa with high %D (85–96%D) (Scheme 4A–C). Chloride, bromide, iodide, methoxy, ethoxy, or methylene acetal, nitrile, ester, nitro, and alkyne groups on the aromatic ring of 4c–4q are preserved under the mild oxidation conditions (Scheme 4A). Substituted acrolein 4r–4v and propynal 4w–4aa smoothly underwent MnO2 oxidation to provide 2r–2aa without loss of the alkene and alkyne moieties (Scheme 4B and C). The synthetic utility was further demonstrated by the synthesis of 2v with a bromo group at the α-position of cinnamaldehyde. In addition, Bz, THP, and TBS protecting groups of 4y, 4z, and 4aa were also maintained under the conditions. These propargyl alcohols 4y, 4z, and 4aa were smoothly converted to the corresponding propynal derivatives 2y, 2z, and 2aa with high %D, respectively.
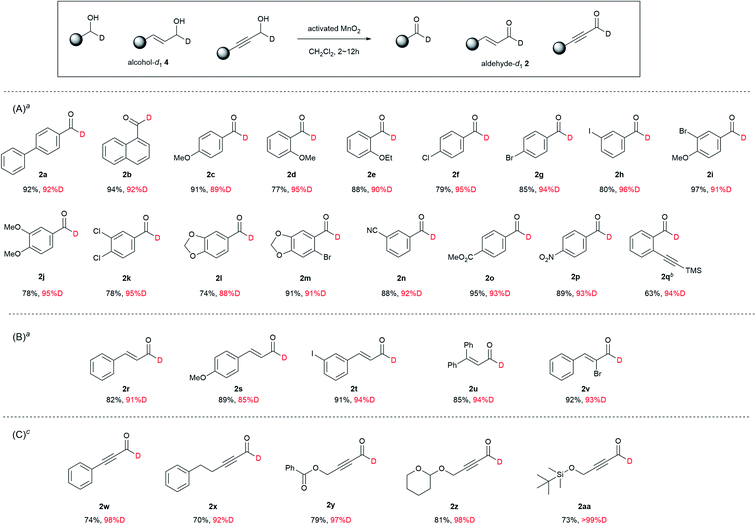 |
| Scheme 4 MnO2 oxidation of alcohol-d1 4. (A) synthetic examples of aromatic aldehyde-d1 2, (B) synthetic examples of substituted acrolein-d1 2, and (C) synthetic examples of substituted propynal-d1 2. a2 h, b6 h, c12 h. | |
In conjunction with our recent efforts toward elucidation of biosynthetic reaction mechanisms of terpene synthases using d-labelled prenols,15,16 we needed geranylgeraniol-d2 (6) as an enzyme substrate. Previously, the synthesis of 6 (ref. 17) and other acyclic prenol-d2 derivatives18 was performed in four steps from 5 via reduction of ester 7 with LiAlD4. However, commercially available LiAlD4 is almost out of stock in recently years. In addition, low temperature conditions (−20 °C) is required for the LiAlD4 reduction to avoid the undesired 1,4-reduction. We expected that NaBD4/MnO2 system would be an alternative to the LiAlD4 procedure to prepare 6, conveniently. According to the literature,19 geranylgeraniol (5) was converted to aldehyde 8 by MnO2 oxidation (Scheme 5). Aldehyde 8 was subjected to NaBD4/MnO2 to deliver d-enriched aldehyde 9 which was subsequently reduced by NaBD4 to provide geranylgeraniol-d2 (6) in 70% yield over four steps with satisfactory deuterium incorporation ratio (94% D). Under the conditions, the undesired 1,4-addition reaction was not observed. Thus, an operationally simple and mild deuteration of prenols-d2 was achieved by application of NaBD4/MnO2 system.
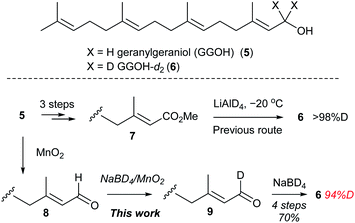 |
| Scheme 5 Synthesis of geranylgeraniol-d2 (6) from geranylgeraniol (5). | |
Previously, Brecker et al. investigated 13C kinetic isotope effects (KIEs) in the oxidation of cinnamyl alcohol using MnO2, Dess–Martin periodinane, and Swern oxidation (DMSO/(COCl)2/Et3N) to gain insight into the reaction mechanism.20 Comparison of the kinetic isotope of effects revealed the following order MnO2 > Dess–Martin oxidation ≈ Swern oxidation. The higher 13C KIE using MnO2 displayed that the C–H bond breaking in the intermediate is irreversible and rate-determining, and the oxidation proceeded via energy rich transition state. On the other hand, the lower 13C KIEs observed in Swern oxidation and Dess–Martin oxidation indicated that the intramolecular C–H bond cleavage in these oxidation reaction processes would not be slower to be rate-limiting.
Experimental results in Table 1 clearly shows that the degrees of %D are as follows MnO2 > PDC > TEMPO ≈ Dess–Martin > SO3–pyridine/DMSO. It is speculated that higher %D of MnO2 oxidation and lower %D of SO3–pyridine/DMSO oxidation would correlate to the 13C KIE data (MnO2 > Swern oxidation). It is interesting to note that the %D value in Scheme 4 depended on the substrates. The oxidation of propargyl alcohols 4w–4aa resulted in higher %D than those of the other alcohols. The oxidation of 4w–4aa needed a longer reaction period to complete the reactions. As mentioned in the previous 13C KIE studies, the rate limiting steps of the MnO2 oxidation relies on the C–H cleavage step of the reaction intermediate. It is considered that the slower C–H cleavage would provide the higher %D.
3. Conclusions
We have established a facile synthesis of aldehyde-d1 derivatives by NaBD4/MnO2 system. The new method is characterized by a high degree of functional group compatibility and a wide range of substrate scope including the synthesis of d-containing unsaturated aldehydes. Aromatic aldehyde-d1 derivatives such as 2c and 2g would be a useful synthetic intermediate for olefination, amination, hydride reduction, Suzuki cross coupling, and Sonogashira coupling reactions.9e,10c Substituted acroleins and propynals can be used for Michael addition reaction, cycloaddition reaction, and transition metal catalysed transformations. In this context, NaBD4/MnO2 system would offer vital opportunity to the synthesis of highly functionalized d-labelled molecules via facile preparation of aromatic and unsaturated aldehyde-d1 2. Deuterium-labelled compounds are often needed for the investigation of the mechanisms or determination of the rate-limiting step. The present synthetic method supports the studies from the viewpoint of the facile preparation of aldehyde-d1 2 and its derivatives. Further application and mechanism studies are ongoing in our laboratory.
Author contributions
Y. Yasuno and HO are contributed equally. Y. Yasuno, HO, and TS designed the synthetic route. TS wrote the manuscript. HO, Y. Yasuno, and AN prepared ESI.† HO, Y. Yasuno, AN, K. Kumadaki, K. Kitsuwa, KO, YT, and Y. Yamamoto performed syntheses of 2.
Conflicts of interest
There are no conflicts to declare.
Acknowledgements
This work was financially supported from JSPS Kakenhi (JP19H04661 for TS, JP20K09396 for AN, and JP21K14629 for YYasuno), the OCU ‘Think globally, act locally’ Research Grant for Young Scientists 2021 through the hometown donation fund of Osaka City for AN, the Uehara Memorial Foundation for AN, and the Sasakawa Scientific Research Grant from The Japan Science Society for HO.
Notes and references
- H. C. Urey, F. G. Brickwedde and G. M. Murphy, Phys. Rev., 1932, 39, 164 CrossRef CAS.
- J. Yang, in Deuterium: Discovery and Applications in Organic Chemistry, Elsevier, Amsterdam, 2016 Search PubMed.
-
(a) T. Pirali, M. Serafini, S. Cargnin and A. A. Genazzani, J. Med. Chem., 2019, 62, 5276 CrossRef CAS;
(b) J. Atzrodt, V. Derdau, W. J. Kerr and M. Reid, Angew. Chem., Int. Ed., 2018, 57, 1758 CrossRef CAS;
(c) A. Mullard, Nat. Rev. Drug Discovery, 2016, 15, 219 CrossRef CAS PubMed;
(d) A. Katsnelson, Nat. Med., 2013, 19, 656 CrossRef CAS PubMed;
(e) J. M. Herbert, J. Labelled Compd. Radiopharm., 2010, 53, 658 CrossRef CAS.
- Synthesis of PhCDO (type A):
(a) A. S. K. Raj, A. S. Narode and R.-S. Liu, Org. Lett., 2021, 23, 1378 CrossRef PubMed;
(b) M. Zeng, C. Lou, J.-W. Xue, H. Jiang, L. Kaiwen, Z. Chen, S. Fu and G. Yin, Appl. Organomet. Chem., 2021, 35, e6093 CrossRef CAS;
(c) L. Kang, J. Zhang, H. Yang, J. Quan and G. Jinlong, Org. Lett., 2020, 22, 9118 CrossRef CAS PubMed;
(d) J.-Y. Gu, W. Zhang, S. R. Jackson, Y.-H. He and Z. Guan, Chem. Commun., 2020, 56, 13441 RSC;
(e) J.-L. Xu, H. Tian, J.-H. Kang, W.-X. Kang, W. Sun, R. Sun, Y.-M. Li and M. Sun, Org. Lett., 2020, 22, 6739 CrossRef CAS PubMed;
(f) C. Lázaro-Milla, J. Macicior, H. Yanai and P. Almendros, Chem.–Eur. J., 2020, 26, 8983 CrossRef PubMed;
(g) Y. Yang, X. Zhang, L.-P. Zhong, J. Lan, X. Li, C.-C. Li and L. W. Chung, Nat. Commun., 2020, 11, 1850 CrossRef CAS PubMed;
(h) Y. Zhang, W. Schilling, D. Riemer and S. Das, Nat. Protoc., 2020, 15, 822 CrossRef CAS PubMed;
(i) F. Hu, Z. Chen, Y. Tan, D. Xu, S. Huang, S. Jia, X. Gong, W. Qin and H. Yan, Org. Lett., 2020, 22, 1934 CrossRef CAS PubMed;
(j) J.-X. Wang, X.-T. Zhou, Q. Han, X.-X. Guo, X.-H. Liu, C. Xue and H.-B. Ji, New J. Chem., 2019, 43, 19415 RSC;
(k) T. Kaicharla, B. M. Zimmermann, M. Oestreich and J. F. Teichert, Chem. Commun., 2019, 55, 13410 RSC;
(l) S.-S. Meng, L.-R. Lin, X. Luo, H.-J. Lv, J.-L. Zhao and A. S. C. Chan, Green Chem., 2019, 21, 6187 RSC;
(m) G.-F. Pan, X.-L. Zhang, X.-Q. Zhu, R.-L. Guo and Y.-Q. Wang, iScience, 2019, 20, 229 CrossRef CAS PubMed;
(n) G. Nicolau, G. Tarantino and C. Hammond, ChemSusChem, 2019, 12, 4953 CrossRef CAS PubMed;
(o) A. K. Bains, A. Kundu, S. Yadav and D. Adhikari, ACS Catal., 2019, 9, 9051 CrossRef CAS;
(p) J. Qian, J. Zhang, H. Yang, L. Kang and G. Jiang, Chem. Sci., 2019, 10, 8812 RSC;
(q) R. D. Kardile and R.-S. Liu, Org. Lett., 2019, 21, 6452 CrossRef CAS PubMed;
(r) J. L. DiMeglio, A. G. Breuhaus-Alvarez, S. Li and B. M. Bartlett, ACS Catal., 2019, 9, 5732 CrossRef CAS;
(s) T. Yatabe, N. Mizuno and K. Yamaguchi, ACS Catal., 2018, 8, 11564 CrossRef CAS;
(t) D. Wang, W. Liu, Y. Hong and X. Tong, Org. Lett., 2018, 20, 5002 CrossRef CAS PubMed;
(u) M. Zhang, Y. Zhai, S. Ru, D. Zang, S. Han, H. Yu and Y. Wei, Chem. Commun., 2018, 54, 10164 RSC;
(v) Z.-P. Wang, Y. He and P.-L. Shao, Org. Biomol. Chem., 2018, 16, 5422 RSC;
(w) T. C. Malig, D. Yu and J. E. Hein, J. Am. Chem. Soc., 2018, 140, 9167 CrossRef CAS;
(x) S. Shirase, K. Shinohara, H. Tsurugi and K. Mashima, ACS Catal., 2018, 8, 6939 CrossRef CAS;
(y) W. Schilling, D. Riemer, Y. Zhang, N. Hatami and S. Das, ACS Catal., 2018, 8, 5425 CrossRef CAS;
(z) Z.-Z. Zhou, M. Liu, L. Lv and C.-J. Li, Angew. Chem., Int. Ed., 2018, 57, 2616 CrossRef CAS.
- Synthesis of PhCDO (type B):
(a) D.-X. Zhu, H. Xia, J.-G. Liu, L. W. Chung and M.-H. Xu, J. Am. Chem. Soc., 2021, 143, 2608 CrossRef CAS PubMed;
(b) S. K. Mahesh, J. B. Nanubolu and G. Sudhakar, J. Org. Chem., 2019, 84, 7815 CrossRef CAS.
- Synthesis of PhCDO (type C): Y. Kuang, H. Cao, H. Tang, J. Chew, W. Chen, X. Shi and J. Wu, Chem. Sci., 2020, 11, 8912 RSC.
- Synthesis of PhCDO (type D):
(a) Z. Liu, P. Wang, Z. Yan, S. Chen, D. Yu, X. Zhao and T. Mu, Beilstein J. Org. Chem., 2020, 16, 645 CrossRef CAS PubMed;
(b) Z. Liu, Z. Yang, B. Yu, X. Yu, H. Zhang, Y. Zhao, P. Yang and Z. Liu, Org. Lett., 2018, 20, 5130 CrossRef CAS.
- Synthesis of PhCDO (type E):
(a) A. Baykal and B. Plietker, Eur. J. Org. Chem., 2020, 1145 CrossRef CAS;
(b) S. K. Mahesh, J. B. Nanubolu and G. Sudhakar, J. Org. Chem., 2019, 84, 7815 CrossRef CAS;
(c) N. A. Eberhardt, N. P. N. Wellala, Y. Li, J. A. Krause and H. Guan, Organometallics, 2019, 38, 1468 CrossRef CAS;
(d) T. Li, B.-H. Xu, D.-P. Zhu, Y.-F. Wang and S.-J. Zhang, Org. Chem. Front., 2018, 5, 1933 RSC.
-
(a) V. G. Landge, K. K. Shrestha, A. J. Grant and M. C. Young, Org. Lett., 2020, 22, 9745 CrossRef CAS;
(b) S. C. Gadekar, V. Dhayalan, A. Nandi, I. L. Zak, S. Barkai, M. S. Mizrachi, S. Kozuch and A. Milo, ChemRxiv, 2020, 1 Search PubMed;
(c) A. Palazzolo, T. Naret, M. Daniel-Bertrand, D.-A. Buisson, S. Tricard, P. Lesot, Y. Coppel, B. Chaudret, S. Feuillastre and G. Pieters, Angew. Chem., Int. Ed., 2020, 59, 20879 CrossRef CAS;
(d) Y. Sawama, Y. Miki and H. Sajiki, Synlett, 2020, 31, 699 CrossRef CAS;
(e) H. Geng, X. Chen, J. Gui, Y. Zhang, Z. Shen, P. Qian, J. Chen, S. Zhang and W. Wang, Nat. Catal., 2019, 2, 1071 CrossRef CAS PubMed;
(f) W. Liu, L.-L. Zhao, L.-M. Melaimi, L. Cao, X. Xu, J. Bouffard, G. Bertrand and X. Yan, Chem, 2019, 5, 2484 CrossRef CAS.
-
(a) J.-Y. Dong, W.-T. Xu, F.-Y. Yue, H.-J. Song, Y.-X. Liu and Q.-M. Wang, Tetrahedron, 2021, 82, 131946 CrossRef CAS;
(b) Y. Zhang, P. Ji, Y. Dong, Y. Wei and W. Wang, ACS Catal., 2020, 10, 2226 CrossRef CAS;
(c) J. Dong, X. Wang, Z. Wang, H. Song, Y. Liu and Q. Wang, Chem. Sci., 2020, 11, 1026 RSC.
-
(a) M. Y. S. Ibrahim and S. E. Denmark, Angew. Chem., Int. Ed., 2018, 57, 10362 CrossRef CAS;
(b) W. J. Kerr, R. M. Reid and T. Tuttle, Angew. Chem., Int. Ed., 2017, 56, 7808 CrossRef CAS.
- Synthesis of cinnamaldehyde-d1:
(a) B. D. Mokar and C. S. Yi, Organometallics, 2019, 38, 4625 CrossRef CAS;
(b) X. Li, S. Wu, S. Chen, Z. Lai, H.-B. Luo and C. Sheng, Org. Lett., 2018, 20, 1712 CrossRef CAS;
(c) Y. Shi, B. Jung, S. Torker and A. H. Hoveyda, J. Am. Chem. Soc., 2015, 137, 8948 CrossRef CAS PubMed;
(d) T. Fujihara, C. Cong, T. Iwai, J. Terao and Y. Tsuji, Synlett, 2012, 23, 2389 CrossRef CAS.
- Synthesis of 3-phenylpropinal-d1:
(a) B. Zhou, Q. Wu, Z. Dong, J. Xu and Z. Yang, Org. Lett., 2019, 21, 3594 CrossRef CAS PubMed;
(b) A. Verlee, T. Heugebaert, T. van der Meer, P. Kerchev, F. Van Breusegem and C. V. Stevens, Org. Biomol. Chem., 2018, 16, 9359 RSC;
(c) A. Vallet, A. Janin and R. Romanet, J Labelled Compd, 1971, 7, 80 CrossRef CAS.
- Alternative practical synthesis of PhCDO from benzyl, see ref. 4c, 4i, 4p, and 4w.
-
(a) R. Stepanova, H. Inagi, K. Sugawara, K. Asada, T. Nishi, D. Ueda, Y. Yasuno, T. Shinada, K. Miki, M. Fujihashi and T. Sato, ACS Chem. Biol., 2020, 15, 1517 CrossRef CAS;
(b) Q. Chen, J. Li, Z. Liu, T. Mitsuhashi, Y. Zhang, H. Liu, Y. Ma, J. He, T. Shinada, T. Sato, Y. Wang, H. Liu, I. Abe, P. Zhang and G. Wang, Plant Commun., 2020, 100051 CrossRef;
(c) Y. Totsuka, S. Ueda, T. Kuzuyama and T. Shinada, Bull. Chem. Soc. Jpn., 2015, 44, 575 CrossRef;
(d) A. Meguro, Y. Motoyoshi, K. Teramoto, S. Ueda, Y. Totsuka, Y. Ando, T. Tomita, S.-Y. Kim, T. Kimura, M. Igarashi, R. Sawa, T. Shinada, M. Nishiyama and T. Kuzuyama, Angew. Chem., Int. Ed., 2015, 54, 4353 CrossRef CAS.
- For a review using stable isotopes in the study of terpene biosynthesis: J. Rinkel and J. S. Dickschat, Beilstein J. Org. Chem., 2015, 11, 2493 CrossRef CAS.
- V. A. Clausen, R. L. Edelstein and M. D. Distefano, Biochemistry, 2001, 40, 3920 CrossRef CAS PubMed.
- N. Duhamel, D. Martin, R. Larcher, B. Fedrizzi and D. Barker, Tetrahedron Lett., 2016, 57, 4496 CrossRef CAS.
- M. P. Doyle and M. Yan, ARKIVOC, 2002,(viii), 180 Search PubMed.
- L. Brecker, M. F. Kögl, C. E. Tyl, R. Kratzerb and B. Nidetzky, Tetrahedron Lett., 2006, 47, 4045 CrossRef CAS.
Footnotes |
† Electronic supplementary information (ESI) available. See DOI: 10.1039/d1ra05405h |
‡ HO and Y. Yasuno contributed equally to this study. |
|
This journal is © The Royal Society of Chemistry 2021 |
Click here to see how this site uses Cookies. View our privacy policy here.