DOI:
10.1039/D1RA05093A
(Paper)
RSC Adv., 2021,
11, 30898-30910
Effect of different superfine grinding technologies on the physicochemical and antioxidant properties of tartary buckwheat bran powder
Received
1st July 2021
, Accepted 12th September 2021
First published on 17th September 2021
Abstract
The effect of shear crushing, airflow comminution, and wet grinding on the physical and chemical properties of Tartary buckwheat bran (TBB) powder was compared. Superfine grinding significantly reduces the particle size of bran (1.644 μm), while increasing the protein content (23.60%), water-holding capacity (4.38 g g−1), solubility (21.077 g 100 g−1), bulk density (0.34 g mL−1), and tap density (0.53 g mL−1) providing good processing characteristics. The antioxidant properties of bran powder prepared by the three methods mentioned above were compared. The results showed that different bran powders subjected to superfine grinding displayed varying levels of antioxidant capacity. The quercetin content (2.18 g 100 g−1) of the wet-grinding bran powder (WGBP) was twice that of the control group, while no rutin was detected. The total flavonoid content (TFC) and total phenolic content (TPC) were significantly different from those of other groups. The DPPH, ˙OH, and ABTS+ removal rates were 60.74%, 86.62%, and 92.98%, respectively, while that of ˙OH was significantly higher than in the other treatment groups. The control group, shear crushed, and airflow comminution bran exhibited no significant differences in TFC, TPC, and oxidation resistance, except for the ability to remove ˙OH. TBB powder obtained via superfine grinding displayed superior taste and functional characteristics, providing a theoretical reference for the processing of this bran.
1. Introduction
Tartary buckwheat (Fagopyrum tataricum Gaertn) is a unique medicinal and food crop in China, mainly concentrated in high-altitude alpine regions and mountainous plateau areas. This bran is highly nutritious and healthy and has been attracting increasing attention due to its unique chemical composition and nutritional efficacy in preventing and controlling a variety of diseases. Tartary buckwheat is rich in various functional components, such as flavonoids, phenolic acids, essential amino acids, minerals, and bioactive peptides.1 It displays antioxidant, anti-aging, anti-tumor, and antibacterial properties while having a beneficial effect on hypoglycemia and blood pressure and can be used to combat fatigue and other symptoms.2 Rutin, a polyphenolic compound widely found in plants, exhibits several health benefits, such as antioxidant and anti-inflammatory activity.3 Buckwheat is the only cereal containing rutin and is considered its primary dietary source.4 Studies have found that adding rutin to a digestive flour mixture can improve pepsin digestibility.5 Although the molecular structures of rutin and quercetin are similar, they differ in that the rutin in the C ring is replaced by –OH in quercetin (Fig. 1), suggesting a homogeneous relationship.6 Quercetin, a typical flavonoid, represents a secondary metabolite in the plant kingdom. In plants, quercetin exists in glycosylated forms, such as glycoside, galactoside, rhamnoside, arabinoside, and rutin glycoside. In addition, quercetin displays higher antioxidant activity than rutin, exhibiting anti-allergic, anti-cancer, hypoglycemic, and antibacterial properties.6,7
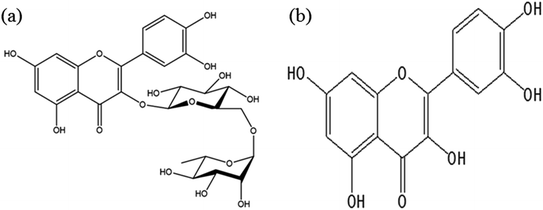 |
| Fig. 1 Molecular structure of rutin (a) and quercetin (b). | |
Tartary buckwheat bran (TBB) is a by-product produced during Tartary buckwheat flour processing, accounting for about 24.4%. It is rich in protein, fat, dietary fiber, polyphenols, and flavonoids. The total phenolic content (TPC) in buckwheat bran and shells is 2 to 7 times higher than in barley, triticale, and oats.8 The free phenolic extracts from TBB exhibits a dose-dependent anti-proliferative effect on human hepatocellular carcinoma HepG2 cells, colon cancer Caco-2 cells, and breast cancer MDA-MB-231 cells.9 According to related reports, the rutin content in buckwheat bran is higher than other components.4 However, when buckwheat grains are crushed, only buckwheat flour is used for food processing, while the shell and bran by-products are discarded as waste. TBB can be used as a source of rutin to enrich wheat food products. By adding 2%, 4%, and 6% rutin, its product content is 0.28–1.35 g 100 g−1, reaching the recommended daily intake of rutin. In addition, rutin enrichment also promotes antioxidant activity in wheat products by improving DPPH and ABTS free radical scavenging and enhancing iron reduction.4 After baking, the fatty acid, polysaccharide, and polyphenol content in Tartary buckwheat flour decrease. However, the amino acid and total flavonoid content (TFC) are higher than in TBB powder, which shows a decrease in these substances. In addition, the TBB rutin content decreases after baking, while a significant increase of 68.92% is evident in Tartary buckwheat flour.10 This indicates that different processing methods should be adopted according to the characteristics of materials for maximum productivity.
Superfine grinding is when material particles are quickly crushed to the micron level using mechanical equipment and is essential for material pretreatment during food processing.11 Superfine powder has various physical and chemical properties superior to bulk or granular materials, such as good fluidity, adsorption, chemical activity, easy dissolution of nutrients, and biological activity retention.12 Furthermore, since it can improve biological potency in the body and maximize the utilization of biological resources, it has been widely used in various fields, such as agriculture, medicine, food, cosmetics, and aerospace.13–15 The total dietary and soluble dietary fiber extracted from buckwheat shells after superfine grinding is higher than that obtained from coarse buckwheat shell powder. Moreover, the water-holding capacity, oil-holding capacity, solubility, nitrite ion adsorption ability, and free radical scavenging ability of the dietary fiber derived from buckwheat shells are superior to that of coarse buckwheat shell powder after micropulverization.16 In addition, the antioxidant capacity of airflow comminution insoluble citrus residue to free phenol was higher than that of mechanical grinding.17 Reducing the particle size of insoluble dietary fiber using different micronization technologies may affect the crushing efficiency, physical and chemical properties (such as water retention capacity, swelling power (SP), oil retention capacity, and cation exchange capacity), functional characteristics (such as glucose adsorption capacity, and amylase), and inhibitory activity.18,19 However, the methods currently used for the superfine grinding of TBB are too simple and lack innovation. This study employs three different superfine grinding methods, including shear crushing, airflow comminution, and wet grinding, to improve the taste, utilization, and value of TBB. In addition, TBB can be further employed in food research and development after superfine grinding.
Consumers favor TBB due to its excellent health benefits. However, although the physicochemical properties and antioxidant activity of TBB dietary fiber have been explored, few studies have examined the impact of different superfine grinding processes. Therefore, this study employs three different grinding methods for TBB powder preparation to simultaneously determine these components and provide a basis for the related research, popularization, and application.
2. Materials and methods
2.1. Materials and chemicals
The Tartary buckwheat (Chuanqiao No. 2) was purchased from Liangshan Prefecture, China, while the TBB was provided and processed by the Huantai Biotechnology Co., Ltd. (Chengdu, China). The rutin standard, gallic acid standard (chromatography grade, purity ≥ 98%), Folin-phenol reagent, and DPPH were purchased from the Shanghai Yuanye Biotechnology Co., Ltd. (Shanghai, China). The copper sulfate (CuSO4), potassium sulfate (K2SO4), boric acid (H3BO3), sulfuric acid (H2SO4), ethanol, petroleum ether, hydrogen peroxide (H2O2), phosphate, methanol (analytical grade), and methyl red were obtained from the Chengdu Kelong Chemical Co., Ltd. (Chengdu, China). The sodium hydroxide (NaOH), ferrous chloride (FeCl2), and salicylic acid were purchased from the Tianjin Zhiyuan Chemical Reagent Co., Ltd. (Tianjin, China), while the aluminum trichloride (AlCl3) and potassium acetate were acquired from the Guangdong Guanghua Technology Co., Ltd. (Shantou, China).
2.2. Preparation of the TBB powder
The TBB was crushed into powder using three superfine grinding methods, including shear crushing, airflow comminution, and wet grinding. The TBB powder was dried with hot air at 40 °C for 1 h, while uncrushed Tartary buckwheat was used as a blank control (BC). Using a high-speed pulverizer (ZN-200A, Zhongnan Pharmaceutical Machinery Factory, Changsha, China), the dry TBB powder was ground for 5 min at a roll speed of 25
000 rpm to obtain shear-crushing bran powder (SCBP).
The TBB was passed through a 100-mesh sieve and ground at a feed pressure of 0.55 MPa and crushing pressure of 1.10 MPa using a superfine airflow pulverizer (YQ50-1, Shanghai Saishan Powder Machinery Manufacturing Co., Ltd, Shanghai, China) to obtain airflow-comminution bran powder (ACBP). Water was added to the SCBP after it was passed through a 100-mesh sieve to create a 10% mixture, which was ground for 1 h using a wet-grinding machine (SNM03, Sunller Machinery Equipment Co., Ltd, Shanghai, China) containing a 600.00 g zirconia ball (0.60 mm diameter). The grinding process occurred at a circulating water temperature was 8 °C, a peristaltic pump speed of 120 rpm, and a wet-grinding machine speed of 2600 rpm. Next, the powder was prepared using a spray dryer (SD-1000, Tokyo Rikakikai Co., Ltd, Tokyo, Japan), with an inlet temperature of 164 °C, a feed liquid concentration of 5.85%, a cleaning interval of 10 min, a wind speed of 0.43 m3 min−1, an injection flow of 620 mL h−1, and a pressure of 0.18 MPa, to obtain the wet-grinding bran powder (WGBP). All the powder samples were stored in a cool, dry, closed environment for later use.
2.3. Scanning electron microscopy (SEM) observation and particle size
The TBB powder morphology was observed using SEM (JSM-7500F, JEOL, Tokyo, Japan) by modifying the method described by Wang et al.20 and Li et al.21 Appropriate sample amounts were evenly distributed on the conductive adhesive. First, the SEM stage was sprayed with gold, after which the samples were added. The sample morphology was observed at an operating voltage of 15.00 kV, while clear, typical particles were selected for the images. The particle sizes of the TBB powder were determined using a dry–wet dual-purpose laser particle size analyzer (JL-6000, Chengdu Jingxin Powder Testing Equipment Co., Ltd, Chengdu, China) in dry mode.
2.4. Color, fluidity, and filling of TBB
Before performing the measurements, the surface of the TBB was smoothed, and the L*, a*, and b* values of each sample group were measured using a colorimeter (WF32, Hangzhou Caipu Technology Co., Ltd, Hangzhou, China). L* represented the difference in brightness. a* denoted the difference between red and green, where red represented a positive value and green represented a negative value. b* signified the difference between yellow and blue, with yellow denoting a positive value and blue denoting a negative value.
The angle of repose and slip angle were measured according to a method described by Zhao et al.22 with minor modifications. The funnel was fixed at a certain height above the coordinate paper, and a 10.00 g test sample of TBB powder was collected. The repose angle tan
θ was calculated by adding TBB powder from the funnel until a cone was formed with its top making contact with the bottom of the funnel. The radius R and height H of the cone were measured, while the H to R ratio was used as the tangent value. Average values were obtained by repeating the experiments five times for each sample.
Eqn (1) was used to calculate the angle of repose.
|
 | (1) |
where
H was the cone height, cm and
R was the cone radius, cm.
A 3.00 g sample was accurately weighed and laid flat in the middle of a smooth glass plate, the one end of which was gradually pushed upward. Next, the plate was tilted to about 90% TBB powder, and angle measurement between the plate and the horizontal surface represented the slip angle. Average values were obtained by repeating the experiment five times for each sample.
The bulk density and tap density are typically used to express the filling properties of a powder. According to a method outlined by Zhao et al.23 and appropriately modified, a 10 mL measuring cylinder (mass W1) was filled with powder to the scale line, then weighed (W2), and measured three times in parallel to obtain the average value. The bulk density of the powder was calculated according to the following formula (eqn (2)):
|
 | (2) |
The measuring cylinder containing a certain volume of powder was vibrated 100 times at a specific amplitude until changes were no longer evident in the powder volume. The volume (V) was recorded after vibration, while the measurement was repeated three times to obtain an average value. The calculation formula (eqn (3)) for the tap density was as follows:
|
 | (3) |
2.5. Determination of protein content
The protein of TBB powder was determined using Automatic Kjeldahl nitrogen analyzer (K1100, Shandong Hanon Scientific Instrument Co., Ltd, Jinan, China) according to Kjeldahl method. 0.20–2.00 g sample (accurate to 0.001 g) was weighted into the digestion tube, and then 0.40 g CuSO4, 6.00 g K2SO4 and 20 mL H2SO4 were added to the digestion furnace. When the temperature of the digestion furnace reached 420 °C, continued to digest for 1 h, then the liquid in the digestion tube was green and transparent, taken out and cooled, 50 mL water was added, and the process of automatic liquid addition, distillation, titration and recording titration data was realized on the Automatic Kjeldahl nitrogen analyzer (with NaOH solution, H2SO4 standard solution and H3BO3 solution containing mixed indicator A or B before use).
Eqn (4) was used to calculate the protein content.
|
 | (4) |
where
X was the protein content in the sample,
V1 was the volume of H
2SO
4 standard titrant consumed by test solution (mL),
V2 denoted the volume of H
2SO
4 standard titrant consumed by reagent blank (mL),
c signified the H
2SO
4 standard titration solution concentration (mol L
−1), 0.0140 was the mass of nitrogen equivalent to 1.0 mL H
2SO
4 [
c(½H
2SO
4) = 1.000 mol L
−1] standard titration solution (g),
m represented the mass of sample (g),
V3 denoted the volume of digestive liquid absorbed (mL), and 6.31 was the conversion coefficient of nitrogen to protein.
2.6. Water-holding capacity, oil-holding capacity, and gel hydration properties
The TBB water-holding capacity and oil-holding capacity were determined using a method reported by Zhao et al.22 with some modifications. The water-holding capacity of the TBB powder was measured as follows: a 1.00 g sample of the dry powder with different particle sizes was accurately weighed and placed in a 100 mL beaker. Then, 50 mL of distilled water was added, after which the mixture was stirred magnetically at a constant temperature for 30 min and centrifuged at 3000 rpm for 20 min. Next, the residue was weighed after removing the upper water layer.
The oil-holding capacity of the TBB powder was determined as follows: a 3.00 g powder sample was accurately weighed and placed in a dry 50 mL centrifuge tube. Then, 24 mL of peanut oil was added and mixed via electromagnetic stirring for 30 min. The mixture was removed and centrifuged at 3500 rpm for 20 min, after which the supernatant was discarded. The oil and water attached to the inner and outer walls of the centrifuge tube were wiped dry, and the precipitation mass was weighed. Average values were obtained after repeating the experiments five times for each sample. The water-holding capacity and oil-holding capacity were calculated according to the following formula (eqn (5)):
|
 | (5) |
where
m1 was the sample weight and
m2 was the residue weight.
The water absorption index (WAI), water solubility index (WSI), and SP of the TBB were investigated using a method described by Yu et al.24 with some modifications. The TBB powder (1 g) was stirred evenly in 20 mL distilled water, placed in a centrifuge tube, and immersed in a water bath at a constant temperature of 90 °C for 10 min. The heated paste was cooled in ice water for 10 min, and then freezing centrifuged at 3000 × g at 4 °C for 10 min. The supernatant was taken out and poured into the aluminum box which was pre-dried to constant weight. The supernatant was evaporated at 105 °C until reaching a constant weight to obtain a dry solid. The residue (Wr) and dried supernatant (Ws) were weighed, and the test was repeated three times for each sample. The calculation formulas for the WAI (eqn (6)), WSI (eqn (7)), and SP (eqn (8)) were as follows:
|
WSI (g 100 g−1) = Ws/Wi × 100
| (7) |
|
SP (g g−1) = Wr/(Wi − Ws)
| (8) |
where
Wi denoted the sample weight (g).
2.7. Determination of the rutin content, quercetin content, TFC, and TPC
The rutin and quercetin content in the TBB was evaluated using High Performance Liquid Chromatography (HPLC) (Waters 2685-2489, Hangzhou Ruixi Technology Co., Ltd, Hangzhou, China) by appropriately modifying the method described by Bai et al.25 The rutin (5.00 mg) and quercetin standards (5.00 mg) were separately dissolved with methanol and diluted to 10 mL to obtain a standard solution at a concentration of 0.5 mg mL−1. The two standard solutions (1 mL each) were mixed at equal volumes to obtain a 0.25 mg mL−1 mixed standard solution, which was then filtered using a 0.45 μm microporous membrane. A C18 column (4.6 mm × 200 mm, 5 μm) was used for the analysis. The mobile phase contained a mixture of buffer solution (0.4% phosphoric acid) and methanol (50
:
50, v/v) and was subjected to 0.45 μm membrane filtration and ultrasonic degassing. HPLC analysis was performed at 30 °C at a flow rate of 1.0 mL min−1, while the column liquid was monitored at 360 nm.
The TFC of the TBB was determined according to a method delineated by Li et al.26 with some modifications. The flavonoid concentrate (8 mg) was dissolved in 50 mL of 70% ethanol to obtain the extract. Next, 1 mL of the extract was treated with 2 mL of AlCl3 (0.1 M), 3 mL of potassium acetate (1.0 M), and 4 mL of 70% ethanol to determine the TFC in the TBB. After 30 min, the absorbance of the mixture was measured at 420 nm, and the TFC (the mass fraction of rutin) was expressed by the standard calibration curve of rutin.
The TPC of the TBB was determined using a method described by Guo et al.27 After the extract or control (125 μL) was mixed evenly with deionized water (500 μL), a Folin-phenol reagent (125 μL) was added, after which the mixture was left at room temperature for 6 min. Then, 7% sodium carbonate aqueous solution (1.25 mL) was added, and the volume was adjusted to 3.0 mL with deionized water. After reacting in a dark environment for 90 min, the absorbance was determined at 760 nm using a Ultraviolet (UV)-visible spectrophotometer (UV-3600, Shimadzu, Japan). The TPC of the sample was expressed as g (gallic acid) eq. 100 g−1 dry weight (DW).
2.8. Determination of the DPPH, ˙OH, and ABTS+ scavenging effect
The DPPH scavenging activity was determined using a method reported by Verardo et al.28 with minor modifications. The extract (0.1 mL) was added to 2.9 mL of a 100 mM DPPH methanol solution. The absorbance was measured at 517 nm after 30 min (at 25 °C).
The ˙OH scavenging effect of TBB was determined by appropriately modifying the technique described by Wang et al.20 The ˙OH scavenging rate was obtained using the salicylic acid capture hydroxyl radical method. Here, 1 mL of 6 mM FeSO4 and 1 mL of 8 mM salicylic acid were added to 4 mL of the measurement solution. The reaction was initiated by adding 1 mL of 24 mM H2O2 and placing the mixture in a water bath at 37 °C for 20 min. The absorbance was measured at 510 nm after centrifugation at 3800 rpm for 10 min. Distilled water was used instead of H2O2 as the blank, and instead of extract as the control.
Eqn (9) was used to calculate the ˙OH scavenging rate.
|
 | (9) |
where
A0 denoted the absorbance when distilled water was used instead of the sample solution as a BC.
A1 signified the absorbance of the sample solution at the measurement wavelength, and
A2 represented the background absorbance value of the sample solution when distilled water was used instead of H
2O
2.
The ABTS+ of the TBB powder was evaluated by slightly modifying the method outlined by Sun et al.29 First, 7 mM of the ABTS+ solution and 2.45 mM potassium persulfate were mixed in equal quantities. The mixture was left in the dark at room temperature for 12 h to generate ABTS+ free radicals, obtaining the ABTS+ free radical mother solution. Before the test, the ABTS+ free radical mother solution was diluted with methanol to prepare the ABTS+ free radical working solution. Then, 0.3 mL of the sample and 2.7 mL of the ABTS+ radical working solution were mixed and incubated at 30 °C for 6 min, and the absorbance value As was measured at 745 nm and 30 °C after incubation. Under the same conditions, the absorbance value Ac of the mixed solution of 0.3 mL of methanol and 2.7 mL of ABTS+ free radical was taken as the control. The scavenging capacity of each sample for ABTS+ radicals was calculated using the following equation (eqn (10)):
|
 | (10) |
2.9. Statistical analysis
Excel 2010 and SPSS 20.0 software (SPSS, Inc., Chicago, IL) were used for data analysis in this study, while the graphs were created using OriginPro 8.5. All sample analyses were repeated three times, and the results were expressed as mean ± standard deviation. Analysis of Variance (ANOVA)-Dunken was used to analyze the significant data differences. Different letters indicated significant differences (p < 0.05), while the same letters indicated non-significant differences (p > 0.05).
3. Results and discussion
3.1. SEM observation and particle size analysis
SEM was used to observe the BC and TBB subjected to different crushing methods, and the images are shown in Fig. 2. The microscopic morphology and distribution of the different samples varied significantly. The particles in the BC and SCBP appeared lumpy, with uneven particle sizes and diverse structures (Fig. 2A and B), while those in the ACBP and the WGBP were smaller and more uniform (Fig. 2C and D). The particles in the WGBP were polygonal, spherical, or irregular in shape, and the particle structures were relatively complete (Fig. 2D). These results were consistent with those obtained by Tong et al.,30 who examined dry and wet rice flour morphology. And the agglomeration phenomenon has appeared in WGBP. Three different methods were used to crush the TBB powder. According to Table 1, obvious differences were evident between the grain sizes of the BC and superfine crushed bran. The particle size distribution is expressed as the surface area weighted average diameter (D3,2), the volume median diameter (D4,3), the average particle size (D50), the equivalent diameter at a 10% cumulative volume (D10) and equivalent diameter, and the cumulative volume at 90% (D90). The variation trends in the D3,2, D4,3, D10, and D90 particle size parameters were similar to that of D50. The D50 values of SCBP, ACBP, and WGBP were 17.741 μm, 17.883 μm, and 1.644 μm, respectively, all reaching the superfine powder range (10–25 μm). Compared with the average BC particle size (26.680 μm), that of the superfine TBB powder was significantly affected by the crushing methods (p < 0.05).
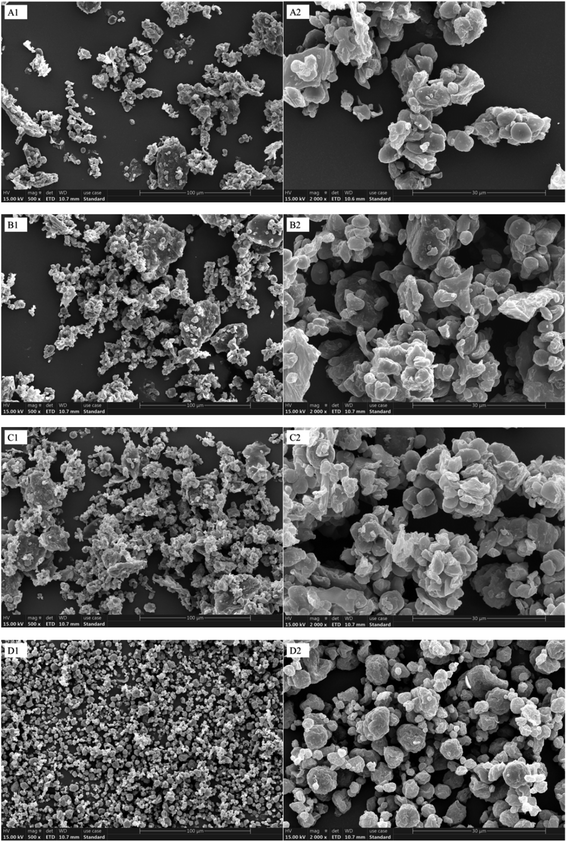 |
| Fig. 2 Morphology of TBB powder with different crushing methods observed by SEM. (A) BC bran powder showed aggregation and uneven particle sizes. (B) SCBP appeared lumpy, with uneven particle sizes and diverse structures. (C) ACBP particles were smaller and more uniform. (D) WGBP were polygonal, spherical, or irregular in shape, and the particle structures were relatively complete. | |
Table 1 Effects of different crushing methods on particle size of TBB
Particle size/μm |
BC |
SCBP |
ACBP |
WGBP |
D10 |
1.513 |
0.947 |
0.930 |
0.365 |
D50 |
26.680 |
17.741 |
17.883 |
1.644 |
D90 |
71.684 |
54.194 |
53.946 |
13.479 |
D[4,3] |
40.559 |
23.007 |
22.916 |
4.423 |
D[3,2] |
4.058 |
2.648 |
2.611 |
0.943 |
Research involving superfine ground apple powder indicated that the agglomeration became more severe in conjunction with extended grinding time, exhibiting a gradual increase in the particle size. This may be because the particle size of the powder reached a certain limit, which increased the mutual surface adsorption force, leading to intermolecular aggregation.31 The lowest D50 value was obtained via wet grinding, indicating that this technique could reduce the particle size of bran powder and produce more evenly distributed particles, which was consistent with the research results obtained by Yu et al.24 Soaking is an essential step in wet grinding. During the soaking process, the TBB becomes soft and breaks easily, reducing the grain size. This phenomenon was observed by Zhang et al.,32 who indicated that soaking caused the glutinous rice flour obtained via wet milling to exhibit exceedingly small particle sizes and a more uniform distribution. The narrower the particle size distribution range, the better the uniformity of the powder. Compared with the BC, micronization enhanced the coarse grain taste of the bran powder, improving its application in various types of food and health products. Jiang et al.33 reported that Vaccinium bracteatum Thunb leaves could be crushed into finer particles after superfine grinding, displaying excellent uniformity when mixed with other active ingredients. This can be attributed to more particles per unit weight than other coarse particles when they are finer.34 Additionally, research by Li et al.35 showed that the uniformity of soybean residue powder increased after superfine grinding while displaying improved bioavailability, adsorption performance, and solubility.
3.2. Color, fluidity, and filling properties
The color differences of the TBB powder were obtained using different crushing methods, as shown in Table 2. The ΔL value represented the difference in brightness from the BC. The maximum ΔL value of the ACBP was 48.01, which was significantly different from the other three groups (p < 0.05). The Δb value indicated the differences between the yellow and blue color levels in the tested samples and the BC. The larger the value, the higher the level of yellow. The Δb value of the WGBP was the highest (19.68), exhibiting significant differences from the SCBP (15.86) and ACBP (15.68) (p < 0.05). The fluidity of the powder was compared by measuring the angle of repose and slip. Generally, the smaller the angle of repose and slip, the better the fluidity. Fig. 3a shows the angles of repose and slips of the four types of superfine TBB powder, which were significantly different from the BC samples (p < 0.05). After shear crushing, airflow comminution, and wet grinding, the slip angles of the superfine powder increased substantially. The 37° slip angle of the SCBP increased by 5° compared to the BC (32°), while the 38.67° of ACBP increased by 6.67°, and the 42.67° of the WGBP increased by 10.67°. Furthermore, the angle of repose of the superfine powder also increased significantly. The 27.30° of the SCBP increased by 3.72° compared to the BC (23.58°), while the 29.30° of the ACBP increased by 5.72°, and the 33.06° of WGBP increased by 9.28°. Comparing bulk density and tap density could measure the importance of particles interaction in TBB powder.36 The bulk and tap densities of the bran powder obtained via different crushing methods are shown in Table 2. The bulk and tap densities of the WGBP were the most substantial at 0.34 g mL−1 and 0.53 g mL−1, respectively, exhibiting significant differences from those of the other samples (p < 0.05). No significant differences were evident between the BC bulk and tap densities and those of the SCBP and ACBP.
Table 2 Effects of different crushing methods on the color parameters, filling and hydration properties of TBBa
|
BC |
SCBP |
ACBP |
WGBP |
Mean bars with different letters (a–d) in the same bile salt concentration at different incubation times differ significantly (p < 0.05). |
Colour parameters |
ΔL |
39.90 ± 0.62d |
45.93 ± 0.51b |
48.01 ± 0.90a |
42.94 ± 0.30c |
Δa |
3.87 ± 0.02b |
5.00 ± 0.02a |
4.88 ± 0.09a |
4.96 ± 0.01a |
Δb |
14.10 ± 0.01c |
15.86 ± 0.08b |
15.68 ± 0.07b |
19.68 ± 0.14a |
ΔE |
— |
6.38 ± 0.56b |
8.35 ± 0.50a |
7.48 ± 0.49b |
Filling |
Bulk density (g mL−1) |
0.26 ± 0.01b |
0.27 ± 0.00b |
0.24 ± 0.01c |
0.34 ± 0.01a |
Tap density (g mL−1) |
0.48 ± 0.01b |
0.45 ± 0.00bc |
0.47 ± 0.01b |
0.53 ± 0.02a |
Hydration |
WAI (g g−1) |
5.317 ± 0.050a |
5.036 ± 0.027b |
5.149 ± 0.143ab |
4.275 ± 0.106c |
WSI (g 100 g−1) |
15.058 ± 0.129b |
15.280 ± 0.162b |
14.284 ± 0.203c |
21.077 ± 0.101a |
SP (g g−1) |
6.259 ± 0.061a |
5.944 ± 0.020b |
6.007 ± 0.181ab |
5.147 ± 0.141c |
 |
| Fig. 3 Effects of different crushing methods on the fluidity (a), water-holding capacity, and oil-holding capacity (b) of TBB. Mean bars with different letters (a–d) in the same bile salt concentration at different incubation times differ significantly (p < 0.05). | |
The brightness of the ACBP was the most substantial, which was consistent with the experimental conclusion of He et al.37 The brightness of the powder was negatively correlated with the particle size. Therefore, smaller particles increase the relative surface area, enhancing the reflective effect and the brightness value. Jiang et al.33 confirmed that the particle sizes of Vaccinium bracteatum Thunb leaf powder were small while displaying a dark, reddish and yellowish color. Due to the contact between bran and water during the wet grinding process, some slightly soluble flavonoids in water were dissolved. These compounds adhered to the particle surface after drying, reducing the brightness of the wet ground powder and significantly increasing the yellowness value. Improving the color, which is a vital sensory index, increases the commercial value of products. In addition, protein content, particle size, and ash content significantly affect the brightness and yellowness of bran powder. The grinding degree of grain varies in conjunction with different grinding instruments, impacting the material composition of the final powder. The color of the powder substantially influences the color of the subsequently processed products. Therefore, ACBP might be more readily accepted by consumers since it displayed the highest level of brightness.37 A particle size decrease gradually increased the angle of repose and slip of the bran powder. This could be attributed to higher surface adhesion and adsorption properties of the superfine powder particles, as well as the decreased fluidity caused by their adsorption and agglomeration characteristics.38 These findings are consistent with the observations of Hong et al.,39 who reported that powder fluidity typically declines in conjunction with decreased particle size due to polymer formation.40 Contrarily, Wang41 used airflow comminution for the superfine grinding of Lentinus edodes powder processed via ordinary crushing. The results showed that the angle of repose declined in conjunction with a decrease in the particle sizes of the Lentinus edodes powder, indicating that higher fluidity was achieved after superfine grinding. This may be ascribed to the fact that the surface of the Lentinus edodes powder is smooth and spherical after airflow comminution, limiting agglomeration. Bulk density can be used in the food industry as an essential parameter for evaluating powder quality.42 For example, during the preparation of sheet or capsule products, high bulk density is conducive to product formation,43 which may be attributed to the fact that tap density is closely related to the specific surface area of the powder. The specific surface area increased in conjunction with a decrease in the particle size of the powder, increasing the tap density. Smaller particle sizes increased the homogeneity of the powder, providing a larger contact area with the surrounding particle environment, resulting in smaller gaps between the particles and a higher tap density.41 These findings were consistent with the observations of Zhang et al.,43 who reported that the superfine Lycium ruthenicum Murray powder displayed the most significant bulk density and smaller particle sizes than the other two powders. At the same volume as the superfine treatment, smaller particle sizes decreased the particle gaps, increasing the density and mass, as well as the bulk and tap densities, rendering the product more conducive to human absorption. The bulk and tap densities of the powder depend primarily on the distribution, shapes, sizes, and adhesion patterns of the particles.11
3.3. Protein content
Tartary buckwheat protein contains eight essential amino acids, which could be used as one of the main biologically active substances. TBB is rich in plant protein, while protein could be described as one of the important compounds in food research and development. The protein content of TBB processed by different crushing methods was shown in Fig. 4. As shown in Fig. 4, there was no significant difference on the protein content of SCBP and ACBP (p > 0.05). With the decrease of particle size, the protein content of the WGBP was the highest (23.60%), exhibiting significant differences from the BC (20.19%), SCBP (20.19%) and ACBP (21.79%) (p < 0.05).
 |
| Fig. 4 Effects of different crushing methods on protein content. Mean bars with different letters (a–d) in the same bile salt concentration at different incubation times differ significantly (p < 0.05). | |
With the increase of the grinding time and degree, the particle size of TBB decreases, the large particles were basically destroyed, and the sample was in contact with the solvent more fully, resulting in the increase of the protein content of WGBP. These results were consistent with those obtained by Li et al.,44who found the protein-bound aggregates are fully separated into free state during the superfine grinding process, which promoted the increase of protein dissolution rate. Additionally, some studies have shown that plant protein can be used as a source of bioactive peptides, which can exert direct biological activity after consumption. Bioactive peptides are amino acid sequences that exert physiological functions in the body after digestion in vivo or hydrolysis or release in vitro.1 Moughan et al.45 discovered that bioactive peptides have certain antioxidant properties, therefore, the antioxidant activity of TBB may be related to the protein content. Moreover, Ma et al.46 reported that the scavenging ability of buckwheat protein to free radicals was enhanced after digestion in vitro.
3.4. Water/oil-holding capacity and hydration properties
The water-holding capacity reflects the binding ability between the sample and water and is significant when the mass of the sample per unit mass increases. Furthermore, the water-holding capacity of particles with different sizes changes due to distinct property modifications.38 The oil-holding capacity refers to the ability of a material to absorb oil per unit mass and substantially impacts the oil addition and mixing effect during raw material processing. Fig. 3b shows that shear crushing and airflow comminution had little influence on the water-holding capacity and oil-holding capacity of the bran. The water-holding capacity of the sample after shear crushing decreased from 3.15 g g−1 of BC to 2.76 g g−1, and decreased to 2.68 g g−1 of ACBP, with no significant difference (p > 0.05). The 4.38 g g−1 water-holding capacity value of the WGBP increased by 1.23 g g−1 compared to the BC. After superfine grinding, the oil-holding capacity of TBB decreased from 1.00 g g−1 to 0.84 g g−1 in the WGBP. The solubility and SP are vital indexes that reflect the processing quality of the TBB powder since they determine the SP and the loss of soluble solids during the cooking process of Tartary buckwheat products. According to Table 2, the water absorption, water solubility, and SP of the WGBP were significantly different from those of the other groups (p < 0.05), at 4.275 g g−1, 21.077 g 100 g−1, and 5.147 g g−1, respectively. Moreover, the BC, SCBP, and ACBP displayed no significant effect on the hydration properties (p > 0.05).
The decrease in the water-holding capacity of the SCBP and ACBP may be due to the dissolution of soluble substances caused by the cell breakage in the powder, slightly weakening the water-holding capacity. However, the water-holding capacity of the WGBP increased significantly, which was consistent with the experimental results obtained by Chen et al.31 This may be ascribed to the fact that grinding reduces the volume density while increasing the gaps and liquid holding space, significantly improving the water-holding capacity. Zhu et al.47 indicated that the water-holding capacity of powder derived from grape dreg dietary fiber doubled after superfine grinding. Moreover, the SP, oil-holding capacity, and adsorption capacity of NO2− increased 1.5 times after superfine grinding. Due to the high dietary fiber content in the TBB, the oil-holding capacity decreased slightly in conjunction with a decline in the average particle size. The long-chain structure of the dietary fiber is broken during the superfine grinding process, increasing the short-chain and changing the dietary fiber composition, which reduces the dietary fiber on water and oil. Compared with other treatment groups, the WGBP exhibited the lowest water absorption and SP, while its water solubility increased by nearly 40% compared to the BC. This may be ascribed to the fact that superfine grinding reduces the particle diameter of the bran and destroys the microstructure of the sample, affecting the hydration and SP of the material.25
3.5. Rutin content, quercetin content, TFC, and TPC
Flavonoids can be used to evaluate the antioxidant activity of TBB. Therefore, the content of rutin and quercetin content in the TBB was determined to identify the TBB flavonoids. Table 3 indicates that the rutin and quercetin content changed substantially in all the samples after superfine grinding. The rutin content in the TBB powder was significantly affected by superfine grinding (p < 0.05), decreasing by 18.97% in the SCBP and by 21.28% in the ACBP, while no rutin was detected in the WGBP. The quercetin content was higher in the SCBP and WGBP than in the BC. The effect of superfine grinding on the TFC in TBB powder is shown in Fig. 5a. The TFC in the bran powder decreased after superfine grinding and differed significantly between the WGBP and the other treatment groups (p < 0.05). The TFC in the samples was minimally affected by the three types of superfine grinding treatments, while it was highest in the BC at 9.50 g 100 g−1. Although the TFC level in WGBP was the lowest (8.69 g 100 g−1), it decreased by 8.53% compared to the BC. Phenolic compounds are bioactive ingredients found in plant products that exhibit significant health benefits while playing a crucial role in the antioxidant activity of plant materials.48 As shown in Fig. 5b, the TPC of the WGBP was slightly higher than that obtained via the other two crushing methods, and no significant differences were evident between the BC, SCBP, and ACBP (p > 0.05).
Table 3 Effects of different crushing methods on the content of rutin and quercetin, and the removal rate of DPPH, ˙OH, and ABTS+ of TBBa
|
BC |
SCBP |
ACBP |
WGBP |
Mean bars with different letters (a–d) in the same bile salt concentration at different incubation times differ significantly (p < 0.05). |
Rutin |
3.90 ± 0.07a |
3.16 ± 0.13b |
2.68 ± 0.37b |
— |
Quercetin |
1.03 ± 0.04c |
1.44 ± 0.06b |
0.97 ± 0.04c |
2.18 ± 0.03a |
DPPH scavenging rate/% |
63.73 ± 0.60a |
62.84 ± 0.69a |
64.07 ± 1.28a |
60.74 ± 0.54b |
˙OH scavenging rate/% |
26.75 ± 0.82d |
42.57 ± 0.47b |
34.54 ± 0.94c |
86.62 ± 0.22a |
ABTS+ scavenging rate/% |
89.73 ± 0.23c |
90.16 ± 0.33c |
90.80 ± 0.14b |
92.98 ± 0.06a |
 |
| Fig. 5 Effects of different crushing methods on TFC (a), and TPC (b) of TBB. Mean bars with different letters (a–d) in the same bile salt concentration at different incubation times differ significantly (p < 0.05). | |
No rutin was detected in the WGBP. This may be due to the conversion of rutin to quercetin during processing since the quercetin content doubled in the WGBP. Two rutin-degrading enzymes were also found in the Tartary buckwheat. They are responsible for rutin degradation in aqueous solutions, mainly producing quercetin and a small amount of rutin.49 These results showed that the rutin content of the TBB was affected by the grinding degree. Compared with the dry grinding method, the wet grinding technique significantly reduced the rutin content of bran, which was consistent with the experimental results obtained by Yu et al.24 The amount of rutin hydrolysate in buckwheat bran is related to the particle size and hydrolysis conditions. Zheng49 found that due to the smaller particle size and increased uniformity of the superfine grinding TBB powder, rutin degrading enzyme can more fully contact with water, so that all of the rutin hydrolyzed, and its degradation to quercetin content increased slightly with decreasing particle size. Some of the flavonoids in the bran powder dissolved in the water during the wet grinding process, resulting in a loss of TFC. Zheng et al.50 used a planetary ball mill to dry crush buckwheat bran and found that the extraction rate of the flavonoids increased after superfine grinding due to decreased average particle size and higher powder uniformity. This could be attributed to the fact that contact between the flavonoid substances and the extraction solvent increased during the extraction process. The bran was subjected to various combined forces for extended periods during grinding, resulting in a slight TFC decrease in both the SCBP and ACBP compared with the BC. A study by Cai et al.51 regarding the effect of superfine grinding on buckwheat polyphenols and antioxidant activity showed that grinding common buckwheat powder using an airflow crusher first decreased the free flavonoid and TFC content, followed by an increase in conjunction with the degree of micropulverization, although the decreasing range was small. This may be ascribed to the destruction of the flavonoid structure by the combined forces during the crushing process, resulting in loss. However, the particle sizes of TBB powder decreased, while the uniformity and the surface area making contact with the extraction solvent increased, elevating the extraction rate of the buckwheat powder flavonoids. The smaller WGBP particle sizes increased the area making contact with the extraction reagent, slightly increasing the TPC. Polyphenols belong to a heterogeneous group and exhibit various biological benefits, such as anti-inflammatory, antibacterial, and antioxidant properties.52 The TPC of common buckwheat, as well as Tartary buckwheat hulls and bran, are reportedly higher than in flour. Furthermore, the TPC of TBB is higher than that of common buckwheat.53 In addition, Zielinska et al.54 used buckwheat hulls to produce tea, which was tested for TPC and TFC, antioxidant activity, and anti-glycation activity. The results showed that the TPC (3.56 ± 0.19 mg g−1) extracted from the dried buckwheat hulls with 80% methanol was 64 times lower than green tea. The temperature of the water and soaking time may also affect the TPC content in tea.
3.6. DPPH, ˙OH, and ABTS+ scavenging effect
TFC and TPC do not necessarily indicate the level of antioxidant activity in plants. Therefore, the antioxidant activity of each phenolic compound must be considered quantitatively.55 Table 3 shows the ability of coarse TBB powder and three kinds of TBB micro-powders to scavenge DPPH, ˙OH, and ABTS+ during this experiment, indicating a certain level of antioxidant activity. DPPH is a stable free radical in organic solvents that are commonly used to evaluate the ability of antioxidants to scavenge free radicals. The DPPH scavenging ability of the bran powder extracts exposed to three types of superfine grinding is shown in Table 3. The DPPH clearance rate of the WGBP was the lowest (60.74%), displaying significant differences from the other groups (p < 0.05), while no significant differences (p > 0.05) were apparent between the other three samples. The DPPH clearance rates of the BC, SCBP, and ACBP were 63.73%, 62.84%, and 64.07%, respectively. After superfine grinding, the ability of the TBB powder to scavenge ˙OH increased. The ability of the WGBP to remove ˙OH (86.62%) was significantly (p < 0.05) higher than that of the BC (26.57%), SCBP (42.57%), and ACBP (34.54%). According to Table 3, each TBB powder exhibited the ability to scavenge ABTS+ free radicals, indicating a specific degree of anti-oxidation. After superfine grinding, the ABTS+ removal ability of TBB increased, while that of the WGBP (92.98%) was significantly (p < 0.05) higher than the BC (89.73%), SCBP (90.16%), and ACBP (90.80%). The ABTS+ removal rate of the three superfine powders was higher than the BC.
These results demonstrated that superfine grinding treatment could effectively improve the scavenging of DPPH, ˙OH, and ABTS+ of TBB. Li et al.56 found that buckwheat bran powder displayed a higher DPPH removal ability than refined buckwheat powder. The TPC and TFC levels in the outer layer of buckwheat grains were higher than in the inner layer, while the DPPH clearance level was mostly determined by the rutin content and TPC in the extract.57 In addition, the phenolic compounds in bran extract can provide electrons and proton hydrogen to react with ˙OH. The rutin and quercetin in the buckwheat extract contain O-dihydroxyl groups on the B-ring structure, providing proton hydrogen and electrons to react with hydroxyl free radicals to facilitate free radical scavenging.20,58 The varying ˙OH scavenging ability of different buckwheat powder extracts is related to the different TPC and TFC levels. He et al. believed that the differences in ˙OH scavenging ability were not related to the rutin content but rather the TPC in buckwheat extract.58 The ABTS+ clearance rate of the samples exposed to superfine grinding was higher than the BC, indicating that superfine grinding could effectively improve the antioxidant activity of TBB. This may be attributed to the fact that superfine grinding effectively promotes the dissolution of active antioxidant ingredients in bran, enhancing its antioxidant activity.59
4. Conclusions
This study evaluates the influence of three superfine grinding methods on the physicochemical and antioxidant properties of TBB powder. SEM and particle size results show that the particle structure of the WGBP was more complete while exhibiting the smallest average particle size. The WGBP exhibits the highest protein content, superior water-holding capacity and solubility to the SCBP and ACBP while also displaying the highest bulk and tap densities. The SCBP displays the highest brightness level of all the samples. Furthermore, the quercetin content of the WGBP far exceeds the other samples, while no rutin is detected. In addition, the TFC and TPC levels of WGBP are significantly different from the other groups, while the ˙OH clearance rate is substantially higher than other treatment groups. The SCBP, ACBP, and BC exhibit no significant TFC, TPC, and antioxidant capacity differences. This study provides a theoretical reference for the development of SCBP, ACBP, and WGBP products in the food industry. Moreover, the role of peptides and other polyphenols in the antioxidant activity of TBB, and the effects of superfine grinding on the microstructure of TBB particles need to be further investigated.
Conflicts of interest
There are no conflicts to declare.
Acknowledgements
This work was supported by Science and Technology Support Program of Sichuan [2018NZ0097 and 19ZDYF1593], and Graduate student innovation fund of Xihua University [ycjj2018055].
References
- D. Morales, M. Miguel and M. Garces-Rimon, Pseudocereals: a novel source of biologically active peptides, Crit. Rev. Food Sci. Nutr., 2021, 61, 1537–1544 CrossRef CAS.
- L. J. Lv, Y. Xia, D. Z. Zou, H. R. Han, Y. L. Wang, H. Y. Fang and M. H. Li, Fagopyrum tataricum (L.) Gaertn.: A Review on its Traditional Uses, Phytochemical and Pharmacology, Food Sci. Technol. Res., 2017, 23, 1–7 CrossRef CAS.
- Y. J. Cho and S. Lee, Extraction of rutin from Tartary buckwheat milling fractions and evaluation of its thermal stability in an instant fried noodle system, Food Chem., 2015, 176, 40–44 CrossRef CAS PubMed.
- Y. J. Cho, I. Y. Bae, G. E. Inglett and S. Lee, Utilization of tartary buckwheat bran as a source of rutin and its effect on the rheological and antioxidant properties of wheat-based products, Ind. Crops Prod., 2014, 61, 211–216 CrossRef CAS.
- X. N. Guo, H. Y. Yao and Z. X. Chen, Effect of heat, rutin and disulfide bond reduction on in vitro pepsin digestibility of Chinese tartary buckwheat protein fractions, Food Chem., 2007, 102, 118–122 CrossRef CAS.
- X. D. Cui and Z. H. Wang, Preparation and properties of rutin-hydrolyzing enzyme from tartary buckwheat seeds, Food Chem., 2012, 132, 60–66 CrossRef CAS.
- M. C. Herrera and M. D. L. D. Castro, Ultrasound-assisted extraction for the analysis of phenolic compounds in strawberries, Anal. Bioanal. Chem., 2004, 379, 1106–1112 CrossRef CAS.
- G. E. Inglett, D. Chen, M. Berhow and S. Lee, Antioxidant activity of commercial buckwheat flours and their free and bound phenolic compositions, Food Chem., 2011, 125, 923–929 CrossRef CAS.
- F. H. Li, X. L. Zhang, S. J. Zheng, K. K. Lu, G. H. Zhao and J. Ming, The composition, antioxidant and antiproliferative capacities of phenolic compounds extracted from tartary buckwheat bran [Fagopyrum tartaricum (L.) Gaerth], J. Funct. Foods, 2016, 22, 145–155 CrossRef CAS.
- R. H. Ge and H. Wang, Nutrient components and bioactive compounds in tartary buckwheat bran and flour as affected by thermal processing, Int. J. Food Prop., 2020, 23, 127–137 CrossRef CAS.
- W. Wang, Master, Studies on the functional properties and application of superfine grinding wheat bran, Henan University of Technology, 2016 Search PubMed.
- X. Han and Z. X. Guo, Application of superfine grinding technology in grain processing, Cereal Feed Ind., 2016, 3, 13–16 Search PubMed.
- Y. Wang, Master, Study on ultrafine green tea powder applied to cosmetics and food, Zhejiang University, 2010 Search PubMed.
- J. Hou, Y. J. Dong, C. S. Liu, G. S. Gai, G. Y. Hu, Z. Y. Fan and L. L. Xu, Nutrient Release Characteristics of Coated Fertilizers by Superfine Phosphate Rock Powder and its Effects on Physiological Traits of Chinese Cabbage, J. Plant Nutr., 2014, 38, 1254–1274 CrossRef.
- M. Zhang and L. Wang, The research progress of super micron-milling in food processing, J. Wuxi Univ. Light Ind., 2003, 22, 106–110 Search PubMed.
- F. M. Zhu, B. Du, R. F. Li and J. Li, Effect of micronization technology on physicochemical and antioxidant properties of dietary fiber from buckwheat hulls, Biocatal. Agric. Biotechnol., 2014, 3, 30–34 CrossRef.
- B. B. Tao, F. Y. Ye, H. Li, Q. Hu, S. Xue and G. H. Zhao, Phenolic Profile and in Vitro Antioxidant Capacity of Insoluble Dietary Fiber Powders from Citrus (Citrus junos Sieb. ex Tanaka) Pomace as Affected by Ultrafine Grinding, J. Agric. Food Chem., 2014, 62, 7166–7173 CrossRef CAS.
- C. F. Chau, Y. T. Wang and Y. L. Wen, Different micronization methods significantly improve the functionality of carrot insoluble fibre, Food Chem., 2007, 100, 1402–1408 CrossRef CAS.
- A. Sangnark and A. Noomhorm, Effect of particle sizes on functional properties of dietary fibre prepared from sugarcane bagasse, Food Chem., 2003, 80, 221–229 CrossRef CAS.
- Y. J. Wang, Master, Quality Properties of Graded Buckwheat Flour, Northwest A&F University, 2017 Search PubMed.
- W. H. Li, F. S. Zhang, P. L. Liu, Y. F. Bai, L. Gao and Q. Shen, Effect of high hydrostatic pressure on physicochemical, thermal and morphological properties of mung bean (Vigna radiata L.) starch, J. Food Eng., 2011, 103, 388–393 CrossRef CAS.
- G. H. Zhao, M. W. Zhang, R. F. Zhang, L. Liu, Z. C. Wei and D. F. Shi, Effect of airflow ultrafine grinding on physicochemical properties of Rhodomyrtus tomentosa fruit power, Food Sci., 2016, 37, 17–21 CAS.
- X. Y. Zhao, Z. B. Yang, G. S. Gai and Y. F. Yang, Effect of superfine grinding on properties of ginger powder, J. Food Eng., 2009, 91, 217–222 CrossRef.
- D. D. Yu, J. C. Chen, J. Ma, H. X. Sun, Y. Q. Yuan, Q. Ju, Y. Z. Teng, M. Yang, W. H. Li, K. Fujita, E. Tatsumi and G. Z. Luan, Effects of different milling methods on physicochemical properties of common buckwheat flour, LWT, 2018, 92, 220–226 CrossRef CAS.
- C. Z. Bai, M. L. Feng, X. L. Hao, Q. M. Zhong, L. G. Tong and Z. H. Wang, Rutin, quercetin, and free amino acid analysis in buckwheat (Fagopyrum) seeds from different locations, Genet. Mol. Res., 2015, 14, 19040–19048 CrossRef CAS PubMed.
- B. C. Li, Y. Q. Li and Q. B. Hu, Antioxidant activity of flavonoids from tartary buckwheat bran, Toxicol. Environ. Chem., 2015, 98, 429–438 CrossRef.
- X. D. Guo, C. S. Wu, Y. J. Ma, J. Parry, Y. Y. Xu, H. Liu and M. Wang, Comparison of milling fractions of tartary buckwheat for their phenolics and antioxidant properties, Food Res. Int., 2012, 49, 53–59 CrossRef CAS.
- V. Verardo, V. Glicerina, E. Cocci, A. G. Frenich, S. Romani and M. F. Caboni, Determination of free and bound phenolic compounds and their antioxidant activity in buckwheat bread loaf, crust and crumb, LWT, 2018, 87, 217–224 CrossRef CAS.
- D. Sun, S. Q. Huang and S. B. Cai, The effects of different processing methods on the total phenolics content (TPC), flavonoids, and antioxidant activities of tartary buckwheat, Food Ferment. Ind., 2016, 42, 141–147 CAS.
- L. T. Tong, X. X. Gao, L. Z. Lin, Y. J. Liu, K. Zhong, L. Y. Liu, X. R. Zhou, L. Wang and S. M. Zhou, Effects of semidry flour milling on the quality attributes of rice flour and rice noodles in China, J. Cereal Sci., 2015, 62, 45–49 CrossRef CAS.
- R. Chen and L. He, Effect of superfine grinding on physicochemical properties of apple powder, Food Sci., 2017, 38, 150–154 Search PubMed.
- H. Zhang, F. F. Wu, D. Xu and X. M. Xu, Effects of milling methods on the properties of glutinous rice flour and sweet dumplings, J. Food Sci. Technol., 2021, 58, 1848–1857 CrossRef CAS.
- L. Jiang, Q. X. Xu, M. Qiao, F. F. Ma, K. Thakur and Z. J. Wei, Effect of superfine grinding on properties of Vaccinium bracteatum Thunb leaves powder, Food Sci. Biotechnol., 2017, 26, 1571–1578 CrossRef PubMed.
- C. K. Riley, S. A. Adebayo, A. O. Wheatley and H. N. Asemota, Surface properties of yam (Dioscorea sp.) starch powders and potential for use as binders and disintegrants in drug formulations, Powder Technol., 2008, 185, 280–285 CrossRef CAS.
- G. H. Li, W. Y. Guo, X. L. Gao, Y. H. Wang and S. S. Sun, Effect of superfine grinding on physicochemical and antioxidant properties of soybean residue powder, Food Sci. Nutr., 2020, 8, 1208–1214 CrossRef CAS PubMed.
- Y. Chen, B. C. Zhang, Y. H. Sun, J. G. Zhang, H. J. Sun and Z. J. Wei, Physicochemical properties and adsorption of cholesterol by okra (Abelmoschus esculentus) powder, Food Funct., 2015, 6, 3728–3736 RSC.
- C. A. He, Z. Zhang, L. J. Wang, H. Liu, M. Wang, X. T. Sun and Y. L. Li, Effect of different milling methods on characteristics and in vitro starch digestibility of tartary buckwheat flour, J. Chin. Cereals Oils Assoc., 2017, 32, 19–25 Search PubMed.
- M. H. Huang, R. H. Wu, Q. G. He, Q. G. Yang, H. Pan, E. Z. Zhang, R. B. Huang, H. J. Yang, H. Zhang and Z. L. Pan, Physical characteristics of Camellia chrysantha (Hu) Tuyama flower powder with different particle sizes, Food Sci., 2018, 39, 76–82 Search PubMed.
- S. J. Hong, P. R. Das and J. B. Eun, Effects of superfine grinding using ball-milling on the physical properties, chemical compositions, and antioxidant properties of Quercus salicina (Blume) leaf powders, J. Sci. Food Agric., 2021, 101, 3123–3131 CrossRef CAS PubMed.
- K. E. Ileleji and B. Zhou, The angle of repose of bulk corn stover particles, Powder Technol., 2008, 187, 110–118 CrossRef CAS.
- H. D. Wang, Master, Processing of edible mushroom essence seasoning with Lentinus edodes ultrafine powder and its application, Jiangnan University, 2012 Search PubMed.
- X. Huang, J. Y. Dou, D. Li and L. J. Wang, Effects of superfine grinding on properties of sugar beet pulp powders, LWT, 2018, 87, 203–209 CrossRef CAS.
- J. T. Zhang, Y. S. Dong, T. Nisar, Z. X. Fang, Z. C. Wang and Y. R. Guo, Effect of superfine-grinding on the physicochemical and antioxidant properties of Lycium ruthenicum Murray powders, Powder Technol., 2020, 372, 68–75 CrossRef CAS.
- D. M. Li, Master, Study on preparation ultra-fine integral lily powder and its physical and chemical properties, Nanchang University, 2007 Search PubMed.
- P. J. Moughan, S. M. Rutherfurd, C. A. Montoya and L. A. Dave, Food-derived bioactive peptides- a new paradigm, Nutr. Res. Rev., 2014, 27, 16–20 CrossRef CAS PubMed.
- Y. Ma, Y. L. Xiong, J. Zhai, H. Zhu and T. Dziubla, Fractionation and evaluation of radical scavenging peptides from in vitro digests of buckwheat protein, Food Chem., 2010, 118, 582–588 CrossRef CAS PubMed.
- F. M. Zhu, B. Du and J. Li, Effect of ultrafine grinding on physicochemical and antioxidant properties of dietary fiber from wine grape pomace, Food Sci. Technol. Int., 2014, 20, 55–62 CrossRef PubMed.
- S. C. Ren and J. T. Sun, Changes in phenolic content, phenylalanine ammonia-lyase (PAL) activity, and antioxidant capacity of two buckwheat sprouts in relation to germination, J. Funct. Foods, 2014, 7, 298–304 CrossRef CAS.
- H. Zheng, Master, Superfine comminution of tartary buckwheat bran and its property of micropowder, Northwest A&F University, 2007 Search PubMed.
- H. Zheng, M. Wang and D. Wu, The Effect of Super Micro-milling on Physical chemistry and Functional Properties of Tartary Buckwheat Bran, Food Ferment. Ind., 2006, 8, 5–9 Search PubMed.
- T. Cai, L. P. Wang, M. Liu, X. H. Tian, Y. X. Liu, N. N. Wu, Q. L. Lin and B. Tan, The Influence of Micronization on Polyphenols and Antioxidant Activity of Buckwheat Powder, J. Chin. Cereals Oils Assoc., 2015, 30, 95–99 CAS.
- P. Trouillasa, C. A. Callistea, D. P. Allaisc, A. Simonb, A. Marfaka, C. Delageb and J. L. Durouxa, Antioxidant, anti-inflammatory and antiproliferative properties of sixteen water plant extracts used in the Limousin countryside as herbal teas, Food Chem., 2003, 80, 399–407 CrossRef.
- C. Li, F. Xu, C. Cao, M. Y. Shang, C. Y. Zhang, J. Yu, G. X. Liu, X. Wang and S. Q. Cai, Comparative analysis of two species of Asari Radix et Rhizoma by electronic nose, headspace GC–MS and chemometrics, J. Pharm. Biomed. Anal., 2013, 85, 231–238 CrossRef CAS.
- D. Zielinska, D. Szawara-Nowak and H. Zielinski, Antioxidative and anti-glycation activity of buckwheat hull tea infusion, Int. J. Food Prop., 2012, 16, 228–239 CrossRef.
- T. Morishita, H. Yamaguchi and K. Degi, The Contribution of Polyphenols To Antioxidative Activity In Common Buckwheat and Tartary Buckwheat Grain, Plant Prod. Sci., 2015, 10, 99–104 CrossRef.
- F. H. Li, Y. Yuan, X. L. Yang, S. Y. Tao and J. Ming, Phenolic Profiles and Antioxidant Activity of Buckwheat (Fagopyrum esculentum Möench and Fagopyrum tartaricum L. Gaerth) Hulls, Brans and Flours, J. Integr. Agric., 2013, 12, 1684–1693 CrossRef.
- P. V. Hung and N. Morita, Distribution of phenolic compounds in the graded flours milled from whole buckwheat grains and their antioxidant capacities, Food Chem., 2008, 109, 325–331 CrossRef CAS PubMed.
- Y. Y. He, B. L. Feng, T. Deng, S. Q. An, J. F. Gao and Y. Chai, Antioxidant activity of ethanol extracts of different buckwheat, Acta Agric. Boreali-Occident. Sin., 2007, 6, 76–79 Search PubMed.
- X. Zhang, Master, Study on the Physicochemical and Functional Properties of Superfine Powder of Defatted Gardenia Fruit, Zhejiang A&F University, 2018 Search PubMed.
|
This journal is © The Royal Society of Chemistry 2021 |
Click here to see how this site uses Cookies. View our privacy policy here.