DOI:
10.1039/D1RA02276H
(Paper)
RSC Adv., 2021,
11, 17587-17594
Polymerization of a biscalix[5]arene derivative†
Received
22nd March 2021
, Accepted 9th May 2021
First published on 13th May 2021
Abstract
Recent decades have seen an increased interest in the preparation of polymers possessing host or guest moieties as the end group, which has enabled new polymeric materials such as self-healable, shape-memory, and stimuli-responsive materials. Such polymers are commonly synthesized by tethering the host or guest moieties to polymers. On the other hand, there are limited reports demonstrating the preparation of host- or guest-appended polymers by directly polymerizing the corresponding host- or guest-appended monomers, which is valuable for easy access to diverse polymers from single molecular species. However, reactive host and/or guest moieties of the monomer interfere with the polymerization reaction. Here, we report that a biscalix[5]arene host-appended molecule can be polymerized with various monomers to form the corresponding host-appended polymers. The host–guest complexation behavior of calix[5]arene-appended polymers with fullerene derivatives was studied by 1H NMR and UV/Vis spectroscopic techniques, which revealed that the long polymer chains did not prevent host–guest complexation even when the fullerene derivative was equipped with a polymer chain. Thus, the present study shows the potential for developing polymers that have various combinations of polymer chains.
Introduction
Increased interest in the combination of supramolecular chemistry and polymer science has emerged in recent years. The introduction of host–guest moieties onto the polymer termini endows the polymers with a dynamic nature, leading to the development of functional soft materials.1,2 In addition, the host–guest approach has enabled easy access to distinctly shaped polymers such as block, star, and brush polymers.3–9 Macrocyclic hosts, e.g., cyclodextrins,10–16 crown ethers,17–21 pillararenes,22–25 calixpyrroles,26–28 calixarenes29–33 and others,34–36 have been commonly adopted as host moieties due to their high binding affinity toward the corresponding guests. To date, many polymeric materials relying on host–guest complexation between macrocyclic hosts and the corresponding guests have been reported, which has corroborated that the host–guest approach is a powerful tool for the development of new polymeric materials, including self-healable, shape-memory, and stimuli-responsive materials.37–40
To fully leverage the potential of host- or guest-appended polymers, challenges still remain in their synthetic pathway to be addressed. Polymers possessing a host or guest moiety as the end group have been commonly prepared by tethering the host or guest moieties to polymers. On the other hand, there are limited reports describing the preparation of host- or guest-appended polymers by directly polymerizing the corresponding host- or guest-appended monomers.41,42 Although the latter synthetic pathway is valuable for easy access to diverse polymers from single molecular species, reactive host and/or guest moieties of the monomer prevent the polymerization reaction. We thus sought to examine whether an appropriately designed host-appended monomer molecule can be polymerized to form the corresponding host-appended polymers.
Our group has developed structurally well-defined supramolecular architectures using host–guest interactions between calix[5]arene and fullerene,43–45 one of which corroborated that the strong calix[5]arene–fullerene association can be used for tethering long polymer chains.46 In this work, we designed calix[5]arene-appended monomer 1 with a 2-bromoisobutylate moiety as an atom transfer radical polymerization (ATRP) initiator (Fig. 1). 1 was subjected to ATRP reaction with various methacrylate monomers to produce corresponding calix[5]arene-appended polymers.
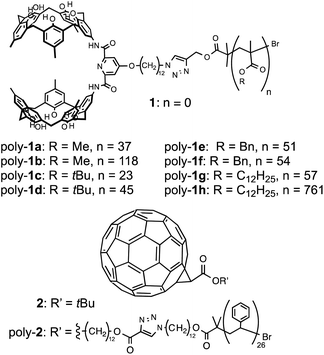 |
| Fig. 1 Molecular structures of a calix[5]arene monomer, calix[5]arene polymers and fullerene derivatives. | |
Results and discussion
The syntheses of 1 and poly-2 are outlined in Schemes 1 and 2. 3 (ref. 47) was prepared according to a reported method. Azidation of 3 and subsequent hydrolysis afforded 5 in good yield. 5 converted into corresponding acid chloride, which was subjected to condensation reaction with separately prepared 6 (ref. 44) to afford biscalix[5]arene monoazide 7 in 94% yield. The copper mediated cyclization reaction between 7 and 8 (ref. 48) yielded the target monomer molecule 1 in 52% yield. The synthesis of poly-2 commenced with the polymerization reaction of a known compound 9.46 The ATRP reaction of 9 with styrene monomer successfully proceeded to give poly-9. The copper mediated cyclization reaction between poly-9 and 10 (ref. 49) furnished desired poly-2.
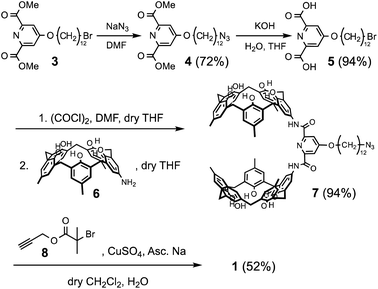 |
| Scheme 1 Synthesis of 1. DMF = N,N-dimethylformamide, THF = tetrahydrofuran, Asc.Na = sodium ascorbate. | |
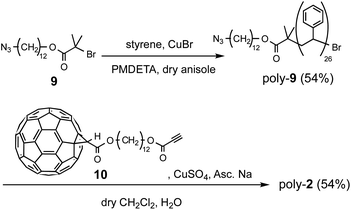 |
| Scheme 2 Synthesis of poly-2. PMDETA = N,N,N′,N′′,N′′-pentamethyl-diethylenetriamine. | |
The polymerizations of 1 with the methacrylate monomers were conducted at two different mixing ratios, as summarized in Scheme 3 and Table 1. The ATRP reaction proceeded for methyl methacrylate to afford the corresponding polymers poly-1a,b. When 1 was mixed with 100 equiv. of methyl methacrylate monomer, poly-1a (Mn = 5250, PDI = 1.28) was given by the polymerization reaction (entry 1). A higher molecular weight was achieved (poly-1b; Mn = 13
400, PDI = 1.24) when the reaction was performed in the presence of 1000 equiv. of methyl methacrylate monomer (entry 2). 1 was polymerized with tert-butyl and benzyl methacrylate monomers to yield the corresponding polymers poly-1c–f (entries 3–6). Fair increases in the molecular weight were observed for the polymerizations with tert-butyl and benzyl methacrylate monomers when the mixing ratio of [1]
:
[monomer] was increased from 1
:
100 to 1
:
1000. Polymers with large molecular weights were produced by the polymerization reaction with n-dodecyl methacrylate monomer (entries 7 and 8). The conversion yield of the methacrylate monomers to the polymers surpasses that of the previously reported polymerization reactions of host or guest appended monomer molecules.41,42 These results indicate that the calix[5]arene moiety does not interfere with the ATRP reaction, which allows access to polymers with various molecular structures in their side chains from single monomeric species.
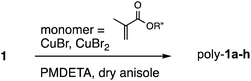 |
| Scheme 3 Polymerization of 1 with methacrylate monomers. The results were summarized in Table 1. | |
Table 1 Summary of the results of polymerization of 1 under various conditions. Me = methyl. tBu = tert-butyl. Bn = benzyl
Entrya |
Monomer |
Equiv.b |
Resulting polymer |
Mnc (g mol−1) |
PDIc |
Kad (L mol−1) |
Detailed synthetic procedures are shown in the ESI. Equivalent methacrylate monomers used for polymerization. Determined by size-exclusion chromatography (SEC) based on polystyrene standards in chloroform. Binding constant between the resulting polymer and 2. |
1 |
R′′ = Me |
100 |
Poly-1a |
5250 |
1.28 |
26 000 ± 1000 |
2 |
R′′ = Me |
1000 |
Poly-1b |
13 400 |
1.24 |
15 000 ± 1000 |
3 |
R′′ = tBu |
100 |
Poly-1c |
4820 |
1.20 |
17 000 ± 300 |
4 |
R′′ = tBu |
1000 |
Poly-1d |
7960 |
1.23 |
15 300 ± 600 |
5 |
R′′ = Bn |
100 |
Poly-1e |
10 600 |
1.47 |
15 000 ± 500 |
6 |
R′′ = Bn |
1000 |
Poly-1f |
11 000 |
1.47 |
13 000 ± 1000 |
7 |
R′′ = n-C12H25 |
100 |
Poly-1g |
16 000 |
1.49 |
20 500 ± 400 |
8 |
R′′ = n-C12H25 |
1000 |
Poly-1h |
195 000 |
1.21 |
19 700 ± 700 |
The difference in the resulting molecular weight is most likely due to the solubility of polymers toward the anisole solvent. For example, the solubility of the benzyl methacrylate polymer gradually decreased upon increasing the degree of polymerization. When approximately 55 monomers were incorporated into the polymer chain through the ATRP reaction, the benzyl methacrylate polymer may have precipitated out, which results in the termination of the polymerization process to yield poly-1e,f. Thus, despite the initial concentration of methyl methacrylate, approximately 55 equiv. of benzyl methacrylate was consumed by the polymerization reaction. The termination of the polymerization reaction in anisole by an increase in methacrylate content within the polymer chain was seen in the methyl and tert-butyl methacrylate polymers (poly-1a–d). On the other hand, independent of the initial concentration of n-dodecyl methacrylate, ≈60–75% methacrylate was incorporated into the resulting polymer poly-1g,h. The n-dodecyl methacrylate polymer had good solubility owing to the long dodecyl side chain, which supports the efficient proceeding of the ATRP reaction; thus, a larger degree of polymerization was observed in the n-dodecyl methacrylate polymer (poly-1g,h) than in the other methacrylate polymers (poly-1a–f).
The binding ability of the end groups within poly-1a–h was studied using UV/Vis spectroscopy. 2 exhibited a discernible absorption band up to 700 nm with characteristic peaks at 329 and 427 nm and a broad band at 501 nm in chloroform (Fig. 2a and S24†). An increase in the absorption band at approximately 450 nm was observed upon the addition of poly-1a. No discernible absorption band was observed at 430 nm in the solution of poly-1a; thus, the increase in the UV/Vis spectra of 2 suggests that the calix[5]arene–fullerene host–guest complex is formed in solution. The binding constant of (2.6 ± 0.1) × 104 L mol−1 was estimated by a 1
:
1 fitting model50 based on the changes in the absorption intensity at 430 nm. Increases in the intensity of absorption were also seen in the solutions of 2 upon the addition of poly-1b–h (Fig. S25–S31†), indicating the formation of calix[5]arene–fullerene host–guest complexes in solution. The binding constant between poly-1b–h and 2 was estimated to be on the order of 104 L mol−1 (Table 1). The binding constants are close to those observed in the host–guest complexes between biscalix[5]arenes and C60 derivatives;43,46,51 thus, these results indicate that the long polymer chains do not impact the binding affinity between calix[5]arene and fullerene.
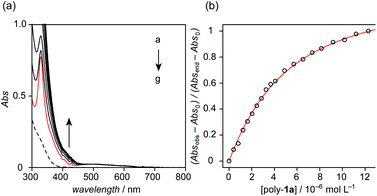 |
| Fig. 2 (a) Changes in the UV/Vis absorption spectrum of 2 (2.0 × 10−5 mol L−1) upon the addition of poly-1a. The concentrations of poly-1a are (a–g) 0.0, 2.0, 4.0, 6.4, 8.0, 10, and 12 × 10−5 mol L−1, estimated from its Mn. The red line and broken line indicate the absorption spectra of poly-1a (2.0 × 10−5 mol L−1) and 2 (2.0 × 10−5 mol L−1), respectively. The spectra were recorded in chloroform at 25 °C. (b) Plot of normalized ΔAbs (400 nm) against [poly-1a] and the fitting curve obtained by a 1 : 1 fitting model, where Absobs, Abs0, and Absend denote the observed Abs at each concentration, the Abs of poly-1a, and the Abs of poly-1a in the presence of 2 (12 × 10−5 mol L−1), respectively. | |
The 1H NMR study supported the formation of calix[5]arene–fullerene host–guest complexes in solution. Calix[5]arene stably exists in a cone conformation due to the intramolecular cyclic hydrogen bonding of five phenolic hydroxyl groups.44,52 The calix[5]arene ring undergoes ring inversion at room temperature, which is slow on the NMR timescale. Therefore, the methylene protons give a broad 1H NMR resonance at room temperature. A characteristic broad resonance appeared at approximately 4 ppm in the chloroform-d solution of poly-1c,53 which is assignable to bridge methylene protons of calix[5]arene moieties (Fig. 3a). When 1.0 equiv. of 2 was added to the chloroform solution, the broad resonance split into two resonances (Fig. 3b). The change in the resonance reflects that the ring inversion of calix[5]arene moieties became slower, which comes from an increase in the energetic barrier of the ring inversion by the encapsulation of fullerene molecules in the calix[5]arene cavity.
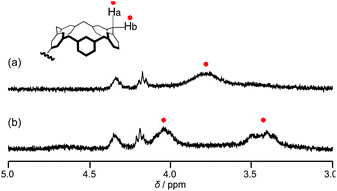 |
| Fig. 3 Expanded 1H NMR spectra of poly-1c (a) in the absence and (b) in the presence of 2 in chloroform-d. | |
To gain detailed insight into calix[5]arene–fullerene complexation, time-dependent changes in UV/Vis absorption were monitored. A chloroform solution of a 1
:
1 mixture of 1 and 2 was placed in a quartz cuvette, and the absorbance value at 427 nm was recorded every 80 s. A gradual increase in the absorption value was observed in the solution (Fig. S32†). When the measurement time reached approximately 10 min, the increase in the absorption value was saturated. On the other hand, the absorbance value of 427 nm of a 1
:
1 mixture of poly-1b and 2 slowly increased and saturated after approximately 20 min. Assuming that all 2 molecules were consumed by the association at 3000 s, the association rate constants of 1 and 2 and poly-1b and 2 were estimated to be (330 ± 30) × 10−3 L mol−1 s−1 and (140 ± 10) × 10−3 L mol−1 s−1, respectively (Fig. 4).54 The slower association between poly-1b and 2 most likely comes from the long methyl methacrylate chains of poly-1b. The long methacrylate chain of poly-1b fairly prevented 2 from approaching its calix[5]arene cavity.
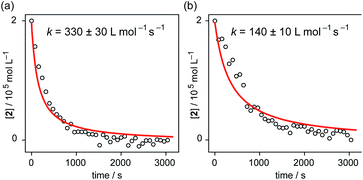 |
| Fig. 4 Time-dependent concentration changes of 2 seen in (a) an equimolar mixture of 1 and 2 and (b) an equimolar mixture of poly-1b and 2 in chloroform. The concentrations of 2 were estimated by the absorbance value at 427 nm. | |
Finally, the formation of a styrene-methacrylate diblock polymer was studied using poly-1c and poly-2 (Fig. 5a and S33†). Analogous to 2, poly-2 exhibited a discernible absorption band up to 700 nm with characteristic peaks at 329 and 429 nm. Upon the addition of poly-1c, the absorption band at approximately 420 nm gradually became intense, which indicates the formation of a calix[5]arene–fullerene host–guest complex in solution. The 1
:
1 fitting model gave rise to a binding constant of (2.4 ± 0.2) × 104 L mol−1 (Fig. 5b). The binding constant is in the same order of magnitude as that of a biscalix[5]arene–C60 complex reported.29 This result indicates that the end groups of calix[5]arene and fullerene within the polymer chains can recognize each other to form host–guest complexes, which is not prevented by the polymer chain.
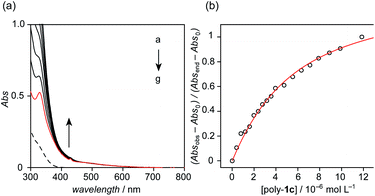 |
| Fig. 5 (a) Changes in the UV/Vis absorption spectrum of poly-2 (2.0 × 10−5 mol L−1) upon the addition of poly-1c. The concentrations of poly-1c are (a–g) 0.0, 2.0, 4.0, 6.3, 8.0, 10, and 12 × 10−5 mol L−1 estimated from its Mn. The red line and broken line indicate the absorption spectra of poly-1c (2.0 × 10−5 mol L−1) and poly-2 (2.0 × 10−5 mol L−1), respectively. The spectra were recorded in toluene at 25 °C. (b) Plot of normalized ΔAbs (400 nm) against [poly-1c] and the fitting curve obtained by a 1 : 1 fitting model, where Absobs, Abs0, and Absend denote the observed Abs at each concentration, the Abs of poly-1c, and the Abs of poly-1c in the presence of poly-2 (12 × 10−5 mol L−1), respectively. | |
Conclusions
In summary, methacrylate polymers were successfully prepared via an ATRP reaction using a calix[5]arene-appended monomer. The complexation capability between the calix[5]arene moiety and fullerene molecule was determined by UV/Vis absorption spectroscopy and NMR spectroscopy. Time-dependent UV analysis revealed that a long methacrylate chain fairly prevented fullerene from approaching the calix[5]arene cavity, although the polymer chains did not influence the binding affinity between calix[5]arene and fullerene. In addition, host–guest complexation was observed in a mixture of calix[5]arene-appended polymethacrylate and fullerene-appended polystyrene, which indicates that the high binding affinity between calix[5]arene and fullerene is effective in developing styrene-methacrylate diblock polymers. The present work shows the potential for developing various diblock polymers.
Experimental section
General
All reagents and solvents were commercial reagent grade and were used without further purification unless otherwise noted. Dry anisole and dry N,N-dimethylformamide (DMF) were obtained by distillation over CaH2. Methacrylate monomers were passed through aluminum oxide before being used for the polymerization reactions. The styrene monomer was washed with saturated aqueous NaOH, and the resulting oil was distillated over CaH2 under the reduced pressure before being used for the polymerization reaction. 1H and 13C NMR spectra were recorded on a Varian mercury-300 spectrometer, and chemical shifts were reported on the delta scale in ppm relative to residual chloroform (δ = 7.26 and 77.0 for 1H and 13C, respectively). UV/Vis absorption spectra were recorded on a JASCO V-760 spectrometer. High resolution mass spectra (HRMS) were recorded on a Thermo Scientific LTQ Orbitrap XL hybrid FTMS by electron splay ionization (ESI) methods. Melting points (Mp) were measured with a Yanagimoto micro melting point apparatus. Infrared (IR) spectra were recorded on a JASCO FT/IR-4600 spectrometer with ZeSe ATR accessory. Preparative separations were performed by silica gel gravity column chromatography (Silica Gel 60 N (spherical, neutral)). Recycling preparative GPC-HPLC separations were carried out on JAI LC-5060 using preparative JAIGEL-2.5HH, 2HH, columns in series. Size exclusion chromatogram was recorded on Shimadzu LC-20AC with CTO-20AC accessory and TOSOH UV-8011 detector using preparative Shodex GPC-K-804, K-803, K-802.5 columns in series. The number-average molecular weights (Mn) of the poly methyl methacrylates (PMMAs) were determined by the size exclusion chromatography (SEC) based on polystyrene standards in chloroform. 3,47 6,44 8,48 9 (ref. 46) and 10 (ref. 49) were synthesized according to reported methods.
Dimethyl 4-((12-azidododecyl)oxy)pyridine-2,6-dicarboxylate (4)
To a solution of 3 (1.56 g, 3.38 mmol) in dry DMF (33.8 mL) was added NaN3 (440 mg, 6.77 mmol). After being stirred overnight at 100 °C under an argon atmosphere, the reaction mixture was diluted with ethyl acetate, and passed through celite. The organic layer was washed with saturated aqueous NaCl, dried over anhydrous Na2SO4, and concentrated in vacuo. The crude product was purified by column chromatography on silica gel (0–60% ethyl acetate in n-hexane, eluent) gave desired product 4 as a white solid (1.02 g, 72%). Mp 124–126 °C; 1H NMR (300 MHz, CDCl3): 7.79 (s, 2H), 4.13 (t, J = 7.2 Hz, 2H), 4.00 (s, 6H), 3.25 (t, J = 7.7 Hz, 2H), 1.79–1.88 (m, 2H), 1.53–1.64 (m, 2H), 1.21–1.48 (m, 16H) ppm; 13C NMR (75 MHz, CDCl3): δ 167.2, 165.2, 149.7, 114.6, 69.1, 53.2, 51.5, 29.5, 29.4, 29.2, 29.1, 28.8, 28.7, 26.7, 25.8 ppm; FTIR-ATR (KBr): ν 2920, 2854, 2103, 1735, 1723, 1595, 1463, 1435, 1345, 1370, 1313, 1246, 1099, 1056, 1011, 757 cm−1; HRMS (ESI+) calcd for C21H33N4O5 m/z 421.24455 [M + H]+, found m/z 421.24432.
4-((12-Azidododecyl)oxy)pyridine-2,6-dicarboxylic acid (5)
To a solution of 4 (1.00 g, 2.38 mmol) in THF (23.8 mL) was added KOH (668 mg, 11.9 mmol) diluted with water (23.8 mL). After being stirred for 2 h at 40 °C under an argon atmosphere, the reaction mixture was added ethyl acetate and water. The water layer was washed with ethyl acetate, and acidified with 1 M hydrochloric acid to pH 1. The precipitate was filtered off, washed with water, and dried over in vacuo to give desired product 5 as a white solid (874 mg, 94%). Mp 144–146 °C; 1H NMR (300 MHz, CD3OD): 7.82 (s, 2H), 4.22 (t, J = 7.1 Hz, 2H), 3.26 (t, J = 7.6 Hz, 2H), 1.80–1.89 (m, 2H), 1.48–1.62 (m, 4H), 1.32–1.42 (m, 14H) ppm; 13C NMR (75 MHz, CD3OD): δ 168.2, 165.5, 148.8, 113.7, 69.2, 51.0, 29.2, 28.9, 28.8, 28.5, 28.4, 26.4, 25.5 ppm; FTIR-ATR (KBr): ν 3503, 3096, 2928, 2851, 2096, 1923, 1728, 1597, 1464, 1329, 1185, 1035, 683 cm−1; HRMS (ESI−) calcd for C19H27N4O5 m/z 391.19869 [M − H]−, found m/z 391.19836.
((12-Azidododecyl)oxy)biscalix[5]arene 7
To a solution of 5 (480 mg, 1.20 mmol) and dry DMF (60 μL, 0.060 mmol) in dry THF (60 mL) was added oxalyl chloride (1.03 mL, 12.0 mmol) at 0 °C. After being stirred for 2 h at room temperature under an argon atmosphere, the reaction mixture was concentrated in vacuo. The crude product in dry THF (60 mL) was added 6 (1.80 g, 2.99 mmol). After being stirred for 24 h at room temperature under an argon atmosphere, the reaction mixture was concentrated in vacuo. The crude product was diluted with ethyl acetate. The precipitate was filtered off, washed with ethyl acetate, and dried over in vacuo to give desired product 7 as a yellow solid (1.76 g, 94%). Mp >300 °C; 1H NMR (300 MHz, CDCl3): 9.37 (s, 2H), 8.81 (s, 10H), 7.90 (s, 2H), 7.61 (s, 4H), 6.90–7.02 (m, 16H), 4.16 (t, J = 7.3 Hz, 2H), 3.40–4.00 (m, 20H), 3.25 (t, J = 7.7 Hz, 2H), 2.23 (s, 12H), 2.11 (s, 12H), 1.91 (s, 6H), 1.77–1.90 (m, 2H), 1.52–1.65 (m, 2H), 1.41–1.52 (m, 2H), 1.20–1.41 (m, 14H) ppm; 13C NMR (75 MHz, CDCl3): δ 168.1, 161.5, 151.21, 147.8, 147.7, 130.8, 130.6, 129.8, 129.7, 127.5, 126.5, 126.4, 125.8, 121.8, 111.5, 69.2, 51.5, 31.5, 31.3, 29.5, 29.2, 29.2, 28.8, 28.7, 20.5, 20.4 ppm; FTIR-ATR (KBr): ν 3279, 3012, 2924, 2856, 2095, 1684, 1599, 1540, 1483, 1457, 1344, 1288, 1226, 1035, 859, 780 cm−1; HRMS (ESI+) calcd for C97H103N6O13 m/z 1559.75776 [M + H]+, found m/z 1559.75970.
Biscalix[5]arene-appended monomer 1
To a solution of 7 (400 mg, 0.26 mmol) in CH2Cl2 (13 mL) and water (3.3 mL) were added 8 (110 mg, 0.51 mmol), sodium ascorbate (Asc.Na) (100 mg, 0.51 mmol), and CuSO4 (82 mg, 0.51 mmol). After being stirred for 48 h at room temperature under an argon atmosphere, the reaction mixture was diluted with CH2Cl2. The organic layer was washed with water, dried over anhydrous Na2SO4, and concentrated in vacuo. The crude product was purified by column chromatography on silica gel (0–20% ethyl acetate in toluene, eluent) and was passed through HPLC (10–12.5% ethyl acetate in toluene, eluent) gave desired product 1 as a yellow solid (240 mg, 52%). Mp >300 °C; 1H NMR (300 MHz, CDCl3): 9.36 (s, 2H), 9.08 (s, 2H), 8.80 (s, 4H), 8.78 (s, 4H), 7.91 (s, 2H), 7.60 (s, 4H), 7.60 (s, 1H), 6.92–7.02 (m, 16H), 5.31 (s, 2H), 4.32 (t, J = 8.0 Hz, 2H), 4.16 (t, J = 7.0 Hz, 2H), 3.40–4.00 (m, 20H), 2.22 (s, 12H), 2.11 (s, 12H), 1.91 (s, 6H), 1.78–1.90 (m, 4H), 1.40–1.53 (m, 2H), 1.20–1.40 (m, 14H) ppm; 13C NMR (75 MHz, CDCl3): δ 171.5, 168.2, 161.5, 151.21, 147.8, 147.7, 142.2, 130.8, 130.6, 130.6, 129.9, 129.7, 127.5, 126.5, 126.5, 126.4, 125.8, 123.6, 121.9, 111.6, 69.1, 59.2, 55.7, 50.4, 31.6, 31.3, 30.6, 30.2, 29.5, 29.4, 29.4, 29.2, 29.0, 26.4, 25.8, 20.4, 20.4 ppm; FTIR-ATR (KBr): ν 3231, 3009, 2922, 2855, 1734, 1684, 1600, 1549, 1481, 1456, 1378, 1347, 1283, 1224, 1156, 1102, 1035, 856, 790, 691 cm−1; HRMS (ESI+) calcd for C104H112BrN6O15 m/z 1763.73636 [M + H]+, found m/z 1763.73911.
Poly-1a
1 (20.0 mg, 11.3 μmol), dry anisole (240 μL), and methyl methacrylate (MMA) (130 μL, 1.22 mmol, Nacalai Tesque, Inc., Japan, ≥99%) were placed in a Schlenk-flask. After three cycles of freeze–pump–thaw, CuBr (1.8 mg, 13 μmol), CuBr2 (1.4 mg, 6.3 μmol), and N,N,N′,N′′,N′′-pentamethyl-diethylenetriamine (PMDETA) (5.1 μL, 25 μmol) were added in sequence to the resulting solution under a nitrogen atmosphere. After being stirred for 3 h in the dark at 65 °C, the resulting mixture was further stirred for 1 h at room temperature under an open-air atmosphere. The resulting mixture was diluted with CH2Cl2, passed through aluminum oxide 90 (CH2Cl2, eluent), and then concentrated in vacuo. The crude product was purified by reprecipitation from CH2Cl2 solution with n-hexane to afford desired product poly-1a as a white sold (16.5 mg, 28%). 1H NMR (300 MHz, CDCl3): δ 9.31 (s, 2H), 9.08 (s, 2H), 8.79 (s, 8H), 7.92 (s, 2H), 7.58 (s, 4H), 7.57 (s, 1H), 6.93–6.99 (m, 16H), 5.18 (s, 2H), 4.33 (m, 2H), 4.16 (m, 2H), 3.40–4.00 (m, 20H), 3.59 (m, 3H × n), 2.22 (s, 12H), 2.13 (s, 12H), 1.72–2.04 (m, 4H + 2H × n), 1.16–1.49 (m, 22H), 0.70–1.14 (m, 3H × n) ppm; Mn,SEC = 5252 g mol−1, PDI = 1.28.
Poly-1b
1 (20.0 mg, 11.3 μmol), dry anisole (240 μL), and methyl methacrylate (MMA) (1.30 mL, 12.2 mmol, Nacalai Tesque, Inc., Japan, ≥99%) were placed in a Schlenk-flask. After three cycles of freeze–pump–thaw, CuBr (1.8 mg, 13 μmol), CuBr2 (1.4 mg, 6.3 μmol), and N,N,N′,N′′,N′′-pentamethyl-diethylenetriamine (PMDETA) (5.1 μL, 25 μmol) were added in sequence to the resulting solution under a nitrogen atmosphere. After being stirred for 3 h in the dark at 65 °C, the resulting mixture was further stirred for 1 h at room temperature under an open-air atmosphere. The resulting mixture was diluted with CH2Cl2, passed through aluminum oxide 90 (CH2Cl2, eluent), and then concentrated in vacuo. The crude product was purified by reprecipitation from CH2Cl2 solution with n-hexane to afford desired product poly-1b as a white sold (85 mg, 56%). 1H NMR (300 MHz, CDCl3): δ 9.29 (s, 2H), 9.09 (s, 2H), 8.79 (s, 8H), 7.93 (s, 2H), 7.58 (s, 4H), 7.58 (s, 1H), 6.94–7.01 (m, 16H), 5.18 (s, 2H), 4.33 (m, 2H), 4.14 (m, 2H), 3.40–4.00 (m, 20H), 3.59 (m, 3H × n), 2.22 (s, 12H), 2.14 (s, 12H), 1.70–2.04 (m, 4H + 2H × n), 1.16–1.52 (m, 22H), 0.71–1.12 (m, 3H × n) ppm; Mn,SEC = 13
353 g mol−1, PDI = 1.24.
Poly-1c
1 (40.0 mg, 22.6 μmol), dry anisole (480 μL), and tert-butyl methacrylate (tBMA) (395 μL, 2.44 mmol, Tokyo Chemical Industry Co., Ltd., Japan, >98%) were placed in a Schlenk-flask. After three cycles of freeze–pump–thaw, CuBr (3.5 mg, 25 μmol), CuBr2 (2.7 mg, 12 μmol), and N,N,N′,N′′,N′′-pentamethyl-diethylenetriamine (PMDETA) (10.2 μL, 48.9 μmol) were added in sequence to the resulting solution under a nitrogen atmosphere. After being stirred for 3 h in the dark at 65 °C, the resulting mixture was further stirred for 1 h at room temperature under an open-air atmosphere. The resulting mixture was diluted with CH2Cl2, passed through aluminum oxide 90 (CH2Cl2, eluent), and then concentrated in vacuo. The crude product was purified by GPC to give desired product poly-1c as a white sold (69.7 mg, 64%). 1H NMR (300 MHz, CDCl3): δ 9.29 (s, 2H), 9.09 (s, 2H), 8.79 (s, 8H), 7.93 (s, 2H), 7.58 (s, 4H), 7.58 (s, 1H), 6.94–7.01 (m, 16H), 5.18 (s, 2H), 4.34 (m, 2H), 4.17 (m, 2H), 3.40–4.00 (m, 20H), 2.22 (s, 12H), 2.14 (s, 12H), 1.70–2.14 (m, 4H + 2H × n), 0.84–1.51 (9H × n), 1.21–1.51 (m, 22H), 0.84–1.19 (m, 3H × n) ppm; Mn,SEC = 4821 g mol−1, PDI = 1.20.
Poly-1d
1 (20.0 mg, 11.3 μmol), dry anisole (240 μL), and tert-butyl methacrylate (tBMA) (1.97 mL, 12.2 mmol, Tokyo Chemical Industry Co., Ltd., Japan, >98%) were placed in a Schlenk-flask. After three cycles of freeze–pump–thaw, CuBr (1.8 mg, 13 μmol), CuBr2 (1.4 mg, 6.3 μmol), and N,N,N′,N′′,N′′-pentamethyl-diethylenetriamine (PMDETA) (5.1 μL, 25 μmol) were added in sequence to the resulting solution under a nitrogen atmosphere. After being stirred for 3 h in the dark at 65 °C, the resulting mixture was further stirred for 1 h at room temperature under an open-air atmosphere. The resulting mixture was diluted with CH2Cl2, passed through aluminum oxide 90 (CH2Cl2, eluent), and then concentrated in vacuo. The crude product was purified by GPC to give desired product poly-1d as a white sold (56.4 mg, 58%). 1H NMR (300 MHz, CDCl3): δ 9.29 (s, 2H), 8.81 (s, 10H), 8.62 (s, 4H), 7.93 (s, 2H), 7.58 (s, 4H), 7.58 (s, 1H), 6.94–7.00 (m, 16H), 5.18 (s, 2H), 4.33 (m, 2H), 4.18 (m, 2H), 3.40–4.00 (m, 20H), 2.22 (s, 12H), 2.14 (s, 12H), 1.70–2.14 (m, 4H + 2H × n), 0.80–1.50 (9H × n), 1.21–1.49 (m, 22H), 0.80–1.14 (m, 3H × n) ppm; Mn,SEC = 7957 g mol−1, PDI = 1.23.
Poly-1e
1 (20.0 mg, 11.3 μmol), dry anisole (240 μL), and benzyl methacrylate (BnMA) (205 μL, 1.21 mmol, KYOEISHA CHEMICAL, Co., Inc., Japan, >97%) were placed in a Schlenk-flask. After three cycles of freeze–pump–thaw, CuBr (1.8 mg, 13 μmol), CuBr2 (1.4 mg, 6.3 μmol), and N,N,N′,N′′,N′′-pentamethyl-diethylenetriamine (PMDETA) (5.1 μL, 25 μmol) were added in sequence to the resulting solution under a nitrogen atmosphere. After being stirred for 3 h in the dark at 65 °C, the resulting mixture was further stirred for 1 h at room temperature under an open-air atmosphere. The resulting mixture was diluted with CH2Cl2, passed through aluminum oxide 90 (CH2Cl2, eluent), and then concentrated in vacuo. The crude product was purified by reprecipitation from CH2Cl2 solution with methanol and by GPC to give desired product poly-1e as a white sold (67.1 mg, 52%). 1H NMR (300 MHz, CDCl3): δ 9.28 (s, 2H), 9.09 (s, 2H), 8.79 (s, 8H), 7.93 (s, 2H), 7.58 (s, 4H), 7.58 (s, 1H), 7.15–7.53 (m, 5H × n), 6.94–7.01 (m, 16H), 5.16 (s, 2H), 4.74–5.13 (m, 2H × n), 4.27 (m, 2H), 4.16 (m, 2H), 3.40–4.00 (m, 20H), 3.50 (m, 3H × n), 2.22 (s, 12H), 2.14 (s, 12H), 1.65–2.08 (m, 4H + 2H × n), 1.19–1.41 (m, 22H), 0.60–1.17 (m, 3H × n) ppm; Mn,SEC = 10
572 g mol−1, PDI = 1.47.
Poly-1f
1 (20.0 mg, 11.3 μmol), dry anisole (240 μL), and benzyl methacrylate (BnMA) (2.05 mL, 12.1 mmol, KYOEISHA CHEMICAL, CO., Inc., Japan, >97%) were placed in a Schlenk-flask. After three cycles of freeze–pump–thaw, CuBr (1.8 mg, 13 μmol), CuBr2 (1.4 mg, 6.3 μmol), and N,N,N′,N′′,N′′-pentamethyl-diethylenetriamine (PMDETA) (5.1 μL, 25 μmol) were added in sequence to the resulting solution under a nitrogen atmosphere. After being stirred for 3 h in the dark at 65 °C, the resulting mixture was further stirred for 1 h at room temperature under an open-air atmosphere. The resulting mixture was diluted with CH2Cl2, passed through aluminum oxide 90 (CH2Cl2, eluent), and then concentrated in vacuo. The crude product was purified by reprecipitation from CH2Cl2 solution with methanol and by GPC to give desired product poly-1f as a white sold (114 mg, 85%). 1H NMR (300 MHz, CDCl3): δ 9.28 (s, 2H), 9.10 (s, 2H), 8.79 (s, 8H), 7.93 (s, 2H), 7.58 (s, 4H), 7.58 (s, 1H), 7.11–7.54 (m, 5H × n), 6.93–7.04 (m, 16H), 5.20 (s, 2H), 4.73–5.16 (m, 2H × n), 4.28 (m, 2H), 4.17 (m, 2H), 3.40–4.00 (m, 20H), 2.22 (s, 12H), 2.14 (s, 12H), 1.65–2.08 (m, 4H + 2H × n), 1.20–1.41 (m, 22H), 0.50–1.18 (m, 3H × n) ppm; Mn,SEC = 10
953 g mol−1, PDI = 1.42.
Poly-1g
1 (20.0 mg, 11.3 μmol), dry anisole (240 μL), and dodecyl methacrylate (355 μL, 1.22 mmol, KYOEISHA CHEMICAL, CO., Inc., Japan, >98%) were placed in a Schlenk-flask. After three cycles of freeze–pump–thaw, CuBr (1.8 mg, 13 μmol), CuBr2 (1.4 mg, 6.3 μmol), and N,N,N′,N′′,N′′-pentamethyl-diethylenetriamine (PMDETA) (5.1 μL, 25 μmol) were added in sequence to the resulting solution under a nitrogen atmosphere. After being stirred for 3 h in the dark at 65 °C, the resulting mixture was further stirred for 1 h at room temperature under an open-air atmosphere. The resulting mixture was diluted with CH2Cl2, passed through aluminum oxide 90 (CH2Cl2, eluent), and then concentrated in vacuo. The crude product was purified by reprecipitation from CH2Cl2 solution with methanol and by GPC to give desired product poly-1g as a white sold (65.5 mg, 36%). 1H NMR (300 MHz, CDCl3): δ 9.29 (s, 2H), 9.09 (s, 2H), 8.79 (s, 8H), 7.92 (s, 2H), 7.58 (s, 4H), 7.56 (s, 1H), 6.94–7.01 (m, 16H), 5.16 (s, 2H), 4.32 (m, 2H), 4.16 (m, 2H), 3.40–4.00 (m, 20H), 3.91 (m, 2H × n), 2.22 (s, 12H), 2.14 (s, 12H), 1.71–2.07 (m, 4H + 4H × n), 1.13–1.51 (m, 22H + 18H × n), 0.70–1.13 (m, 6H × n) ppm; Mn,SEC = 16
006 g mol−1, PDI = 1.49.
Poly-1h
1 (20.0 mg, 11.3 μmol), dry anisole (240 μL), and dodecyl methacrylate (3.55 mL, 12.2 mmol, KYOEISHA CHEMICAL, CO., Inc., Japan, >98%) were placed in a Schlenk-flask. After three cycles of freeze–pump–thaw, CuBr (1.8 mg, 13 μmol), CuBr2 (1.4 mg, 6.3 μmol), and N,N,N′,N′′,N′′-pentamethyl-diethylenetriamine (PMDETA) (5.1 μL, 25 μmol) were added in sequence to the resulting solution under a nitrogen atmosphere. After being stirred for 3 h in the dark at 65 °C, the resulting mixture was further stirred for 1 h at room temperature under an open-air atmosphere. The resulting mixture was diluted with CH2Cl2, passed through aluminum oxide 90 (CH2Cl2, eluent), and then concentrated in vacuo. The crude product was purified by reprecipitation from CH2Cl2 solution with methanol and by GPC to give desired product poly-1h as a white sold (1.42 g, 60%). 1H NMR (300 MHz, CDCl3): δ 9.29 (s, 2H), 9.09 (s, 2H), 8.79 (s, 8H), 7.93 (s, 2H), 7.58 (s, 4H), 7.58 (s, 1H), 6.94–7.00 (m, 16H), 5.19 (s, 2H), 4.32 (m, 2H), 4.17 (m, 2H), 3.40–4.00 (m, 20H), 3.91 (m, 2H × n), 2.21 (s, 12H), 2.13 (s, 12H), 1.69–2.04 (m, 4H + 4H × n), 1.14–1.58 (m, 22H + 18H × n), 0.60–1.13 (m, 6H × n) ppm; Mn,SEC = 195
197 g mol−1, PDI = 1.21.
Poly-9
9 (110 mg, 0.30 mmol), dry anisole (3.0 mL), and styrene (17.2 mL, 1.22 mmol, Nacalai Tesque, Inc., Japan, ≥99%) were placed in a Schlenk-flask. After three cycles of freeze–pump–thaw, CuBr (43 mg, 0.30 mmol) and N,N,N′,N′′,N′′-pentamethyl-diethylenetriamine (PMDETA) (94 μL, 0.45 mol) were added in sequence to the resulting solution under a nitrogen atmosphere. After being stirred for 24 h in the dark at 80 °C, the resulting mixture was further stirred for 1 h at room temperature under an open-air atmosphere. The resulting mixture was diluted with CH2Cl2, passed through aluminum oxide 90 (CH2Cl2, eluent), and then concentrated in vacuo. The crude product was purified by reprecipitation from CH2Cl2 solution with methanol and by GPC to give desired product poly-9 as a white sold (500 mg, 54%). 1H NMR (300 MHz, CDCl3): δ 6.31–7.37 (m, 5H × n), 4.34–4.57 (m, 2H), 3.47–3.61 (m, 3H), 3.26 (t, J = 7.8 Hz, 2H), 0.80–2.14 (m, 26H + 3H × (n − 1)) ppm; Mn,SEC = 3118 g mol−1, PDI = 1.08.
Poly-2
To a solution of poly-9 (60 mg) in CH2Cl2 (1.1 mL) and water (0.3 mL) were added 8 (23.5 mg, 23.0 μmol), sodium ascorbate (Asc.Na) (4.6 mg, 23 μmol), and CuSO4 (3.7 mg, 23 μmol). After being stirred for 48 h at room temperature under an argon atmosphere, the reaction mixture was diluted with CH2Cl2. The organic layer was washed with water, dried over anhydrous Na2SO4, and concentrated in vacuo. The crude product was purified by GPC to give desired product poly-2 as brown solid (51 mg, 54%). 1H NMR (300 MHz, CDCl3): δ 8.04 (s, 1H), 6.31–7.75 (m, 5H × n), 4.79 (s, 1H), 4.31–4.50 (m, 6H), 4.11–4.21 (m, 2H), 3.44–3.61 (m, 3H), 0.80–2.14 (m, 46H + 3H × (n − 1)) ppm; Mn,SEC = 4109 g mol−1, PDI = 1.02.
Conflicts of interest
There are no conflicts to declare.
Acknowledgements
We are grateful to the Natural Science Center for Basic Research Development (N-BARD) and Hiroshima University for HRMS measurements. This work was supported by a Grant-in-Aid for Young Scientists, JSPS KAKENHI (20K15335 granted to T. Hirao); a Grant-in-Aid for Scientific Research on Innovative Areas, JSPS KAKENHI (JP19H04585: coordination asymmetry, granted to T. Haino); and a Grant-in-Aid for Challenging Exploratory Research, JSPS KAKENHI (20K21196 granted to T. Haino). Funding from the Mazda Foundation, the Iketani Science and Technology Foundation, the Ube Industries Foundation, the Asahi Glass Foundation, the Kao Foundation for Arts and Science, the Sumitomo Foundation, the Nagase Science Technology Foundation, the Research Foundation for Opto-Science and Technology, the Electric Technology Research Foundation of Chugoku, and the Iwatani Naoji Foundation is gratefully acknowledged.
Notes and references
- P. Cordier, F. Tournilhac, C. Soulié-Ziakovic and L. Leibler, Nature, 2008, 451, 977–980 CrossRef CAS PubMed.
- M. Burnworth, L. Tang, J. R. Kumpfer, A. J. Duncan, F. L. Beyer, G. L. Fiore, S. J. Rowan and C. Weder, Nature, 2011, 472, 334–337 CrossRef CAS PubMed.
- T. Park and S. C. Zimmerman, J. Am. Chem. Soc., 2006, 128, 11582–11590 CrossRef CAS PubMed.
- H. Ohkawa, G. B. W. L. Ligthart, R. P. Sijbesma and E. W. Meijer, Macromolecules, 2007, 40, 1453–1459 CrossRef CAS.
- A. Bertrand, F. Lortie and J. Bernard, Macromol. Rapid Commun., 2012, 33, 2062–2091 CrossRef CAS PubMed.
- M. C. Stuparu, A. Khan and C. J. Hawker, Polym. Chem., 2012, 3, 3033–3044 RSC.
- B. V. K. J. Schmidt and C. Barner-Kowollik, Angew. Chem., Int. Ed., 2017, 56, 8350–8369 CrossRef CAS PubMed.
- S. Bhaumik, K. Ntetsikas and N. Hadjichristidis, Macromolecules, 2020, 53, 6682–6689 CrossRef CAS PubMed.
- W. Yasen, R. Dong, A. Aini and X. Zhu, J. Mater. Chem. B, 2020, 8, 8219–8231 RSC.
- Z.-X. Zhang, X. Liu, F. J. Xu, X. J. Loh, E.-T. Kang, K.-G. Neoh and J. Li, Macromolecules, 2008, 41, 5967–5970 CrossRef CAS.
- A. Harada, R. Kobayashi, Y. Takashima, A. Hashidzume and H. Yamaguchi, Nat. Chem., 2011, 3, 34–37 CrossRef CAS PubMed.
- B. V. K. J. Schmidt, T. Rudolph, M. Hetzer, H. Ritter, F. H. Schacher and C. Barner-Kowollik, Polym. Chem., 2012, 3, 3139–3145 RSC.
- Z.-X. Zhang, K. L. Liu and J. Li, Angew. Chem., Int. Ed., 2013, 52, 6180–6184 CrossRef CAS PubMed.
- Y. Zheng, A. Hashidzume and A. Harada, Macromol. Rapid Commun., 2013, 34, 1062–1066 CrossRef CAS PubMed.
- J. Wang, X. Wang, F. Yang, H. Shen, Y. You and D. Wu, Langmuir, 2014, 30, 13014–13020 CrossRef CAS PubMed.
- S. Nomimura, M. Osaki, J. Park, R. Ikura, Y. Takashima, H. Yamaguchi and A. Harada, Macromolecules, 2019, 52, 2659–2668 CrossRef CAS.
- F. Huang, D. S. Nagvekar, C. Slebodnick and H. W. Gibson, J. Am. Chem. Soc., 2005, 127, 484–485 CrossRef CAS PubMed.
- M. Lee, R. B. Moore and H. W. Gibson, Macromolecules, 2011, 44, 5987–5993 CrossRef CAS.
- D. Liu, D. Wang, M. Wang, Y. Zheng, K. Koynov, G. K. Auernhammer, H.-J. Butt and T. Ikeda, Macromolecules, 2013, 46, 4617–4625 CrossRef CAS.
- X. Ji, J. Chen and M. Xue, Macromol. Chem. Phys., 2014, 215, 536–543 CrossRef CAS.
- M. A. Soto and J. Tiburcio, Chem. Commun., 2016, 52, 14149–14152 RSC.
- S. Sun, X.-Y. Hu, D. Chen, J. Shi, Y. Dong, C. Lin, Y. Pan and L. Wang, Polym. Chem., 2013, 4, 2224–2229 RSC.
- W. Xia, M. Ni, C. Yao, X. Wang, D. Chen, C. Lin, X.-Y. Hu and L. Wang, Macromolecules, 2015, 48, 4403–4409 CrossRef CAS.
- V. Kardelis, K. Li, I. Nierengarten, M. Holler, J.-F. Nierengarten and A. Adronov, Macromolecules, 2017, 50, 9144–9150 CrossRef CAS.
- Y. Wu, H. Li, Y. Yan, X. Shan, M. Zhao, Q. Zhao, X. Liao and M. Xie, ACS Macro Lett., 2019, 8, 1588–1593 CrossRef CAS.
- E. S. Silver, B. M. Rambo, C. W. Bielawski and J. L. Sessler, J. Am. Chem. Soc., 2014, 136, 2252–2255 CrossRef CAS PubMed.
- X. Ji, C. Guo, X.-S. Ke, X. Chi, M. D. Moore and J. L. Sessler, Chem. Commun., 2017, 53, 9648 RSC.
- X. Ji, W. Chen, L. Long, F. Huang and J. L. Sessler, Chem. Sci., 2018, 9, 7746–7752 RSC.
- T. Haino, E. Hirai, Y. Fujiwara and K. Kashihara, Angew. Chem., Int. Ed., 2010, 49, 7899–7903 CrossRef CAS PubMed.
- A. Pappalardo, F. P. Ballistreri, G. L. Destri, P. G. Mineo, G. A. Tomaselli, R. M. Toscano and G. Trusso Sfrazzetto, Macromolecules, 2012, 45, 7549–7556 CrossRef CAS.
- Y. Tsunoda, K. Fukuta, T. Imamura, R. Sekiya, T. Furuyama, N. Kobayashi and T. Haino, Angew. Chem., Int. Ed., 2014, 53, 7243–7247 CrossRef CAS PubMed.
- N. Nitta, M. Takatsuka, S.-i. Kihara, R. Sekiya and T. Haino, ACS Macro Lett., 2018, 7, 1308–1311 CrossRef CAS.
- N. Nitta, M. Takatsuka, S.-i. Kihara, T. Hirao and T. Haino, Angew. Chem., Int. Ed., 2020, 59, 16690–16697 CrossRef CAS PubMed.
- M. Fumagalli, K. Belal, H. Guo, F. Stoffelbach, G. Cooke, A. Marcellan, P. Woisel and D. Hourdet, Soft Matter, 2017, 13, 5269–5282 RSC.
- X. Ji, R.-T. Wu, L. Long, X.-S. Ke, C. Guo, Y.-J. Ghang, V. M. Lynch, F. Huang and J. L. Sessler, Adv. Mater., 2018, 30, 1705480 CrossRef PubMed.
- J. Liu, C. S. Y. Tan and O. A. Scherman, Angew. Chem., Int. Ed., 2018, 57, 8854–8858 CrossRef CAS PubMed.
- B. Gyarmati, B. Á. Szilágyi and A. Szilágyi, Eur. Polym. J., 2017, 93, 642–669 CrossRef CAS.
- X. Ji, M. Ahmed, L. Long, N. M. Khashab, F. Huang and J. L. Sessler, Chem. Soc. Rev., 2019, 48, 2682–2697 RSC.
- G. Sinawang, M. Osaki, Y. Takashima, H. Yamaguchi and A. Harada, Polym. J., 2020, 52, 839–859 CrossRef CAS.
- J. Wankar, N. G. Kotla, S. Gera, S. Rasala, A. Pandit and Y. A. Rochev, Adv. Funct. Mater., 2020, 30, 1909049 CrossRef CAS.
- E. Elacqua, A. Croom, K. B. Manning, S. K. Pomarico, D. Lye, L. Young and M. Weck, Angew. Chem., Int. Ed., 2016, 55, 15873–15878 CrossRef CAS PubMed.
- R. Deng, M. Milton, S. K. Pomarico and M. Weck, Polym. Chem., 2019, 10, 5087–5093 RSC.
- T. Haino, Y. Matsumoto and Y. Fukazawa, J. Am. Chem. Soc., 2005, 127, 8936–8937 CrossRef CAS PubMed.
- T. Hirao, M. Tosaka, S. Yamago and T. Haino, Chem.–Eur. J., 2014, 20, 16138–16146 CrossRef CAS PubMed.
- T. Hirao, H. Kudo, T. Amimoto and T. Haino, Nat. Commun., 2017, 8, 634 CrossRef PubMed.
- T. Hirao, K. Fukuta and T. Haino, Macromolecules, 2020, 53, 3563–3570 CrossRef CAS.
- C. Adam, L. Faour, V. Bonnin, T. Breton, E. Levillain, M. Sallé, C. Gautier and D. Canevet, Chem Commun., 2019, 55, 8426–8429 RSC.
- D. M. Henn, W. Fu, S. Mei, C. Y. Li and B. Zhao, Macromolecules, 2017, 50, 1645–1656 CrossRef CAS.
- H. Yan, S. Chen, M. Lu, X. Zhu, Y. Li, D. Wu, Y. Tu and X. Zhu, Mater. Horiz., 2014, 1, 247–250 RSC.
- The biscalix[5]arenes are known to favorably form 1
:
1 complexes with fullerene and methano fullerenes (see ref. 46). - T. Hirao, Y. Iwabe, N. Fujii and T. Haino, J. Am. Chem. Soc., 2021, 143, 4339–4345 CrossRef CAS PubMed.
- T. Haino, M. Yanase, C. Fukunaga and Y. Fukazawa, Tetrahedron, 2006, 62, 2025–2035 CrossRef CAS.
- Since the appearance of the protons of the polymethacrylates, it is difficult to monitor the bridge methylene protons of calix[5]arene moieties of poly-1a,b, and poly-1e–h.
- Rate equation (second order):
where k is the rate constant, [A] is the concentration of 2, and [A0] is the initial concentration of 2.
Footnotes |
† Electronic supplementary information (ESI) available. See DOI: 10.1039/d1ra02276h |
‡ These authors contributed equally to this work. |
|
This journal is © The Royal Society of Chemistry 2021 |
Click here to see how this site uses Cookies. View our privacy policy here.