DOI:
10.1039/D1RA02377B
(Paper)
RSC Adv., 2021,
11, 15573-15580
Persulfate-activated charcoal mixture: an efficient oxidant for the synthesis of sulfonated benzo[d][1,3]oxazines from N-(2-vinylphenyl)amides and thiols in aqueous solution†
Received
25th March 2021
, Accepted 20th April 2021
First published on 27th April 2021
Abstract
A series of 2,4-aryl-4-((arylsulfonyl)methyl)-4H-benzo[d][1,3]oxazines in good to excellent yields have directly been obtained from N-(2-vinylphenyl)amides and thiols by employing a mixture of K2S2O8-activated charcoal in aqueous acetonitrile solution at 50 °C. A plausible mechanism for the reaction is reported. It reveals that the reaction follows a radical pathway and the persulfate has been the oxygen source for formation of the sulfone group in the products. It is worth mentioning that this protocol utilizes an easily accessible K2S2O8-activated charcoal mixture and thiols, respectively, as an oxidant and sulfonylating precursors for the first time.
Introduction
Benzoxazines and their derivatives are an important class of heterocycles frequently found in many natural products1 and biologically active compounds2 (e.g. 6-chloro-N-ethyl-4-methyl-4-phenyl-4H-benzo[d][1,3]oxazin-2-amine and 2-chloro-1-(2,2,4,4-tetramethyl-2H-benzo[d][1,3]oxazin-1(4H)-yl)ethanone). Likewise, as the key structural motif changes the physical and chemical properties of the parent molecule, the sulfone (–SO2–) functionality has widely been installed in a variety of functional materials3 (pristine polysulfone networks) and drugs4 (dapsone, diazoxide and sulfisoxazole). Therefore, development of a practical method for the synthesis of sulfonated benzo[d][1,3]oxazines has drawn significant attention from chemists, pharmacists and biologists. In 2018, Wu and co-workers5 reported visible-light photocatalysis for the preparation of sulfonated benzo[d][1,3]oxazines from N-(2-vinylphenyl)amides, DABCO·(SO2)2 and diazonium salts (Scheme 1a).5 Similarly, the acid-mediated oxythiolation of o-vinylanilides with N-(arylthio)-succinimides and m-CPBA was reported by the Anbarasan group in 2018 (Scheme 1b).6 In 2019, Li and co-workers7 disclosed a metal-catalyzed approach for the synthesis of sulfonated benzo[d][1,3]oxazines from N-(2-vinylphenyl)amides and alkyl(aryl)sulfinates (Scheme 1c).7 Last year, Huang et al.8 described an electrochemical method for the synthesis of sulfonated benzo[d][1,3]oxazines from N-(2-vinylphenyl)amides and sulfonyl hydrazines, cf. Scheme 1d.8
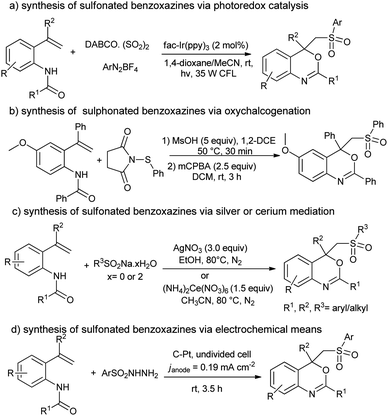 |
| Scheme 1 Various protocols for the synthesis of sulfonated benzo[d][1,3]oxazines yet reported in literature. | |
Persulfate (S2O82−, also known as peroxydisulfate or peroxodisulfate) as readily available and efficient oxidizing reagent has extensively been utilized for organic synthesis9 and environmental remediation10 in recent decades. Persulfate is a white crystalline solid, cheap, chemically stable at ambient conditions, easy-to handle and convenient to transport. Moreover, upon activation11 by heat, metal ions, carbon materials, base, or ultra-violet radiation, persulfate affords a powerful one-electron oxidant such as sulfate anion-radical (SO4˙−, E° = 2.5–3.1 V) that has longer life span than HO˙ precursors, hydrogen peroxide and ozone. In spite of this potentiality, to the best of our knowledge, the use of persulfate for the synthesis of sulfonated benzo[d][1,3]oxazines has never been reported. In view of this and in continuation of our ongoing attention to explore the applications of persulfate,12 herein we disclose our findings on the preparation of 2,4-aryl-4-((arylsulfonyl)methyl)-4H-benzo[d][1,3]oxazines from N-(2-vinylphenyl)amides and thiols by employing mixture of K2S2O8-activated charcoal in aqueous acetonitrile solution at 50 °C (Scheme 2). Furthermore, a plausible mechanism for the reaction is reported, vide infra. Especially, this is a simple and highly efficient method for the construction of C–S, C–O and S–O bonds in one step and utilizes, at the first time, easily accessible K2S2O8-activated charcoal mixture and thiols, respectively, as an oxidant and sulfonylating precursors.
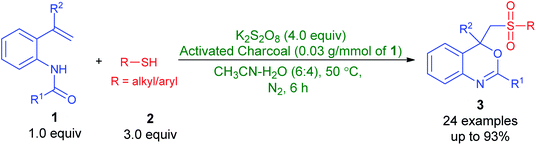 |
| Scheme 2 The synthesis of 2,4-aryl-4-((arylsulfonyl)methyl)-4H-benzo[d][1,3]oxazines from N-(2-vinylphenyl)amides and thiols employing mixture of K2S2O8-activated charcoal in aqueous acetonitrile solutional 50 °C reported in this work. | |
Results and discussion
We chose N-(2-(1-phenylvinyl)phenyl)benzamide (1a, 1.0 mmol) and benzenethiol (2a, 2.0 mmol) as model substrates to optimize conditions for this reaction, and the obtained results are summarized in Table 1. By using 2.0 mmol of K2S2O8 as an oxidant and CH3CN/H2O (6
:
4, v/v mL) as a solvent, the mixture of 1a and 2a was heated at 80 °C under nitrogen gas atmosphere for 36 h. Fortunately, the expected sulfonated oxindole, i.e., 2,4-diphenyl-4-((phenylsulfonyl)methyl)-4H-benzo[d][1,3]oxazine (3aa) was produced in 26% yield (Table 1, entry 1). The product 3aa was isolated and characterized by NMR and mass analysis (ESI). Encouraged by this result, 2.0 equiv., of different oxidants include Na2S2O8, (NH4)2S2O8, hydrogen peroxide and di-tert-butyl peroxide (DTBP) (Table 1, entries 2–5) were tested, and K2S2O8 was found to be the best choice (Table 1, entry 1). Thus, K2S2O8 was taken as an oxidant for further all optimizations and reactions. Replacing reaction medium CH3CN/H2O (6
:
4, v/v mL) with other common solvents such as methanol, acetonitrile, DMSO, water and EtOAc–H2O (5
:
5, v/v mL) could also afforded the desired product 3aa, but in poor yield (Table 1, entries 6–10). To improve the product yield, we then investigated the stoichiometry of K2S2O8 to substrate N-(2-(1-phenylvinyl)phenyl)benzamide (1a). A slightly improved yield (43%) of 3aa was noticed by increasing the amount of K2S2O8 to 4.0 equiv. (Table 1, entries 11–13); however further raising the amount of K2S2O8 did not improve the reaction efficiency drastically (Table 1, entries 14–15). Under similar conditions, the reaction gave 54% of product (3aa) with higher quantity of benzenethiol (3.0 equiv., Table 1, entry 16). Additional optimizations revealed that the reaction atmosphere was crucial for outcome of the reaction. The reaction proceeded efficiently under nitrogen gas atmosphere while the involvement of air or molecular oxygen brings down yield of 3aa, cf. entries 18 and 19 in Table 1. To further improve the product yield, we decided to activate the persulfate by a mild protocol. As the productivity of sulfate radicals from persulfate can be influenced by the activation types.
Table 1 Selected results of screening the optimal conditions for the synthesis of 2,4-aryl-4-((arylsulfonyl)methyl)-4H-benzo[d][1,3]oxazines from N-(2-vinylphenyl)amides and thiolsa
Recently, granulated activated carbon has been reported to successfully activate persulfate under a mild condition.13 It has a certain advantage in being non-metallic species free from metal leaching problems. Also, the activation of persulfate by granulated activated carbon proceeds on the surface of activated carbon during the radical propagation mechanism.14 Thus, the influence of activated charcoal was studied for the formation of 3aa from 1a and 2a under the reaction conditions mentioned in entry 16 of Table 1. By addition of 40 mg of activated charcoal to the mixture of 1a, 2a and K2S2O8 in CH3CN/H2O (6
:
4, v/v mL) at room temperature, expected product 3aa was obtained in 64% yield (Table 1, entry 20). However, rate of the reaction was too low (52 h). More examinations revealed that the complete conversion of mixture of 1a (1.0 equiv.) and 2a (3.0 equiv.) to 3aa in 93% yield required K2S2O8 (4.0 equiv.) and activated charcoal (0.03 g mmol−1 of 1a) in CH3CN/H2O (6
:
4, v/v mL) at 50 °C for 6 h (Table 1, entry 24). Control experiments revealed that K2S2O8 (Table 1, entry 26) was essential, and no desired product was detected in its absence. From these experiments, we determined the optimized conditions as: N-(2-vinylphenyl)amide (1.0 equiv.), thiol (3.0 equiv.), K2S2O8 (4.0 equiv.), and activated charcoal (0.03 g mmol−1 of N-(2-vinylphenyl)amide) in CH3CN/H2O (6
:
4, v/v mL) under nitrogen gas atmosphere at 50 °C for 6 h (Table 1, entry 24).
With the optimized conditions in hand, the substrate scope and functional group tolerance was studied and the obtained results are presented in Table 2. First, the reactivity of N-(2-vinylphenyl)amides with substituent on the benzamide ring (1a–1i) was studied. Indeed, this protocol was found applicable to both electron-donating group and electron-withdrawing group substituent. For instances, methyl-, methoxy-, fluoro-, chloro- and bromo-substituted N-(2-vinylphenyl)amides provided the corresponding desired products (3ba–3fa) in 84–92% yields. Notably, the amides with alkyl substituent, such as methyl and tert-butyl groups were effective for this reaction and converted to the corresponding benzo[d][1,3]oxazines (3ga and 3ha) in moderate to good yields. Nevertheless, no desired product (3ia) could be collected when benzyl-substituted N-(2-vinylphenyl)amide was treated with benzenethiol, cf. Table 2. Next, we studied the scope of substituted alkenes (1j–1p) in the reaction system. Various substituent include tolyl-, 4-fluorophenyl and 4-chlorophenyl at the α-position of styrenes, afforded products (3ja–3la) in good yields. Likewise, the present system could also be employed to N-(2-(prop-1-en-2-yl)phenyl)benzamide and N-(2-(prop-1-en-2-yl)phenyl)pivalamide giving products (3ma and 3na) with a yield of 89% and 76% respectively. However, no desired product (3oa or 3pa) could be collected when either N-(2-(1-(4-methoxyphenyl)vinyl)phenyl)benzamide or mono-substituted alkene such as N-(2-vinylphenyl)benzamide was treated with benzenethiol, cf. Table 2.
Table 2 Substrate scope for the synthesis of 2,4-aryl-4-((arylsulfonyl)methyl)-4H-benzo[d][1,3]oxazines from various N-(2-vinylphenyl)amides and benzenethiola
Unless stated otherwise, all reactions were performed in a Schlenk tube with N-(2-vinylphenyl)amides (1a–1p, 1.0 mmol), benzenethiol (2a, 3.0 mmol), K2S2O8 (4.0 mmol) and activated charcoal (0.03 g) in CH3CN/H2O (6 : 4, v/v mL) under nitrogen gas atmosphere at 50 °C for 6 h. |
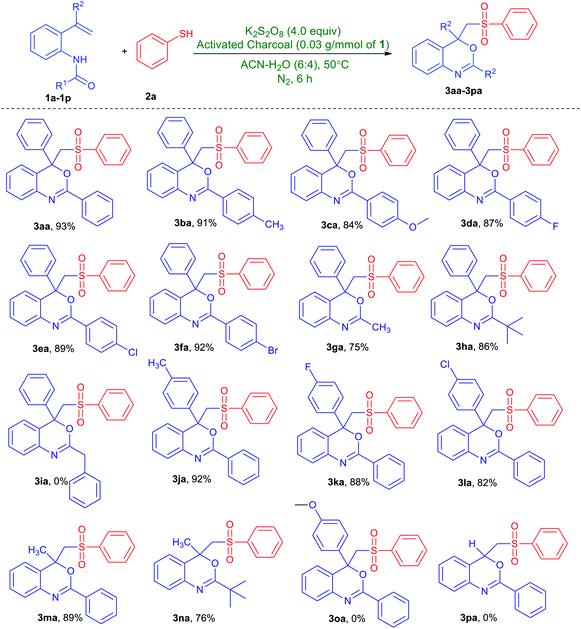 |
To further explore the substrate scope, we then studied the scope of thiols (Table 3). Thiols with methyl-, methoxy- and chloro-group at the para-position of the arene ring produced the desired products in excellent yields (3ab–3ad). However, 4-nitrobenzenethiol leads to a dramatic decrease of the reaction efficiency and afforded product 3ae in 10% yield. To our delight, this protocol is also applicable to cyclohexanethiol and thiophene-2-thiol and was converted to the corresponding products (3af and 3ag) in significant yield. Unfortunately, thiols with a hydroxy or an amino group on the arene ring could not afford the desired product (3ah and 3ai).
Table 3 Substrate scope for the synthesis of 2,4-aryl-4-((arylsulfonyl)methyl)-4H-benzo[d][1,3]oxazines from N-(2-(1-phenylvinyl)phenyl)benzamide and various thiolsa
Unless stated otherwise, all reactions were performed in a Schlenk tube with N-(2-(1-phenylvinyl)phenyl)benzamide (1a, 1.0 mmol), thiols (2b–2i, 3.0 mmol), K2S2O8 (4.0 mmol) and activated charcoal (0.03 g) in CH3CN/H2O (6 : 4, v/v mL) under nitrogen gas atmosphere at 50 °C for 6 h. |
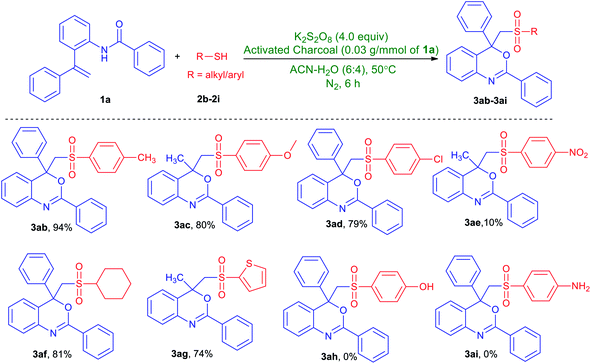 |
This reaction could also be performed on a gram scale. As shown in Scheme 3, treatment of 1.9 g (6 mmol) of 4-methyl-N-(2-(1-phenylvinyl)phenyl)benzamide (1b) with 3 equiv. of benzenethiol (2a) under the optimized reaction conditions (Table 1, entry 24) afforded the desired 4-phenyl-4-((phenylsulfonyl)methyl)-2-p-tolyl-4H-benzo[d][1,3]oxazine (3ba) in 88% (2.4 g) isolated yield, clearly demonstrating the preparative practicality of this protocol.
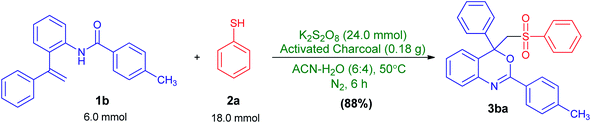 |
| Scheme 3 Gram-scale synthesis. | |
To understand the mechanism of this transformation, some control experiments were carried out as described in Scheme 4. On addition of 2 equiv. of radical scavenger 2,2,6,6-tetramethylpiperidine-1-oxyl (TEMPO) under the standard conditions, no desired product (3aa) was observed (instead thiol·TEMPO adduct detected by GCMS analysis). In other words, TEMPO completely inhibited this reaction indicating that the reaction follows a radical pathway.7,8 In addition, the sulfur-containing benzo[d][1,3]oxazine (VI) could also be converted to the sulfone-containing benzo[d][1,3]oxazine (3aa) in good yield under similar reaction conditions in the absence of thiol. Thus, it would be reasonable to deduce that sulfur-containing benzo[d][1,3]oxazine (VI) was the plausible intermediate in this reaction.6
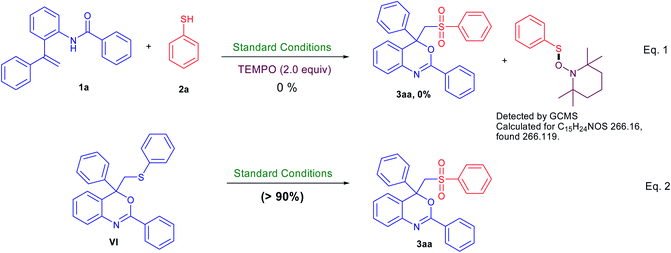 |
| Scheme 4 Control experiments for mechanistic studies. | |
On the basis of the results described above and previous reports, a plausible mechanism is outlined in Scheme 5. Initially, the activated charcoal-assisted heterolytic cleavage of S2O82− affords sulfate radical anions (SO4˙−), which then abstract the hydrogen atoms from the thiol (2) to afford a sulfur-centered sulfonyl radical (II).13,14 The addition of radical II to C
C bond of N-(2-vinylphenyl)amide (1) would lead to the formation of alkyl radical III. Later, III undergoes an intramolecular radical cyclization to provide a new radical intermediate IV. Subsequently, the radical IV was further oxidized to the corresponding carbocation (V) by oxidant followed by deprotonation to afford the sulfur-containing benzo[d][1,3]oxazine (VI).5 However, a cationic cyclization cannot be excluded completely, in which the alkyl radical intermediate III is further oxidized to carbocation and subsequently trapped by the carbonyl group of amide.6 The resulting VI would be rapidly oxidized to desired sulfone-containing benzo[d][1,3]oxazine (3) by K2S2O8.7,13 The influence of activated charcoal on reactivity of K2S2O8 is not clear, however, we believe that the high surface area and micro porosity of activated charcoal may play role through widespread interactions and scission of S2O82− to more powerful sulfate radical anions (SO4˙−) that accelerates the reaction under mild conditions.14
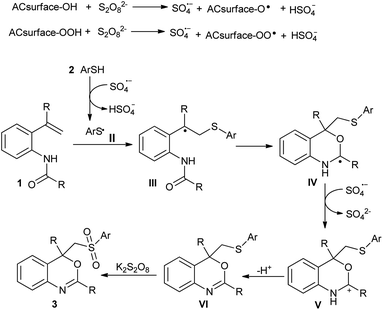 |
| Scheme 5 A plausible mechanism for the formation of 2,4-aryl-4-((arylsulfonyl)methyl)-4H-benzo[d][1,3]oxazines from N-(2-vinylphenyl)amides and thiols. | |
In summary, a mild and cost-efficient protocol was developed for the synthesis of 2,4-aryl-4-((arylsulfonyl)methyl)-4H-benzo[d][1,3]oxazines from N-(2-vinylphenyl)amides and thiols by employing mixture of K2S2O8-activated charcoal in aqueous acetonitrile solution at 50 °C. The facile formation of new C–S, C–O and S–O bonds take place in a one-pot procedure. Versatility of this synthetic method for a broad range of N-(2-vinylphenyl)amides and thiols as well as the benefits of use of easily accessible K2S2O8-activated charcoal mixture and thiols, respectively, as an oxidant and sulfonylating precursors. Further studies on the mechanism and applications are ongoing in our laboratory.
Experimental section
General procedure for the synthesis of 2,4-aryl-4-((arylsulfonyl)methyl)-4H-benzo[d][1,3]oxazines
An oven-dried Schlenk-tube equipped with a magnetic stir bar was charged with N-(2-vinylphenyl)amides (1.0 mmol, 1.0 equiv.), thiol (3.0 mmol, 3.0 equiv.), K2S2O8 (4.0 mmol, 4.0 equiv.) and activated charcoal (0.03 g). To this mixture, CH3CN/H2O (6
:
4, v/v mL, 10 mL) was added. Then, the tube was sealed and inlet/outlet for N2 gas was provided by a side-neck. Resultant mixture was vigorously stirred under nitrogen gas atmosphere at 50 °C for 6 h. After the completion (as indicated by TLC, ≈6 h) volatiles were evaporated under reduced pressure and then admixed with aqueous K2CO3 solution (20 mL). The organic matters are extracted with ethyl acetate, dried over Na2SO4 and evaporated under reduced pressure to yield a pale-yellow gummy-solid, which was purified by a column chromatography using a mixture of ethyl acetate and hexane. The identity and purity of the product was confirmed by spectroscopic analysis as well as by a comparison with authentic samples spectra, vide infra.
Conflicts of interest
There are no conflicts to declare.
Acknowledgements
Authors are gratefully acknowledge the financial support from Council of Industrial Scientific Research (CSIR), New Delhi, India, through extra mutual research grant with a project number 02(0380)/19/EMR-II. Priya and D. C., thank the CSIR, New Delhi for their senior research fellowships.
References
-
(a) N. Kumar, N. Yadav, N. Amarnath, V. Sharma, S. Shukla, A. Srivastava, P. Prasad, A. Kumar, S. Garg, S. Singh, S. Sehrawat and B. Lochab, Integrative natural medicine inspired graphene nanovehicle-benzoxazine derivatives as potent therapy for cancer, Mol. Cell. Biochem., 2019, 454, 123–138 CrossRef CAS PubMed;
(b) K. Zhang, M. Han, Y. Liu and P. Froimowicz, Design and synthesis of bio-based high-performance trioxazine benzoxazine resin via natural renewable resources, ACS Sustainable Chem. Eng., 2019, 7, 9399–9407 CrossRef CAS;
(c) Y. Lyu and H. Ishida, Natural-sourced benzoxazine resins, homopolymers, blends and composites: a review of their synthesis, manufacturing and applications, Prog. Polym. Sci., 2019, 99, 101168 CrossRef CAS;
(d) J. Sheng, X. Su, C. Cao and C. Chen, Synthesis of benzo[1,3]oxazines via copper(i)-catalyzed cascade annulation of nitriles, aldehydes and diaryliodonium salts, Org. Chem. Front., 2016, 3, 501–504 RSC;
(e) B. V. S. Reddy, R. A. Babu, M. R. Reddy, B. J. M. Reddy and B. Sridhar, Intramolecular C–O/C–S bond insertion of α-diazoesters for the synthesis of 2-aryl-4H-benzo[d][1,3]oxazine and 2-aryl-4H-benzo[d][1,3]thiazine derivatives, RSC Adv., 2014, 4, 44629–44633 RSC;
(f) A. A. A. Qahtani, T. M. A. Turki, A. A. Mousa, S. A. A. Mazroa, M. Khan and H. Z. Alkhathlan, Simple and selective synthesis of 1,3-benzoxazine derivatives, Orient, J. Chem., 2012, 28, 287–295 Search PubMed;
(g) J. Tanabe, M. Sue, A. Ishihara and H. Iwamura, Occurrence and characterization of 2-hydroxy-1,4-benzoxazin-3-one and indole hydroxylases in juvenile wheat, Biosci. Biotechnol. Biochem., 1999, 63, 1614–1617 CrossRef CAS PubMed.
-
(a) W. -C. Lee, H. -C. Shen, W. -P. Hu, W. -S. Lo, C. Murali, J. K. Vandavasi and J. -J. Wang, Iodine-catalyzed, stereo- and regioselective synthesis of 4-arylidine-4h-benzo[d][1,3]oxazines and their applications for the synthesis of quinazoline 3-oxides, Adv. Synth. Catal., 2012, 354, 2218–2228 CrossRef CAS;
(b) Z. Tang, W. Chen, Z. Zhu and H. Liu, Synthesis of 2,3-Diaryl-3,4-dihydro-2H-1,3-benzoxazines and their fungicidal activities, J. Heterocyclic Chem., 2011, 48, 255 CrossRef CAS;
(c) J. Ilas, Z. Jakopin, T. Borstnar, M. Stegnar and D. Kikelj, 3,4-dihydro-2h-1,4-benzoxazine derivatives combining thrombin inhibitory and glycoprotein IIb/IIIa receptor antagonistic activity as a novel class of antithrombotic compounds with dual function, J. Med. Chem., 2008, 51, 5617–5629 CrossRef CAS PubMed;
(d) A. Fensome, R. Bender, R. Chopra, J. Cohen, M. A. Collins, V. Hudak, K. Malakian, S. Lockhead, A. Olland, K. Svenson, E. A. Terefenko, R. J. Unwalla, J. M. Wilhelm, S. Wolfrom, Y. Zhu, Z. Zhang, P. Zhang, R. C. Winneker and J. Wrobel, Synthesis and structure-activity relationship of novel 6-aryl-1,4- dihydrobenzo[d][1,3]oxazine-2-thiones as progesterone receptor modulators leading to the potent and selective nonsteroidal progesterone receptor agonist tanaproget, J. Med. Chem., 2005, 48, 5092–5095 CrossRef CAS PubMed;
(e) N. Dias, J.-F. Goossens, B. Baldeyrou, A. Lansiaux, P. Colson, A. D. Salvo, J. Bernal, A. Turnbull, D. J. Mincher and C. Bailly, Oxoazabenzo[de]anthracenes conjugated to amino acids: synthesis and evaluation as DNA-binding antitumor agents, Bioconjugate Chem., 2005, 16, 949–958 CrossRef CAS PubMed;
(f) K. Waisser, J. Gregor, L. Kubicová, V. Klimesová, J. Kunes, M. Machácek and J. Kaustová, New groups of antimycobacterial agents: 6-chloro-3-phenyl-4-thioxo-2H-1,3-benzoxazine-2(3H)-ones and 6-chloro-3-phenyl-2H-1,3 benzoxazine-2,4(3H)-dithiones, Eur. J. Med. Chem., 2000, 35, 733–741 CrossRef CAS PubMed.
-
(a) Z. Tian and D. Kim, Solid electrolyte membranes prepared from poly(arylene ether sulfone)-g-poly(ethylene glycol) with various functional end groups for lithium-ion battery, J. Membr. Sci., 2021, 621, 119023 CrossRef CAS;
(b) H. Li, M. Hong, A. Scarpaci, X. He, C. Risko, J. S. Sears, S. Barlow, P. Winget, S. R. Marder, D. Kim and J.-L. Brédas, Chemical stabilities of the lowest triplet state in aryl sulfones and aryl phosphine oxides relevant to OLED applications, Chem. Mater., 2019, 31, 1507–1519 CrossRef CAS;
(c) X. Wang, L. Chen, S. Y. Chong, M. A. Little, Y. Wu, W.-H. Zhu, R. Clowes, Y. Yan, M. A. Zwijnenburg, R. S. Sprick and A. I. Cooper, Sulfone-containing covalent organic frameworks for photocatalytic hydrogen evolution from water, Nat. Chem., 2018, 10, 1180–1189 CrossRef CAS PubMed;
(d) N. A. Alenazi, M. A. Hussein, K. A. Alamry and A. M. Asiri, Modified polyether-sulfone membrane: a mini review, Des. Monomers Polym., 2017, 20, 532–546 CrossRef CAS PubMed;
(e) M. Podgórski, C. Wang, Y. Yuan, D. Konetski, I. Smalyukhand and C. N. Bowman, Pristine polysulfone networks as a class of polysulfide-derived high-performance functional materials, Chem. Mater., 2016, 28(14), 5102–5109 CrossRef;
(f) H. Sasabe, Y. Seino, M. Kimura and J. Kido, A m-terphenyl-modified sulfone derivative as a host material for high-efficiency blue and green phosphorescent OLEDs, Chem. Mater., 2012, 24, 1404–1406 CrossRef CAS;
(g) R. Nolte, K. Ledjeff, M. Bauer and R. Mülhaupt, Partially sulfonated poly (arylene ether sulfone) -A versatile proton conducting membrane material for modern energy conversion technologies, J. Membr. Sci., 1993, 83, 211–220 CrossRef CAS.
-
(a) J. George, Metabolism and interactions of antileprosy drugs, Biochem. Pharmacol., 2020, 177, 113993–114001 CrossRef CAS PubMed;
(b) M. Ibrahim, A. Latif, A. A. Ali, A. I. Ribeiro, U. Farooq, F. Ullah, A. Khan, A. A. Harrasi, M. Ahmad and M. Ali, Macrocyclic sulfone derivatives: Synthesis, characterization, in vitro biological evaluation and molecular docking, Drug Dev. Res., 2020 DOI:10.1002/ddr.21775;
(c) M. R. Shaaban, T. A. Farghaly and A. M. R. Alsaedi, Synthesis, antimicrobial and anticancer evaluations of novel thiazoles incorporated diphenyl sulfone moiety, Polycycl. Aromat. Compd., 2020 DOI:10.1080/10406638.2020.1837887;
(d) K. A. Scott and J. T. Njardarson, Analysis of US FDA-approved drugs containing sulfur atoms, Top. Curr. Chem., 2018, 376, 1–34 CrossRef CAS PubMed;
(e) M. Feng, B. Tang, S. H. Liang and X. Jiang, Sulfur containing scaffolds in drugs: synthesis and application in medicinal chemistry, Curr. Top. Med. Chem., 2016, 16, 1200–1216 CrossRef CAS PubMed;
(f) A. L. Lorincz and R. W. Pearson, Sulfapyridine and sulfone type drugs in dermatology, Arch. Dermatol., 1962, 85, 2–16 CrossRef CAS PubMed.
- T. Liu, D. Zheng, Z. Li and J. Wu, Synthesis of sulfonated benzo[d][1,3]oxazines by merging photoredox catalysis and insertion of sulfur dioxide, Adv. Synth. Catal., 2017, 360, 865–869 CrossRef.
- M. Chaitanya and P. Anbarasan, Acid-mediated oxychalcogenation of o-vinylanilides with N-(arylthio/arylseleno)succinimides, Org. Lett., 2018, 20, 1183–1186 CrossRef CAS PubMed.
- J. Wu, Y. Zong, C. Zhao, Q. Yan, L. Sun, Y. Li, J. Zhao, Y. Ge and Z. Li, Silver or cerium-promoted free radical cascade difunctionalization of o-vinylanilides with sodium aryl- or alkylsulfinates, Org. Biomol. Chem., 2019, 17, 794–797 RSC.
- T.-J. He, W.-Q. Zhong and J.-M. Huang, The synthesis
of sulfonated 4H-3,1-benzoxazines via an electro-chemical radical cascade cyclization, Chem. Commun., 2020, 56, 2735–2738 RSC.
-
(a) J. K. Laha, M. K. Hunjan, S. Hegde and A. Gupta, Aroylation of electron-rich pyrroles under minisci reaction conditions, Org. Lett., 2020, 22, 1442–1447 CrossRef CAS PubMed;
(b) H. Tian, H. Yang, C. Tian, G. An and G. Li, Cross-dehydrogenative coupling of strong C(sp3)−H with N-heteroarenes through visible-light-induced energy transfer, Org. Lett., 2020, 22, 7709–7715 CrossRef CAS PubMed;
(c) V. D. Nguyen, V. T. Nguyen, G. C. Haug, H. T. Dang, H. D. Arman, W. C. Ermler and O. V. Larionov, Rapid and chemodivergent synthesis of N-heterocyclic sulfones and sulfides: mechanistic and computational details of the persulfate-initiated catalysis, ACS Catal., 2019, 9, 4015–4024 CrossRef CAS;
(d) G. K. Rastogi, B. Deka, M. L. Deb and P. K. Baruah, Diastereoselective sp3-C–H functionalization of arylmethyl ketones and transformation of E- to Z-products through photocatalysis, Eur. J. Org. Chem., 2019, 2020, 424–428 CrossRef;
(e) S. Manna and K. R. Prabhu, Visible-light-mediated direct decarboxylative acylation of electron- deficient heteroarenes using α-ketoacids, J. Org. Chem., 2019, 84, 5067–5077 CrossRef CAS PubMed.
-
(a) W. Dong, T. Cai, Y. Liu, L. Wang, H. Chen, W. Zeng, J. Li and W. Li, Rapid removal of organic pollutants by a novel persulfate/brochantite system: Mechanism and implication, J. Colloid Interface Sci., 2021, 585, 400–407 CrossRef CAS PubMed;
(b) G. Chen, Y. Yu, L. Liang, X. Duan, R. Li, X. Lu, B. Yan, N. Li and S. Wang, Remediation of antibiotic wastewater by coupled photocatalytic and persulfate oxidation system: A critical review, J. Hazard. Mater., 2021, 408, 124461 CrossRef CAS PubMed;
(c) J. Lee, U. Gunten and J.-H. Kim, Persulfate-based advanced oxidation: critical assessment of opportunities and roadblocks, rapid removal of organic pollutants by a novel persulfate/brochantite system: mechanism and implication, Environ. Sci. Technol., 2020, 54, 3064–3081 CrossRef CAS PubMed;
(d) D. Zhi, Y. Lin, L. Jiang, Y. Zhou, A. Huang, J. Yang and L. Luo, Remediation of persistent organic pollutants in aqueous systems by electrochemical activation of persulfates: A review, J. Environ. Manage., 2020, 260, 110125 CrossRef CAS PubMed;
(e) S. Wacławek, H. V. Lutze, K. Grübel, V. V. T. Padil, M. Černík and D. D. Dionysiou, Chemistry of persulfates in water and wastewater treatment: a review, Chem. Eng. J., 2017, 330, 44–62 CrossRef;
(f) L. W. Matzek and K. E. Carter, Activated persulfate for organic chemical degradation: A review, Chemosphere, 2016, 151, 178–188 CrossRef CAS PubMed;
(g) B.-T. Zhang, Y. Zhang, Y. Teng and M. Fan, Sulfate radical and its application in decontamination technologies, Crit. Rev. Environ. Sci. Technol., 2015, 45, 1756–1800 CrossRef CAS;
(h) C. Chen, Y. Cao, X. Wu, Y. Cai, J. Liu, L. Xu, K. Ding and L. Yu, Energy saving and environment-friendly element-transfer reactions with industrial application potential, Chin. Chem. Lett., 2020, 31, 1078–1082 CrossRef CAS.
-
(a) S. F. C. Acevedoa, L. A. B. Buitrago, D. D. Dionysiou and F. M. Martínez, Ultraviolet light-mediated activation of persulfate for the degradation of cobalt cyano complexes, J. Hazard. Mater., 2020, 392, 122389–122396 CrossRef PubMed;
(b) J. Yu, H. Feng, L. Tang, Y. Pang, G. Zeng, Y. Lu, H. Dong, J. Wang, Y. Liu, C. Feng, J. Wang, B. Peng and S. Ye, Metal-free carbon materials for persulfate-based
advanced oxidation process: Microstructure, property and tailoring, Prog. Mater. Sci., 2020, 111, 100654 CrossRef CAS;
(c) W. Song, J. Li, Z. Wang and X. Zhang, A mini review of activated methods to persulfate-based advanced oxidation process, Water Sci. Technol., 2019, 79, 573–579 CrossRef CAS PubMed;
(d) B. Wang, Y.-Ni. Li and L. Wang, Metal-free activation of persulfates by corn stalk biochar for the degradation of antibiotic norfloxacin: Activation factors and degradation mechanism, Chemosphere, 2019, 237, 124454–124462 CrossRef CAS PubMed;
(e) I. A. Ike, K. G. Linden, J. D. Orbell and M. Duke, Critical review of the science and sustainability of persulphate advanced oxidation processes, Chem. Eng. J., 2018, 338, 651–669 CrossRef CAS;
(f) J. Wang and S. Wang, Activation of persulfate (PS) and peroxymonosulfate (PMS) and application for the degradation of emerging contaminants, Chem. Eng. J., 2018, 334, 1502–1517 CrossRef CAS;
(g) R. Xiao, Z. Luo, Z. Wei, S. Luo, R. Spinney, W. Yang and D. D. Dionysiou, Activation of peroxymonosulfate/persulfate by nanomaterials for sulfate radical-based advanced oxidation technologies, Curr. Opin. Chem. Eng., 2018, 19, 51–58 CrossRef.
-
(a) Priya and P. Natarajan, An overview on the organic synthesis and transformations initiated by sulfate radical anions (SO4˙−) produced from aqueous persulfate solutions under transition metal-free conditions, in Modern Green Chemistry and Heterocyclic Compounds, ed. R. S. Shinde and A. K. Haghi, Apple Academic Press, 2020, ISBN: 978-177188-8325 Search PubMed;
(b) P. Natarajan, Priya and D. Chuskit, Transition-metal-free and organic solvent-free conversion of N-substituted 2-aminobiaryls into corresponding carbazoles via intramolecular oxidative radical cyclization induced by peroxodisulfate, Green Chem., 2017, 19, 5854–5861 RSC.
-
(a) J. Li, K. Zhu, R. Li, X. Fan, H. Lin and H. Zhang, The removal of azo dye from aqueous solution by oxidation with peroxydisulfate in the presence of granular activated carbon: Performance, mechanism and reusability, Chemosphere, 2020, 259, 127400 CrossRef CAS PubMed;
(b) M. Forouzesh, A. Ebadi, A. A. Meybodi and R. Khoshbouy, Transformation of persulfate to free sulfate radical over granular activated carbon: Effect of acidic oxygen functional groups, Chem. Eng. J., 2019, 374, 965–974 CrossRef CAS;
(c) M. Forouzesh, A. Ebadi and A. A. Meybodi, Degradation of metronidazole antibiotic in aqueous medium using activated carbon as a persulfate activator, Sep. Purif. Technol., 2019, 210, 145–151 CrossRef CAS;
(d) Y. -Y. Ahn and E. T. Yun, Heterogeneous metals and metal-free carbon materials for oxidative degradation through persulfate activation: A review of heterogeneous catalytic activation of persulfate related to oxidation mechanism, Korean J. Chem. Eng., 2019, 36, 1767–1779 CrossRef CAS;
(e) J. Chen, W. Hong, T. Huang, L. Zhang, W. Li and Y. Wang, Activated carbon fiber for heterogeneous activation of persulfate: implication for the decolorization of azo dye, Environ. Sci. Pollut. Res., 2016, 23, 18564–18574 CrossRef CAS PubMed;
(f) S. Yang, X. Yang, X. Shao, R. Niu and L. Wang, Activated carbon catalyzed persulfate oxidation of Azo dye acid orange 7 at ambient temperature, J. Hazard. Mater., 2011, 186, 659–666 CrossRef CAS PubMed.
-
(a) M. Forouzesh, A. Ebadi and A. A. Meybodi, Continuous fixed-bed oxidation of metronidazole by the sulfate radical based process over nitric acid treated granular activated carbon, J. Water Process. Eng., 2020, 36, 101280 CrossRef;
(b) C. Liang, Y. -T. Lin and W. -H. Shih, Treatment of trichloroethylene by adsorption and persulfate oxidation in batch studies, Ind. Eng. Chem. Res., 2009, 48, 8373–8380 CrossRef CAS.
Footnote |
† Electronic supplementary information (ESI) available: General aspects, procedure, experimental characterization data and copies of 1H and 13C NMR spectra for products. See DOI: 10.1039/d1ra02377b |
|
This journal is © The Royal Society of Chemistry 2021 |