DOI:
10.1039/D1RA01063H
(Paper)
RSC Adv., 2021,
11, 11490-11494
Rhodium(III)-catalyzed annulation of enamides with sulfoxonium ylides toward isoquinolines†
Received
8th February 2021
, Accepted 12th March 2021
First published on 19th March 2021
Abstract
An efficient rhodium(III)-catalyzed C–H activation followed by intermolecular annulation between enamides and sulfoxonium ylides has been developed. The transformation proceeds smoothly with a broad range of substrates, affording a series of isoquinoline derivatives in moderate to good yields under additive-free conditions.
Introduction
Isoquinolines represent important structural motifs frequently found in natural products, functional materials and pharmaceuticals.1 Their unusual bioactive properties, such as antispasmodic,2 antiinflammatory,3 antihypertensive4 and antitumour5 activities, have attracted much attention (Fig. 1). Consequently, the alternative efficient synthetic methodology for isoquinolines is of great importance.6 Traditional methods, including the famous Bischler–Napieralski,7 Pictet–Gams8 and Pictet–Spengler9 reactions, are well-established. Most of these protocols suffer from harsh reaction conditions and environmental problems. In this regard, transition metal-catalyzed C–H activation/annulation would be valuable and complementary to the known classic methodology, enabling the direct access to a series of isoquinoline derivatives with minimum environmental impact and fewer synthetic steps.10–13 On the other hand, sulfoxonium ylides are attractive starting materials that can be easily accessed and widely be utilized as transition metal-carbene precursors in coupling reactions.14,15 The use of sulfoxonium ylide in the synthesis of isoquinoline through Rh(III)-catalyzed C–H activation was reported by Li and co-workers. In their transformation, the ortho C–H bond of amidines is activated by rhodium catalyst to give isoquinolines with sulfoxonium ylides in the presence of 30% Zn(OTf)2 (Scheme 1a).16 A ruthenium-catalyzed mono ortho-C–H annulation of benzimidates with sulfoxonium ylides was developed for the synthesis of substituted isoquinolines by Wang group in the presence of mesitylenic acid (Scheme 1b).17 Very recently, preparation of isoquinoline derivatives by Rh(III)-catalyzed coupling reaction of benzylamine and sulfoxonium ylides using water as solvent is achieved by Wu and coworkers (Scheme 1c).18
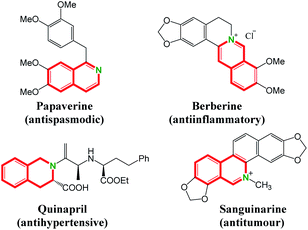 |
| Fig. 1 Representative bioactive isoquinolines. | |
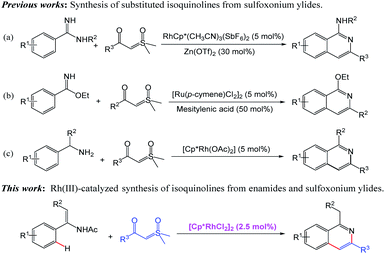 |
| Scheme 1 Synthetic strategies toward isoquinolines. | |
Enamides are valuable building blocks in organic synthesis having tunable reactivity and potential usage in various transformations.19 The potential of enamide chemistry has been witnessed by transition-metal-catalyzed coupling reactions.20 As part of our continuing interest in metal-catalyzed enamide-directed C–H functionalization reaction and the synthesis of heterocyclic compounds,21 herein, we present a novel Rh(III)-catalyzed cascade transformation from enamides and sulfoxonium ylides for the preparation of isoquinolines. This method allows the approach of a range of diversified 1,3-disubstituted isoquinolines with moderate to good yields under mild conditions without the use of additives.
Results and discussion
We initiated our investigation on the model reaction of enamide (1a) and sulfoxonium ylide (2a) to optimize various reaction parameters. The results have been summarized in Table 1. At the outset, our study was treated by enamide (1a) with sulfoxonium ylide (2a) in the presence of [RhCp*Cl2]2/AgSbF6 and NaOAc in THF at 100 °C under N2 for 20 h (entry 1, Table 1). However, the target product 3aa was not detected in the reaction. Subsequently, when different solvents such as DMF, DCE and HFIP were studied on this reaction (entry 2–4, Table 1), we were pleased to find that the reaction occurred in HFIP, affording the desired annulation product 3aa in a yield of 25%. The experimental results show that the protic solvent-HFIP has a better promotion effect on the reaction. Different inorganic bases (LiOAc, KOAc, CsOAc and Cu(OAc)2) were then screened (entry 5–8, Table 1), but the results were no better than that obtained with NaOAc (entry 4, Table 1). The yield was improved to 46% when AgSbF6 was removed (compare entry 9 with entry 4, Table 1). Next, the yield increased to 71% in the absence of NaOAc (compare entry 14 with entry 9, Table 1). The other transition metal catalysts such as [Rh(cod)Cl]2 and RhCl3 were probed as well. The reaction results indicated that they were less effective than [RhCp*Cl2]2 (entry 15–16, Table 1). Control experiment showed that an absence of the Rh catalyst led to no formation of 3aa (entry 17, Table 1). The temperature reduction (80 °C) or elevation (120 °C) had an adverse effect (entry 18–19, Table 1). Therefore, the best result (71%) was achieved by using [RhCp*Cl2]2 (2.5 mol%) in HFIP at 100 °C under N2 for 20 h.
Table 1 Optimization of the reaction conditionsa

|
Entry |
Catalyst |
Additive/base |
Solvent |
Yieldb (%) |
Reaction conditions: 1a (0.3 mmol), 2a (0.2 mmol), [RhCp*Cl2]2 (2.5 mol%), additive (20 mol%), base (1.0 equiv.), solvent (2.0 mL), 100 °C, under N2, for 20 h. N.R. = no reaction. Isolated yields. 80 °C. 120 °C. |
1 |
[RhCp*Cl2]2 |
AgSbF6/NaOAc |
THF |
N.R. |
2 |
[RhCp*Cl2]2 |
AgSbF6/NaOAc |
DMF |
N.R. |
3 |
[RhCp*Cl2]2 |
AgSbF6/NaOAc |
DCE |
N.R. |
4 |
[RhCp*Cl2]2 |
AgSbF6/NaOAc |
HFIP |
25 |
5 |
[RhCp*Cl2]2 |
AgSbF6/LiOAc |
HFIP |
19 |
6 |
[RhCp*Cl2]2 |
AgSbF6/KOAc |
HFIP |
18 |
7 |
[RhCp*Cl2]2 |
AgSbF6/CsOAc |
HFIP |
20 |
8 |
[RhCp*Cl2]2 |
AgSbF6/Cu(OAc)2 |
HFIP |
5 |
9 |
[RhCp*Cl2]2 |
—/NaOAc |
HFIP |
46 |
10 |
[RhCp*Cl2]2 |
—/LiOAc |
HFIP |
30 |
11 |
[RhCp*Cl2]2 |
—/KOAc |
HFIP |
28 |
12 |
[RhCp*Cl2]2 |
—/CsOAc |
HFIP |
29 |
13 |
[RhCp*Cl2]2 |
—/Cu(OAc)2 |
HFIP |
12 |
14 |
[RhCp*Cl2]2 |
— |
HFIP |
71 |
15 |
[Rh(cod)Cl]2 |
— |
HFIP |
N.R. |
16 |
RhCl3 |
— |
HFIP |
N.R. |
17 |
— |
— |
HFIP |
N.R. |
18c |
[RhCp*Cl2]2 |
— |
HFIP |
53 |
19d |
[RhCp*Cl2]2 |
— |
HFIP |
65 |
With the optimized reaction conditions in hand, we then investigated the generality and scope of enamide. Diversified enamides bearing various aryl moieties substituted by electron-donating groups and electron-withdrawing groups reacted smoothly, affording the desired products in moderate to good yields. Among them, the enamide substrates with the para-position substituted by various electron-donating groups, such as –Me, –Et and –OMe could be smoothly converted into the desired products (Scheme 2, 3ba–3da). When the electron-withdrawing groups including –F, –Cl, –Br and –CF3 were introduced to the para-position of benzene ring of enamide, it was also tolerated to the standard reaction conditions, and gave the good yield (Scheme 2, 3ea–3ha). We further introduced –F and –CF3 groups into the 3-position of benzene ring of enamide 1a and good results were also obtained (Scheme 2, 3ia–3ja). However, the yield decreased markedly when ortho position of the benzene ring was substituted by –OMe or –F (Scheme 2, 3ka–3la). This may be due to steric effects. Interestingly, fused isoquinoline 3oa could also be obtained by this method in the yield of 60%. It was noteworthy that the methyl or phenyl substituted enamide on olefinic bond could also be converted into corresponding isoquinoline products 3pa or 3qa, which further expanding the scope of this protocol.
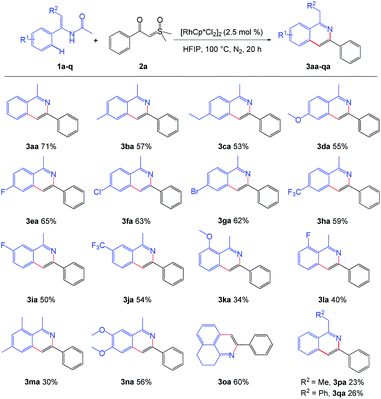 |
| Scheme 2 Scope of the enamides. a Reaction conditions: 1 (0.3 mmol), 2a (0.2 mmol), [RhCp*Cl2]2 (2.5 mol%), in HFIP (2.0 mL), at 100 °C, under N2, for 20 h. | |
Next, the scope of sulfoxonium ylides was examined and the results are summarized in Scheme 3. Both the electron-donating and electron-withdrawing groups of the phenyl rings tolerated well, giving the desired isoquinolines in moderate to good yields (Scheme 3, 3ab–3am). When the phenyl group in sulfoxonium ylide 2a was switched to alkyl group, the reaction still performed smoothly to give the corresponding products (Scheme 3, 3ao–3aq). However, when the phenyl ring was replaced by a furan ring, 3an was obtained in a lower yield.
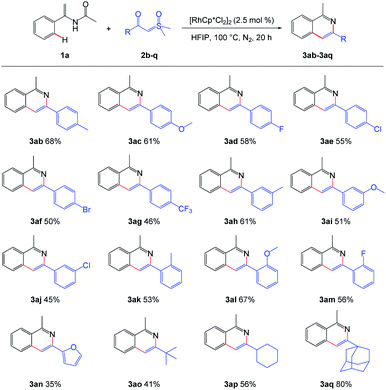 |
| Scheme 3 Scope of the sulfoxonium ylides. a Reaction conditions: 1a (0.3 mmol), 2 (0.2 mmol), [RhCp*Cl2]2 (2.5 mol%), in HFIP (2.0 mL), at 100 °C, under N2, for 20 h. | |
With the established substrate scope of the products, we conducted a series of experiments to investigate the possible mechanism. Initially, when the reaction of 1a was performed with D2O and sulfoxonium ylide 2a under standard conditions for 0.5 h, 25% of 1a were recovered and no deuterium was found at the ortho position of the benzene ring, showing no H/D exchange (Scheme 4a). It should indicate that the C–H bond activation of enamide might follow an irreversible process. Then, two parallel independent reactions (Scheme 4b) and the one-pot deuterium competition reaction (Scheme 4c) of substrates 1a and d5-1a were carried out, giving kH/kD values of 1.8 and 3.5 respectively. Both results indicate that the ortho C–H bond cleavage of enamide was likely involved in the turnover-limiting step. Moreover, the intermolecular competition experiment between electron-rich and electron-deficient enamides (1b vis 1e) shows a ratio of products 3ba and 3ea of 1
:
1.30 based on the yields, suggesting that the aryl Csp2–H bond activation possibly proceeded through concerted metallation–deprotonation (CMD) process instead of electrophilic rhodiumization pathway (Scheme 4d). Finally, the competitive coupling-cyclization of α-(4-methylbenzoyl)-sulfoxonium ylide (2b) and α-(4-fluorobenzoyl) sulfoxonium ylide (2d) with enamide (1a) led to the yields of 3ab and 3ad with a ratio of 1.14
:
1 based on the yields (Scheme 4e), indicating that electron-rich sulfoxonium ylide more easily forms a rhodium-carbene than the electron-deficient sulfoxonium ylide, which facilitates the formation of isoquinoline.
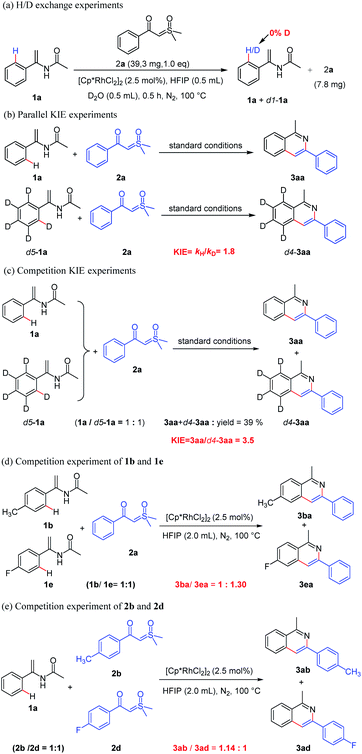 |
| Scheme 4 Mechanism study experiments. | |
On the basis of the above experimental results and precedent literatures,14d,15d,18 a plausible reaction mechanism was proposed as shown in Scheme 5. The process begins with coordination of nitrogen atom of enamide 1a to the rhodium atom of A and subsequent Csp2–H activation via the concerted metalation-deprotonation (CMD) afford the key five-membered rhodacycle C, which is trapped by the sulfoxonium ylide 2a to form the rhodium-carbene D through the elimination of DMSO. Next, migratory insertion of carbene species into the Rh–C(sp2) bond in the intermediate D provides a six-membered rhodacycle intermediate E. Finally, protonolysis of E produces the acylmethylated intermediate F, which undergoes successive addition, elimination and aromatization steps under the acidic conditions to afford the target product 3aa.
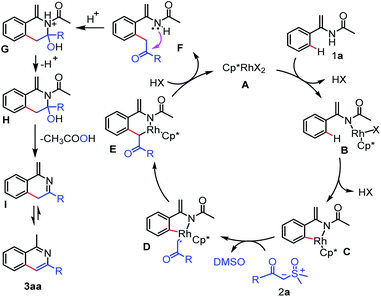 |
| Scheme 5 Proposed reaction pathway. | |
Conclusions
In summary, we have disclosed a novel strategy for the synthesis of isoquinolines via rhodium(III)-catalyzed C–H activation and annulation. The useful building blocks of enamides and sulfoxonium ylides are applied, and a range of substituted isoquinolines are prepared under mild reaction conditions. This versatile method needs not any additives such as silver salts and mesitylenic acid. Further investigation to expand the applications of enamides is underway in our laboratory.
Conflicts of interest
There are no conflicts to declare.
Acknowledgements
This research was supported by the Natural Science Foundation of China (No. 21672186) and Important Project of Zhejiang province (2020C01149).
Notes and references
- For selected examples, see:
(a) M. Croisy-Delcey, A. Croisy, D. Carrez, C. Huel, A. Chiaroni, P. Ducrot, E. Bisagni, L. Jin and G. Leclercq, Bioorg. Med. Chem., 2000, 8, 2629–2641 CrossRef CAS PubMed;
(b) D. Collado, E. Perez-Inestrosa, R. Suau, J.-P. Desvergne and H. Bouas-Laurent, Org. Lett., 2002, 4, 855–858 CrossRef CAS PubMed;
(c) Y. Kashiwada, A. Aoshima, Y. Ikeshiro, Y.-P. Chen, H. Furukawa, M. Itoigawa, T. Fujioka, K. Mihashi, L. M. Cosentino, S. L. Morris-Natschke and K.-H. Lee, Bioorg. Med. Chem., 2005, 13, 443–448 CrossRef CAS PubMed;
(d) Q. Zhao, S. Liu, M. Shi, C. Wang, M. Yu, L. Li, F. Li, T. Yi and C. Huang, Inorg. Chem., 2006, 45, 6152–6160 CrossRef CAS PubMed;
(e) K. W. Bentley, Nat. Prod. Rep., 2006, 23, 444–463 RSC.
- W.-S. Yu, Z.-N. Wu, Z.-F. Qiu, C.-J. Zhao, F.-L. Zhang and Z.-Z. Yang, Russ. J. Org. Chem., 2020, 56, 1295–1299 CrossRef CAS.
- L. M. Mori-Quiroz, S. L. Hedrick, A. R. De Los Santos and M. D. Clift, Org. Lett., 2018, 20, 4281–4284 CrossRef CAS PubMed.
- B. B. Borase, H. M. Godbole, G. P. Singh, P. R. Upadhyay, A. Trivedi, V. Bhat and G. G. Shenoy, Synth. Commun., 2020, 50, 48–55 CrossRef CAS.
- K. Bhadra and G. S. Kumar, Med. Res. Rev., 2011, 31, 821–862 CrossRef CAS PubMed.
- For a selected examples, see:
(a) Y.-N. Niu, Z.-Y. Yan, G.-L. Gao, H.-L. Wang, X.-Z. Shu, K.-G. Ji and Y.-M. Liang, J. Org. Chem., 2009, 74, 2893–2896 CrossRef CAS PubMed;
(b) R. He, Z.-T. Huang, Q.-Y. Zheng and C. Wang, Angew. Chem., Int. Ed., 2014, 53, 4950–4953 CrossRef CAS PubMed;
(c) Y. Yang, J.-X. Yu, X.-H. Ouyang and J.-H. Li, Org. Lett., 2015, 17, 3982–3985 CrossRef PubMed;
(d) J.-B. Feng and X.-F. Wu, Adv. Synth. Catal., 2016, 358, 2179–2185 CrossRef CAS;
(e) R. S. Phatake, P. Patel and C. V. Ramana, Org. Lett., 2016, 18, 292–295 CrossRef CAS PubMed;
(f) R. Gujjarappa, N. Vodnala and C. C. Malakar, Adv. Synth. Catal., 2020, 362, 4896–4990 CrossRef CAS.
- A. Bischler and B. Napieralski, Ber. Dtsch. Chem. Ges., 1893, 26, 1903–1908 CrossRef.
- A. Pictet and A. Gams, Ber. Dtsch. Chem. Ges., 1910, 43, 2384–2391 CrossRef CAS.
- A. Pictet and T. Spengler, Ber. Dtsch. Chem. Ges., 1911, 44, 2030–2036 CrossRef CAS.
- For selected examples of Rh species as catalyst, see:
(a) P. C. Too, Y.-F. Wang and S. Chiba, Org. Lett., 2010, 12, 5688–5691 CrossRef CAS PubMed;
(b) G. Song, D. Chen, C.-L. Pan, R. H. Crabtree and X. Li, J. Org. Chem., 2010, 75, 7487–7490 CrossRef CAS PubMed;
(c) L. Zheng, J. Ju, Y. Bin and R. Hua, J. Org. Chem., 2012, 77, 5794–5800 CrossRef CAS PubMed;
(d) Z. Shi, D. C. Koester, M. Boultadakis-Arapinis and F. Glorius, J. Am. Chem. Soc., 2013, 135, 12204–12207 CrossRef CAS PubMed;
(e) S.-C. Chuang, P. Gandeepan and C.-H. Cheng, Org. Lett., 2013, 15, 5750–5753 CrossRef CAS PubMed;
(f) N. J. Webb, S. P. Marsden and S. A. Raw, Org. Lett., 2014, 16, 4718–4721 CrossRef CAS PubMed;
(g) H. Chu, S. Sun, J.-T. Yu and J. Cheng, Chem. Commun., 2015, 51, 13327–13329 RSC;
(h) S. Zhang, D. Huang, G. Xu, S. Cao, R. Wang, S. Peng and J. Sun, Org. Biomol. Chem., 2015, 13, 7920–7923 RSC;
(i) A. B. Weinstein and J. A. Ellman, Org. Lett., 2016, 18, 3294–3297 CrossRef CAS PubMed;
(j) X. Wu, H. Xiong, S. Sun and J. Cheng, Org. Lett., 2018, 20, 1396–1399 CrossRef CAS PubMed;
(k) R. Nie, R. Lai, S. Lv, Y. Xu, L. Guo, Q. Wang and Y. Wu, Chem. Commun., 2019, 55, 11418–11421 RSC.
- For selected examples of Ru species as catalyst, see:
(a) L. Ackermann and S. Fenner, Org. Lett., 2011, 13, 6548–6551 CrossRef CAS PubMed;
(b) S. Ruiz, F. J. Sayago, C. Cativiela and E. P. Urriolabeitia, J. Mol. Catal. A: Chem., 2017, 426, 407–418 CrossRef CAS.
- For selected examples of Co species as catalyst, see:
(a) B. Sun, T. Yoshino, M. Kanai and S. Matsunaga, Angew. Chem., Int. Ed., 2015, 54, 12968–12972 CrossRef CAS PubMed;
(b) G. Sivakumar, A. Vijeta and M. Jeganmohan, Chem.–Eur. J., 2016, 22, 5899–5903 CrossRef CAS PubMed;
(c) C. Kuai, L. Wang, B. Li, Z. Yang and X. Cui, Org. Lett., 2017, 19, 2102–2105 CrossRef CAS PubMed;
(d) F. Yang, J. Yu, Y. Liu and J. Zhu, Org. Lett., 2017, 19, 2885–2888 CrossRef CAS PubMed;
(e) X.-C. Li, C. Du, H. Zhang, J.-L. Niu and M.-P. Song, Org. Lett., 2019, 21, 2863–2866 CrossRef CAS PubMed.
- For selected examples of Pd species as catalyst, see:
(a) N. Zhang, B. Li, H. Zhong and J. Huang, Org. Biomol. Chem., 2012, 10, 9429–9439 RSC;
(b) Z.-W. Zhang, A. Lin and J. Yang, J. Org. Chem., 2014, 79, 7041–7050 CrossRef CAS PubMed;
(c) B. Nie, W. Wu, W. Zeng, Q. Ren, J. Zhang, Y. Zhang and H. Jiang, Adv. Synth. Catal., 2020, 362, 1362–1369 CrossRef CAS.
- For selected reviews of sulfoxonium ylides, see:
(a) J. D. Neuhaus, R. Oost, J. Merad and N. Maulide, Top. Curr. Chem., 2018, 376, 15–61 CrossRef PubMed;
(b) J. Vaitla, A. Bayer and K. H. Hopmann, Synlett, 2019, 30, 1377–1383 CrossRef CAS;
(c) X. Wu, S. Sun, J.-T. Yu and J. Cheng, Synlett, 2019, 30, 21–29 CrossRef CAS;
(d) J. Vaitla and A. Bayer, Synthesis, 2019, 51, 612–628 CrossRef CAS.
- For selected examples of sulfoxonium ylides, see:
(a) M. Barday, C. Janot, N. R. Halcovitch, J. Muir and C. Aissa, Angew. Chem., Int. Ed., 2017, 56, 13117–13121 CrossRef CAS PubMed;
(b) Y. Xu, X. Zhou, G. Zheng and X. Li, Org. Lett., 2017, 19, 5256–5259 CrossRef CAS PubMed;
(c) X. Wu, H. Xiong, S. Sun and J. Cheng, Org. Lett., 2018, 20, 1396–1399 CrossRef CAS PubMed;
(d) Y. Xu, G. Zheng, X. Yang and X. Li, Chem. Commun., 2018, 54, 670–673 RSC;
(e) C. You, C. Pi, Y. Wu and X. Cui, Adv. Synth. Catal., 2018, 360, 4068–4072 CrossRef CAS;
(f) R. Nie, R. Lai, S. Lv, Y. Xu, L. Guo, Q. Wang and Y. Wu, Chem. Commun., 2019, 55, 11418–11421 RSC;
(g) G.-D. Xu, K. L. Huang and Z.-Z. Huang, Adv. Synth. Catal., 2019, 361, 3318–3323 CrossRef CAS;
(h) J. Yu, S. Wen, D. Ba, W. Lv, Y. Chen and G. Cheng, Org. Lett., 2019, 21, 6366–6369 CrossRef CAS PubMed;
(i) Y. Huang, X. Lyu, H. Song and Q. Wang, Adv. Synth. Catal., 2019, 361, 5272–5276 CrossRef CAS;
(j) N. Lv, Z. Chen, Z. Liu and Y. Zhang, J. Org. Chem., 2019, 84, 13013–13021 CrossRef CAS PubMed;
(k) Z. Shen, C. Pi, X. Cui and Y. Wu, Chin. Chem. Lett., 2019, 30, 1374–1378 CrossRef CAS;
(l) J. Zhang, X. Wang, D. Chen, Y. Kang, Y. Ma and M. Szostak, J. Org. Chem., 2020, 85, 3192–3201 CrossRef CAS PubMed;
(m) Y. Wu, C. Pi, X. Cui and Y. Wu, Org. Lett., 2020, 22, 361–364 CrossRef CAS PubMed;
(n) C. N. Kona, Y. Nishii and M. Miura, Org. Lett., 2020, 22, 4806–4811 CrossRef CAS PubMed;
(o) Q.-W. Gui, F. Teng, Z.-C. Li, X.-F. Jin, M. Zhang, J.-N. Dai, Y.-W. Lin, Z. Cao and W.-M. He, Org. Chem. Front., 2020, 7, 4026–4030 RSC.
- G. Zheng, M. Tian, Y. Xu, X. Chen and X. Li, Org. Chem. Front., 2018, 5, 998–1002 RSC.
- X. Shi, R. Wang, X. Zeng, Y. Zhang, H. Hu, C. Xie and M. Wang, Adv. Synth. Catal., 2018, 360, 4049–4053 CrossRef CAS.
- R. Nie, R. Lai, S. Lv, Y. Xu, L. Guo, Q. Wang and Y. Wu, Chem. Commun., 2019, 55, 11418–11421 RSC.
- For selected examples, see:
(a) R. Matsubara and S. Kobayashi, Acc. Chem. Res., 2008, 41, 292–301 CrossRef CAS PubMed;
(b) J.-H. Xie, S.-F. Zhu and Q.-L. Zhou, Chem. Rev., 2011, 111, 1713–1760 CrossRef CAS PubMed;
(c) K. Gopalaiah and H. B. Kagan, Chem. Rev., 2011, 111, 4599–4657 CrossRef CAS PubMed;
(d) M.-N. Zhao, Z.-H. Ren, Y.-Y. Wang and Z.-H. Guan, Chem. Commun., 2012, 48, 8105–8107 RSC;
(e) G. Liu, X. Liu, Z. Cai, G. Jiao, G. Xu and W. Tang, Angew. Chem., Int. Ed., 2013, 52, 4235–4238 CrossRef CAS PubMed;
(f) N. Hu, G. Zhao, Y. Zhang, X. Liu, G. Li and W. Tang, J. Am. Chem. Soc., 2015, 137, 6746–6749 CrossRef CAS PubMed;
(g) T. Ju, Q. Fu, J.-H. Ye, Z. Zhang, L.-L. Liao, S.-S. Yan, X.-Y. Tian, S.-P. Luo, J. Li and D.-G. Yu, Angew. Chem., Int. Ed., 2018, 57, 13897–13901 CrossRef CAS PubMed.
- For selected examples, see:
(a) H. Zhou, Y.-H. Xu, W.-J. Chung and T.-P. Loh, Angew. Chem., 2009, 121, 5459–5461 CrossRef;
(b) S. Rakshit, F. W. Patureau and F. Glorius, J. Am. Chem. Soc., 2010, 132, 9585–9587 CrossRef CAS PubMed;
(c) D. R. Stuart, P. Alsabeh, M. Kuhn and K. Fagnou, J. Am. Chem. Soc., 2010, 132, 18326–18339 CrossRef CAS PubMed;
(d) M. P. Huestis, L. Chan, D. R. Stuart and K. Fagnou, Angew. Chem., Int. Ed., 2011, 50, 1338–1341 CrossRef CAS PubMed;
(e) K. D. Hesp, R. G. Bergman and J. A. Ellman, J. Am. Chem. Soc., 2011, 133, 11430–11433 CrossRef CAS PubMed;
(f) M. Chen, Z.-H. Ren, Y.-Y. Wang and Z.-H. Guan, Angew. Chem., Int. Ed., 2013, 52, 14196–14199 CrossRef CAS PubMed;
(g) B. Li, N. Wang, Y. Liang, S. Xu and B. Wang, Org. Lett., 2013, 15, 136–139 CrossRef CAS PubMed;
(h) M.-N. Zhao, Z.-H. Ren, Y.-Y. Wang and Z.-H. Guan, Org. Lett., 2014, 16, 608–611 CrossRef CAS PubMed;
(i) M.-N. Zhao, L. Yu, R.-R. Hui, Z.-H. Ren, Y.-Y. Wang and Z.-H. Guan, ACS Catal., 2016, 6, 3473–3477 CrossRef CAS;
(j) P. Shi, S. Li, L.-M. Hu, C. Wang, T.-P. Loh and X.-H. Hu, Chem. Commun., 2019, 55, 11115–11118 RSC.
-
(a) W. Yu, J. Chen, K. Gao, Z. Liu and Y. Zhang, Org. Lett., 2014, 16, 4870–4873 CrossRef CAS PubMed;
(b) W. Yu, W. Zhang, Y. Liu, Y. Zhou, Z. Liu and Y. Zhang, RSC Adv., 2016, 6, 24768–24772 RSC;
(c) W. Yu, W. Zhang, Y. Liu, Z. Liu and Y. Zhang, Org. Chem. Front., 2017, 4, 77–80 RSC;
(d) W. Zhang, W. Yu, Q. Yan, Z. Liu and Y. Zhang, Org. Chem. Front., 2017, 4, 2428–2432 RSC.
Footnote |
† Electronic supplementary information (ESI) available. See DOI: 10.1039/d1ra01063h |
|
This journal is © The Royal Society of Chemistry 2021 |