DOI:
10.1039/D1RA02372A
(Paper)
RSC Adv., 2021,
11, 14079-14084
Metal-free, regioselective, visible light activation of 4CzIPN for the arylation of 2H-indazole derivatives†
Received
25th March 2021
, Accepted 31st March 2021
First published on 15th April 2021
Abstract
Highly regioselective organo photocatalysis of 4CzIPN (1,2,3,5-tetrakis(carbazol-9-yl)-4,6-dicyanobenzene) for the arylation of 2H-indazole is demonstrated. The present synthetic route provides a highly safe and easily accessible aniline precursor as an arylation reagent. The photoactivated 4CzIPN organocatalyst is found to be more efficient for single electron transfer without any organic base for the radical reaction. The carbazole-based photocatalyst (4CzIPN) with wide redox potential is stable and recyclable for further reaction transformations. Many indazole and aniline derivatives were used in the reaction and provided the arylated indazole derivatives in good to excellent yield.
Introduction
Indazole, an important structural motif present in many drug molecules and bioactive natural products have been attracting the synthetic community due to the broad spectrum of its biological activities.1–8 From extensive research work, the medicinal community looks to indazole as a bioisostere of benzimidazole, indazole and purine.1 Among the different types of indazole, compounds incorporated with 2H-indazole or 1H-indazole have been receiving much attention over the last two decades owing to their broad spectrum of biological activities.1 In particular, 2H-indazole derivatives have been widely investigated and some notable activities are HIV protease inhibition,2 anti-inflammatory,3 anti-microbial,4 anti-tumor,5 etc. Moreover, drug molecules like MK-4827, Niraparib, and Pazopanib are incorporated with the 2H-indazole motif and they are used for anticancer,6 anti-inflammatory7 and tyrosine kinase inhibitor8 activities respectively (Fig. 1). A C–H activation with a transition metal catalyst requires a pre-activated organometallic compound which is introduced in the transmetalation step. However direct methodology to activate the C–H bond in an aromatic compound9a would not require any organometallic compounds. The pre-activation strategy generates more bi-product in stoichiometry amount which can be avoided in direct C–H bond functionalization.9a,b To the best of our knowledge, direct arylation of indazole is largely depend on metal catalyst as well as stoichiometric base in the presence of halobenzene as an arylating source. These reactions suffer from very expensive additives as well as transition metal catalysts, harsh reaction temperature, and stability of the palladium or iridium catalyst.10a–d,g,11 Two alternative methodologies10e,f have been recently reported for the arylation of indazole using phenyldiazonium salt through a photochemical reaction path.
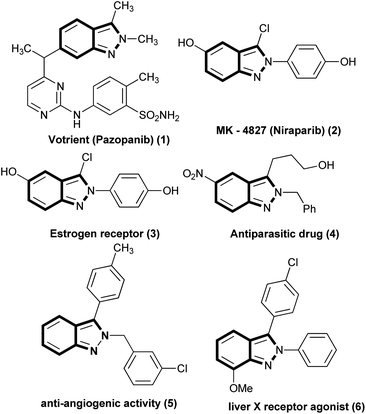 |
| Fig. 1 Representative example for 2H-indazole based drug and pharmaceutically active molecules. | |
The main drawback associated with phenyldiazonium salt is the potential instability of the salt whatsoever the choice of counter ion.12 The synthetic community has tended to focus on aniline and its derivatives instead of phenyldiazonium salt to overcome the safety issues associated with the salt. Many synthetic attempt have been proposed with the aim of generating the phenyldiazonium salts in situ and for subsequent arylation reactions.12 Despite these interest, to the best of our knowledge no arylation reaction on any type of indazole moiety using aniline as arylating precursor have been reported (Fig. 2).
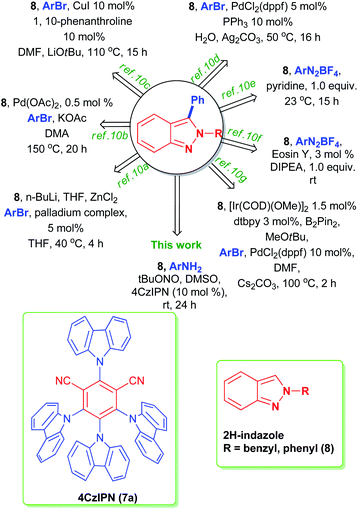 |
| Fig. 2 Various synthetic strategies for arylation on 2H-indazole. | |
Our endeavour was consequently to find a metal free procedure and to use safer arylation precursor. In recent years, there has been flourishing interest in the visible light mediated photochemical organic transformation.13 In this process, the sunlight as an energy source replaces the conventional thermal energy for an organic reaction. Although this sustainable process is interesting, the transition metal based redox catalyst for the photochemical reactions suffer from high cost and possible trace impurities in the final product. However, organo-catalytic photochemistry is superior in these aspects, particularly the straightforward work up procedure and less toxicity of organic photocatalyst.14 In this context, donor–acceptor fluorophore has been emerging as potent organo-photocatalyst. Based upon the photophysical and the photochemical properties of organic dye molecules,14 we were interested on compound 1,2,3,5-tetrakis(carbazol-9-yl)-4,6-dicyanobenzene (4CzIPN).15 The organic dye compound with carbazole unit as donor and dicyanobenzene as acceptor displayed significant redox window, excellent chemical stability and vide range of application in various organic transformations. Extending our interest on demonstrating organocatalytic arylation reaction,16 herein, we describe a metal-free carbazole based organo-photocatalyzed arylation on indazole by coupling of anilines with 2H-indazole as a nucleophilic partner. This approach involves an in situ generation of phenyldiazonium salt and subsequent functionalization of 2H-indazole catalysed by 4CzIPN. Our method provides a sustainable, safe, toxic less and less economic synthetic strategy to the indazole derivatives with good to excellent yield.
Results and discussion
p-Bromoaniline (9a) and indazole (8a) were chosen for finding the suitable reaction conditions. As expected, the indazole derivative 10a was obtained in 52% when 5 mol% of 7a was used in 7 W blue LED light in the presence of tBuONO in acetonitrile solvent (entry 1, Table 1). Surprisingly, 15 mol% of 4CzIPN under the same condition provided 83% of the product 10a (entry 2, Table 1). We could able to reduce the catalytic loading up to 10 mol% without affecting the reaction conversion (entry 3, Table 1). However, the reaction with 2 mol% of 4CzIPN showed no catalytic role as the yield of this reaction (entry 4, Table 1) is as same as yield of reaction without any catalyst (Scheme 3, eqn (a)). To prove the efficiency of the 4CzIPN, we compared with other carbazole based organic-photocatalyst. All these catalysts were prepared using the literature reports.17 The catalyst 4CzPN (7b), 2CzPN (7c) and 5CzPN (7d) under the same conditions (entry 2, Table 1) afforded the 10a in 54%, 49% and 64% yields respectively (entries 5–7, Table 1). On the other hand, THC (7e) and carbazole (7f) provided the indazole 10a in lower yields of 14% and 31% respectively (entries 8 & 9, Table 1). Lowering the reaction time from 24 h to 12 h influenced the reaction (entry 10, Table 1), Hence, reaction time of 24 h was considered as ideal for this transformation. Upon a brief solvent screening (entries 11–14, Table 1), it was identified that acetonitrile was suitable for synthesis of compound 10a with highest yield of 80% (entry 3, Table 1). In acetonitrile and DMSO solvents, the product was obtained in good yield. This is probably due to the efficiency of these solvents for the conversion of aniline to diazonium salt with the help of tBuONO. In addition, these polar solvents could stabilize the ionic intermediates by solvating them (Scheme 4). Reducing the equivalent of indazole 8a decreased the yield of the arylated product 10a to 71% (entry 15, Table 1). A comparison of various light source (entries 16–18, Table 1) for this arylation reaction showed that 7 W blue LED is superior than other lights. The absorption and intensity of the lights were recorded and provided in the ESI.†
Table 1 Optimization of the organophotocatalyzed arylation on 2H-indazolea
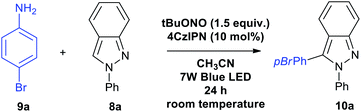
|
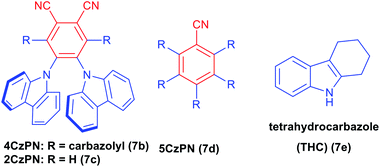
|
S. no. |
Variation of standard conditions |
Solvent |
Yieldb |
Unless otherwise noted: aReaction conditions: 1 equiv. of 9a (0.6 mmol), 2 equiv. of 8a (1.2 mmol), 1.5 equiv. of tBuONO (0.9), 10 mol% of catalyst, room temperature, 24 h. bIsolated yield. |
1 |
5 mol% of 4CzIPN (7a) |
CH3CN |
52 |
2 |
15 mol% of 4CzIPN |
CH3CN |
83 |
3 |
10 mol% of 4CzIPN |
CH3CN |
80 |
4 |
2 mol% of 4CzIPN |
CH3CN |
36 |
5 |
10 mol% of 4CzPN (7b) |
CH3CN |
54 |
6 |
10 mol% of 2CzPN (7c) |
CH3CN |
49 |
7 |
10 mol% of 5CzPN (7d) |
CH3CN |
64 |
8 |
10 mol% of THC (7e) |
CH3CN |
14 |
9 |
10 mol% of carbazole (7f) |
CH3CN |
31 |
10 |
10 mol% of 4CzIPN, 12 h |
CH3CN |
55 |
11 |
10 mol% of 4CzIPN |
DMSO |
76 |
12 |
10 mol% of 4CzIPN |
DMF |
62 |
13 |
10 mol% of 4CzIPN |
THF |
47 |
14 |
10 mol% of 4CzIPN |
Water |
52 |
15 |
1 equivalent of 8a |
CH3CN |
71 |
16 |
6 W green LED instead of 7 W blue LED |
CH3CN |
73 |
17 |
24 W blue LED instead of 7 W blue LED |
CH3CN |
54 |
18 |
3 W blue LED instead of 7 W blue LED |
CH3CN |
51 |
Having optimized ideal reaction parameters in hand, we next demonstrated the scope of the reaction by varying aromatic amine derivatives (9). The aromatic aniline bearing electron withdrawing groups such as –CF3, –CN and –CO2Me tolerated the photochemical reaction and provided the arylated indazole products 10d, 10e and 10w respectively, in good yields. The carbon–halogen (C–X) bond was intact in the photochemical reaction and corresponding products were obtained in good yields. These halogen containing substrates (10a–c) are very useful for further synthetic elaborations with the help of cross coupling reactions. The aniline substrates with electron donating groups such as –Me and –OMe provided lower yield than that of electron withdrawing as well as halogen groups. This is probably due to the stabilization of aryl radical formed from in situ generated phenyldiazonium salt. The electron donating groups on aniline lowers the reactivity by stabilizing the electron deficiency on aryl radical. Next, steric factor on substrate was tested and ortho substituted compounds lowered the yield (10j–l) than para substituted aniline precursor (10c, 10a & 10e). Aliphatic amine and heterocyclic amine like isopropyl amine and 4-amino pyridine respectively did not participate in the reaction (entry 21, 26, 27, Scheme 1).
The scope of the reaction was then performed by varying different 2N substitution on indazole. The halogen groups at para position on this 2N phenyl ring lower the yield of the product (10m & 10n) compared to electron donating groups such as –Me and –OMe (10p & 10q). Methoxy substituted indazole (8i) also participated in the ideal condition and provided the corresponding product (10x). Ortho methyl substitution provided the product 10y in trace quantity. This is because of the steric hindrance that destabilize the cationic intermediate V in Scheme 4. Similarly, benzylated compounds (entries 19 and 20, Scheme 1) gave very poor yields of arylated indazoles 10s (30%) and 10t (27%) respectively. On the other hand, pyridyl substitution on 2N position, aliphatic amine and heterocyclic amine did not show any product formation (10u, 10z, 10aa).
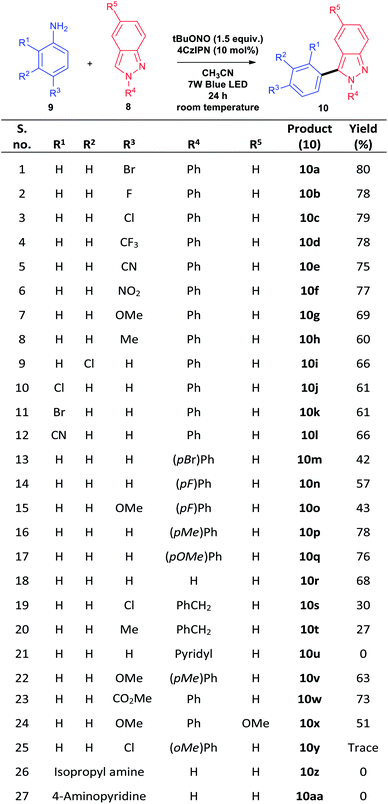 |
| Scheme 1 Scope of the organophotocatalyzed arylation on 2H-indazole derivatives. Reaction conditions: 1 equiv. of 9a (0.6 mmol), 2 equiv. of 8a (1.2 mmol), 1.5 equiv. of tBuONO (0.9), 10 mol% of catalyst, room temperature, 24 h. bIsolated yield. | |
In order to check out the robustness of the photochemical reaction, we performed a reaction with 1.0 g scale of indazole 8a under the optimized reaction condition and the product 10a was obtained in 78%. During this reaction, the catalyst was recovered with help of silica gel column chromatography. The recovered catalyst 7a was used for arylation of indazole 8a in the presence of p-chloroaniline (9c) and the corresponding functionalized product 10c was obtained in excellent yield (Scheme 2, eqn (b)). Next, we focussed on control experiments to find out the reaction path. As the entry shows in Scheme 3, aniline (9a) and indazole (8a) were reacted in the presence of diazotizing agent tBuONO and in acetonitrile solvent without light and without any catalyst at room temperature (Scheme 3, eqn (a)). The expected arylated product 10a was obtained, however with low yield of 32% which is indicating the need of a catalyst. It has been clear from eqn (b) and (c) in Scheme 3 that photoactivation of the catalyst 4CzIPN (7a) is necessary for this transformation. To understand the reactivity of the counter ion of phenyldiazonium salt, we performed a reaction with p-bromophenyldiazonium salt 11 (Scheme 3, eqn (d)) in the standard reaction conditions. We confirmed that anions –BF4− and –tBuO− have not shown any difference in the reaction conversion. To confirm the formation of Electron Donor–Acceptor (EDA) complex between catalyst and reactant if any, a UV-Vis spectroscopy study was performed. As expected, no shift in UV-Vis absorbance of the mixtures (Scheme 3, eqn (e)). When we perform a reaction using TEMPO under the standard conditions, we found the formation of TEMPO trapped radical species 10ab/10ac (Scheme 3, eqn (f)). The aryl radical is formed only from diazo compound which is confirmed by a control experiment performed in the absence of tBuONO (Scheme 3, eqn (g)). No product formation was formed in the absence of tBuONO.
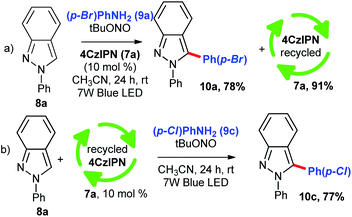 |
| Scheme 2 Recycling the catalyst. | |
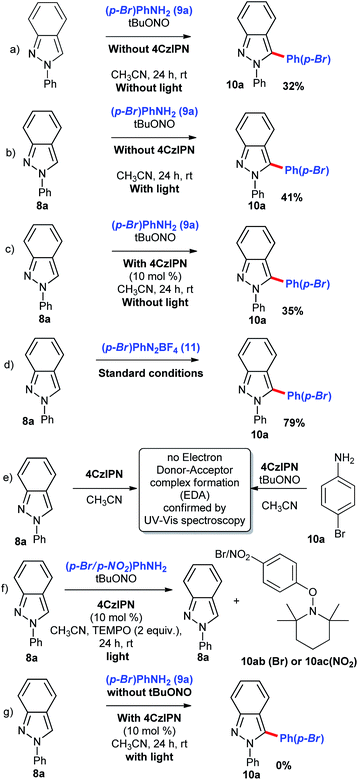 |
| Scheme 3 Control experiments. | |
Based on the control experiments and the scope of the reactions, we propose a possible reaction mechanism for the formation of indazole 10a (Scheme 4). Aniline (9) reacts with tert-butyl nitrite and converts into diazonium salt I. Upon oxidation of the reduced carbazole catalyst 7b′, diazonium salt I undergoes Single Electron Transfer (SET) process to produce aryl radical II, nitrogen, tBuO− and 4CzIPN (7a). The phenyl radical reacts with indazole 8a in a homolytic aromatic substitution (HAS) reaction to generate intermediate III. On the other hand, the catalyst 4CzIPN (7a) is activated by the 7 W blue LED light and then oxidizes the intermediate III through SET. While completing the catalytic cycle, the reaction generates carbocation species IV. Based on the yield we obtained for 2N substituted indazole, we propose the resonance stabilization involved in the carbocation intermediate IV and V as shown in Scheme 4. Finally, the deprotonation of V with the help of tBuO− produces the indazole 10a.
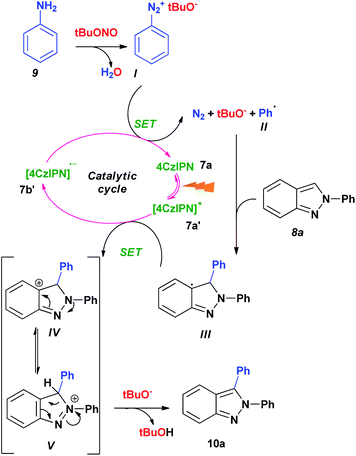 |
| Scheme 4 Possible reaction mechanism. | |
Experimental
General procedure for the synthesis of 3-(4-bromophenyl)-2-phenyl-2H-indazole 10a
Into a mixture of 0.6 mmol of 9a and 1.2 mmol of 8a in 1 ml of acetonitrile was added 10 mol% of catalyst 7a and 1.2 equivalent of tBuONO at room temperature. The reaction mixture was then stirred at room temperature for 24 h. Then the reaction mixture was then quenched with dichloromethane and water. Organic layer was separated and dried using sodium sulphate and concentrated under reduced pressure. The residue was purified by column chromatography using hexane to afford the indazole compound 10a.
Conclusions
In conclusion, 4CzIPN (7a) catalyst has been successfully involved in the metal-free photoarylation of 2H indazole derivatives with anilines. The reaction involves simple and mild operation conditions. The strategy employs anilines as arylating reagent which is safe and easy to handle than the diazonium salt which is involved in the reported procedure as source of arylation. The more sustainable energy of visible light mediated photoarylation using 4CzIPN produces the indazole derivatives in good to excellent yields.
Conflicts of interest
There are no conflicts to declare.
Acknowledgements
S. R. sincerely thanks DST-SERB, Government of India, New Delhi for financial support under DST INSPIRE Faculty Program (Grant No. DST/INSPIRE/04-I/2017/000002). R. S. thanks SASTRA Deemed University, Thanjavur, India for the research fellowship.
Notes and references
-
(a) S. G. Zhang, C. G. Liang and W. H. Zhang, Molecules, 2018, 23, 2783 CrossRef PubMed
;
(b) I. Denya, S. F. Malan and J. Joubert, Expert Opin. Ther. Pat., 2018, 28, 441 CrossRef CAS PubMed
;
(c) A. S. A. K. Chakraborty, N. Upmanyu and A. Singh, Austin J. Anal. Pharm. Chem., 2016, 3, 1076 Search PubMed
;
(d) D. D. Gaikwad, A. D. Chapolikar, C. G. Devkate, K. D. Warad, A. P. Tayade, R. P. Pawar and A. J. Domb, Eur. J. Med. Chem., 2015, 90, 707 CrossRef CAS PubMed
;
(e) Y. Jia, J. Zhang, J. Feng, F. Xu, H. Pan and W. Xu, Chem. Biol. Drug Des., 2014, 83, 306 CrossRef CAS PubMed
;
(f) A. Schmidt, A. Beutler and B. Snovydovych, Eur. J. Org. Chem., 2008, 2008, 4073 CrossRef
;
(g) A. Jennings and M. Tennant, J. Chem. Inf. Model., 2007, 47, 1829 CrossRef CAS PubMed
;
(h) H. Cerecetto, A. Gerpe, M. Gonzalez, V. J. Aran and C. O. de Ocariz, Mini-Rev. Med. Chem., 2005, 5, 869 CrossRef CAS PubMed
. - W. Han, J. C. Pelletier and C. N. Hodge, Bioorg. Med. Chem. Lett., 1998, 8, 3615 CrossRef CAS PubMed
. - G. Picciola, F. Ravenna, G. Carenini, P. Gentili and M. Riva, Farmaco, Ed. Sci., 1981, 36, 1037 CAS
. - X. Li, S. Chu, V. A. Feher, M. Khalili, Z. Nie, S. Margosiak, V. Nikulin, J. Levin, K. G. Sprankle, M. E. Tedder, R. Almassy, K. Appelt and K. M. Yager, J. Med. Chem., 2003, 46, 5663 CrossRef CAS PubMed
. -
(a) P. G. Baraldi, G. Balboni, M. G. Pavani, G. Spalluto, M. A. Tabrizi, E. D. Clercq, J. Balzarini, T. Bando, H. Sugiyama and R. Romagnoli, J. Med. Chem., 2001, 44, 2536 CrossRef CAS PubMed
;
(b) S. Qian, J. Cao, Y. Yan, M. Sun, H. Zhu, Y. Hu, Q. He and B. Yang, Mol. Cell. Biochem., 2010, 345, 13 CrossRef CAS PubMed
. - C. K. Chung, P. G. Bulger, B. Kosjek, K. M. Belyk, N. Rivera, M. E. Scott, G. R. Humphrey, J. Limanto, D. C. Bachert and K. M. Emerson, Org. Process Res. Dev., 2014, 18, 215 CrossRef CAS
. -
(a) Y. Cheng, G. Li, Y. Liu, Y. Shi, G. Gao, D. Wu, J. Lan and J. You, J. Am. Chem. Soc., 2016, 138, 4730 CrossRef CAS PubMed
;
(b) Y. Lian, R. G. Bergman, L. D. Lavis and J. A. Ellman, J. Am. Chem. Soc., 2013, 135, 7122 CrossRef CAS PubMed
;
(c) M. De Angelis, F. Stossi, K. A. Carlson, B. S. Katzenellenbogen and J. A. Katzenellenbogen, J. Med. Chem., 2005, 48, 1132 CrossRef CAS PubMed
;
(d) L. J. Huang, M. L. Shih, H. S. Chen, S. L. Pan, C. M. Teng, F. Y. Lee and S. C. Kuo, Bioorg. Med. Chem., 2006, 14, 528 CrossRef CAS PubMed
. - W. Frank and S. Chutian, PCT Int. Appl., WO 2014040373 A1 20140320, 2014 Search PubMed
. -
(a) F.-X. Felpin and S. Sengupta, Chem. Soc. Rev., 2019, 48, 1150 RSC
;
(b) A. Shamsabadi and V. Chudasama, Org. Biomol. Chem., 2019, 17, 2865 RSC
. -
(a) S. A. Sadler, A. C. Hones, B. Roberts, D. Blakemore, T. B. Marder and P. G. Steel, J. Org. Chem., 2015, 80, 5308–5314 CrossRef CAS PubMed
;
(b) F. Belkessam, M. Aidene, J. F. Soule and H. Doucet, ChemCatChem, 2017, 9, 2239 CrossRef CAS
;
(c) K. Hattori, K. Yamaguchi, J. Yamaguchi and K. Itami, Tetrahedron, 2012, 68, 7605 CrossRef CAS
;
(d) S. A. Ohnmacht, A. J. Culshaw and M. F. Greaney, Org. Lett., 2010, 12, 224 CrossRef CAS PubMed
;
(e) K. C. C. Aganda, J. Kim and A. Lee, Org. Biomol. Chem., 2019, 17, 9698 RSC
;
(f) S. Vidyacharan, B. T. Ramanjaneyulu, S. Jang and D.-P. Kim, ChemSusChem, 2019, 12, 2581 CrossRef CAS PubMed
;
(g) K. Basu, M. Poirier and R. T. Ruck, Org. Lett., 2016, 18, 3218 CrossRef CAS PubMed
. - S. Ghosh, S. Mondal and A. Hajra, Adv. Synth. Catal., 2020, 362, 3768 CrossRef CAS
. - A. Dahiya, A. K. Sahoo, T. Alam and B. K. Patel, Chem.–Asian J., 2019, 14, 4454 CrossRef CAS PubMed
. -
(a) M. K. Bogdos, E. Pinard and J. A. Murphy, Beilstein J. Org. Chem., 2018, 14, 2035 CrossRef CAS PubMed
;
(b) S. G. E. Amos, M. Garreau, L. Buzzetti and J. Waser, Beilstein J. Org. Chem., 2020, 16, 1163 CrossRef CAS PubMed
. - L. Marzo, S. K. Pagire, O. Reiser and B. Konig, Angew. Chem., Int. Ed., 2018, 57, 10034 CrossRef CAS PubMed
. - T. Y. Shang, L. H. Lu, Z. Cao, Y. Liu, W. M. He and B. Yu, Chem. Commun., 2019, 55, 5408 RSC
. - R. Saritha, S. B. Annes, S. Saravanan and S. Ramesh, Org. Biomol. Chem., 2020, 18, 2510 RSC
. -
(a) J. Luo and J. Zhang, ACS Catal., 2016, 6, 873–877 CrossRef CAS
;
(b) K. Donabauer, M. Maity, A. L. Berger, G. S. Huff, S. Crespi and B. Konig, Chem. Sci., 2019, 10, 5162 RSC
.
Footnote |
† Electronic supplementary information (ESI) available. See DOI: 10.1039/d1ra02372a |
|
This journal is © The Royal Society of Chemistry 2021 |
Click here to see how this site uses Cookies. View our privacy policy here.