DOI:
10.1039/D1RA02528G
(Paper)
RSC Adv., 2021,
11, 17755-17759
Highly-efficient red-to-yellow/green upconversion sensitized by phthalocyanine palladium with high triplet energy-level†
Received
31st March 2021
, Accepted 4th May 2021
First published on 17th May 2021
Abstract
Soluble 3,7,11,15-tetra(tert-butyl)phthalocyanine palladium (TBPcPd) and 3,7,11,15-tetra(pentyloxy)phthalocyanine palladium (POPcPd) were synthesized and employed as sensitizers in expectation of achieving red-to-yellow/green upconversion (UC), doped with rubrene (Rub) and 9,10-bis(phenylethynyl)anthracene (BPEA), respectively. Under excitation of a 655 nm diode laser (∼1.5 W cm−2), a maximum red-to-green UC efficiency of 0.07% and a maximum red-to-yellow UC efficiency of 8.03% were obtained and the latter can drive a Si-photodiode to generate obvious photocurrent. The results showed that although a large triplet energy-level difference (ΔE = 3Esen. − 3Eanni.) of the sensitizer (3Esen.)/annihilator (3Eanni.) pair helps to improve the upconversion, the sensitizer/annihilator pair with a ΔE value less than zero still works. However, when the ΔE ≤ −0.05 eV, this bicomponent pair is not valid anymore. Thus, a comparison of the ΔE value can predict whether the sensitizer/annihilator pair is useful, which presents a quantitatively evaluated approach for exploring new-type upconversion systems for the first time.
1 Introduction
Triplet–triplet annihilation upconversion (TTA-UC), which is the observation of emission at higher energy (shorter wavelength) under the excitation of lower energy (longer wavelength), has attracted much attention owing to its potential applications for TTA-UC integrated dye-sensitized solar cells (DSSC),1–7 and photoelectrochemistry.8,9 Concretely, TTA-UC is facilitated by selective excitation of a strongly absorbing sensitizer in a longer wavelength region that internally converts to the triplet excited state; the triplet–triplet energy transfer (TTT) then occurs from the sensitizer to the annihilator and finally produces one single excited state between two annihilators (TTA), the latter thus emitting upconversion fluorescence.10 For the purpose of solar energy applications, the upconversion of the near infrared (NIR) to the visible light is attractive due to its making the best of harvesting the sunlight. Therefore, highly-efficient sensitizers in the NIR region are of importance. Fortunately, metallated phthalocyanine complexes (MPcs) are possibly one of the most suitable NIR triplet sensitizers because their Q bands of absorption are just right in the near infrared (NIR) region and have high molar absorbance coefficients.11 However, the MPcs as sensitizer are actually seldom reported yet.12 The reasons are possibly owing to the poor solubility of the MPcs without substitution groups; on the other hand, the soluble MPcs with long flexible alkyl chain substitution are needed heavy and complicated synthesis procedures.
In this work, three phthalocyanine complexes: phthalocyanine palladium (PcPd), 3,7,11,15-tetra(tert-butyl)phthalocyanine palladium (TBPcPd) and 3,7,11,15-tetra(pentyloxy)phthalocyanine palladium (POPcPd) were synthesized by one-pot method (see ESI section, Fig. S1–S8, ESI†). The results showed that phthalocyanine complexes (TBPcPd and POPcPd) with peripheral short alkyl chains are soluble in common organic solvents, while phthalocyanine palladium (PdPc) is insoluble. So, the two soluble TBPcPd and POPcPd were used as sensitizers, doped with rubrene (Rub) and 9,10-bis(phenylethynyl) anthracene (BPEA), respectively to obtain TBPcPd/Rub, TBPcPd/BPEA, POPcPd/Rub and POPcPd/BPEA pairs. Under the excitation of 655 nm diode laser, the UC efficiency and related triplet energy-level difference (ΔE = 3Esen. − 3Eanni.) of sensitizer (3Esen.)/annihilator (3Eanni.) pair were investigated.
2 Experimental section
2.1 Materials and upconversion solutions
All chemicals used in the synthesis, with the exception of usual solvents and regents, were purchased from Acros and Aldrich Co. and used without further purification. Annihilators rubrene (Rub) and 9,10-bis(phenylethynyl)anthracene (BPEA) were purchased from J&K Scientific Ltd. Palladium phthalocyanines (PcPd, TBPcPd and POPcPd) were synthesized and characterized in our lab (see ESI section†).
Four sensitizer/annihilator pairs (TBPcPd/Rub, TBPcPd/BPEA, POPcPd/Rub and POPcPd/EBPEA) with different concentration ratios were prepared in toluene and kept in the dark prior to measurements.
2.2 TTA-UC measurements
Absorption and fluorescence spectra were measured with a Hitachi U-3500 spectrophotometer and FLS920 Edinburgh fluorophotometer, respectively. The UC spectra was recorded with PR655 SpectraScan colorimeter under the excitation of 655 nm diode laser. The sensitizer/annihilator pair in toluene were degassed for 15 min before measurement. The UC efficiency (ΦUC) was calculated by eqn (1),10 |
 | (1) |
where Ar and As are the absorbance of the reference (ZnPc) and sensitizer at the excitation wavelength (655 nm), respectively. Fs and Fr stand for the integrated UC of annihilator and integrated fluorescence of ZnPc, respectively. Φr is the fluorescence quantum yield of ZnPc (20% in DMSO).13 ηs and ηr are the refractive index of sensitizer/annihilator solution and ZnPc solution (to simplify, ηs and ηr are the refractive index of toluene and DMSO). The equation is multiplied by a factor of 2, accounting for the fact that two absorbed photons are required to produce one up converted photon. Thus, the various parameters based on the eqn (1) above are presented in Table S1.†
3 Results and discussion
3.1 Absorption and photoluminescence
Molecular structures of palladium phthalocyanines (PcPd, TBPcPd and POPcPd) and annihilators (Rub and BPEA) are shown in Fig. 1. As can be seen that TBPcPd and POPcPd modified with substituents show better solubility, while PcPd without substituents is insoluble. Thus, both TBPcPd and POPcPd can act as sensitizers in toluene. As shown in Fig. 2, the maximum Q-bands of TBPcPd and POPcPd are located at 666 nm with the absorption edge near to 700 nm (TBPcPd) or beyond 700 nm (POPcPd). Relative to TBPcPd, POPcPd presents redshift both in absorption spectrum and fluorescence spectrum. We noticed that the phosphorescence peaks of TBPcPd and POPcPd are located at 1015 nm and 1026 nm, respectively (see Fig. 2, inset). Thus, the triplet energy levels of TBPcPd (3Esen = 1240/1015 nm) and POPcPd (3Esen = 1240/1026 nm) are calculated at 1.222 eV and 1.209 eV, respectively.
 |
| Fig. 1 Molecular structures of palladium phthalocyanines (PcPd, BPcPd and POPcPd) and annihilators (BPEA and Rub) as well as the photographs of three MPcs dispersed in toluene (10 μM). | |
 |
| Fig. 2 Absorption (left), fluorescence (right) and phosphorescence (inset) spectra of TBPcPd and POPcPd in toluene at 10 μM (fluorescence spectra were measured under the excitation of 655 nm Xe lamp while phosphorescence spectra (inset) were obtained under the excitation of 655 nm diode laser, N2 atmosphere and room temperature). | |
As for annihilator, BPEA exhibits dual absorption bands (438 nm and 457 nm) and two intensive fluorescence peaks located at 477 nm and 508 nm. Rub shows three absorption bands (460–560 nm) and fluorescence peaks at 556 nm and 600 nm (Fig. 3). All the optical properties of sensitizers (TBPcPd and POPcPd) and annihilators (Rub and BPEA) as well as the triplet energy-levels (3Esen. and 3Eanni.) are presented in Table 1.
 |
| Fig. 3 Absorbance and emission spectra of BPEA and Rub in toluene (10 μM) under the excitation of respective maximum absorption. | |
Table 1 The optical properties and the triplet energy-levels of sensitizers and annihilators (solvent: toluene, 10 μM)
Sensitizer |
λabs (nm) |
λp (nm) |
3Esen. (eV) |
TBPcPd |
333 (B), 632–660 (Q) |
1015 |
1.222 |
POPcPd |
332, 380 (B), 600–666 (Q) |
1026 |
1.209 |
Annihilator |
λabs (nm) |
λf (nm) |
Φf |
3Eanni. (eV) |
Rub |
460–526 |
556, 600 |
98% |
1.140 (ref. 14) |
BPEA |
438, 457 |
477, 508, 545 |
86% |
1.260 (ref. 15) |
3.2 TTA upconversion performances
Herein, we employed 655 nm red laser as the excitation light source due to lack of the NIR laser. When the TBPcPd/Rub and TBPcPd/BPEA pairs in degassed toluene were irradiated by the 655 nm diode laser (∼1.5 W cm−2), respectively, the red-to-yellow UC from TBPcPd/Rub (optimized concentration ratio: 14 μM/1.2 mM) pair and red-to-green UC from TBPcPd/BPEA (optimized concentration ratio: 10 μM/5 mM) pair were recorded (Fig. S9 and S10†). Power-dependent upconversion spectra (Fig. 4) showed that both upconversion show rapidly enhanced with the anti-Stokes shifts (EUC–Eex) at 0.32 eV for red-to-yellow (568 nm) (Fig. 4a) and at 0.58 eV for red-to-green (508 nm) (Fig. 4b). We noticed that the red-to-yellow UC intensity showed much stronger than red-to-green UC. The former efficiency (ΦUC) was calculated at 8.03% and later at 0.07% (Fig. S11 and Table S1, ESI†).
 |
| Fig. 4 Power-dependent upconversion of TBPcPd/Rub (a) and TBPcPd/BPEA (b) as well as the photographs with 655-filter (inset) in deaerated toluene at 655 nm excitation, ([TBPcPd]/[Rub]: 14 μM/1.2 mM, [TBPcPd]/[BPEA]: 10 μM/5 mM). | |
TTA-UC efficiency (ΦUC) is mainly dependent of two quantum processes: the triplet–triplet energy transfer (TTT) and the triplet–triplet annihilation (TTA). The TTT process can be evaluated by the Stern–Volmer equation: P0/P = Ksv[Q] + 1 = kq × 3τ × [Q] + 1,16 where P0/P is the ratio of the phosphorescence of sensitizer without and with annihilator. [Q] and Ksv are the molar concentration of annihilator and the Stern–Volmer constant, respectively. 3τ is the phosphorescence lifetime of sensitizer; kq is the quenching constant that can represent the TTT process.17 So, the phosphorescence intensity of TBPcPd quenched by Rub or BPEA, respectively, was measured (Fig. S12 and S13, ESI†) and the results are presented in Fig. 5a (curve i, ii). Clearly, TBPcPd/Rub pair possesses much larger Ksv value (5.26 mM−1) than TBPcPd/BPEA pair (3.09 mM−1). For the given sensitizer TBPcPd (3τ is the same), larger Ksv value means larger the quenching constant (kq) of the TTT process. Therefore, TTT process of TBPcPd/Rub pair is more efficient than TBPcPd/BPEA pair.
 |
| Fig. 5 Stern–Volmer curves (a) and the plots of upconversion integral versus power density (b) of TBPcPd/Rub, TBPcPd/BPEA and POPcPd/Rub upon excitation at 655 nm. | |
On the other hand, the triplet–triplet annihilation process (TTA) could be estimated by the plot of UC integral versus the excited power. As presented in Fig. 5b (curve i, ii), there are two different straight lines with slope ∼2 and ∼1. The former stands for the unsaturated stage of TTA while the later for the saturated one.18,19 The crossing point of both lines is defined as the threshold value (Ith),19,20 that is, the lowest excitation intensity is required for the most effective TTA process. Thus, the Ith value of TBPcPd/Rub pair (490 mW cm−2) is almost twice smaller than that of the TBPcPd/BPEA pair (1016 mW cm−2), indicating that the former possesses more efficient TTA than the latter. Clearly, both TTT (evaluated by Ksv) and TTA (evaluated by Ith) of BPcPd/Rub pair are more efficient than those of TBPcPd/BPEA pair.
Under the identical measurement conditions, POPcPd/Rub presents the red-to-yellow UC with the efficiency (ΦUC) at 1.84%, along with the Stern–Volmer constant (Ksv = 3.78 mM−1) and threshold excitation intensity (Ith = 550 mW cm−2), as shown in Fig. S14–S16, ESI,† Fig. 5a (curve iii) and Fig. 5b (curve iii). However, the POPcPd/BPEA pair has not been observed the upconversion phenomenon (Fig. S14, ESI†). All related UC performance parameters of different sensitizer/annihilator pairs are presented in Table 2.
Table 2 Parameters of different sensitizer/annihilator pairs in degassed toluene at the respective optimized concentration ratio
Sen./anni. |
λUC (nm) |
EUC–Eex (eV) |
KSV (mM−1) |
Ith (mW cm−2) |
ΔE (eV) |
ΦUC (%) |
TBPcPd/Rub |
568 |
0.32 |
5.65 |
490 |
0.082 |
8.03 |
POPcPd/Rub |
568 |
0.32 |
3.64 |
550 |
0.069 |
1.83 |
TBPcPd/BPEA |
508 |
0.58 |
2.83 |
1016 |
−0.038 |
0.07 |
POPcPd/BPEA |
— |
— |
— |
— |
−0.051 |
— |
Further analysis found that the UC efficiency (ΦUC) is strongly dominated by the triplet energy-level difference (ΔE = 3Esen. − 3Eanni.) of sensitizer (3Esen.)/annihilator (3Eanni.) pair, since sensitizer with higher 3Esen. value than annihilator (3Eanni.) can ensure the former triplet energy transfer top-down to the latter21,22 and then the subsequent TTA. As shown in Fig. 6a, under the excitation of 655 nm, the sensitizer TBPcPd reaches the triplet excited state (T1) via intersystem crossing (ISC). Since the 3Esen. value of TBPcPd (1.222 eV) is 0.082 eV higher than Rub (3Eanni. = 1.140 eV),14 this TTT process is efficient and resultly produces abundant triplet 3Rub* molecules for TTA process. Therefore, the UC efficiency of TBPcPd/Rub pair is as high as 8.03%. For POPcPd/Rub pair, the ΔE value (3Esen. − 3Eanni. = 1.209 eV − 1.14 eV) is at 0.069 eV (lower than the TBPcPd/Rub pair). Accordingly, the UC efficiency of POPcPd/Rub pair is dramatically reduced to 1.84% (Fig. 6b).
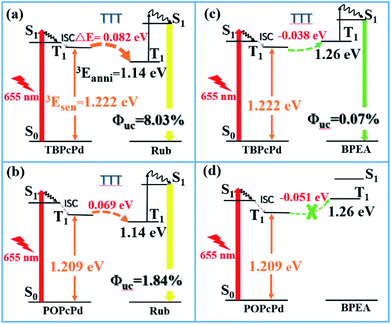 |
| Fig. 6 The influence of triplet energy-level difference (ΔE = 3Esen. − 3Eanni.) upon the TTA-UC efficiency ((a) TBPcPd/Rub; (b) POPcPd/Rub; (c) TBPcPd/BPEA; (d) POPcPd/BPEA). | |
Noted that the 3Esen. value of TBPcPd is lower than the 3Eanni. value of BPEA (3Eanni.), where such triplet–triplet energy transfer (TTT) can occur from the bottom up. As shown in Fig. 6c, the ΔE value of TBPcPd/BPEA pair is equal to −0.038 eV, the red-to-green upconversion can also be observed with the efficiency at 0.07%. However, when the ΔE value of POPcPd/BPEA pair is equal to −0.051 eV, the UC cannot happen anymore (Fig. 6d and S14, ESI†). Therefore, a comparison of the triplet energy-levels of sensitizer and annihilator can predict whether the upconversion system works, which can provide a simple evaluated approach for exploring the new-type upconversion sensitizers.
3.3 TTA-UC application
The potential prospect toward TTA-upconversion can be applied in upconversion-powered solar-cell. Here, the silicon diode (photosensitive area at 9 mm2) was integrated with red-to-yellow upconversion pair (TBPcPd/Rub solution, ΦUC = 8.03%) that is irradiated by a diode solid state laser (655 nm, ∼1.5 W cm−2). The yellow upconverted photons radiated by the TBPcPd/Rub solution can be absorbed by Si-diode that is placed near the quartz cuvette, as shown in Fig. 7 (inset). To confirm that the photocurrent of Si-diode was indeed induced by the yellow upconversion, a short-pass filter (<650 nm) was placed between the red laser and the quartz cuvette in order to eliminate wavelengths longer than 650 nm.
 |
| Fig. 7 Under excitation of 655 nm (∼1.5 W cm−2), I–V curve of TTA-UC powered Si-photodiode ((a) dark current curve, (b) the deaerated toluene, (c) TBPcPd solution alone in deaerated toluene, (d) the TBPcPd/Rub solution in deaerated toluene). Inset: the scheme of TTA upconversion-driven Si-photodiode. | |
Thus, the photocurrent curve generated by the TBPcPd/Rub solution can be recorded by a computer controlled Keithley-4200 power meter (as shown in Fig. 7, blue solid line (d)). As can be seen that the current density of short circuit (JSC) and voltage of open circuit (VOC) obtained from TBPcPd/Rub are at 9.7 mA cm−2 and 0.47 V, indicating that TTA red-to-yellow upconversion and solar-cell integration have potential applications.
4 Conclusions
In summary, we synthesized two soluble palladium phthalocyanine complexes (TBPcPd and POPcPd) acting as the triplet sensitizers, doped with different annihilator (Rub and BPEA), respectively. Under the excitation of 655 nm diode laser (∼1.5 W cm−2), the UC efficiencies of TBPcPd/Rub (red-to-yellow), TBPcPd/BPEA (red-to-yellow) and POPcPd/BPEA (red-to-green) are at 8.03%, 1.84% and 0.07%, respectively. The TBPcPd/Rub upconversion-powered solar-cell can produce the current density of short circuit (JSC) and voltage of open circuit (VOC) at 9.7 mA cm−2 and 0.47 V, respectively, indicating that TTA red-to-yellow upconversion and solar-cell integration have potential applications.
Meanwhile, it was found that although large triplet energy-level difference (ΔE = 3Esen. − 3Eanni.) of sensitizer (3Esen.)/annihilator (3Eanni.) pair helps to improve the upconversion, the sensitizer/annihilator pair still works when the ΔE value is less than zero and the bicomponent pair doesn't work anymore when the ΔE ≤ −0.05 eV. To our best knowledge, the current study is firstly presented quantitative criteria, which can provide a simple evaluated approach for exploring the new sensitizer/annihilator pairs.
Conflicts of interest
There are no conflicts to declare.
Acknowledgements
The authors are grateful to National Natural Science Foundation of China (51873145, 51803147, 51673143), Natural Science Foundation of Jiangsu Province-Excellent Youth Foundation (No. BK20170065), Qing Lan Project of Jiangsu Province of China, 333 High-level Talents Training Project of Jiangsu Province (No. BRA2018340) and Six Talent Summits Project of Jiangsu Province (No. XCL-79) for the financial supports.
Notes and references
- A. Nattestad, Y. Y. Cheng, R. W. MacQueen, T. F. Schulze, F. W. Thompson, A. J. Mozer, B. Fückel, T. Khoury, M. J. Crossley, K. Lips, G. G. Wallace and T. W. Schmidt, J. Phys. Chem. Lett., 2013, 4, 2073–2078 CrossRef CAS PubMed
. - V. Gray, D. Dzebo, M. Abrahamsson, B. Albinsson and K. Moth-Poulsen, Phys. Chem. Chem. Phys., 2014, 16, 10345–10352 RSC
. - T. F. Schulze, Y. Y. Cheng, B. Fückel, R. W. MacQueen, A. Danos, N. J. L. K. Davis, M. J. Y. Tayebjee, T. Khoury, R. G. C. R. Clady, N. J. Ekins-Daukes, M. J. Crossley, B. Stannowski, K. Lips and T. W. Schmidt, Aust. J. Chem., 2012, 65, 480–485 CrossRef CAS
. - T. F. Schulze, Y. Y. Cheng, T. Khoury, M. J. Crossley, B. Stannowski, K. Lips and T. W. Schmidt, J. Photonics Energy, 2013, 3, 034598 CrossRef CAS
. - T. F. Schulze, J. Czolk, Y.-Y. Cheng, B. Fückel, R. W. MacQueen, T. Khoury, M. J. Crossley, B. Stannowski, K. Lips, U. Lemmer, A. Colsmann and T. W. Schmidt, J. Phys. Chem. C, 2012, 116, 22794–22801 CrossRef CAS
. - L. Frazer, J. K. Gallaher and T. W. Schmidt, ACS Energy Lett., 2017, 2, 1346–1354 CrossRef CAS
. - T. Dilbeck and K. Hanson, J. Phys. Chem. Lett., 2018, 9, 5810–5821 CrossRef CAS PubMed
. - R. S. Khnayzer, J. Blumhoff, J. A. Harrington, A. Haefele, F. Deng and F. N. Castellano, Chem. Commun., 2012, 48, 209–211 RSC
. - B. Wang, B. Sun, X. Wang, C. Ye, P. Ding, Z. Liang, Z. Chen, X. Tao and L. Wu, J. Phys. Chem. C, 2014, 118, 1417–1425 CrossRef CAS
. - T. N. Singh-Rachford and F. N. Castellano, Coord. Chem. Rev., 2010, 254, 2560–2573 CrossRef CAS
. - X.-F. Zhang and W. Guo, J. Photochem. Photobiol., A, 2011, 225, 117–124 CrossRef CAS
. - Y. Che, W. Yang, G. Tang, F. Dumoulin, J. Zhao, L. Liu and Ü. İşci, J. Mater. Chem. C, 2018, 6, 5785–5793 RSC
. - A. Ogunsipe, J.-Y. Chen and T. Nyokong, New J. Chem., 2004, 28, 822–827 RSC
. - T. N. Singh-Rachford and F. N. Castellano, J. Phys. Chem. A, 2008, 112, 3550–3556 CrossRef CAS PubMed
. - F. Zhong and J. Zhao, Dyes Pigm., 2017, 136, 909–918 CrossRef CAS
. - C. Ye, B. Wang, R. Hao, X. Wang, P. Ding, X. Tao, Z. Chen, Z. Liang and Y. Zhou, J. Mater. Chem. C, 2014, 2, 8507–8514 RSC
. - C. Ye, B. Sun, X. Wang, J. Yang, P. Ding, S. Zhu, Q. He, Z. Liang and X. Tao, Dyes Pigm., 2014, 102, 133–141 CrossRef CAS
. - Y. Y. Cheng, T. Khoury, R. G. Clady, M. J. Tayebjee, N. J. Ekins-Daukes, M. J. Crossley and T. W. Schmidt, Phys. Chem. Chem. Phys., 2010, 12, 66–71 RSC
. - A. Monguzzi, J. Mezyk, F. Scotognella, R. Tubino and F. Meinardi, Phys. Rev. B: Condens. Matter Mater. Phys., 2008, 78, 195112 CrossRef
. - A. Haefele, J. Blumhoff, R. S. Khnayzer and F. N. Castellano, J. Phys. Chem. Lett., 2012, 3, 299–303 CrossRef CAS
. - C. Ye, L. Zhou, X. Wang and Z. Liang, Phys. Chem. Chem. Phys., 2016, 18, 10818–10835 RSC
. - A. Turshatov, D. Busko, Y. Avlasevich, T. Miteva, K. Landfester and S. Baluschev, ChemPhysChem, 2012, 13, 3112–3115 CrossRef CAS PubMed
.
Footnotes |
† Electronic supplementary information (ESI) available. See DOI: 10.1039/d1ra02528g |
‡ These authors contributed equally to this work. |
|
This journal is © The Royal Society of Chemistry 2021 |
Click here to see how this site uses Cookies. View our privacy policy here.