DOI:
10.1039/D1RA04756F
(Paper)
RSC Adv., 2021,
11, 27659-27673
Design, synthesis, biological assessment and in silico ADME prediction of new 2-(4-(methylsulfonyl) phenyl) benzimidazoles as selective cyclooxygenase-2 inhibitors†
Received
19th June 2021
, Accepted 30th July 2021
First published on 17th August 2021
Abstract
A novel series of benzimidazole derivatives wherein 4-(methylsulfonyl) phenyl pharmacophore attached via its C-2 position was designed and synthesized. These compounds were evaluated in vitro as cyclooxygenase-1(COX-1)/cyclooxygenese-2(COX-2) inhibitors. Furthermore, the synthesized compounds were also in vivo evaluated for their anti-inflammatory activity and ulcerogenic liability. Examination of histopathological lesions was also performed to evaluate the cariogenic effect of most active compounds. In silico prediction of physicochemical properties, ADME, and drug-likeness profiles were also studied. Several compounds as 11b, 11k, 12b, and 12d showed selective inhibition to (COX-2) isozyme. Compound 11b showed the most potent (COX-2) inhibitory activity with (IC50 = 0.10 μM) and selectivity index (SI = 134); the tested compounds also have shown good anti-inflammatory activity. Regarding the ulcerogenic liability, compound 11b was also safest one (Ulcer Index) (UI = 0.83). The results of the molecular docking studies is closely related to the results of the in vitro COX-2 inhibitory activities.
1. Introduction
Non-steroidal anti-inflammatory drugs (NSAIDs) are used to treat a wide range of inflammatory diseases, such as pain, fever and arthritis.1,2 NSAIDs are still the first choice for the management of such cases.3 The inflammatory process starts with the biotransformation of arachidonic acid (AA) in the cell membrane, and is then catalyzed by both cyclooxygenases (COXs) and lipoxygenase (LOX) enzyme families to produce prostaglandins (PGs) and leukotrienes (LTs), respectively.4
NSAIDS are capable of reducing the production of key pro-inflammatory mediator's prostaglandins (PGs) by the inhibition of constitutive (COX-1) and inducible (COX-2) isozymes.5 However, certain side effects are associated with their long use including bleeding, hepatotoxicity, and cardiovascular disorders. The inhibition of constitutive COX-1 is responsible for these side effects because it is considered a housekeeping enzyme, which is responsible for maintaining the integrity of gastric, renal and platelet cell functions.6
Non-selective NSAIDS usually inhibit both COX-1 and COX-2 and consequently might show all the above side effects. Indomethacin (1), as a potent non-selective NSAID, has substantial medical applications in the treatment of inflammation diseases including osteoarthritis, rheumatoid, and ankylosing spondylitis.7
Clinical trials have shown that selective COX-2 inhibitors as celecoxib have significantly better safety profiles.8–10 According to structure–activity relationship (SAR) studies, selective COX-2 inhibitors are diaryl heterocyclic compounds bearing –SO2NH2, or –SO2CH3 groups (Fig. 1). The whole COX-2 inhibitor molecule is pushed by a sterically bulky group into the hydrophobic and large in volume COX-2 enzyme.11–14
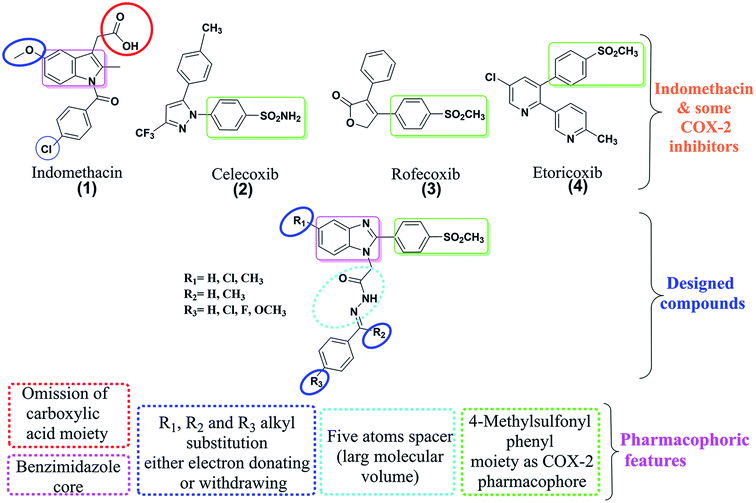 |
| Fig. 1 Chemical structures of traditional non-selective NSAIDs (indomethacin 1), some selective (COX-2) inhibitor drugs (celecoxib 2, rofecoxib 3 and etoricoxib 4) and the designed cyclooxygenase-2 (COX-2) selective inhibitors. | |
Benzimidazole derivatives are of great medicinal importance due to their wide biological activities such as anti-inflammatory,15 anticancer,16,17 antimicrobial,18–20 antiviral,21 antihypertensive,22,23 antifungal24 and antibacterial activities.25–27
Therefore, our strategy to modify indomethacin structure to get selective COX-2 inhibitor is summarized as follows,
(i) Benzimidazole core instead of the indole core.
(ii) Five atom spacers that have a large molecular volume.
(iii) 4-Methylsulfonyl phenyl moiety at position 2 replaced the methyl group of indomethacin to develop COX-2 selectivity that can bind to the hydrophobic residue of COX-2 active site.
(iv) Omission of a carboxylic acid moiety at position 3 to overcome ulcerogenic potential.
(v) R1, R2 and R3 alkyl substitution either electron-donating or withdrawing at position 5 to explore their electronic effect on anti-inflammatory activity (Fig. 1).
Herein, two series of indomethacin analogues were designed, synthesized and screened for their in vitro (COX1/2) inhibition and in vivo anti-inflammatory activities. In addition, physicochemical parameters and molecular docking studies to explore molecular patterns on the COX-2 binding site will be discussed. Ulcer index and histopathological study for stomach samples are also included.
2. Results and discussion
2.1. Chemistry
The synthesis of target compounds is depicted in Schemes 1 and 2. In the first step, benzimidazole derivatives 7a–c were obtained from the reaction of 1-2-phenylenediamine derivatives 5a–c with 4-(methylthio) benzaldehyde (6) through oxidative condensation and cyclization according to a reported procedure28 using either traditional heating or microwave irradiation. The use of microwave heating has the advantages of obtaining a good yield of up to 92%, less time consumed and DMF solvent used. Next, Compounds 7a–c were oxidized by oxone, wherein, –SCH3 was oxidized to –SO2CH3, resulting in 8a–c with a yield of up to 95%.29 Then, the alkylation of 8a–c with ethyl chloroacetate produces N-alkylated ester products 9a–c with a yield of up to 66%. The formation of 9a–c was confirmed by IR, 1H NMR, and elemental analysis. The IR spectrum showed an ester (C
O) broad band at the range of 1730–1745 cm−1. The 1H NMR spectrum showed one triplet signal of aliphatic proton CH3CH2–, quartet signal of aliphatic proton CH3CH2– and a single signal of aliphatic proton –CH2CO in ranges of 1.12–1.17 δ, 4.10–4.16 δ and 5.33–5.35 δ, respectively.30 After that, compounds 9a–c were reacted with hydrazine hydrate to produce moderate yields of hydrazides 10a–c. The IR spectra of compounds 10a–c showed amide (C
O), –NH2 and –NH bands at approximately 1690, 3225 and 3330 cm−1, respectively. The 1H NMR spectra showed the disappearance of the signal of ethyl ester c protons and the appearance of exchangeable hydrazide –NH2 and –NH protons at about δ 4.43 and δ 9.60, respectively. Finally, the condensation of the synthesized hydrazide derivatives 10a–c with different aldehydes or ketones resulted in benzylidene 11a–l and compounds 12a–f, respectively, in moderate yield. The IR spectra of compounds 11a–l and 12a–f showed the absence of hydrazide NH2 bands.29 1H NMR spectra of 11a–l and 12a–f showed the respective proton patterns of the desired compounds, it also showed the presence of two geometrical isomers (E/Z) in different ratios (see ESI Table 1S†), which was confirmed not only by the presence of NCH2, N
CH, and CONH peaks as pairs of singlets but also confirmed by nuclear Overhauser effect spectroscopy (NOESY). Furthermore, both Z and E isomers, energies were minimized and the calculated total energy of compound 11b was 96.17 kcal mol−1, which is lower if it were in E configuration (see ESI Table 2S†), we concluded that the E isomer is predominant in agreement with already reported data of this type of reaction.31
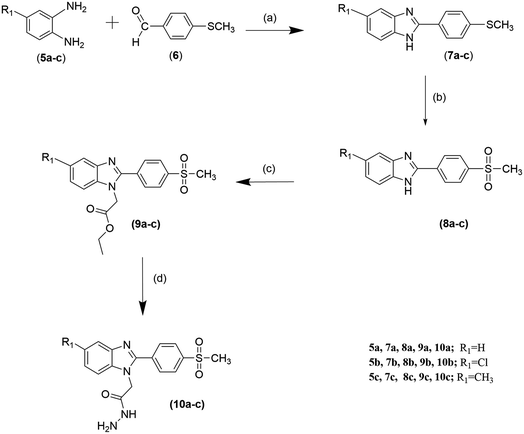 |
| Scheme 1 Synthesis of compounds 10a–c. Reagents and conditions: (a) DMF, Na2S2O5, 110 °C, 5 h; (b) oxone, H2O, 100 °C, 4 h; (c) DMF, K2CO3, ethyl chloroacetate stirring 6 h at 0 °C; (d) hydrazine hydrate, 4 h at rt. | |
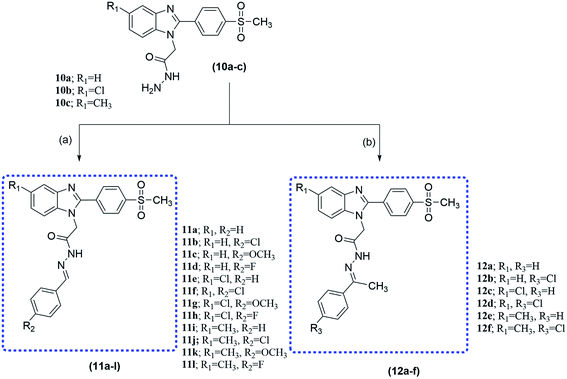 |
| Scheme 2 Synthesis of compounds 11a–l and 12a–f. Reagents and conditions: (a) aromatic aldehydes, catalytic glacial acetic acid, ethanol, 78 °C 2 h; (b) acetophenones, catalytic glacial acetic acid, 78 °C 2 h. | |
2.2. Biology
2.2.1. In vitro cyclooxygenase (COX) inhibition assay. The synthesized compounds were in vitro screened to determine their ability to inhibit both bovine COX-1 and COX-2 isozymes.32 The potency of the tested compounds was determined as the concentration causing 50% enzyme inhibition (IC50). The COX-2 selectivity index (SI value) was calculated and specified as SI = IC50 (COX-1)/IC50 (COX-2) and then compared to those of standards indomethacin, diclofenac sodium and celecoxib. As demonstrated in Table 1, compounds 11b, 12b, and 12d exhibited very low selectivities towards COX-1 isozyme (IC50 = 13.41, 12.54, 13.21 μM), (respectively) compared to those of reference drugs indomethacin and celecoxib (IC50 = 0.04, 15.10 μM). On the other hand, COX-2 selectivity of compounds 11b, 12b, and 12d is high (IC50 = 0.10 μM) compared to reference drugs indomethacin and celecoxib (IC50 = 0.51, 0.05 μm) (respectively).
Table 1 In vitro COX-1 and COX-2 inhibition of test compounds, indomethacin, diclofenac sodium and celecoxib as reference drugs for COX inhibition
Compd. |
COX-1a (IC50 μm) |
COX-2a (IC50 μm) |
Selectivity indexb (SI) |
The concentration of the tested compound required to produce an inhibition of 50 percent of COX-1 or COX-2. The results (IC50, μm) are the mean of three determinations acquired using assay Kits of an ovine COX-1/COX-2 (Cayman Chemicals Inc., Ann Arbor, MI, USA) and the mean deviation is <10% of the mean value. Selectivity index of in vitro COX-2 (COX-1 IC50/COX-2 IC50). |
a11 |
9.87 |
0.19 |
52 |
11b |
13.41 |
0.10 |
134 |
11c |
11.31 |
0.11 |
103 |
11d |
10.23 |
0.12 |
85 |
11f |
10.24 |
0.13 |
79 |
11g |
8.74 |
0.13 |
67 |
11h |
7.98 |
0.22 |
36 |
11j |
11.23 |
0.11 |
102 |
11k |
12.31 |
0.10 |
123 |
11l |
7.96 |
0.34 |
23 |
12a |
8.97 |
0.28 |
32 |
12b |
12.54 |
0.10 |
125 |
12d |
13.21 |
0.10 |
132 |
12f |
10.88 |
0.19 |
57 |
Celecoxib |
15.10 |
0.05 |
309 |
Diclofenac Sod. |
3.80 |
0.84 |
5 |
Indomethacin |
0.04 |
0.51 |
0.1 |
All tested compounds showed a higher selectivity index range (SI = 23–134) than that of reference drugs indomethacin and diclofenac (SI = 0.1, 5), (respectively). From tested compounds, the lowest selectivity against COX-2 was noticed for compounds 11l, 12a and 11h (SI = 23, 32, 36), (respectively), but all are better than indomethacin.
2.2.2. Anti-inflammatory activity. To determine anti-inflammatory activities of the target compounds (11a–11d, 11f–11h, 11j–11i, 12a–12b, 12d and 12f) the carrageenan-induced rat paw edema model was used.33 Results are shown in Table 2. From the data obtained, most potent COX-2 inhibitor compounds 11b, 11c, 11j, 11k, 12b and 12d exhibit correlated anti-inflammatory activity as they reduce inflammation after 6 hours by 95%, 93%, 93%, 95%, 95%, and 94.7%, (respectively). While compounds 11d, 11f and 11g, which exhibited moderate COX-2 inhibition activities (IC50 = 0.12, 0.13 and 0.13 μM, respectively) have acceptable inflammation reduction properties (90%, 90% and 78% reduction of inflammation after 6 hours, (respectively) compared to the reference drug indomethacin 97% reduction of inflammation after 6 hours) and celecoxib (96% reduction of inflammation after 6 hours).
Table 2 % Inhibition of paw edema for test compounds, celecoxib and indomethacin at 1, 3 and 6 h after carrageenan injection
Compounds |
Edema thickness (mm) ± SEMa (edema inhibition %) |
1 h (% inhibition) |
3 h (% inhibition) |
6 h (% inhibition) |
Data expressed as % inhibition and analyzed by one-way ANOVA (n = 4), P < 0.05, all were significant from control. |
Control |
2.620 ± 0.053 |
2.232 ± 0.067 |
1.897 ± 0.094 |
Celecoxib |
0.690 ± 0.017 (74%) |
0.142 ± 0.009 (94%) |
0.070 ± 0.005 (96%) |
Indomethacin |
0.094 ± 0.001 (77%) |
0.083 ± 0.005 (96%) |
0.051 ± 0.004 (97%) |
a11 |
1.048 ± 0.027 (60%) |
0.812 ± 0.037 (64%) |
0.578 ± 0.049 (69%) |
11b |
0.357 ± 0.012 (86%) |
0.155 ± 0.002 (93%) |
0.094 ± 0.006 (95%) |
11c |
0.487 ± 0.019 (81%) |
0.181 ± 0.013 (92%) |
0.130 ± 0.010 (93%) |
11d |
0.053 ± 0.019 (78%) |
0.427 ± 0.017 (81%) |
0.183 ± 0.014 (90%) |
11f |
0.577 ± 0.020 (78%) |
0.449 ± 0.008 (80%) |
0.191 ± 0.011 (90%) |
11g |
1.017 ± 0.007 (61%) |
0.785 ± 0.018 (65%) |
0.410 ± 0.018 (78%) |
11h |
1.30 ± 0.079 (50%) |
1.063 ± 0.020 (52%) |
0.754 ± 0.026 (60%) |
11j |
0.505 ± 0.022 (81%) |
0.194 ± 0.018 (91%) |
0.129 ± 0.008 (93%) |
11k |
0.464 ± 0.014 (82%) |
0.189 ± 0.017 (92%) |
0.111 ± 0.009 (94%) |
11l |
1.378 ± 0.009 (47%) |
1.102 ± 0.086 (51%) |
0.860 ± 0.010 (55%) |
12a |
1.308 ± 0.101 (50%) |
1.017 ± 0.024 (54%) |
0.766 ± 0.006 (60%) |
12b |
0.522 ± 0.009 (80%) |
0.240 ± 0.014 (89%) |
0.104 ± 0.005 (95%) |
12d |
0.286 ± 0.009 (89%) |
0.154 ± 0.008 (93%) |
0.098 ± 0.005 (95%) |
12f |
1.038 ± 0.014 (60%) |
0.801 ± 0.013 (64.%) |
0.563 ± 0.012 (70%) |
2.2.3. Ulcerogenic liability. Further screening was performed for the most active anti-inflammatory compounds 11b, 12d and 11k to determine the ulcerogenic effect (ulcer index), number of ulcers and preventive index.34 Results and relative ulcerogenicity related to indomethacin and celecoxib are shown (see ESI Table S3†). Results showed that all tested compounds have a lower ulcerogenic effect than the reference drugs celecoxib and indomethacin. Compound 11b was safest one (Ulcer Index) (UI = 0.83). Also compounds 12d and 11k showed lower ulcerogenic effect (Ulcer Index) (UI = 1–4.5) (respectively) compared to that of non-selective COX-2, indomethacin (UI = 13) and that of selective COX-2, celecoxib (UI = 3.5).
2.2.4. Histo-pathological study. Examination of histopathological lesions was performed to evaluate the cariogenic effect of the most active compounds 11b, 12d and 11k on both glandular and non-glandular portions of rat's stomach, and to compare the severity of those lesions with those induced by celecoxib and indomethacin as reference drugs.35 In the control negative group, a normal histological structure of the stomach could be found. The glandular stomach showed normal mucosa, submucosa and muscolosa. Moreover, a normal histological structure of the non-glandular stomach could be detected (see ESI Fig. S1A†).In the indomethacin group, severe lesions could be found in the form of degenerative changes and necrosis of glandular and non-glandular stomach. The glandular part exhibited ulcerative lesions and massive leucocytic infiltration, congestion and edema in the submucosal layer. Hyalinosis of the muscular layer might possibly be associated with diffuse leucocytic infiltration. The non-glandular stomach showed hyperkeratosis associated with multi-focal erosive and ulcerative lesions as well as hyperkeratosis (see ESI Fig. S1B†).
In the celecoxib group, mild lesions could be found in the glandular stomach, mainly degenerative changes of the mucosal lining, and mild lymphocytic infiltration in the submucosal layer. The non-glandular stomach portion exhibited minimal hyperkeratosis (see ESI Fig. S1C†).
The administration of compounds 11b and 12d showed mild pathological lesions represented by degenerative changes of the mucosal lining and minimal submucosal lymphocytic infiltration. The normal histological structure could be found in the non-glandular stomach histological structure (see ESI Fig. S1D†).
The administration of compound 11k revealed the presence of mild to moderate lesions mainly in the form of degeneration in the lining epithelium associated with mild necrotic changes. Furthermore, mild to moderate submucosal congestion associated with mild leucocytic infiltration was found, together with mild hyalinosis in the muscular layer. Moderate hyperkeratosis of the non-glandular stomach could be detected with the absence of any erosive or ulcerative lesions (see ESI Fig. S1E†) (Table 3).
Table 3 Pathological lesions on glandular and non-glandular of stomach caused by administration of most active compounds, indomethacin, and celecoxiba
Lesion |
11b |
12d |
11k |
Celecoxib |
Indomethacin |
Negative |
−/+ minimal, +/mild, ++/moderate, +++/severe. |
Glandular stomach |
|
|
|
|
|
|
Mucosa |
|
|
|
|
|
|
Degenerative changes |
++ |
+/++ |
+++ |
+/++ |
+++ |
−/+ |
Nuclear pyknosis |
— |
— |
+ |
+ |
+++ |
— |
Erosion |
+ |
−/+ |
+ |
+ |
+++ |
— |
Ulcer |
— |
— |
— |
— |
+++ |
— |
Submucosa |
|
|
|
|
|
|
Congestion |
+ |
+ |
+ |
++ |
+++ |
−/+ |
Leukocytic infiltration |
+ |
+ |
++ |
+ |
+++ |
— |
Edema |
−/+ |
+ |
+ |
+ |
+++ |
— |
Muscolosa |
|
|
|
|
|
|
Degenerative changes |
++ |
+ |
+ |
+ |
+++ |
— |
Hyalinosis |
+ |
+ |
+ |
+ |
+++ |
— |
Leukocytic infiltration |
+ |
−/+ |
++ |
+ |
+++ |
— |
Non-glandular stomach |
|
|
|
|
|
|
Erosion |
— |
— |
— |
— |
++ |
— |
Ulcer |
— |
— |
— |
— |
−/+ |
— |
Hyperkeratosis |
— |
+ |
+ |
+ |
+++ |
— |
2.3. Molecular docking study
A molecular modeling study was conducted to explain the potential binding modes and the difference in the selectivity profile of the most active compounds toward COX subtypes.36 COX-2 crystalline structure data were obtained from a protein data bank (PDB ID: 1CX2).49 Initial rescoring methodology (London dG) and the final restoring methodology (GBVI/WSA dG) were used to score fifty poses of the targeted compounds and celecoxib. Triangle Matcher protocol was used for placement, and Rigid Receptor was used for post-placement refinement. The investigated compounds showed binding modes as those of celecoxib with Arg120, which is the key amino acid of the active site. Celecoxib interacts with COX-2 active site through a sulfonamide moiety to form hydrogen bonds with Arg120 and through the Diazole ring to form a pi–H bond with Ser353. Furthermore, compounds 11b, 12d, 11k and 12b have extra binding interactions to receptor active sites, which may explain their superior activity compared to the activity of the rest of the compounds. The binding of the crystal structure of celecoxib with COX-2 receptor active site occurs by forming 2 hydrogen bonds with Ser353 and Arg120 amino acids through the diazole ring and sulfonyl moiety with 3.76, 2.84 Å, respectively, and with affinity binding at the energy of −17.66 kcal mol−1 (Fig. 2 a and b for celecoxib). The docking process of the tested compounds shows adequate interaction with affinity range (energy score) (−12.13, −16.95 kcal mol−1) between the active pharmacophore and amino acid active site inside COX-2 receptors with a distance range 3.18–4.36 Å from the main residue. These results complement those of celecoxib. The compound's higher selectivity is due to its higher binding affinity (see ESI Table S4†). Noticing that the compound 11b interacts through its diazole ring and methyl sulfonyl moiety with Ala527, Arg120 amino acids of COX-2 active site with a distance of 4.09, 3.26 Å, respectively (Fig. 2 c and d for 11b), while compound 12b interacts with Ala 527, Ser353 and Arg120 through its Diazole ring, as well as
N–H and –SO2CH3 moieties with distances from the main residue equal 4.04, 3.18 and 3.28 Å respectively (see ESI Fig. S2a and b† for 12b). Compound 12d interacts through its diazole ring and –SO2CH3 moieties with Ala527and Arg120with distances of 4.03 and 3.29 Å, respectively (see ESI Fig. S2c and d† for 12d). While compound 11k interacts through its benzo moiety, diazole ring,
N–NH and –SO2CH3 with Gly526, Ala527, Ser352 and Arg120 with distances of 4.17, 3.99, 3.30 and 3.29, respectively (see ESI Fig. S2e and f† for 11k).
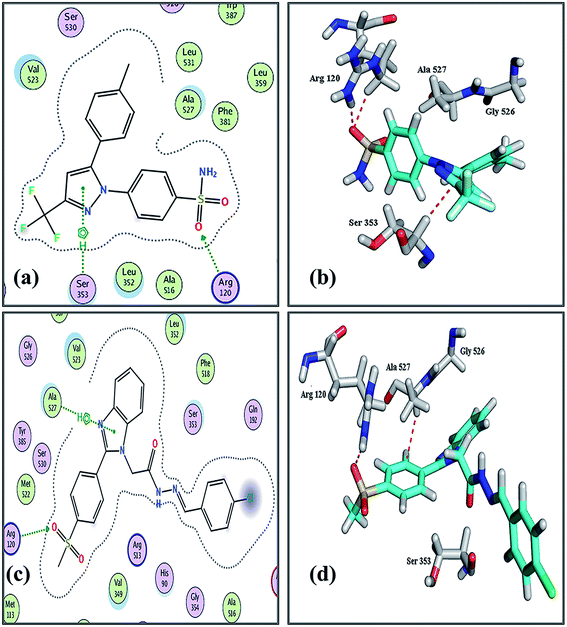 |
| Fig. 2 2D and 3D interaction of celecoxib (a) and (b) compound 11b (c) and (d) (respectively). | |
2.4. Computational analysis
2.4.1. In silico prediction of physicochemical properties, pharmacokinetics and drug-likeness profiles. Clinical trials of new investigated drugs are known to be extremely difficult due to improper ADME (absorption, distribution, metabolism, and excretion) properties, as well as high costs. As a result, evaluating the pharmacokinetic properties of a new drug is a significant phase in drug development.20,38 In silico ADME screens can now be used to identify the most promising compounds and reduce late-stage drug attrition.39 A compromise between pharmacodynamic and pharmacokinetic properties is required to achieve a favorable in vivo response. Additionally, oral bioavailability, brain penetration, the volume of distribution, and clearance predictions provide additional information about drug dosage and regimen.40Many parameters are investigated using virtual screening methods, such as human intestinal absorption HIA, partition coefficient (log
p), drug solubility (S), topological polar surface area (TPSA), cell permeability, and drug-likeness score. An available oral drug is chosen in accordance with Lipinski's rule of five if the molecular weight < 500, the number of hydrogen bond donors < 5, the number of hydrogen bond acceptors < 10, and Log
P is <5.41 The number of rotatable bonds is used to reflect molecular flexibility, which is important for oral bioavailability. It has also been proposed that the number of hydrogen bonding groups be replaced by topological polar surface area (TPSA) as a 3d descriptor in measuring percentage absorption (percent ABS) since it is inversely proportional to % ABS. % ABS = 109–0.345 PSA.
Oral bioavailability should be high for compounds with TPSA of less than 140 A2 and 10 or less rotatable bonds.42 Herein, to predict the pharmacokinetic parameters of the most active compounds, we used the Molsoft,43 SwissADME,44 and pkCSM software.45
Results in (see ESI Table S6†) show that most compounds obey Lipinski's rule, with MW ranging from 423.49 to 484.93 (<500), log
P values ranging from 3.24 to 4.64 (<5), HBD ranging is 1 (<5), and HBA ranging from 5 to 6 (<10). As a result, they should have good oral absorption, but variations in bioactivity cannot be attributed to this property. Furthermore, the compounds had numbers of rotatable bonds ranging from 7 to 8 (<10) and TPSA values of 101.3 and 104.80 A2 (140 A2), respectively, with oral absorption percentage ranging from 70.69 to 73.87%, suggesting good permeability, absorption, and transport across biological membranes. In general, it is understood that an orally available molecule that meets both Lipinski's and Veber's rules is considered to have a combination of lipophilicity and hydrophilicity.
Compounds 11b, 11k and 12b, as illustrated in Table 4 satisfy both Lipinski's and Veber's criteria, indicating that they may be used as drug-like molecules. Molsoft software was also used to evaluate the drug-likeness model score Table 4.
Table 4 Lipinski's drug-likeness of the most active compounds predicted by Swiss ADME and Molsoft software
Compound |
11b |
11k |
12b |
12d |
Drug likeness model score |
0.24 |
−0.06 |
0.41 |
0.03 |
Lipinski violation |
0 |
0 |
0 |
1 |
Bioavailability score |
0.55 |
0.55 |
0.55 |
0.55 |
The in silico analysis of the subsequent pharmacokinetic parameters was also carried out using pkCMS online software, the predicted ADME parameters' outcomes are depicted (see ESI Table S6†). The findings showed that all the tested molecules have significant oral absorption values and nearly similar water solubility values. All the tested compounds are expected to have high cellular permeability, especially in intestinal cells (92.84–99.57%) the tested compounds were discovered to be substrates of P-glycoprotein, which is a member of the ATP-binding cassette transporter found primarily in epithelial cells. In addition, the observed lipophilicities have a negative correlation with the tested compound's water solubility potentials, but a positive correlation with human colon adenocarcinoma (CaCo-2) permeability.
Investigated compounds exhibited no correlation between the lipophilicity and drug permeability as measured by the previously reported (Caco-2) cell line assay.46,47 According to the drug distribution prediction, compounds 11k and 12d have the best blood–brain barrier (BBB) permeability in comparison to other tested compounds. Results show that the investigated compounds were relatively active, inhibiting CYP2C19, CYP2C9, and CYP3A4. The parameters related to metabolism and excretion did not show any significant differences between the tested compounds, apart from compound 11k, which had a higher overall clearance than the others. In conclusion, the prediction of the toxicological properties of the tested compounds was applied by the pkCSM software. Here, results show that all investigated compounds have no hepatotoxicity or skin sensitization. Regarding cardiotoxicity, all the tested compounds show no inhibition except for compounds 12b. Despite this, the toxicant of the tested compounds in T. pyriformis was high.
3. Conclusion
A novel series of potent COX-2 inhibitory benzimidazole derivatives was designed, synthesized and evaluated in vitro as (COX-1)/(COX-2) inhibitors and in vivo as anti-inflammatory agents. All synthesized compounds showed higher selectivity than indomethacin. Compounds 11b, 11k, 12b and 12d showed the most robust activity with high COX-2 selectivity and inhibitory activity (SI = 134, 123, 125, 132, respectively) (IC50 = 0.10 μM). The selected compounds showed a good gastric safety profile upon histopathological lesions examination from the rat stomach. Finally, in silico ADME predictions, physic–chemical properties and drug-likeness profiles of the most active compounds were performed. As a result, it is possible to say that compounds 11b, 12b and 12d should be subjected to further studies.
4. Experimental
4.1. Chemistry
Melting points were detected by the Tomas–Hoover capillary melting apparatus without any correction. All solvents, chemicals, and reagents were obtained from Aldrich Chemical Company (Milwaukee, WI), and El Nasr pharmaceutical chemical companies, Cairo, Egypt. Infrared (IR) spectra were obtained using films on KBr diks on a Schimadzu FT-IR 8400S spectrophotometer and values were presented as cm−1. The purity of the synthesized compounds and the reaction progress was monitored using precoated thin layer chromatography (TLC) silica gel plates 60F254 with a thickness of 0.25 supplied from MERCK, Darmstadt, Germany. The UV lamp was used to monitor the reaction process. 13C NMR and 1H NMR spectra were measured on a Bruker Avance III 400 MHz spectrophotometer (faculty of pharmacy, Benisuef University and Mansoura University, Egypt) using dimethyl sulfoxide (DMSO-d6) or D2O as a solvent. The chemical shift was estimated in ppm on δ scale and J (coupling constant) was estimated in Hertz. Microanalysis for C, H, and N were performed on a PerkinElmer 2400 analyzer (PerkinElmer, Norwalk, CT. USA) at the regional center for mycology and Biotechnology, Al-Azhar University, Egypt. All results were within ± 0.4% of the theoretical values.
4.1.1. General procedure for the synthesis of (7a–c).
4.1.1.1 By traditional method. A mixture of 4-methylthio benzaldehyde (3.2 mmol, 0.5 g), and an equal amount of substituted o-phenylene diamine in addition to sodium metabisulfite (1 g) dissolved in N,N-dimethyl formamide (DMF, 10 ml). The mixture was heated under reflux with stirring at 110 °C for 5–10 h. After completion of the reaction (monitored with TLC), the reaction mixture was poured into ice and the solid precipitate obtained was collected by filtration and washed several times with cold water (5 × 20 ml). The crude product was recrystallized from ethanol and dried for further uses.28
4.1.1.2 By microwave radiations. A mixture of 4-methylthio benzaldehyde (3.2 mmol, 0.5 g), and an equal amount of substituted o-phenylene diamine in addition to sodium metabisulfite dissolved in N,N-dimethyl formamide (DMF, 10 ml). The mixture was heated by microwave radiation at 120 °C and was stirred at 600 rpm. The completion of the reaction (monitored with TLC), the reaction mixture was poured into ice and the obtained solid precipitate was collected by filtration and washed several times with cold water (5 × 20 ml). The crude product was recrystallized from ethanol and dried for further uses.28
4.1.1.3. 2-(4-(Methylthio) phenyl)-1H-benzo[d]imidazole (7a). Buff solid; yield 92%; mp 225–227 °C; IR: (KBr, cm−1) 3345 (NH imidazole), 3026 (CH aromatic), 1612 (C
C), 1462 (C
N); 1HNMR (DMSO-d6, 400 MHz, δ ppm): 2.56 (s, 3H, SO2CH3), 7.21–7.23 (m, 2H, benzimidazole H-4, H-5), 7.43–7.45 (d, 2H, J = 8.00 Hz, phenyl H-2, H-6), 7.59–7.61 (d, 2H, J = 8.00 Hz, benzimidazole H-6, H-7), 8.10–8.12 (d, 2H, J = 8.00 Hz, phenyl H-3, H-5), 12.77 (s, 1H, imidazole NH, D2O exchangeable). Anal. calcd for C14H12 N2S: C, 69.97; H, 5.03; N, 11.66; found C, 69.58; H, 4.99; N, 12.00.
4.1.1.4. 5-Chloro-2-(4-(methylthio) phenyl)-1H-benzo[d]imidazole (7b). Gray solid; yield 89%; mp 164–165 °C; IR: (KBr, cm−1) 3350 (NH imidazole), 3036 (CH aromatic), 1622 (C
C), 1477 (C
N); 1HNMR (DMSO-d6, 400 MHz, δ ppm): 2.55 (s, 3H, SO2CH3), 7.24–7.26 (d, 1H, J = 8.00 Hz, benzimidazole, H-6), 7.43–7.45 (d, 2H, J = 8.00 Hz, phenyl H-2, H-6), 7.60–7.62 (d, 1H, J = 8.00 Hz, benzimidazole H-7), 7.65 (s, 1H, benzimidazole H-4), 8.09–8.11 (d, 2H, J = 8.00 Hz, phenyl H-3, H-5). Anal. calcd for C14H11Cl N2S C, 61.20; H, 4.04; N, 10.20; found: C, 61.24; H, 4.36; N, 10.51.
4.1.1.5. 5-Methyl-2-(4-(methylthio) phenyl)-1H-benzo[d]imidazole (7c). Off white solid; yield 90%; mp 186–187 °C; IR: (KBr, cm−1) 3335 (NH imidazole), 3012 (CH aromatic), 1605, (C
C), 1460 (C
N); 1HNMR (DMSO-d6, 400 MHz, δ ppm): 2.43 (s, 3H, SO2CH3), 2.53 (s, 3H, aliphatic CH3) δ, 7.01–7.03 (d, 1H, J = 8.00 Hz, benzimidazole, H-6), 7.39 (s, 1H, benzimidazole, H-4), 7.40–7.42 (d, 2H, J = 8.00 Hz, phenyl H-2, H-6), 7.48–7.50 (d, 1H, J = 8.00 Hz, benzimidazole H-7), 8.12–8.14 (d, 2H, J = 8.00 Hz, phenyl H-3, H-5), anal. calcd for C15H14 N2S C, 70.83; H, 5.55; N, 11.01; found: C, 71.20; H, 5.65; N, 11.35.
4.1.2. General procedure for the synthesis of (8a–c). Compounds (7a–c) (2 mmol) dissolved in 10 ml of methyl alcohol. Oxone (3.2 mmol, 1 g) dissolved in water then added and stirred under reflux at 60–70 °C. Oxidation was monitored by TLC then the reaction mixture was poured into cold water (50 ml). The product was extracted with EtOAc, and anhydrous Na2SO4 was used to dry the organic layer to obtain the crude product, which was recrystallized from ethyl alcohol to obtain the targeted compounds 8a–c.48
4.1.2.1. 2-(4-(Methylsulfonyl) phenyl)-1H-benzo[d]imidazole (8a). Buff solid; yield 95%; mp 225–227 °C; IR: (KBr, cm−1) 3355 (NH imidazole), 3037 (CH aromatic), 1621 (C
C), 1473, (C
N) 1315–1151 (SO2); 1HNMR (DMSO-d6, 400 MHz, δ ppm): 3.33 (s, 3H, SO2CH3), 7.42–7.44 (m, 2H, benzimidazole H-4, H-5), 7.77–7.79 (m, 2H, benzimidazole H-6, H-7), 8.19–8.21 (d, 2H, J = 8.00 Hz, phenyl H-2, H-6), 8.43–8.45 (d, 2H, J = 8.00 Hz, phenyl H-3, H-5), (1H, imidazole NH undetectable).13C NMR (100 MHz DMSO-d6): δ 159.2, 148.8, 129.5, 123.4, 121.2, 116.7, 16.8. Anal. calcd for C14H12 N2O2S: C, 61.75; H, 4.44; N, 10.29; found: C, 61.91; H, 4.19; N, 10.25.
4.1.2.2 5-Chloro-2-(4-(methylsulfonyl) phenyl)-1H-benzo[d]imidazole (8b). Gray solid; yield 91%; mp 150–152 °C; IR: (KBr, cm−1) 3359 (NH imidazole), 3045 (CH aromatic), 1633 (C
C), 1489 (C
N), 1318, 1153 (SO2); 1HNMR (DMSO-d6, 400 MHz, δ ppm): 3.31 (s, 3H, SO2CH3), 7.33–7.35 (d, 1H, J = 8.00 Hz, benzimidazole, H-6), 7.70–7.72 (d, 2H, J = 8.00 Hz, benzimidazole H-7), 7.8 (s, 1H, benzimidazole H-4), 8.14–8.16 (d, 2H, J = 8.00 Hz, phenyl H-2, H-6), 8.40–8.42 (d, 2H, J = 8.00 Hz, phenyl H-3, H-5), anal. calcd for:C14H11 ClN2O2S C, 54.81; H, 3.61; N, 9.13; found: C, 54.61; H, 3.61; N, 9.44.
4.1.2.3 5-Methyl-2-(4-(methylsulfonyl) phenyl)-1H-benzo[d]imidazole (8c). Off white solid; yield 90%; mp 179–181 °C; IR: (KBr, cm−1) 3346 (NH imidazole), 3021 (CH aromatic), 1617 (C
C), 1471 (C
N), 1300–1148 (SO2); 1HNMR (DMSO-d6, 400 MHz, δ ppm): 2.43 (s, 3H, aliphatic CH3), δ 3.31 (s, 3H, SO2CH3), 7.18–7.20 (d, 1H, J = 8.00 Hz benzimidazole, H-6), 7.50 (s, 1H, benzimidazole H-4), 7.60–7.62 (d, 1H, J = 8.00 Hz, benzimidazole, H-7), 8.15–8.17 (d, 2H, J = 8.00 Hz, phenyl H2, H-6), 8.39–8.41 (d, 2H, J = 8.00 Hz, phenyl H-3, H-5), anal. calcd for C15H14N2O2S C, 62.92; H, 4.93; N, 9.78; found: C, 62.67; H, 5.08; N, 9.50.
4.1.3. General procedure for the synthesis of (9a–c). A mixture of compounds 8a, 8b or 8c (1.83 mmol), DMF (20 ml) and anhydrous K2CO3 (10 mmol, 1 g) was stirred cold for 2 h then ethyl chloroacetate (1.83 mmol, 0.256 ml) was added portion-wise. The reaction mixture was refluxed for 10 h then poured into ice and the product was obtained upon filtration. The products were confirmed by FTIR spectroscopy and 1H NMR.29,30
4.1.3.1 Ethyl 2-(2-(4-(methylsulfonyl) phenyl)-1H-benzo[d]imidazol-1-yl) acetate (9a). Brown solid; yield 66%; mp 153–155 °C; IR: (KBr, cm−1) 3046 (CH aromatic), 1740 (C
O ester), 1630 (C
C), 1481 (C
N), 1326–1163 (SO2); 1HNMR (DMSO-d6, 400 MHz, δ ppm): 1.12–1.16 (t, 3H, CH3CH2), 3.33 (s, 3H, SO2CH3), 4.10–4.16 (q, 2H, CH3CH2), 5.33 (s, 2H, CH2CO), 7.33–7.36 (m, 2H, benzimidazole H-5, H-6), 7.66–7.68 (d, 1H, J = 8.00 Hz, benzimidazole H-4), 7.77–7.79 (d, 2H, J = 8.00 Hz, benzimidazole H-7), 8.01–8.03 (d, 2H, J = 8.00 Hz, phenyl H-2, H-6), 8.12–8.14 (d, 2H, J = 8.00 Hz, phenyl H-3, H-5), 13C NMR (100 MHz DMSO-d6): δ 14.36, 43.83, 46.55, 61.96, 111.36, 119.98, 123.16, 123.84, 127.95, 127.96, 130.30, 130.31, 135.7, 136.90, 142.19, 142.82, 152.09, 168.59, anal. calcd for C18H18 N2O4S: C, 60.32; H, 5.06; N, 7.82, found: C, 59.94; H, 5.41; N, 7.46.
4.1.3.2 Ethyl 2-(5-chloro-2-(4-(methylsulfonyl) phenyl)-1H-benzo[d]imidazole-1-yl) acetate (9b). Pale brown solid; yield 60%; mp 100–101 °C; IR: (KBr, cm−1) 3046 (CH aromatic), 1748 (C
O ester), 1636 (C
C), 1488 (C
N) 1330–1169 (SO2); 1HNMR (DMSO-d6, 400 MHz, δ ppm): 1.11–1.25 (t, 3H, CH3CH2), 3.33 (s, 3H, SO2CH3), 4.10–4.16 (q, 2H, CH3CH2), 5.33–5.35 (s, 2H, CH2CO), 7.34–7.42 (m, 2H, benzimidazole H-6), 7.73–7.8 (m, 1H, benzimidazole H-7), 7.91 (s 1H, benzimidazole H-4), 7.90–8.02 (q, 2H, phenyl H-2, H-6), 8.12–8.15 (m, 2H, phenyl H-3, H-5), 13C NMR (100 MHz DMSO-d6): δ14.29, 41.13, 43.80, 62.52, 111.35, 119.38, 121.26, 123.19, 123.39, 126.03, 127.78, 130.32, 134.64, 137.68, 141.51, 142.37, 153.16, 167.64, anal. calcd for C18H17CL N2O4S: C, 55.03; H, 4.36; N, 7.13; found: C, 55.04; H, 4.69; N, 7.04.
4.1.3.3 Ethyl 2-(5-methyl-2-(4-(methylsulfonyl) phenyl)-1H-benzo[d]imidazol-1-yl) acetate (9c). Buff solid; yield 58%; mp 115–116 °C; IR: (KBr, cm−1) 3039 (CH aromatic), 1736 (C
O ester), 1627 (C
C), 1477 (C
N), 1321–1155 (SO2); 1HNMR (DMSO-d6, 400 MHz, δ ppm): 1.12–1.17 (t, 3H, CH3CH2), 2.40 (s, 3H, aliphatic CH3), 3.31 (s, 3H, SO2CH3), 4.12–4.16 (q, 2H, CH3CH2), 5.17–5.29 (s, 2H, CH2CO), 7.12–7.14 (d, 1H, J = 8.00 Hz benzimidazole, H-6), 7.46 (s, 1H, benzimidazole H-4), 7.64–7.66 (d, 1H, J = 8.00 Hz benzimidazole H-7), 7.99–8.01 (d, 2H, J = 8.00 Hz, phenyl H-2, H-6), 8.11–8.13 (d, 2H, J = 8.00 Hz, phenyl H-3, H-5), 13C NMR (100 MHz DMSO-d6): δ14.34, 21.90, 40.56, 43.53, 62.19, 110.90, 119.51, 126.03, 127.94, 129.74, 130.21, 130.26, 132.43, 135.10, 141.80, 142.07, 142.90, 151.92, 168.56, anal. calcd for C19H20 N2O4S: C, 61.27; H, 5.41; N, 7.52; found: C, 61.61; H, 5.97; N, 7.70.
4.1.4. General procedure for the synthesis of (10a–c). A mixture of compounds 9a, 9b or 9c (1.39 mmol) and hydrazine hydrate 99% (1.39 mol, 0.071 ml) was refluxed for 6 h in absolute ethanol (20 ml). Then the reaction mixture was poured into the water after cooling. The targeted hydrazides were obtained after filtration and recrystallized from ethanol.28
4.1.4.1 2-(2-(4-(Methylsulfonyl) phenyl)-1H-benzo[d]imidazol-1-yl)acetohydrazide (10a). Off-white solid; yield 73%; mp 183–185 °C; IR: (KBr, cm−1) 3330 (NH), 3225 (NH2), 1694 (C
O amide), 1620 (C
N) 1335–1168 (SO2); 1HNMR (DMSO-d6, 400 MHz, δ ppm): 3.33 (s, 3H, SO2CH3), 4.43 (s, 2H, NH2, D2O exchangeable), 4.91 (s, 2H, CH2), 7.31–7.34 (t, 2H, benzimidazole H-5, H-6), 7.53–7.55 (d, 1H, J = 8.00 Hz, benzimidazole H-4), 7.75–7.76 (d, 1H, J = 8.00 Hz benzimidazole H-7), 8.12–8.14 (d, 4H, J = 8.00 Hz phenyl H-2, H-3, H-5, H-6), 9.6 (s, 1H, NH, D2O exchangeable), 13C NMR (100 MHz DMSO-d6): δ43.80, 46.30, 49.07, 111.25, 119.91, 122.99, 123.58, 127.75, 130.67, 130.70, 135.30, 136.80, 142.07, 142.90, 152.46, 166.53, anal. calcd for C16H16 N4O3S: C, 55.80; H, 4.68; N, 16.27; found: C, 55.66; H, 4.28; N, 16.15.
4.1.4.2 2-(5-Chloro-2-(4-(methylsulfonyl) phenyl)-1H-benzo[d]imidazol-1-yl) acetohydrazide (10b). White solid; yield 70%; mp 136–137 °C; IR: (KBr, cm−1) 3336 (NH), 3231 (NH2), 1698 (C
O amide), 1639 (C
N), 1334–1170 (SO2); 1HNMR (DMSO-d6, 400 MHz, δ ppm): 3.31 (s, 3H, SO2CH3), 4.43 (s, 2H, NH2, D2O exchangble), 4.93 (s, 2H, CH2), 7.32–7.40 (m, 1H, benzimidazole, H-6), 7.71–7.78 (m, 2H, benzimidazole H-7), 8.00 (s, 1H, benzimidazole H-4), 8.112 (s, 4H, phenyl H-2 H-3, H-5, H-6), 9.6 (s, 1H, NH, D2O exchangeable), 13C NMR (100 MHz DMSO-d6): δ40.43, 43.46, 46.44, 111.39, 119.16, 121.25, 123.43, 127.84, 128.16, 130.73, 135.06, 137.60, 142.13, 143.60, 153.57, 166.33, anal. calcd for C16H15Cl N4O3S: C, 50.73; H, 3.99; N, 14.79; found: C, 50.92; H, 3.65; N, 14.61.
4.1.4.3 2-(5-Methyl-2-(4-(methylsulfonyl) phenyl)-1H-benzo[d]imidazol-1-yl) acetohydrazide (10c). Pale yellow solid; yield 67%; mp 146–147 °C; IR: (KBr, cm−1) 3326 (NH), 3219 (NH2), 1690 (C
O amide), 1616 (C
N), 1322–1160 (SO2); 1HNMR (DMSO-d6, 400 MHz, δ ppm): 2.45 (s, 3H, aliphatic CH3), 3.32 (s, 3H, SO2CH3), 4.42 (s, 2H, NH2, D2O exchangeable), 4.88 (s, 2H, CH2), 7.15–7.17 (d, 1H, J = 8.00 Hz, benzimidazole, H-6), 7.40–7.42 (s, 1H, J = 8.00 Hz, benzimidazole H-4), 7.54–7.64 (m, 1H, benzimidazole H-7), 8.10–8.11 (m, 4H, phenyl H-2, H-3, H-5, H-6), 9.62 (s, 1H, NH, D2O exchangble), 13C NMR (100 MHz DMSO-d6): δ14.77, 21.81, 43.86, 46.22, 110.80, 119.56, 125.03, 125.92, 127.90, 130.05, 130.55, 130.60, 135.44, 141.81, 143.22, 152.34, 166.63, anal. calcd for C17H18 N4O3S: C, 56.97; H, 5.06; N, 15.63; found: C, 56.65; H, 5.44; N, 15.79.
4.1.5. General procedure for the synthesis of (11a–i). A mixture of compounds 10a, 10b or 10c (1.45 mmol) and appropriate aromatic aldehyde (1.45 mmol) in ethyl alcohol (25 ml) was refluxed for 6 h in the presence of glacial acetic acid in a catalytic amount. The product was recrystallized from ethanol.37
4.1.5.1 (E)-N′-Benzylidene-2-(2-(4-(methylsulfonyl) phenyl)-1H-benzo[d]imidazol-1-yl) acetohydrazide (11a). Pale brown; yield 60%; mp 150–152 °C; IR: (KBr, cm−1) 3408 (NH), 1699 (C
O), 1614 (C
N), 1320–1158 (SO2); 1HNMR (DMSO-d6, 400 MHz, δ ppm): 3.33 (s, 3H, SO2CH3), 5.62 (s, 2H, CH2), 7.31–7.33 (t, 2H, J = 8.00 Hz benzimidazole H-5, H-6), 7.45–7.47 (d, 4H, J = 8.00 Hz, benzimidazole H-4, H-7, phenyl hydrazone H-2, H-6), 7.74–7.76 (t, 3H, J = 8.00 Hz, phenyl hydrazone H-3, H-4, H-5) 8.04–8.06 (d, 2H, J = 8.00 Hz, phenyl H-2, H-6), 8.07–8.09 (d, 2H, J = 8.00 Hz, phenyl H-3, H-5), 8.12 (s, 1H CH
N), 11.83 (s, 1H, NH, D2O exchangeable), 13C NMR (100 MHz DMSO-d6): δ 43.04 (CH2 aliphatic), 46.26 (SO2CH3), 111.36, 119.87, 122.87, 123.57, 127.58, 127.87, 129.00, 129.25, 129.31, 129.72, 130.27, 130.49, 130.66, 134.42, 135.56, 137.36, 141.99, 142.92, 145.14, 152.51, 167.39. Anal. calcd for C23H20N4O3S: C, 63.87; H, 4.66; N, 12.95; found: C, 64.13; H, 4.53; N, 13.24.
4.1.5.2 (E)-N′-(4-Chlorobenzylidene)-2-(2-(4-(methylsulfonyl) phenyl)-1H-benzo[d]imidazol-1-yl) acetohydrazide (11b). Buff solid; yield 56%; mp 148–150 °C; IR: (KBr, cm−1) 3407 (NH), 1698 (C
O), 1612 (C
N), 1316–1154 (SO2); 1HNMR (DMSO-d6, 400 MHz, δ ppm): 3.31 (s, 3H, SO2CH3), 5.62 (s, 2H, CH2), 7.31–7.33 (t, 2H, J = 8.00 Hz, benzimidazole H-5, H-6), 7.5–7.52 (d, 2H, J = 8.00 Hz, benzimidazole H-4, H-7), 7.77–7.79 (d, 4H, J = 8.00 Hz, phenyl hydrazone H-2, H-3, H-5, H-6), 8.03–8.05 (d, 2H, J = 8.00 Hz, phenyl H-2, H-6), 8.09–8.10 (d, 2H, J = 8.00 Hz, phenyl H-3, H-5), 8.12 (s, 1H, CH
N), 11.88 (s, 1H, NH, D2O exchangble), Anal. calcd for C23H19ClN4O3S: C, 59.16; H, 4.10; N, 12.00; found: C, 59.35; H, 4.22; N, 12.16.
4.1.5.3 (E)-N′-(4-Methoxybenzylidene)-2-(2-(4-(methylsulfonyl) phenyl)-1H-benzo[d]imidazol-1-yl) acetohydrazide (11c). Off white; yield 52%; mp 161–163 °C; IR: (KBr, cm−1) 3401 (NH), 1695 (C
O), 1610, (C
N) 1311, 1150 (SO2); 1HNMR (DMSO-d6, 400 MHz, δ ppm): 3.31 (s, 3H, SO2CH3), 3.7 (s, 3H, OCH3), 5.58 (s, 2H, CH2), 6.94–6.98 (t, 2H, benzimidazole H-5, H-6), 7.3–7.32 (d, 2H, J = 8.00 Hz, benzimidazole H-4, H-7), 7.51–7.53 (d, 1H, J = 8.00 Hz, phenyl hydrazone H-3), 7.61–7.63 (d, 2H, J = 8.00 Hz, phenyl hydrazone H-2, H-6), 7.73–7.75 (d, 1H, J = 8.00 Hz, phenyl hydrazone H-5), 7.96–7.99 (d, 2H, J = 8.00 Hz, phenyl H-2, H-6), 8.05–8.07 (d, 2H, J = 8.00 Hz, phenyl H-3, H-5), 8.08 (s, 1H CH
N), 11.6 (s, 1H, NH, D2O exchangeable), 13C DEPT-Q NMR (100 MHz DMSO-d6): δ 43.57 (CH2 aliphatic), 46.05, (SO2CH3) 55.90, (OCH3), 111.43, 114.73, 114.86, 119.83, 122.96, 123.69, 124.60, 126.70, 127.89, 129.21, 129.36, 130.29, 130.60, 135.49, 137.25, 141.99, 142.73, 146.10, 152.50, 161.32, 168.36. Anal. calcd for C24H22N4O4S C, 62.32; H, 4.79; N, 12.11; found: C, 62.60; H, 4.65; N, 12.37.
4.1.5.4 (E)-N′-(4-Fluorobenzylidene)-2-(2-(4-(methylsulfonyl) phenyl)-1H-benzo[d]imidazol-1-yl) acetohydrazide (11d). Dark yellow; yield 49%; mp 149–151 °C; IR: (KBr, cm−1) 3408 (NH), 1699 (C
O), 1615 (C
N), 1313–1155 (SO2); 1HNMR (DMSO-d6, 400 MHz, δ ppm): 3.29 (s, 3H, SO2CH3), 5.62 (s, 2H, CH2), 7.27–7.29 (t, 2H, benzimidazole H-5, H-6), 7.30–7.32 (d, 2H, J = 8.00 Hz, benzimidazole H-4, H-7), 7.77–7.82 (M, 4H, phenyl hydrazone, H-2, H-3, H-5, H-6) 8.04–8.06 (d, 2H, J = 8.00 Hz, phenyl H-2, H-6), 8.10–8.12 (d, 2H, J = 8.00 Hz, phenyl H-3, H-5), 8.13 (s, 1H, CH
N), 11.84 (s, 1H, NH, D2O exchangeable). 13C NMR (100 MHz DMSO-d6): δ 43.71 (CH2 aliphatic), 46.26 (SO2CH3), 111.44, 116.20, 116.33, 119.74, 122.84, 123.62, 127.87, 129.75, 129.84, 130.26, 130.57, 130.98, 135.47, 137.29, 142.15, 142.92, 143.07, 143.85, 152.46, 162.38, 168.67, anal. calcd for C23H19FN4O3S: C, 61.32; H, 4.25; N, 12.44; found: C, 61.47; H, 4.18; N, 12.70.
4.1.5.5 (E)-N′-Benzylidene-2-(5-chloro-2-(4-(methylsulfonyl) phenyl)-1H-benzo[d]imidazol-1-yl) acetohydrazide (11e). Pale brown solid; yield 45%; mp 149–151 °C; IR: (KBr, cm−1) 3404 (NH), 1695 (C
O), 1611 (C
N), 1312–1149 (SO2); 1HNMR (DMSO-d6, 400 MHz, δ ppm): 3.31 (s, 3H, SO2CH3), 5.64 (s, 2H, CH2), 7.32–7.34 (d, 1H, J = 8.00 Hz, benzimidazole, H-6), 7.44–7.46 (d, 4H, J = 8.00 Hz, benzimidazole H-4, H-7 phenyl hydrazone, H-2, H-6), 7.71–7.73 (t, 1H, J = 8.00 Hz, phenyl hydrazone, H-3, H-4, H-5), 8.01–8.03 (d, 4H, J = 8.00 Hz, phenyl H-2, H-6), 8.05–8.07 (d, 4H, J = 8.00 Hz, phenyl H-3, H-5), 8.13 (s, 1H CH
N), 11.82(s, 1H, NH, D2O exchangeable) 13C NMR (100 MHz DMSO-d6): δ 43.72 (CH2 aliphatic), 46.27 (SO2CH3), 111.77, 112.91, 119.22, 121.15, 127.57, 127.86, 129.22, 130.30, 130.36, 130.58, 130.61, 134.21, 135.18, 136.11, 138.19, 141.57, 142.35, 143.73, 145.21, 153.50, 168.41, anal. calcd for C23H19ClN4O3S: C, 59.16; H, 4.10; N, 12.00; found: C, 59.42; H, 4.23; N, 11.79.
4.1.5.6 (E)-N′-(4-Chlorobenzylidene)-2-(5-chloro-2-(4-(methylsulfonyl) phenyl)-1H-benzo[d]imidazol-1-yl) acetohydrazide (11f). Buff solid; yield 48%; mp 152–154 °C; IR: (KBr, cm−1) 3409 (NH), 1698 (C
O), 1616 (C
N), 1315, 1151 (SO2); 1HNMR (DMSO-d6, 400 MHz, δ ppm): 3.31 (s, 3H, SO2CH3), 5.63 (s, 2H, CH2), 7.30–7.33 (m, 2H, benzimidazole, H-6, H-7), 7.50–7.52 (d, 2H, J = 8.00 Hz, phenyl hydrazone, H-2, H-6), 7.77–7.79 (m, 3H, benzimidazole, H4-phenyl hydrazone, H-3,H-5) 8.03–8.05 (d, 2H, J = 8.00 Hz, phenyl H-3, H-5), 8.09–8.11 (d, 4H, J = 8.00 Hz, phenyl H-2, H-6), 8.12 (s, 1H CH
N), 11.91 (s, 1H, NH, D2O exchangeable), 13C NMR (100 MHz DMSO-d6): δ 43.57 (CH2 aliphatic), 46.20 (SO2CH3), 111.41, 119.26, 122.77, 123.76, 127.89, 129.25, 129.33, 130.25, 133.27, 133.30, 136.06, 136.25, 136.26, 136.52, 136.94, 137.30, 142.03, 142.89, 143.86, 152.48, 164.12. Anal. calcd for C23H18Cl2N4O3S: C, 55.10; H, 3.62; N, 11.17; found: C, 55.37; H, 3.81; N, 11.43.
4.1.5.7 (E)-N′-(4-Methoxybenzylidene)-2-(5-chloro-2-(4-(methylsulfonyl) phenyl)-1H-benzo[d]imidazol-1-yl) acetohydrazide (11g). Yellow solid; yield 41%; mp 162–164 °C; IR: (KBr, cm−1) 3408 (NH), 1699 (C
O), 1615, (C
N), 1315–1152 (SO2); 1HNMR (DMSO-d6, 400 MHz, δ ppm): 3.31 (s, 3H, SO2CH3), 3.75 (s, 3H, OCH3), 5.51 (s, 2H, CH2), 6.92–6.94 (d, 2H, J = 8.00 Hz benzimidazole, H-6, H-7), 7.28–7.30 (d, 2H, J = 8.00 Hz, phenyl hydrazone, H-2, H-6), 7.58–7.60 (d, 3H, J = 8.00 Hz, benzimidazole, H4-phenyl hydrazone, H-3, H-5), 7.94–7.96 (d, 2H, J = 8.00 Hz, phenyl H-2, H-6), 8.00–8.02 (d, 4H, J = 8.00 Hz, phenyl H-3, H-5), 8.06 (s, 1H CH
N)11.7 (s, 1H, NH, D2O exchangble), 13CNMR (100 MHz DMSO-d6): δ 43.74 (CH2 aliphatic), 46.52 (SO2CH3), 55.77, 111.77, 114.72, 114.81, 119.24, 121.13, 123.27, 123.74, 126.85, 126.89, 127.37, 127.82, 129.34, 130.29, 130.69, 130.72, 135.10, 138.18, 141.63, 142.24, 161.32, 168.18. Anal. calcd for C24H21ClN4O4S: C, 58.00; H, 4.26; N, 11.27; found: C, 57.78; H, 4.35; N, 11.51.
4.1.5.8 (E)-N′-(4-Fluorobenzylidene)-2-(5-chloro-2-(4-(methylsulfonyl) phenyl)-1H-benzo[d]imidazol-1-yl) acetohydrazide (11h). Pale yellow; yield 51%; mp 153–155 °C; IR: (KBr, cm−1) 3410 (NH), 1699 (C
O), 1618 (C
N), 1317–1153 (SO2); 1HNMR (DMSO-d6, 400 MHz, δ ppm): 3.31 (s, 3H, SO2CH3), 5.65 (s, 2H, CH2), 7.27–7.31 (m, 3H, benzimidazole H-4, H-6, H-7), 7.76–7.86 (m, 4H, J = 8.4 Hz, phenyl hydrazone, H-2, H-3, H-5, H-6) 8.03–8.05 (d, 2H, J = 8.00 Hz, phenyl H-2, H-6), 8.08–8.10 (d, 4H, J = 8.00 Hz, phenyl H-3, H-5) 8.12 (s, 1H CH
N), 11.81 (s, 1H, NH, D2O exchangeable), 13C NMR (100 MHz DMSO-d6): δ 43.33 (CH2 aliphatic), 46.54 (SO2CH3), 111.75, 116.18, 116.39, 119.25, 121.14, 123.29, 124.57, 127.92, 129.74, 129.83, 130.28, 130.32, 130.93, 136.09, 141.63, 141.68, 142.25, 143.68, 143.93, 162.14, 168.49, Anal. calcd for C23H18ClFN4O3S: C, 56.97; H, 3.74; N, 11.55; found: C, 57.12; H, 3.88; N, 11.69.
4.1.5.9 (E)-N′-Benzylidene-2-(5-methyl-2-(4-(methylsulfonyl) phenyl)-1H-benzo[d]imidazol-1-yl) acetohydrazide (11i). Buff solid; yield 59%; mp 152–154 °C; IR: (KBr, cm−1) 3409 (NH), 1698 (C
O), 1616 (C
N), 1315–1151 (SO2); 1HNMR (DMSO-d6, 400 MHz, δ ppm): 2.45 (s, 3H, CH3), 3.29 (s, 3H, SO2CH3), 5.67 (s, 2H, CH2), 7.14–7.16 (d, 1H, J = 8.00 Hz benzimidazole, H-6), 7.40–7.44 (m, 6H, 7-benzimidazole, H-7-phenyl hydrazone, H-2, H-3, H-4, H-5, H-6) 7.73 (s, 1H, benzimidazole, H-4), 7.90–7.92 (d, 1H, J = 8.00 Hz, phenyl H-3), 7.96–7.98 (d, 1H, J = 8.00 Hz, phenyl H-5), 8.01–8.03 (d, 1H, J = 8.00 Hz, phenyl H-2), 8.07–8.9 (d, 1H, J = 8.00 Hz, phenyl H-6), 8.11 (s, 1H CH
N), 11.80 (s, 1H, NH, D2O exchangeable), 13C NMR DEPQ (100 MHz DMSO-d6): δ 21.50 (CH3 aliphatic), 43.90 (CH2 aliphatic), 46.24 (SO2CH3), 110.76, 126.23, 128.18, 128.83, 129.40, 130.86, 131.15, 132.17, 132.18, 133.31, 133.56, 134.24, 135.18, 135.28, 135.41, 140.87, 140.96, 148.54, 152.01, 152.38, 168.68. Anal. calcd for C24H22N4O3S: C, 64.56; H, 4.97; N, 12.55; found: C, 64.72; H, 5.03; N, 12.79.
4.1.5.10 (E)-N′-(4-Chlorobenzylidene)-2-(5-methyl-2-(4-(methylsulfonyl) phenyl)-1H-benzo[d]imidazol-1-yl) acetohydrazide (11j). Brown solid; yield 48%; mp 165–167 °C; IR: (KBr, cm−1) 3410 (NH), 1697 (C
O), 1612 (C
N), 1322–1156 (SO2); 1HNMR (DMSO-d6, 400 MHz, δ ppm): 2.26 (s, 3H, CH3), 3.30 (s, 3H, SO2CH3), 5.58 (s, 2H, CH2), 7.31–7.33 (T, 1H, J = 8.00 Hz benzimidazole, H-6, H-7), 7.42–7.43 (T, 3H, J = 8.00 Hz phenyl hydrazone, H-3, H-4, H-5), 7.63–7.65 (d, 2H, J = 8.00 Hz benzimidazole, H-4), 7.77, −7.79 (d, 1H, J = 8.00 Hz, benzimidazole, H-7), 7.86–7.88 (d, 2H, J = 8.00 Hz, phenyl hydrazone H-2, H-6), 8.04–8.06 (d, 2H, J = 8.00 Hz, phenyl H-2, H-6), 8.10–8.12 (d, 2H, J = 8.00 Hz, phenyl H-3, H-5) 8.13 (S, 1H CH
N) 11.10 (s, 1H, NH, D2O exchangeable), 13C NMR (100 MHz DMSO-d6): δ 21.79 (CH3), 43.72 (CH2 aliphatic), 56.66, (SO2CH3), 110.96, 119.38, 125.89, 127.80, 129.23, 129.32, 129.42, 129.66, 129.67, 130.14, 130.18, 130.44, 130.49, 133.19, 135.03, 135.62, 140.97, 141.94, 143.88, 152.37, 168.97. Anal. calcd for C24H21ClN4O3S: C, 59.93; H, 4.40; N, 11.65; found: C, 60.16; H, 4.62; N, 11.38.
4.1.5.11 (E)-N′-(4-Methoxybenzylidene)-2-(5-methyl-2-(4-(methylsulfonyl) phenyl)-1H-benzo[d]imidazol-1-yl) acetohydrazide (11k). Off white; yield 53%; mp 170–172 °C; IR: (KBr, cm−1) 3411 (NH), 1670 (C
O), 1615 (C
N), 1322–1157 (SO2); 1HNMR (DMSO-d6, 400 MHz, δ ppm): 1.13 (s, 3H, CH3), 2.44 (s, 3H, CH3), 3.26 (s, 3H, SO2CH3), 5.49 (s, 2H, CH2), 7.14–7.16 (d, 1H, J = 8.00 Hz, benzimidazole, H-6), 7.40–7.42 (m, 4H, phenyl hydrazone, H-2, H-3, H-5, H-6), 7.49 (s, 1H, benzimidazole, H-4), 7.73–7.75 (d, 1H, J = 8.00 Hz, benzimidazole, H-7), 8.00–8.02 (d, 1H, J = 8.00 Hz, phenyl H-2, H-6), 8.06–8.08 (d, 1H, J = 8.00 Hz, phenyl H-3, H-5), 8.10 (s, 1H CH
N), 11.86 (s, 1H, NH, D2O exchangeable). 13C NMR DEPQ (100 MHz DMSO-d6): δ 21.78 (CH3 aliphatic), 43.35 (CH2 aliphatic), 46.24 (SO2CH3), 46.80, 65.56, 61.36, 62.02, 119.52, 124.80, 127.44, 128.17, 129.90, 132.22, 133.19, 133.45, 133.57, 135.09, 135.37, 135.48, 138.67, 140.90, 141.68, 151.98, 152.34, 163.06. Anal. calcd for C25H24N4O4S: C, 63.01; H, 5.08; N, 11.76; found: C, 63.17; H, 5.19; N, 12.02.
4.1.5.12 (E)-N′-(4-Fluorobenzylidene)-2-(5-methyl-2-(4-(methylsulfonyl) phenyl)-1H-benzo[d]imidazol-1-yl) acetohydrazide (11l). Buff solid; yield 57%; mp 166–168 °C; IR: (KBr, cm−1) 3409 (NH), 1696 (C
O), 1618 (C
N), 1325, 1156 (SO2); 1HNMR (DMSO-d6, 400 MHz, δ ppm): 2.44 (s, 3H, CH3), 3.26 (s, 3H, SO2CH3), 5.56 (s, 2H, CH2), 7.14–7.16 (d, 1H, J = 8.00 Hz, benzimidazole, H-6), 7.27 (s, 1H, benzimidazole, H-4) 7.40–7.42 (d, 2H, J = 8.00 Hz, phenyl hydrazone, H-2, H-6), 7.63, −7.65 (d, 1H, J = 8.00 Hz, benzimidazole, H-7), 7.78–7.8 (d, 2H, J = 8.00 Hz, phenyl hydrazone, H-3, H-5), 7.99–8.01 (d, 2H, J = 8.00 Hz, phenyl H-3, H-5), 8.06–8.08 (d, 2H, J = 8.00 Hz, phenyl H-2, H-6), 8.10 (s, 1H CH
N), 11.78 (s, 1H, NH, D2O exchangble), 13C NMR DEPQ (100 MHz DMSO-d6): δ 21.80 (CH3 aliphatic), 43.56 (CH2 aliphatic), 46.06 (SO2CH3), 49.06, 56.28, 61.93, 110.88, 116.16, 116.37, 116.84, 119.54, 127.80, 129.72, 130.20, 130.75, 132.76, 136.13, 137.45, 140.99, 141.99, 144.20, 151.77, 168.63, 191.90. Anal. calcd for C24H21FN4O3S: C, 62.06; H, 4.56; N,12.06; found: C, 62.28; H, 4.72; N, 12.31.
4.1.6. General procedure for the synthesis of (12a–f). A mixture of compounds 10a, 10b or 10c (1.45 mmol) and appropriate aromatic ketones (1.45 mmol) in ethyl alcohol (25 ml) was refluxed for 6 h in the presence of glacial acetic acid in a catalytic. The product recrystallized from ethanol.38
4.1.6.1 (E)-2-(2-(4-(Methylsulfonyl) phenyl)-1H-benzo[d]imidazol-1-yl)-N′-(1-phenylethylidene) acetohydrazide (12a). Off white solid; yield 61%; mp 170–172 °C; IR: (KBr, cm−1) 3405 (NH), 1697 (C
O), 1613 (C
N), 1319–1156 (SO2); 1HNMR (DMSO-d6, 400 MHz, δ ppm): 2.3 (s, 3H, CH3), 3.31 (s, 3H, SO2CH3), 5.64 (s, 2H, CH2), 7.30–7.32 (t, 2H, benzimidazole H-5, H-6), 7.40–7.42 (d, 2H, J = 8.00 Hz, benzimidazole H-4, H-7), 7.76–7.78 (d, 1H, J = 8.00 Hz, phenyl hydrazone H-3), 7.85–7.87 (d, 2H, J = 8.00 Hz, phenyl hydrazone H-2, H-6), 7.88–8.00 (d, 1H, J = 8.00 Hz, phenyl hydrazone H-5), 8.03–8.05 (d, 2H, J = 8.00 Hz, phenyl H-2, H-6), 8.09–8.11 (d, 2H, J = 8.00 Hz, phenyl H-3, H-5), 11.08 (s, 1H, NH, D2O exchangeable). Anal. calcd for C24H22N4O3S: C, 64.56; H, 4.97; N, 12.55; found: C, 64.70; H, 5.14; N, 12.38.
4.1.6.2 (E)-N′-(1-(4-Chlorophenyl) ethylidene)-2-(2-(4-(methylsulfonyl) phenyl)-1H-benzo[d]imidazol-1-yl) acetohydrazide (12b). Dark brown solid; yield 62%; mp 167–169 °C; IR: (KBr, cm−1) 3407 (NH), 1697 (C
O), 1615 (C
N), 1321–1157 (SO2); 1HNMR (DMSO-d6, 400 MHz, δ ppm): 2.29 (s, 3H, CH3), 3.29 (s, 3H, SO2CH3), 5.59 (s, 2H, CH2), 7.3–7.32 (d, 2H, J = 8.00 Hz, benzimidazole, H-5, H-6), 7.46–7.48 (d, 2H, J = 8.00 Hz, benzimidazole, H-4, H-7), 7.62–7.64 (d, 1H, J = 8.00 Hz, phenyl hydrazone H-2), 7.77–7.79 (d, 1H, J = 8.00 Hz, phenyl hydrazone, H-6), 7.86–7.78 (d, 2H, J = 8.00 Hz, phenyl hydrazone, H-3, H-5), 8.04–8.06 (d, 2H, J = 8.00 Hz, phenyl H-2, H-6), 8.09–8.11 (d, 2H, J = 8.00 Hz, phenyl H-3, H-5), 11.09 (s, 1H, NH, D2O exchangeable), 13C NMR (100 MHz DMSO-d6): δ 13.90 (CH3), 43.71 (CH2 aliphatic), 49.20, (SO2CH3), 111.55, 119.83, 123.75, 124.51, 125.86, 127.88, 128.64, 128.82, 128.96, 129.28, 130.23, 130.55, 134.51, 135.53, 137.10, 137.26, 141.99, 142.82, 148.77, 152.53, 169.60. Anal. calcd for C24H21 ClN4O3S: C, 59.93; H, 4.40; N, 11.65; found: C, 60.21; H, 4.35; N, 11.92.
4.1.6.3 (E)-2-(5-Chloro-2-(4-(methylsulfonyl) phenyl)-1H-benzo[d]imidazol-1-yl)-N′-(1-phenylethylidene) acetohydrazide (12c). Buff solid; yield58%; mp 168–170 °C; IR: (KBr, cm−1) 3402 (NH), 1695 (C
O), 1609 (C
N), 1317–1148 (SO2); 1HNMR (DMSO-d6, 400 MHz, δ ppm): 2.29 (s, 3H, CH3), 3.29 (s, 3H, SO2CH3), 5.57 (s, 2H, CH2), 7.34–7.36 (d, 1H, benzimidazole, H-6), 7.41–7.43 (t, 3H, phenyl hydrazone, H-3, H-4, H-5), 7.77–7.79 (d, 1H, J = 8.00 Hz, benzimidazole, H-7), 7.85–7.87 (d, 2H, J = 8.00 Hz, phenyl hydrazone, H-2, H-6), 7.91 (s, 1H, benzimidazole, H-4) 8.01–8.06 (m, 2H, phenyl H-2, H-6), 8.09–8.11 (d, 2H, J = 8.00 Hz, phenyl H-3, H-5) 11.07 (s, 1H, NH, D2O exchangeable), 13C NMR (100 MHz DMSO-d6): δ 14.33 (CH3), 43.94 (CH2 aliphatic), 46.86, (SO2CH3), 111.54, 112.91, 119.20, 121.20, 123.14, 123.66, 126.65, 127.84, 127.90, 128.15, 128.83, 129.33, 129.81, 130.24, 130.31, 132.17, 135.11, 138.32, 141.61, 142.23, 169.29. Anal. calcd for C24H21ClN4O3S: C, 59.93; H, 4.40; N, 11.65; found: C, 60.14; H, 4.63; N, 11.88.
4.1.6.4 (E)-2-(5-Chloro-2-(4-(methylsulfonyl) phenyl)-1H-benzo[d]imidazol-1-yl)-N′-(1-(4-chlorophenyl) ethylidene) acetohydrazide (12d). Brown solid; yield 49%; mp 175–177 °C; IR: (KBr, cm−1) 3407 (NH), 1699 (C
O), 1616 (C
N), 1321–1159 (SO2); 1HNMR (DMSO-d6, 400 MHz, δ ppm): 2.25 (s, 3H, CH3), 3.25 (s, 3H, SO2CH3), 5.69 (s, 2H, CH2), 7.31–7.33 (d, 1H, J = 8.00 Hz, benzimidazole, H-6), 7.46–7.48 (d, 2H, J = 8.00 Hz, benzoimidazole, H-4, H-7), 7.88–7.90 (d, 2H, J = 8.00 Hz, phenyl hydrazone, H-2, H-6), 8.03–8.05 (d, 2H, J = 8.00 Hz, phenyl hydrazone, H-3,H-5), 8.05–8.11 (m, 4H, J = 8.4 Hz, phenyl, H-2, H-3, H5, H-6), 11.18 (s, 1H, NH, D2O exchangeable). 13C NMR (100 MHz DMSO-d6): δ 14.33 (CH3), 43.56 (CH2 aliphatic), 46.64, (SO2CH3), 119.02, 120.81, 123.29, 123.90, 127.81, 127.91, 128.66, 128.68.128.80, 128.87, 130.23, 130.27, 130.26, 130.71, 134.14, 136.86, 142.12, 143.56, 150.40, 153.33, 169.22. Anal. calcd for C24H20 Cl2N4O3S: C, 55.93; H, 3.91; N, 10.87; found: C, 56.25; H, 4.06; N, 11.06.
4.1.6.5 (E)-2-(5-Methyl-2-(4-(methylsulfonyl) phenyl)-1H-benzo[d]imidazol-1-yl)-N′-(1-phenylethylidene) acetohydrazide (12e). Off white; yield 56%; mp 173–175 °C; IR: (KBr, cm−1) 3409 (NH), 1699 (C
O), 1618 (C
N), 1325–1159 (SO2); 1HNMR (DMSO-d6, 400 MHz, δ ppm): 2.7 (s, 3H, CH3), 2.89 (s, 3H, CH3), 3.30 (s, 3H, SO2CH3), 6.16 (s, 2H, CH2), 7.29–7.31 (d, 1H, J = 8.00 Hz, benzimidazole, H-6), 7.61 (s, 1H, benzoimidazole, H-4), 7.68–7.7 (d, 1H, J = 8.00 Hz, benzoimidazole, H-7), 7.96–7.98 (d, 2H, J = 8.00 Hz, phenyl hydrazone, H-2, H-6), 8.04–8.06 (t, 3H, phenyl hydrazone, H-3, H-4, H-5), 8.11–8.13 (d, 2H, J = 8.00 Hz, phenyl, H-2, H-6), 8.43–8.45 (d, 2H, J = 8.00 Hz, phenyl, H-3H-, H-5), 13.22 (s, 1H, NH, D2O exchangeable). 13C NMR (100 MHz DMSO-d6): δ 31.35 (CH3 aliphatic), 36.26 (CH3 aliphatic), 43.73 (CH3 aliphatic), 51.76 (SO2CH3), 111.56, 114.84, 123.04, 123.67, 127.55, 127.92, 128.22, 129.36, 129.53, 130.14, 130.69, 130.83, 133.15, 135.06, 135.28, 139.79, 141.80, 142.01, 149.86, 162.68, 193.17. Anal. calcd for C25H24N4O3S: C, 65.20; H, 5.25; N, 12.17; found: C, 65.52; H, 4.89; N, 12.53.
4.1.6.6 (E)-N′-(1-(4-Chlorophenyl) ethylidene)-2-(5-methyl-2-(4-(methylsulfonyl) phenyl)-1H-benzo[d]imidazol-1-yl) acetohydrazide (12f). Pale brown; yield 46%; mp 180–182 °C; IR: (KBr, cm−1) 410 (NH), 1699 (C
O), 1620 (C
N), 1325–1160 (SO2); 1HNMR (DMSO-d6, 400 MHz, δ ppm): 2.26 (s, 3H, CH3), 2.47 (s, 3H, CH3), 3.28 (s, 3H, SO2CH3), 5.64 (s, 2H, CH2), 7.14–7.16 (d, 1H, J = 8.00 Hz, benzimidazole, H-6), 7.43–7.45 (m, 2H, benzoimidazole, H-4, H-7), 7.56–7.57 (d, 1H, J = 8.00 Hz, phenyl hydrazone, H-2), 7.65–7.67 (d, 1H, J = 8.00 Hz, phenyl hydrazone, H-6), 7.86–7.88 (d, 1H, J = 8.00 Hz, phenyl hydrazone, H-3), 7.93–7.95 (d, 1H, J = 8.00 Hz, phenyl hydrazone, H-5), 8.03–8.05 (d, 2H, J = 8.00 Hz, phenyl, H-2, H-, H-6), 8.09–8.11 (d, 2H, J = 8.00 Hz, phenyl, H-3, H-5), 11.12 (s, 1H, NH, D2O exchangble), 13C NMR (100 MHz DMSO-d6): δ 14.32 (CH3 aliphatic), 21.78 (CH3 aliphatic), 43.71 (CH2 aliphatic), 46.63 (SO2CH3), 110.90, 119.50, 124.51, 125.09, 126.12, 126.80, 127.89, 128.56, 128.78, 129.17, 130.15, 130.19, 130.21, 130.44, 134.57, 136.99, 137.16, 138.56, 142.01, 152.42, 169.38. Anal. calcd for C25H23ClN4O3S: C, 60.66; H, 4.68; N, 11.32; found: C, 60.83; H, 4.92; N, 11.57.
4.2. Biological activity
4.2.1. In vitro cyclooxygenase (COX) inhibition assay. An enzyme immune assay (EIA) kit (Cayman Chemical, Ann Arbor, MI, USA) was used to determine the ability of the targeted compounds shown in Table 1 to inhibit both ovine COX-1 and COX-2 following a previously reported method.32
4.2.2. In vivo anti-inflammatory (AI) assay. Carrageenan induced paw edema model was used in accordance with Nahda University guidelines and was approved by the ethical committee of Nahda University, Beni-Suef, Egypt (approval no. NUB-024-21). The tested compounds 11a–11d, 11f–11h, 11j–11l, 12a, 12b and 12c as well as celecoxib and indomethacin as reference drugs were evaluated for their in vivo anti-inflammatory activities. The first group (negative control) received 5% DMSO aqueous solution (v/v), the second group received celecoxib as a reference drug (10 mg kg−1; po), the third group received indomethacin as a reference drug (10 mg kg−1; po) and the other groups received tested compounds (10 mg kg−1; po) in form of 5% DMSO aqueous solution. The induction of inflammation started 30 minutes after receiving the treatment. Carrageenan (0.02 ml of 1%) was injected sub-plantar to induce paw edema. Paw thickness was measured 1 hour, 3 hours, and 6 hours after the carrageenan injection according to the following equation, edema inhibition (%) = (Tc − Tt/Tc) × 100 where Tt represents the mean increase in paw thickness in rats treated with the tested compound; Tc represents the mean increase in paw thickness in rats of the control group using a previously described method.32 Percent of inhibition for the most active drugs in addition to celecoxib and indomethacin is listed in Table 2.
4.2.3. Ulcerogenic liability. Ulcerogenic effect (ulcer index), number of ulcers, and preventive index were studied for the most potent anti-inflammatory drugs 11b, 12d and 11k compared to those for indomethacin and celecoxib. After 4 h of drug administration, rats were sacrificed. Findings have shown satisfactory results of the tested drug against the reference drugs celecoxib and indomethacin following a previously mentioned method.34
4.2.4. Histo-pathological study for in vivo ulcer liability. 10% buffered formalin was used to flush glandular and non-glandular collected stomach samples for 72 h. Collected samples encountered preparing processes such as trimming, dehydration by different concentrations of alcohol, clearing in xylene and blocking out into paraplast tissue. Rotatory microtome was used to obtain 5μ sections, which were stained by harris hematoxylin and Eosin as a general method of staining then outlined by Bancroft and Stevens according to a previously reported method.35
4.3. Molecular docking study
‘Molecular Operating Environment 2020.01’ software (MOE of Chemical Computing Group Inc., on a Core i7 2.2 GHz workstation) running on a Windows 10 PC which was used to carry out all docking studies and molecular modeling calculations. X-ray crystal structure of COX-2 complexed with a selective inhibitor, celecoxib at 3 Å resolution. The most active compounds as well as celecoxib were docked against COX-2 crystalline structure data obtained from the protein data bank (PDB ID: 1CX2). The MOE software was used to conduct the docking analysis (chemical computing group software, Canada 2020.01). After protonation, the lowest energy conformer of the tested compounds was docked to the active site. The docking conditions for all molecules were the same as for standard drugs. The 2D and 3D pictures are used to study the docking score and the binding interaction.36 The docking phase of the tested compounds reveals that the active pharmacophore and amino acid active site interact well.
Author contributions
HMA-R and KRAA designed the idea, and the protocol of the whole study. MASB synthesized the compounds and wrote the experimental parts. MASB, HMA-R and EKAA interpreted the spectral data and modeling study. EE carried out a histopathological study. All authors read and approved the final manuscript.
Conflicts of interest
There are no conflicts of interest to declare.
References
- M. Gaba, Design, Synthesis and Biological Evaluation of Novel 1, 2, 5-Substituted Benzimidazole Derivatives as Gastroprotective Anti-inflammatory and Analgesic Agents, Med, Chem, 2015, 5(2), 058–063 Search PubMed.
- K. Pavelka, Symptomatic treatment of osteoarthritis: paracetamol or NSAIDs?, Int. J. Clin. Pract, 2004, 58, 5–12 CrossRef PubMed.
- A. M. Shaker, E. K. Abdelall, K. R. Abdellatif and H. M. Abdel-Rahman, Synthesis and biological evaluation of 2-(4-methylsulfonyl phenyl) indole derivatives: multi-target compounds with dual antimicrobial and anti-inflammatory activities, BMC Chem, 2020, 14, 1–15 CrossRef PubMed.
- G. Carullo, F. Galligano and F. Aiello, Structure–activity relationships for the synthesis of selective cyclooxygenase 2 inhibitors: an overview (2009–2016), Med. Chem. Commun, 2017, 8, 492–500 RSC.
- K. R. Abdellatif, P. F. Lamie and H. A. Omar, 3-Methyl-2-phenyl-1-substituted-indole derivatives as indomethacin analogs: design, synthesis and biological evaluation as potential anti-inflammatory and analgesic agents, J. Enzyme Inhib. Med, Chem, 2016, 31, 318–324 CAS.
- D. A. Al-Turki, M. A. Al-Omar, L. A. Abou-Zeid, I. A. Shehata and M. S. Al-Awady, Design, synthesis, molecular modeling and biological evaluation of novel diaryl heterocyclic analogs as potential selective cyclooxygenase-2 (COX-2) inhibitors, Saudi Pharm J, 2017, 25, 59–69 CrossRef PubMed.
- J. Kaur, A. Bhardwaj, Z. Huang and E. E. Knaus, N-1 and C-3 substituted indole Schiff bases as selective COX-2 inhibitors: synthesis and biological evaluation, Bioorganic Med. Chem. Lett, 2012, 22, 2154–2159 CrossRef CAS PubMed.
- M. E. Turini and R. N. DuBois, Cyclooxygenase-2: a therapeutic target, Annu. Rev. Med., 2002, 35–57 CrossRef CAS PubMed.
- V. K. Redasani and S. B. Bari, Synthesis and evaluation of mutual prodrugs of ibuprofen with menthol, thymol and eugenol, Eur, J. Med. Chem., 2012, 56, 134–138 CAS.
- J. L. Masferrer, B. S. Zweifel, P. T. Manning, S. D. Hauser, K. M. Leahy, W. G. Smith, P. C. Isakson and K. Seibert, Selective inhibition of inducible cyclooxygenase 2 in vivo is antiinflammatory and nonulcerogenic, Proc. Natl. Acad. Sci. U.S.A, 1994, 91, 3228–3232 CrossRef CAS PubMed.
- N. H. Amin, A. A. Mohammed and K. R. Abdellatif, Novel 4-methylsulfonylphenyl derivatives as NSAIDS with preferential COX-2 inhibition, Future Med, Chem, 2018, 10, 53–70 CAS.
- S. K. Singh, S. Vobbalareddy, S. Shivaramakrishna, A. Krishnamraju, S. A. Rajjak, S. R. Casturi and V. Akhila, Y.K. Rao, Methanesulfonamide group at position-4 of the C-5-phenyl ring of 1, 5-diarylpyrazole affords a potent class of cyclooxygenase-2 (COX-2) inhibitors, Bioorg. Med. Chem. Lett., 2004, 14, 1683–1688 CrossRef CAS PubMed.
- M. I. García-Aranda, J. E. Gonzalez-Padilla, C. Z. Gómez-Castro, Y. M. Gómez-Gómez, M. C. Rosales-Hernández, E. V. García-Báez, M. O. Franco-Hernández, J. L. Castrejón-Flores and I. I. Padilla-Martínez, Anti-inflammatory effect and inhibition of nitric oxide production by targeting COXs and iNOS enzymes with the 1, 2-diphenylbenzimidazole pharmacophore, Bioorg. Med. Chem., 2020, 28, 115427 CrossRef PubMed.
- M. Biava, G. C. Porretta, G. Poce, S. Supino, S. Forli, M. Rovini, A. Cappelli, F. Manetti, M. Botta and L. Sautebin, Cyclooxygenase-2 inhibitors. 1, 5-diarylpyrrol-3-acetic esters with enhanced inhibitory activity toward cyclooxygenase-2 and improved cyclooxygenase-2/cyclooxygenase-1 selectivity, J. Med. Chem., 2007, 50, 5403–5411 CrossRef CAS PubMed.
- K. Taniguchi, S. Shigenaga, T. Ogahara, T. Fujitsu and M. Matsuo, Synthesis and antiinflammatory and analgesic properties of 2-amino-1H-benzimidazole and 1, 2-dihydro-2-iminocycloheptimidazole derivatives, Chem. Pharm. Bull., 1993, 41, 301–309 CrossRef CAS PubMed.
- S.-Y. Hong, K.-W. Kwak, C.-K. Ryu, S.-J. Kang and K.-H. Chung, Antiproliferative effects of 6-anilino-5-chloro-1H-benzo [d] imidazole-4, 7-dione in vascular smooth muscle cells, Bioorg. Med. Chem., 2008, 16, 644–649 CrossRef PubMed.
- L. I. Kruse, D. L. Ladd, P. B. Harrsch, F. L. McCabe, S. M. Mong, L. Faucette and R. Johnson, Synthesis, tubulin binding, antineoplastic evaluation, and structure-activity relationship of oncodazole analogs, J. Med. Chem., 1989, 32, 409–417 CrossRef CAS PubMed.
- Ö. Ö. Güven, T. Erdoğan, H. Göker and S. Yıldız, Synthesis and antimicrobial activity of some novel phenyl and benzimidazole substituted benzyl ethers, Bioorganic Med. Chem. Lett, 2007, 17, 2233–2236 CrossRef PubMed.
- Z. Ates-Alagoz, S. Yildiz and E. Buyukbingol, Antimicrobial activities of some tetrahydronaphthalene-benzimidazole derivatives, Chemotherapy, 2007, 53, 110–113 CrossRef CAS PubMed.
- M. M. Morcoss, M. El Shimaa, R. A. Ibrahem, H. M. Abdel-Rahman, M. Abdel-Aziz and D. A. Abou El-Ella, Design, synthesis, mechanistic studies and in silico ADME predictions of benzimidazole derivatives as novel antifungal agents, Bioorg. Chem., 2020, 101, 103956 CrossRef CAS PubMed.
- D. Sharma, B. Narasimhan, P. Kumar and A. Jalbout, Synthesis and QSAR evaluation of 2-(substituted phenyl)-1H-benzimidazoles and [2-(substituted phenyl)-benzimidazol-1-yl]-pyridin-3-yl-methanones, Eur, J. Med. Chem., 2009, 44, 1119–1127 CAS.
- J. Y. Xu, Y. Zeng, Q. Ran, Z. Wei, Y. Bi, Q. H. He, Q. J. Wang, S. Hu and J. Zhang, M.Y. Tang, Synthesis and biological activity of 2-alkylbenzimidazoles bearing a N-phenylpyrrole moiety as novel angiotensin II AT1 receptor antagonists, Bioorganic Med. Chem. Lett, 2007, 17, 2921–2926 CrossRef CAS PubMed.
- K. Kubo, Y. Kohara, Y. Yoshimura, Y. Inada, Y. Shibouta, Y. Furukawa, T. Kato, K. Nishikawa and T. Naka, Nonpeptide angiotensin II receptor antagonists. Synthesis and biological activity of potential prodrugs of benzimidazole-7-carboxylic acids, J. Med. Chem., 1993, 36, 2343–2349 CrossRef CAS PubMed.
- V. Klimešová, J. Kočí, M. Pour, J. Stachel, K. Waisser and J. Kaustová, Synthesis and preliminary evaluation of benzimidazole derivatives as antimicrobial agents, Eur, J. Med. Chem., 2002, 37, 409–418 Search PubMed.
- I. N. Shaikh, K. M. Hosamani, H. R. Seetharamareddy and M. H. Hugar, Synthesis and In-vivo Evaluation of Carbonyl-amide Linkage Based New Benzimidazole Derivatives, Arch. Pharm., 2012, 345, 65–72 CrossRef CAS PubMed.
- H. Göker, M. Tunçbilek, G. Ayhan and N. Altanlar, Synthesis of some new benzimidazolecarboxamides and evaluation of their antimicrobial activity, Farmaco Sci, 1998, 53, 415–420 CrossRef.
- J. Horn, The proton-pump inhibitors: similarities and differences, Clin. Ther., 2000, 22, 266–280 CrossRef CAS PubMed.
- C. Kuş, G. Ayhan-Kılcıgil, S. Özbey, F. B. Kaynak, M. Kaya, T. Çoban and B. Can-Eke, Synthesis and antioxidant properties of novel N-methyl-1, 3, 4-thiadiazol-2-amine and 4-methyl-2H-1, 2, 4-triazole-3 (4H)-thione derivatives of benzimidazole class, Bioorg. Med. Chem., 2008, 16, 4294–4303 CrossRef PubMed.
- R. A. Elrayess, N. Ghareb, M. M. Azab and M. M. Said, Synthesis and antimicrobial activities of some novel benzimidazole and benzotriazole derivatives containing β-lactam moiety, Life Sci. J, 2013, 10, 1784–1793 Search PubMed.
- K. Ansari and C. Lal, Synthesis, physicochemical properties and antimicrobial activity of some new benzimidazole derivatives, Eur, J. Med. Chem., 2009, 44, 4028–4033 CAS.
- R. A. Mekheimer, A. M. A. Hameed and K. U. Sadek, First synthesis and isolation of the E-and Z-isomers of some new Schiff bases. Reactions of 6-azido-5-formyl-2-pyridone with aromatic amines, Molecules, 2008, 13, 195–203 CrossRef CAS PubMed.
- B. Roschek Jr, R. C. Fink, D. Li, M. McMichael, C. M. Tower, R. D. Smith and R. S. Alberte, Pro-inflammatory enzymes, cyclooxygenase 1, cyclooxygenase 2, and 5-lipooxygenase, inhibited by stabilized rice bran extracts, J. Med. Food, 2009, 12, 615–623 CrossRef PubMed.
- K. R. Abdellatif, P. F. Lamie and H. A. Omar, 3-Methyl-2-phenyl-1-substituted-indole derivatives as indomethacin analogs: design, synthesis and biological evaluation as potential anti-inflammatory and analgesic agents, J. Enzyme Inhib. Med. Chem., 2016, 31, 318–324 CrossRef CAS PubMed.
- A. Robert, J. E. Nezamis and J. P. Phillips, Effect of prostaglandin E1 on gastric secretion and ulcer formation in the rat, Gastroenterology, 1968, 55, 481–487 CrossRef CAS.
- J. D. Bancroft and M. Gamble, Theory and Practice of Histological Techniques, North Hollywood, USA, 6th edn, 2008 Search PubMed.
- R. G. Kurumbail, A. M. Stevens, J. K. Gierse, J. J. McDonald, R. A. Stegeman, J. Y. Pak, D. Gildehaus, T. D. Penning, K. Seibert and P. C. Isakson, Structural basis for selective inhibition of cyclooxygenase-2 by anti-inflammatory agents, Nature, 1996, 384, 644–648 CrossRef CAS PubMed.
- A. Dubey, S. Srivastava and S. Srivastava, Conventional and microwave assisted synthesis of 2-oxo-4-substituted aryl-azetidine derivatives of benzotriazole: A new class of biological compounds, Bioorganic Med. Chem. Lett, 2011, 21, 569–573 CrossRef CAS PubMed.
- N. Ö. Can, D. Osmaniye, S. Levent, B. N. Sağlık, B. Korkut, Ö. Atlı, Y. Özkay and Z. A. Kaplancıklı, Design, synthesis and biological assessment of new thiazolylhydrazine derivatives as selective and reversible hMAO-A inhibitors, Eur. J. Med. Chem., 2018, 144, 68–81 CrossRef CAS PubMed.
- F. Cheng, W. Li, Y. Zhou, J. Shen, Z. Wu, G. Liu, P. W. Lee and Y. Tang, admetSAR: a comprehensive source and free tool for assessment of chemical ADMET properties, ACS Publications, 2012, 3099–3105 CAS.
- H. Van De Waterbeemd and E. Gifford, ADMET in silico modelling: towards prediction paradise, Nat. Rev. Drug Discov, 2003, 2, 192–204 CrossRef CAS PubMed.
- E. Rajanarendar, S. R. Krishna, D. Nagaraju, K. G. Reddy, B. Kishore and Y. Reddy, Environmentally benign synthesis, molecular properties prediction and anti-inflammatory activity of novel isoxazolo [5, 4-d] isoxazol-3-yl-aryl-methanones via vinylogous Henry nitroaldol adducts as synthons, Bioorganic Med. Chem. Lett, 2015, 25, 1630–1634 CrossRef CAS PubMed.
- D. F. Veber, S. R. Johnson, H.-Y. Cheng, B. R. Smith, K. W. Ward and K. D. Kopple, Molecular properties that influence the oral bioavailability of drug candidates, J. Med. Chem., 2002, 45, 2615–2623 CrossRef CAS PubMed.
- https://www.molsoft.com/.
- http://www.swissadme.ch/index.php.
- http://biosig.unimelb.edu.au/pkcsm/.
- M. A. Mansour, A. M. AboulMagd and H. M. Abdel-Rahman, Quinazoline-Schiff base conjugates: in silico study and ADMET predictions as multi-target inhibitors of coronavirus (SARS-CoV-2) proteins, RSC Adv., 2020, 10, 34033–34045 RSC.
- S. Yamashita, T. Furubayashi, M. Kataoka, T. Sakane, H. Sezaki and H. Tokuda, Optimized conditions for prediction of intestinal drug permeability using Caco-2 cells, Eur. J. Pharm. Sci., 2000, 10, 195–204 CrossRef CAS PubMed.
- D. Huang, F. Qiu, Z. Zhang, L. Shi, C. Cao and S. Ke, Synthesis and Antifungal Activity of Substituted 2-Aryl Benzimidazoles Derivatives, J. Heterocycl. Chem., 2019, 56, 2494–2498 CrossRef CAS.
- https://www.rcsb.org/structure/1CX2.
Footnote |
† Electronic supplementary information (ESI) available. See DOI: 10.1039/d1ra04756f |
|
This journal is © The Royal Society of Chemistry 2021 |
Click here to see how this site uses Cookies. View our privacy policy here.