DOI:
10.1039/D1RA00755F
(Paper)
RSC Adv., 2021,
11, 14306-14313
Insights into the structure of sunscreen lotions: a small-angle neutron scattering study
Received
28th January 2021
, Accepted 9th April 2021
First published on 16th April 2021
Abstract
Sunscreen lotions and creams are arguably the most popular products used to protect the skin against harmful UV radiation. Several studies have been conducted to untangle the internal microstructure of creams and lotions. However, the effect of UV filters and other materials such as preservatives, on the internal microstructure and the aesthetics of these products is not yet fully understood. Using small-angle neutron scattering (SANS), we were able to investigate the effect of adding the commonly used organic UV filters (avobenzone (AVB), ethylhexyl methoxycinnamate (EMC), ethylhexyl triazone (EHT) and bemotrizinol (BMT)) and the water soluble preservatives (1,5-pentanediol (1,5-PD) and 1,2-hexanediol (1,2-HD)), on the internal architecture and microstructure of an oil-in-water (o/w) based sunscreen lotion. Our findings highlight the complexities of these formulations, and how the introduction of different additives could influence their structure and possibly their performance.
1. Introduction
Sun protection creams and lotions are of importance to many individuals due their protection of the human skin against UV radiation. UV radiation, namely UVA and UVB, is responsible for several skin conditions such as erythema (sun burns), photoaging, and skin cancers such as basal cell and squamous cell cancers.1–3 These formulations often contain inorganic and/or organic active ingredients known as UV filters or “sunscreens” to scatter/absorb or attenuate UV radiation.4–6 To achieve the optimum protection against UV radiation, sun protection formulations usually comprise more than one organic UVA and UVB filter. Typical examples of these UV filters are avobenzone, AVB, and homosalate for UVA protection, UVB filters as ethylhexyl methoxycinnamate, EMC, ethylhexyl triazone, EHT, and UVA/UVB filters as bemotrizinol, BMT. A judicious choice of these UV filters is often combined with inorganic UV filters such as zinc oxide (ZnO) and titanium dioxide (TiO2).
In addition to active ingredients, sun protection creams and lotions would also contain ionic, non-ionic or zwitterionic surfactants to stabilise the constituent oil and water phases, in combination with co-surfactant/s to enhance the overall stability and consistency of the formulation. Other ingredients commonly used include preservatives, photo-stabilisers and fragrances. The number of ingredients present in these products suggests the presence of what could be described as a complex multicomponent colloidal system. Despite their availability and wide range, our understanding of these products' microstructure is unfortunately lacking, leading to a formulation approach based on ‘trial and error’.
Junginger et al.7,8 were the first to investigate the microstructure of oil-in-water (o/w) creams. Through a combination of differential scanning calorimetry, X-ray diffraction and transmission electron microscopy measurements, their analysis revealed the complexity of the microstructure and was modelled by five domains: mixed bilayers of surfactants and co-surfactants, interlamellar fixed water layers, bilayers of consistency enhancer, bulk water phase and the oil phase.
Aqueous cream BP was investigated by Teo et al.9 using confocal laser scanning microscopy, where liquid crystalline lamellar regions presenting as long defined needles were observed. These regions changed shape and structure as a function of the water content in the cream, for example, after 50% of the water content was lost by evaporation, the lamellar phases disappeared and the oil in the cream coalesced and formed a homogenous phase.
More recently, Kónya et al.10,11 studied o/w creams comprising isopropyl myristate, cetostearyl alcohol and the non-ionic surfactant glycerol stearate using rheology, X-ray diffraction and microscopy, and they concluded that co-existing surfactant micelles and co-surfactant bilayers formed the body of the cream, on the contrary to the typical literature understanding of the microstructure. The co-existence of different structures in creams stabilised by polyglycerol citrate/stearate and sodium dodecylsulfate was also explored where the presence of multilamellar vesicles12 and bicelles13 were reported.
As highlighted above, several studies have investigated the microstructure of creams, and reported different results as the choice of the emulsifier changed from one cream to another. Indirect effects of active ingredients such as UV filters,14 vitamin E15 and corticosteroids16,17 on creams' aesthetics because of the change in the microstructure have also been investigated. However, far fewer studies have studied the direct effect of active ingredients such as UV filters or preservatives on the microstructure of cream. Semenzato et al.18 studied the effect of the inorganic UV filters ZnO and TiO2 on the internal structure of o/w emulsions using rheology and microscopy, and they observed a strong interaction between the TiO2 particles and the emulsion, leading to a change in the emulsion structure evident by the syneresis of the oil phase, which rendered the formulation unstable.
Preservatives are almost usually present in different topical products, including sun protection products, to protect the formulations against microbial contamination that could arise due to the presence of the high water content. The effect of the water soluble preservative and humectant 1,5-pentanediol on the structure of aqueous creams stabilised by SDS and distearoylphosphatidylcholine (DSPC) were reported by Ahmadi et al.13,19 using small-angle neutron scattering (SANS) and they have shown a significant interaction between the SDS stabilised cream's lamellar phases and the 1,5-pentanediol, compromising the lamellar bodies and the creams' microstructure, but not for the DSPC, indicating that the choice of surfactant affect the performance of these materials; a result that needs further investigation.
Neutron techniques have proven their ability in probing structures and interfaces at a molecular level, providing many insights into various pharmaceutical and cosmetic formulations such as gels,20 creams,12,13 and foams.21,22 Against this background, and to the best of our knowledge, we present, for the first time, a SANS study investigating the microstructure of sunscreen lotions prepared with organic UV filters and preservatives at ‘real-life’ concentrations. Simple lotions comprising widely used ingredients such as liquid paraffin, isopropyl myristate (IPM), cetyl alcohol as a co-surfactant, glycerol monostearate (GMS) as an emulsifier, glycerol as a humectant and water, were first investigated. Lotions comprising a range of preservatives (1,5-pentanediol, 1,2-hexanediol) and organic UVA and UVB filters (EMC, AVB, EHT and BMT) at 7.0, 2.0, 2.0 and 5.0 w/w% respectively, and their mixtures (SPF 30,23 assuming the minimum suggested application amount of 2 mg cm−2) were also investigated. These findings are expected to be of interest and relevance to the personal care and the pharmaceutical products industries.
2. Materials and methods
2.1 Materials
Glycerol monostearate (95% purity), cetyl alcohol (96% purity), isopropyl myristate (98% purity), glycerol (99% purity), 1,5-pentanediol (98% purity) and liquid paraffin were all purchased from Fisher Scientific UK and used as received. 1,2-Hexanediol was a kind gift from Symrise and was used as received. 2-Ethylhexyl 4-methoxycinnamate (98% purity) and deuterium oxide (D2O, 99.9% purity) were purchased from Sigma Aldrich UK and were used received. 2,4-Bis[4-(2-ethylhexyloxy)-2-hydroxyphenyl]-6-(4-methoxyphenyl)-1,3,5-triazine (bemotrizinol, 98% purity) and 1-(4-methoxyphenyl)-3-(4-tert-butylphenyl)-1,3-propanedione (avobenzone, 98% purity), 2,4,6-trianilino(p-carbo-2-ethylhexyl-1-oxy)-1,3,5-triazine (ethylhexyl triazone, 96% purity) were kind gifts from BASF and were used as received.
2.2. Methods
2.2.1 Sample preparation. The lotions, Table 1, were prepared by the standard hot/hot process, where the aqueous and the oily phase were heated separately to 80 °C. The oily phase was then transferred to the aqueous phase and homogenised for 2 minutes at 10
000 rpm using an IKA T25 digital ultra-turrax disperser. The lotions were then allowed to cool to 30 °C under gentle constant stirring at 100 rpm using an IKA Eurostar 60 overhead stirrer.
Table 1 Chemical composition of the lotions prepared in the presence and absence of 1,5-pentanediol (1,5-PD), 1,2-hexanediol (1,2-HD), and a range of UV filters; avobenzone (AVB), ethylhexyl methoxycinnamate (EMC), ethylhexyl triazone (EHT) and bemotrizinol (BMT) in D2O
|
Liq. paraf. |
GMS |
IPM |
Glycerol |
Cetyl alcohol |
1,5-PD |
1,2-HD |
AVB |
EMC |
EHT |
BMT |
D2O |
w/w% |
L1 |
5.0 |
5.0 |
1.5 |
3.0 |
2.0 |
— |
— |
— |
— |
— |
— |
83.5 |
L2 |
5.0 |
5.0 |
1.5 |
3.0 |
2.0 |
2.5 |
— |
— |
— |
— |
— |
81.0 |
L3 |
5.0 |
5.0 |
1.5 |
3.0 |
2.0 |
— |
2.5 |
— |
— |
— |
— |
81.0 |
L4 |
5.0 |
5.0 |
1.5 |
3.0 |
2.0 |
— |
— |
2.0 |
— |
— |
— |
81.5 |
L5 |
5.0 |
5.0 |
1.5 |
3.0 |
2.0 |
— |
— |
— |
7.0 |
— |
— |
76.5 |
L6 |
5.0 |
5.0 |
1.5 |
3.0 |
2.0 |
— |
— |
— |
— |
2.0 |
— |
81.5 |
L7 |
5.0 |
5.0 |
1.5 |
3.0 |
2.0 |
— |
— |
— |
— |
— |
5.0 |
78.5 |
L8 |
5.0 |
5.0 |
1.5 |
3.0 |
2.0 |
— |
— |
2.0 |
7.0 |
2.0 |
5.0 |
67.5 |
L9 |
5.0 |
5.0 |
1.5 |
3.0 |
2.0 |
2.5 |
— |
2.0 |
7.0 |
2.0 |
5.0 |
65.0 |
L10 |
5.0 |
5.0 |
1.5 |
3.0 |
2.0 |
— |
2.5 |
2.0 |
7.0 |
2.0 |
5.0 |
65.0 |
2.2.2 Small-angle neutron scattering (SANS). SANS measurements were performed at 25 °C (±0.5 °C) on the fixed-geometry, time of flight ZOOM diffractometer (ISIS Spallation Neutron Source, Oxfordshire, UK). The Q-range explored on Zoom was between 0.0025–0.5 Å−1. The samples were contained in 1 mm path length, UV-spectrophotometer grade, quartz demountable cuvettes (Starna). The samples were allowed to equilibrate for at least 5 minutes before being transferred to the beamline. The cuvettes were mounted in aluminium holders on top of an enclosed, computer-controlled, sample chamber. Temperature control was achieved using a thermostatted circulating bath pumping fluid through the base of the sample chamber.Experimental measuring times were approximately 30 min. All scattering data were normalised for the sample transmission and the incident wavelength distribution, corrected for instrumental and sample backgrounds using a quartz cell filled with D2O (this also removes the incoherent instrumental background arising from vacuum windows, etc.), and corrected for the linearity and efficiency of the detector response using the instrument specific software package. The data were put onto an absolute scale using a well characterized partially deuterated polystyrene blend standard sample. The scattering pattern from all the measured SANS profiles was checked and was found to be the same for all incident neutrons wavelength and no multiple scattering from the samples were observed.
SANS data for the simple lotion (L1) without any preservatives or UV filters were fitted using a model accounting for the presence of a large ellipsoidal24 oil droplet and a lamellar phase25 on SASview.26 Data from lotions with preservatives and/or UV filters were all fitted to the multilayer model27,28 using FISH,29 accounting for the oil droplets with an ellipsoidal oil globule ≈ Q−3.5 and multilayers accounting for the lamellae present in the lotions. This model has been used successfully before in capturing meaningful insights from multilayers in foams22,30 and creams.13
The model captures the multilayers (treated as a paracrystal to account for repeating spacing) of M surfactant/cosurfactant/water bilayers, of thickness L, separation D and a Gaussian distribution term, σD/D. To this, a Qn term is added to account for the scattering from the large oil droplets present in the lotion. Typical starting values for the heterogeneity of L and D are σ(L)/L and σ(D)/D = 0.2, though these values have shown to have a negligible effect on the overall quality of the fit within reasonable bounds.
3. Results and discussion
3.1 SANS from lotions without any additives
The SANS data from the lotion (L1) prepared without any UV filters or preservatives, (5.0 w/w% liquid paraffin, 5.0 w/w% GMS, 1.5 w/w% IPM, 3.0 w/w% glycerol and 2.0 w/w% cetyl alcohol), Fig. 1, shows two main features; (i) a strong scattering intensity at low Q values from the large oil droplets, following a ≈ Q−3.5 dependence, suggesting that the oil droplets are of an ellipsoidal or a globular shape, (ii) a peak at Q ≈ 0.15 Å−1 corresponding to a d-spacing (d = 2π/Q) value of 42 Å. The shape and the width of the peak, in addition to the unlikelihood of the presence of an inner leaflet in the oil droplet, suggests that it is most likely from a lamellar structure present in the system, rather than a corona of surfactant and co-surfactant on top of the oil droplet. Furthermore, findings from previous work in the literature8,12,13 further support this observation. This has been explored and verified by data fitting, and a model of an ellipsoidal oil droplet with an equatorial radius of 1500 Å (±10 Å) and a polar radius of 300 Å (±5 Å), co-existing with a lamellar structure with one bilayer thickness of 52 Å ± 1 Å, seems to fit the data very well, capturing most of the features highlighted above. The lamellar thickness obtained from the data fitting (52 Å) could be attributed to a lamellar structure comprising cetyl alcohol (21.7 Å)13 and glycerol monostearate (16 Å),31 where some liquid paraffin and IPM incorporated or solubilised within the lamellae could account for the 8 Å increase in the theoretical thickness (44 Å). This difference between the d-spacing value from the SANS profile and the bilayer thickness value from the fitting routine was further explored using the ellipsoid and lamellar model invoked here. If one is to constrain the bilayer thickness to the theoretical 42 Å value, the fit does not capture either the correct peak position in the SANS profile, nor does it capture the overall features observed at low and mid Q, suggesting that the bilayer (and eventually the fit) is sensitive to other factors such as the presence of the oil, volume, polydispersity, etc.
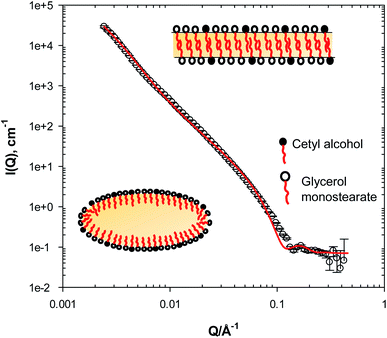 |
| Fig. 1 SANS from lotion (L1) in D2O. Inset is a schematic of the structures (not to scale) captured by the model fitting as described in Section 2.2.2. Solid line is a fit to the ellipsoidal (aspect ratio of 0.2) + lamellar model described in the text. | |
3.2 SANS from lotions with preservatives
The addition of the water-soluble preservatives 1,5-pentanediol (1,5-PD) and 1,2-hexanediol (1,2-HD) has a profound effect on the microstructure of the lotion, with significant changes in the SANS profile observed, Fig. 2. This change is surprising and unexpected, given the current understanding that these materials do not partition into hydrophobic phases due to their high water solubility. The SANS data from the lotion prepared with 2.5 w/w% 1,5-PD (L2) reveals the presence of more defined lamellar structures evident by Bragg peaks-albeit weaker than traditionally observed for creams-at Q ≈ 0.012 and ≈0.024 Å−1 corresponding to d-spacing values of ≈520 and 260 Å. The same observations could be extended to the lotion prepared with 2.5 w/w% 1,2-HD, L3, where the peaks were observed at Q ≈ 0.011 and 0.022 Å−1 corresponding to d-spacing values of ≈570 and 285 Å. The presence of these weaker Bragg peaks could be attributed to the low surfactant concentration in these lotions resulting in a degree of heterogeneity of the lamellae and/or a lower number of layers present as a result of the surfactant concentration. For example, creams formulated with 10 w/w% surfactant (SDS) concentration by Ahmadi et al.13 showed well defined Bragg peaks, in comparison to less defined ones at 2.5 and 4 w/w% surfactant concentration. Data fitting to the multilayer model, Table 2, has also revealed that two structurally similar bilayers are present in these systems. One would expect a stronger scattering behaviour and stronger Bragg diffraction peaks at a higher surfactant concentration. However, it should be noted that the presence of additives and/or higher surfactant concentration could introduce a phase transition, where the lamellar structures in the lotion would be replaced by other structures. Based on the features observed in our SANS data and upon peaks assignment, we can conclude that lamellar structures are indeed present in the lotions of interest here.
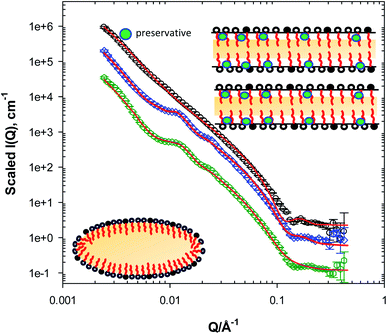 |
| Fig. 2 SANS from L1 (circles), L2 (lotion with prepared with 2.5 w/w% 1,5-PD, diamonds), L3 (lotion prepared with 2.5 w/w% 1,2-HD, hexagons) in D2O. Inset is a schematic of the structures (not to scale) captured by the model fitting as described in Section 2.2.2. Solid lines are fits to the multilayer model described in the text. Data have been offset for clarity. | |
Table 2 Fit (multilayer model) parameters to the scattering data from lotions with 2.5 w/w% 1,5-PD (L2) and 2.5 w/w% 1,2-HD (L3). L: surfactant/cosurfactant layer thickness, M: number of layers and D: interlamellar spacing (spacing between the layers)
System description |
L (±1 Å) |
M |
D (±2 Å) |
L2 |
50 |
2 |
493 |
L3 |
56 |
2 |
460 |
Ahmadi et al.13 have recently explored the effect of 1,5-PD on SDS/cetyl alcohol/stearyl alcohol stabilised pharmaceutical creams using SANS, and it was concluded that the 1,5-PD affected the overall structure of the cream, rendering several of the features reported from the original pure cream weaker, where as a result, they postulated that 1,5-PD interacted with the lamellar phases within the cream, and resulted in a compromised structure. In this work, we can also postulate that both 1,5-PD and 1,2-HD interact with the lamellar phases within the GMS/cetyl alcohol stabilised lotion, however, in our case, it seems that this interaction has promoted the presence and the formation of the lamellar structures within the lotion. This is very interesting as it highlights that the structure and texture of topical formulations changes as the choice of emulsifiers varies in the presence of similar active ingredients or additives.
These observations could be again reiterated from the data fitting, Table 2, where an overall increase in the thickness of the lamellae was observed in comparison with the lotions prepared without any preservative. We could also observe an interlamellar spacing of 490 Å in the presence of 1,5-PD. The presence of 1,2-HD has in a further increase in the lamellar thickness and a decrease in the spacing (D) values obtained from the fitting, further confirming the association of the preservatives with the lamellae. The decrease in the spacing value (30 Å) in the lotion prepared with 1,2-HD is however more substantial than the increase in the lamellae thickness, suggesting that this is not a simple association, and that the presence of 1,2-HD has further implications on the interlamellar water and warrants further investigation. This complex association of the preservatives with the lamellae could be further highlighted by the differences observed between the d-spacing (520 Å and 570 Å) and the fitting routine spacing (D) values (493 and 460 Å), for the lotions prepared with 1,5-PD and 1,2-HD respectively. If we are to constrain the spacing (D) value to the respective d-spacing value from the SANS data in the fitting routine, we are unable to reproduce the same peak position observed in the SANS profile. This strongly suggests that the peaks' position in this case is sensitive to other features observed in the data, namely the intensity of the decaying function at low Q.
3.3 SANS from lotions with organic UV filters
The presence of the organic UV filters has variable but significant effects on the lotions' microstructure as demonstrated by the SANS data in Fig. 3. For example, the addition of 7 w/w% EMC (L5) has highlighted diffraction peaks at Q ≈ 0.013 and a weaker one at 0.026 Å−1, indicating the presence of lamellar structures. However, the peaks' shapes and definition are weaker than that of the system comprising 1,5-PD and 1,2-HD, which could point to the presence of a further disrupted structure and/or structures with a lower volume fraction. Considering the partitioning coefficient value (log
P) for EMC; 6.1,32 we can assume an increased affinity of EMC to the oil and not the hydrophobic chains present within the lamellae. However, the presence of disrupted lamellar bodies strong suggests that EMC has surprisingly been solubilised within the hydrophobic chains. This can also be confirmed with data modelling, Table 3, where the lamellae thickness was found to be ≈63 Å, a significant increase from the lotions prepared without any additives (52 Å). We must however note that even though the multilayer model deployed in this work captures most of the features in the SANS data, it was not capable of capturing the peak at 0.15 Å−1 for the lotions prepared with 7 w/w% EMC. This peak could possibly be attributed to another structure present in this particular lotion and the model could not capture it.
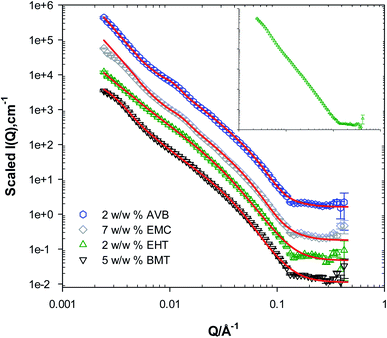 |
| Fig. 3 SANS from lotions prepared with different UV filters in D2O. Hexagons: L4 (2 w/w% avobenzone, AVB). Diamonds: L5 (7 w/w% ethylhexyl methoxycinnamate, EMC). Triangles up: L6 (2 w/w% ethylhexyl triazone, EHT). Triangles down: L7 (5 w/w% bemotrizinol, BMT). Solid lines are fits to the multilayer model described in the text. Data have been offset for clarity. Inset shows SANS data from the lotion (L8) prepared with all the UV filters mentioned above. | |
Table 3 Fit (multilayer model) parameters to the SANS data from lotions with 2 w/w% AVB (L4), 7 w/w% EMC (L5), 2 w/w% EHT (L6) and 5 w/w% BMT (L7). Radius A: polar radius, radius B: equatorial radius, L: surfactant/cosurfactant layer thickness, M: number of layers and D: spacing between the layers
System description |
Radius A (±2 Å) |
Radius B (±5 Å) |
L (±1 Å) |
M |
D (±2 Å) |
L1 |
300 |
1500 |
52 |
1 |
— |
L4 |
— |
— |
60 |
2 |
490 |
L5 |
— |
— |
63 |
2 |
440 |
L6 |
— |
— |
95 |
1 |
— |
L7 |
500 |
625 |
58 |
1 |
— |
If one is to compare the effect of adding 1,5-PD, 1,2-HD and EMC on the structural parameters obtained from the data fitting, the effect of the preservatives on the spacing values (D) in particular is quite significant if compared with EMC, 440 Å. For example, in the presence of 1,5-PD or 1,2-HD, the D values were found to be 493 and 460 Å, respectively. This further proves that active ingredients will behave differently when present in creams or lotions' as their log
P values changes, rather than a simple model where it is assumed that all relatively hydrophobic materials are solubilised in the constituent oil droplets.
Similar observations could be drawn from the SANS data from lotions (L4) prepared with 2 w/w% AVB, Fig. 3. Weak diffraction peaks were observed at similar Q values as with 7 w/w% EMC, with a D value of 440 Å and L of 61 Å. In the presence of 2 w/w% EHT, L6, significant changes in the SANS pattern could be observed such as the absence of any diffraction peaks, suggesting the absence of defined lamellar structures in the lotions, at least at the length scale observed by the neutrons in these experiments. The data modelling in this instance suggests that one layer is present with a thickness of approximately 95 Å. These findings strongly indicate that the presence of EHT has a significant effect on the lotion microstructure, partitioning into the lamellar layer, evident by the absence of any diffraction peak and the overall features of the data. These changes could be attributed to both the molecular size of the EHT molecule and its relatively high log
P value, >7.0.3 For the lotions prepared with BMT, L7, the data fitting using the multilayer model used with the other systems with additives present was not successful, but the data from this system fitted very well to the ellipsoidal and lamellar model employed for the blank lotions, Fig. 3. This points towards a similarity in the structure of both lotions, suggesting that the lamellar structures are not as present in the lotions as with the other UV filters, at least at the neutrons' length scale. BMT has a high log
P value, 12.6,3,33 indicative of preferable solubilisation in the more hydrophobic environment of the oil droplets rather than the lamellae.
The addition of all UV filters (SPF30) to the lotion (L8), inset in Fig. 3, has resulted in a loss of all features observed at mid and high Q. The data shows a strong Q−4 dependence suggesting the presence of a large-spherical- oil droplets. The lack of any features in the data also strongly indicate the absence of lamellar structures at the observed length scale. Given the overall observations, one can also conclude that as the number of UV filters in the sample increases, the oil droplets would solubilise most of the filters due to its high hydrophobicity resulting in the observed SANS data.
3.4 SANS from lotions with organic UV filters and preservatives
The realistic approach of this study was to look at the lotions prepared with mixtures of UV filters and preservatives, mimicking the complex composition of products available to consumers seeking sun protection. As mentioned earlier, the addition of the four UV filters of interest to this study to the lotions, has rendered the lotions' SANS profile featureless apart from a strong Q−4 dependence. The introduction of the preservatives 1,5-PD (L9) and 1,2-HD (L10) to this system has reproduced peaks at mid Q with varying intensities where lotions prepared with 1,2-HD showed the most defined peak, Fig. 4. The data from both lotions containing UV filters and preservatives also show a shift in the peaks position towards high Q if compared to the ones without UV filters but with the preservatives, indicative of larger spacing between the bilayers. This is consistent with our data modelling, Table 4, with spacing values (D) of ≈425 and 400 Å for the lotions (with UV filters) prepared with 1,5-PD and 1,2-HD, respectively. An increase in the lamellar thickness of lotions prepared with the UV filters and 1,2-HD could also be observed, 80 Å. This suggests that the presence of both groups of materials has rendered the lamellae thicker, which could arise from the presence of fractions of the UV filters within the lamellae in addition to the 1,2-HD.
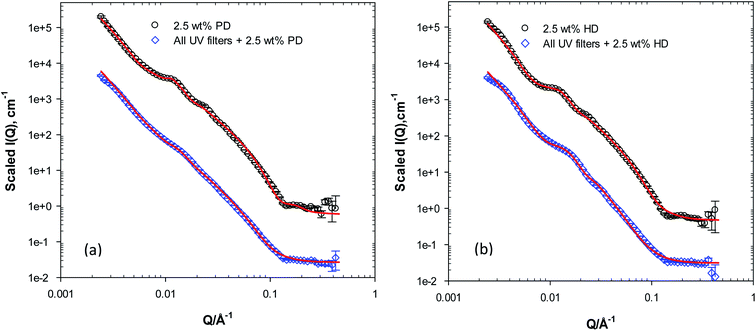 |
| Fig. 4 (a) SANS from lotions prepared with 2.5 w/w% 1,5-PD, L2 (circles) and UV filters + 2.5 w/w% 1,5-PD, L9 (diamonds) in D2O. Solid lines are fits to the multilayer model described in the text. Data have been offset for clarity. (b) SANS from lotions prepared with 2.5 w/w% 1,2-HD, L3 (circles) and UV filters + 2.5 w/w% 1,2-HD, L10 (diamonds) in D2O. Solid lines are fits to the multilayer model described in the text. Data have been offset for clarity. | |
Table 4 Fit (multilayer model) parameters to the SANS data from lotions with 2.5 w/w% 1,5-PD (L2), 2.5 w/w% 1,2-HD (L3), the four UV filters described in the text and 2.5 w/w% 1,5-PD (L9) and the four UV filters + 2.5 w/w% 1,2-HD (L10). L: surfactant/cosurfactant layer thickness, M: number of layers and D: spacing between the layers
System description |
L (±1 Å) |
M |
D (±2 Å) |
L2 |
50 |
2 |
493 |
L3 |
56 |
2 |
460 |
L9 |
60 |
3 |
425 |
L10 |
82 |
2 |
400 |
The changes observed in the SANS data from the lotions prepared with the four UV filters and the diols are indeed interesting. The overall scattering profile from these systems suggests that the diols are still associating with the lamellar network present in the lotions, even with the presence of a high concentration (16 w/w%) of the hydrophobic organic UV filters. Again, a surprising behaviour for materials that have been long presumed to overwhelmingly partition in the water phase. This behaviour could be perhaps attributed to the interfacial activity exerted by these two diols. Gliński et al.34 have investigated the interfacial activity of 1,5-PD and reported surface active species that lowered the water surface tension to ≈45 mN m−1 at similar concentrations. At relatively low concentrations, Romero et al.35 have also shown that 1,2-HD possess high surface activity at the air/water interface and that the presence of 1,2-HD has lowered the surface tension of water to ≈32 mN m−1. This high surface activity could explain the association with the lamellar phase, where the preservative would partition at the lamellae oil-water interface, affecting the curvature and eventually the size and the shape of the lamellar bodies.
However, this does not fully explain the significant decrease in the spacing value (D) between the bilayers in the lotions prepared with UV filters and the diols. D'Arrigo et al.36 and Székely et al.37 have used SANS to investigate the behaviour of both diols of interest to this work in water. A relationship was established between the surface activity and their aggregation behaviour, and it was concluded that-at least-for the 1,2-HD, aggregation was observed at concentrations similar to the ones used in this work. From the SANS data, the authors were able to observe the presence of hydrated spherical aggregates with attractive interactions between them. The association of the preservative molecules with an attractive potential at the interface might perhaps explain this decrease in the spacing value, however, further work investigating this association closely and its effect on the spacing and lamellar thickness is needed. Further investigations are also needed to determine whether the type of emulsifier(s) used in preparing lotions and creams containing these diols could influence their antimicrobial efficacy.
4. Conclusions
The microstructure of sunscreen lotions stabilised by glycerol monostearate and cetyl alcohol was investigated using SANS. Generally, the SANS data showed (a) a strong Q dependence that varied from Q−3.5 to Q−4 which could be an indication of the change of the oil droplets shape from ellipsoidal to spherical, (b) a series of peaks at mid and low Q that were found to be sensitive to the presence of different additives. Lotions prepared without any additives showed the presence of ellipsoidal oil droplets, evident by a strong Q−3.5 dependence, coexisting with lamellar phases. The addition of the diol-based preservatives 1,5-PD and 1,2-HD has results in significant changes in the SANS data, where the presence of lamellar bodies have been promoted evident by the presence of stronger Bragg peaks. These findings strongly indicate that these preservatives interact with the lamellar bodies, contrary to the current understanding, altering lotions and creams' texture and feel.
The presence of the four commonly used organic UV filters had variable effects on the microstructure of the lotions, where the findings and data fitting strongly suggest a high degree of partitioning of the UV filters into the lamellar phases in addition to the oil droplets evident by changes in the lamellae's thickness and interlamellar spacing values. Water soluble preservatives as 1,5-PD and 1,2-HD introduce further complexities in the presence of the UV filters and warrants further studies. It is anticipated that the insights from this work will shed some light on the complexities of sunscreen formulations and provide further knowledge on how active ingredients could compromise the integrity of creams and lotions.
To summarise, one can conclude that the presence of different additives in these systems ‘trigger’ different responses. This could present itself as a change in the interactions between the droplets themselves and/or between the additives and the droplets/lamellae. We hope to resolve these responses further with future contrast variation SANS experiments.
Conflicts of interest
The authors declare no conflict of interest.
Acknowledgements
The authors thank the UK Science and Technology Facilities Council (STFC) for allocation of beamtime at ISIS (RB1920043). The data obtained from the SANS experiments described here could be found: DOI: 10.5286/ISIS.E.RB1920043. This work benefited from the use of the SasView software, originally developed by the DANSE project under NSF award DMR-0520547.
References
- C. Souza and P. M. B. G. M. Campos, Development and photoprotective effect of a sunscreen containing the antioxidants Spirulina and dimethylmethoxy chromanol on sun-induced skin damage, Eur. J. Pharm. Sci., 2017, 104, 52–64 CrossRef CAS PubMed.
- S. H. Yoon, J. I. Park, J. E. Lee, C. H. Myung and J. S. Hwang, In vivo Change of Keratin-Bound Molecules in the Human Stratum Corneum following Exposure to Ultraviolet Radiation, Skin Pharmacol. Physiol., 2019, 32, 254–264 CrossRef CAS PubMed.
- S. Tampucci, S. Burgalassi, P. Chetoni and D. Monti, Cutaneous permeation and penetration of sunscreens: formulation strategies and in vitro methods, Cosmetics, 2018, 5(1) DOI:10.3390/cosmetics5010001.
- S. Smaoui, H. Ben Hlima, I. Ben Chobba and A. Kadri, Development and stability studies of sunscreen cream formulations containing three photo-protective filters, Arabian J. Chem., 2017, 10, S1216–S1222 CrossRef CAS.
- N. Serpone, D. Dondi and A. Albini, Inorganic and organic UV filters: their role and efficacy in sunscreens and suncare products, Inorg. Chim. Acta, 2007, 360, 794–802 CrossRef CAS.
- I. D. Da Silva Souza, et al., Efficient UV Filter Solubilizers Prevent Recrystallization Favoring Accurate and Safe Sun Protection, ACS Appl. Mater. Interfaces, 2018, 10, 40411–40423 CrossRef CAS PubMed.
- H. E. Junginger, Colloidal structures of O/W creams, Pharm. Weekbl., Sci. Ed., 1984, 6, 141–149 CrossRef CAS PubMed.
- T. de Vringer, J. G. H. Joosten and H. E. Junginger, A study of the gel structure in a nonionic O/W cream by X-ray diffraction and microscopic methods, Colloid Polym. Sci., 1987, 265, 167–179 CrossRef CAS.
- Y. H. Teo, P. Goddard, D. A. Barrett and C. D. Melia, The microstructure of creams revealed by confocal laser scanning microscopy, J. Pharm. Pharmacol., 1998, 50, 132 CrossRef CAS.
- M. Kónya, et al., Study of the microstructure of oil/water creams with thermal and rheological methods, J. Therm. Anal. Calorim., 2003, 73, 623–632 CrossRef.
- M. Kónya, I. Dékány and I. Erõs, X-ray investigation of the role of the mixed emulsifier in the structure formation in o/w creams, Colloid Polym. Sci., 2007, 285, 657–663 CrossRef.
- V. Dahl, et al., Structural Analysis of a Modern o/w-Emulsion Stabilized by a Polyglycerol Ester Emulsifier and Consistency Enhancers, Colloids Interfaces, 2018, 2, 3 CrossRef CAS.
- D. Ahmadi, et al., Revealing the Hidden Details of Nanostructure in a Pharmaceutical Cream, Sci. Rep., 2020, 10, 1–12 CrossRef PubMed.
- L. S. Calixto, P. M. B. G. Maia Campos, G. Savary and C. Picard, Interactions between UV filters and active substances in emulsion: effect on microstructure, physicochemical and in vivo properties, Int. J. Pharm., 2018, 553, 220–228 CrossRef CAS PubMed.
- L. Montenegro, L. Rapisarda, C. Ministeri and G. Puglisi, Effects of lipids and emulsifiers on the physicochemical and sensory properties of cosmetic emulsions containing vitamin E, Cosmetics, 2015, 2, 35–47 CrossRef CAS.
- C. Nagelreiter, E. Kratochvilova and C. Valenta, Dilution of semi-solid creams: influence of various production parameters on rheological properties and skin penetration, Int. J. Pharm., 2015, 478, 429–438 CrossRef CAS PubMed.
- C. C. Müller-Goymann and U. Alberg, Modified water containing hydrophilic ointment with suspended hydrocortisone-21-acetate – the influence of the microstructure of the cream on the in vitro drug release and in vitro percutaneous penetration, Eur. J. Pharm. Biopharm., 1999, 47, 139–143 CrossRef.
- A. Semenzato, et al., Chemico-physical and functional properties of inorganic sunscreens in cosmetic products, Int. J. Cosmet. Sci., 1994, 16, 247–255 CrossRef CAS PubMed.
- D. Ahmadi, et al., Journal of Colloid and Interface Science Supramolecular architecture of a multi-component biomimetic lipid barrier formulation, J. Colloid Interface Sci., 2020, 587, 597–612 CrossRef PubMed.
- G. E. Newby, E. B. Watkins, D. H. Merino, P. A. Staniec and O. Bikondoa, In situ Rheo-GISANS of triblock copolymers: gelation and shear effects on quasi-crystalline structures at interfaces, RSC Adv., 2015, 5, 104164–104171 RSC.
- I. Schmidt, B. Novales, F. Boué and M. Axelos, Foaming properties of protein/pectin electrostatic complexes and foam structure at nanoscale, J. Colloid Interface Sci., 2010, 345, 316–324 CrossRef CAS PubMed.
- O. T. Mansour, et al., Assembly of small molecule surfactants at highly dynamic air–water interfaces, Soft Matter, 2017, 13, 8807–8815 RSC.
- BASF, Sunscreen simulator, https://www.sunscreensimulator.basf.com/Sunscreen_Simulator/computation Search PubMed.
- L. A. Feigin and D. I. Svergun, Structure Analysis by Small-Angle X-Ray and Neutron Scattering, Plenum Press, 1987 Search PubMed.
- J. Berghausen, J. Zipfel, P. Lindner and W. Richtering, Influence of Water-Soluble Polymers on the Shear-Induced Structure Formation in Lyotropic Lamellar Phases, J. Phys. Chem. B, 2001, 105, 11081–11088 CrossRef CAS.
- SasView, SasView, http://www.sasview.org/version%205.0 Search PubMed.
- M. Shibayama and T. Hashimoto, Small-Angle X-ray Scattering Analyses of Lamellar Microdomains Based on a Model of One-Dimensional Paracrystal with Uniaxial Orientation, Macromolecules, 1986, 19, 740–749 CrossRef CAS.
- M. Kotlarchyk and S. M. Ritzau, Paracrystal model of the high-temperature lamellar phase of a ternary microemulsion system, J. Appl. Crystallogr., 1991, 24, 753–758 CrossRef CAS.
- R. K. Heenan, FISH, https://www.diamond.ac.uk/Instruments/Soft-Condensed-Matter/small-angle/SAXS-Software/CCP13/FISH.html Search PubMed.
- J. Hurcom, et al., The interfacial structure of polymeric surfactant stabilised air-in-water foams, Soft Matter, 2014, 10, 3003–3008 RSC.
- J. L. Bradley-Shaw, P. J. Camp, P. J. Dowding and K. Lewtas, Molecular Dynamics Simulations of Glycerol Monooleate Confined between Mica Surfaces, Langmuir, 2016, 32, 7707–7718 CrossRef CAS PubMed.
- S. Q. Wang, U. Osterwalder and K. Jung, Ex vivo evaluation of radical sun protection factor in popular sunscreens with antioxidants, J. Am. Acad. Dermatol., 2011, 65, 525–530 CrossRef CAS PubMed.
- K. Skotarczak, et al., Photoprotection: facts and controversies, Eur. Rev. Med. Pharmacol. Sci., 2015, 19, 98–112 CAS.
- J. Gliński, G. Chavepeyer and J. K. Platten, Untypical surface properties of aqueous solutions of 1,5-pentanediol, Colloids Surf., A, 2000, 162, 233–238 CrossRef.
- C. M. Romero, M. S. Páez, J. A. Miranda, D. J. Hernández and L. E. Oviedo, Effect of temperature on the surface tension of diluted aqueous solutions of 1,2-hexanediol, 1,5-hexanediol, 1,6-hexanediol and 2,5-hexanediol, Fluid Phase Equilib., 2007, 258, 67–72 CrossRef CAS.
- G. D'Arrigo, R. Giordano and J. Teixeira, Small-angle neutron scattering studies of aqueous solutions of short-chain amphiphiles, Eur. Phys. J. E: Soft Matter Biol. Phys., 2003, 10, 135–142 CrossRef PubMed.
- N. K. Székely, L. Almásy, A. Rǎdulescu and L. Rosta, Small-angle neutron scattering study of aqueous solutions of pentanediol and hexanediol, J. Appl. Crystallogr., 2007, 40, 307–311 CrossRef.
|
This journal is © The Royal Society of Chemistry 2021 |
Click here to see how this site uses Cookies. View our privacy policy here.