DOI:
10.1039/D1RA00696G
(Paper)
RSC Adv., 2021,
11, 12336-12347
iTRAQ-based proteomic and physiological analyses of broccoli sprouts in response to exogenous melatonin with ZnSO4 stress†
Received
26th January 2021
, Accepted 12th March 2021
First published on 29th March 2021
Abstract
Exogenous melatonin (10 μM) enhances ZnSO4 (4 mM) stress tolerance and regulates the isothiocyanate content of broccoli sprouts. Nevertheless, the molecular mechanism underlying the role of melatonin in isothiocyanate metabolism under ZnSO4 stress is unclear. The effects of exogenous melatonin on growth and isothiocyanate metabolism in broccoli sprouts under ZnSO4 stress during germination were investigated by physio-biochemical methods, quantification of relative gene expression levels, and the isobaric tags for the relative and absolute quantitation (iTRAQ) labelling technique. Compared with sprouts under ZnSO4 stress alone, sprout length, fresh weight and free calcium content increased significantly in sprouts under ZnSO4 stress plus melatonin treatment while electrolyte leakage and malonaldehyde content decreased. The glucosinolate content and myrosinase activity also significantly increased in sprouts under ZnSO4 stress plus melatonin treatment compared with the control, and thus the isothiocyanate and sulforaphane content increased markedly. Meanwhile, the expression of glucoraphanin biosynthesis genes, such as MYB28, CYP83A1, AOP2, BoSAT1, and BoHMT1 was significantly induced by melatonin in sprouts under ZnSO4 stress. Furthermore, compared with sprouts under ZnSO4 stress alone, a total of 145 proteins in broccoli sprouts under ZnSO4 stress plus melatonin treatment showed differential relative abundances. These proteins were divided into 13 functional classes and revealed that pathways for sulfur metabolism, glucosinolate biosynthesis, selenocompound metabolism, biosynthesis of secondary metabolites and peroxisome were significantly enriched. The present study indicates that exogenous melatonin alleviates the adverse effects of ZnSO4 stress on sprout growth and promotes glucoraphanin biosynthesis and the hydrolysis of glucoraphanin to form isothiocyanates in broccoli sprouts.
1. Introduction
Broccoli sprouts have been widely consumed as functional foods and have become increasingly popular due to their high content of bioactive compounds, including flavonoids, ascorbic acid, anthocyanins and isothiocyanates (ITCs).1–3 Among these, ITCs have raised scientific interest for their potential to reduce the risk of diseases including cancer,4,5 inflammatory diseases6,7 and cardiovascular diseases.8,9 In a plant cell, ITCs can be metabolised from glucosinolates (GLSs) via myrosinase (MYR, EC 3.2.1.147). The ITC sulforaphane (4-methylsulfinylbutyl isothiocyanate) is the most promising anticancer agent with its unique properties in cancer prevention and treatment.10 For these reasons, there is immense interest in accumulating ITCs in broccoli sprouts. Germination under abiotic stress, especially ZnSO4 stress, is the most common and effective way of enhancing ITC content in broccoli sprouts.11–13 However, the growth and development of sprouts are found to be markedly inhibited under ZnSO4 stress, although the ITC content increases significantly.13
Melatonin (N-acetyl-5-methoxytryptamine) is a bioactive indole derivative that widely exists in plants. Previous research has shown that melatonin, as a master regulator, plays significant roles in modulating plant metabolism and regulating the growth and development of plants.14,15 In multiple plant species, melatonin treatment can reduce the negative effects associated with stressors, and enhance tolerance to biotic and abiotic stress through the scavenging of reactive oxygen species and modulation of stress-related genes.16,17 Moreover, in the secondary metabolism of the plant, melatonin induces the biosynthesis of flavonoids,18,19 anthocyanins20,21 and carotenoids,22 among other molecules.23 Similarly, according to our previous study, exogenous melatonin (10 μM) counters the harmful effects of ZnSO4 stress (4 mM) and enhances sulforaphane content in broccoli sprouts during germination. Studies indicate that exogenous melatonin may be an ideal biotechnological target for improving ITC-enriched broccoli sprouts grown under stressful conditions.24 Nevertheless, the molecular mechanism underlying the role of melatonin in the resistance to ZnSO4 stress is still unclear.
Based on the issues above, in the present study, an isobaric tag for the relative and absolute quantitation (iTRAQ) labelling technique was employed to characterise the proteomic changes in broccoli sprouts under ZnSO4 and ZnSO4 plus melatonin treatment. The results of physiological and biochemical assays, gene expression levels and comparative proteomic analyses help to clarify the mechanisms by which ITC metabolism in broccoli sprouts is affected in response to ZnSO4 plus melatonin treatment.
2. Experimental
2.1. Plant growth and experimental design
Broccoli seeds (Brassica oleracea L. var. Italica) were surface sterilised by soaking in 1% (v/v) sodium hypochlorite for 15 min and then steeped in distilled water at 30 °C for 4 h. The soaked seeds were spread evenly on a transparent square case (8.5 cm × 9 cm × 8 cm) filled with vermiculite and irrigated with distilled water. The cases were transferred to a controlled environment chamber with a 16 h light/8 h dark cycle at an air temperature of 30 °C. After 1 day of germination, treatments were applied as follows: (1) control check (CK, distilled water); (2) ZnSO4 treatment (Zn, 4 mM ZnSO4); (3) melatonin treatment (MT, 10 μM melatonin); (4) ZnSO4 plus melatonin treatment (ZM, 4 mM ZnSO4 + 10 μM melatonin). Broccoli sprouts were randomly sampled on days 4 and 6, and freeze-dried or stored frozen at −20 °C for further biochemical measurements. The concentrations of the solutions used and the germination times were selected based on our earlier experiments.
2.2. Determination of sprout length, fresh weight, malondialdehyde content, and electrolyte leakage
For the determination of the sprout length and fresh weight (FW), 30 sprouts from each treatment group were randomly selected, and their lengths and weights were measured. The content of malondialdehyde (MDA) was measured based on the method of Madhava and Sresty.25 The electrolyte leakage was measured with a conductivity meter (DDS-307, China) according to the method of Dionisio-Sese and Tobita.26
2.3. Measurements of myrosinase activity, glucosinolate content, isothiocyanate content and sulforaphane content
The MYR activity was measured according to Burow et al.27 with minor modifications. Sprouts were grinded in ice bath conditions with 3 mL 0.1 M phosphate buffer (pH 6.5), and centrifuged at 4 °C at 10
000g for 15 min. Supernatant (0.5 mL) was mixed with 0.5 mL sinigrin (0.25 mM). The content of glucose was determined using a Glucose Kit (F006-1-1, Nanjing Jiancheng Biological Engineering Research Institute, China). The MYR activity was expressed as nmol glucose formed per minute and mg total protein. The total GLS content was determined according to Guo et al.28 The content of ITCs was determined according to the method of Jiao et al.29 The content of sulforaphane was determined according to Guo et al.30
2.4. Determination of total antioxidant capacity and peroxidase activity
The total antioxidant capacity (T-AOC) and peroxidase (POD) activity were determined using a Plant T-AOC Assay Kit (A015-1-2, Nanjing Jiancheng Bioengineering Research Institute, China) and a Plant POD Assay Kit (A084-3, Nanjing Jiancheng Bioengineering Institute, China), respectively.
2.5. Determination of intracellular free calcium
The intracellular free calcium was measured according to the method of Cheng et al.31
2.6. RNA extraction and quantitative real-time PCR analysis
Total RNA was isolated from broccoli sprouts using an E.Z.N.A.™ Plant RNA Kit (R6827-01, OMEGA, USA) as described in the manufacturer's instructions. The RNA samples were reverse transcribed into cDNA by a PrimeScript™ RT Master Mix Kit (RR036A, Takara, Japan). Triplicate quantitative assays were performed on each cDNA using SYBRR Premix Ex-Taq™ (RR420A, Takara, Japan) and the ABI 7500 sequence detection system (Applied Biosystems, Calif., USA) according to the manufacturer's protocol. The sequence-specific primers used in the present study are listed in ESI Table S1.†
2.7. Protein extraction, digestion, and iTRAQ labelling
The total protein in 4 day-old broccoli sprouts was extracted using a Plant Total Protein Extraction Kit (PE0230, Sigma, USA). The protein concentration was determined using a Pierce™ Coomassie Protein Assay Kit (23200, Thermo Scientific, USA) using bovine serum albumin as the standard. The sample was reduced, alkylated, and then submitted to digestion with trypsin according to the method developed by Cheng et al.31 Afterwards, each sample was labelled separately using the iTRAQ 8-Plex Kit (4381662, Sigma-Aldrich, USA) according to the manufacturer's instructions. Finally, all samples were combined and lyophilised.
2.8. LC-MS/MS and data analysis
The labelled samples were fractionated using a Thermo UHPLC U3000 Pump system (Thermo Fisher Scientific, San Jose, CA) with an ACQUITY UPLC BEH C18 RP column (1.7 μm particle size, 2.1 × 100 mm; Waters, USA). Detailed specific parameters for the LC-MS/MS analysis are given in our previous research.31,32 The raw MS/MS files were processed using the Proteome Discoverer Software 1.4 (ESI Table S2 and ESI Fig. S2†). Protein identification was performed using the Sequest HT engine against the uniprot Arabidopsis thaliana database. The search parameters were as follows: trypsin was selected as the enzyme, with the tolerance set at one missed cleavage, a peptide allowance of 10 ppm, and an MS and MS/MS allowance of 0.02 Da. To be identified as important differentially abundant proteins (DAPs), a protein needed to contain at least one unique peptide with a p-value less than 0.05 and a fold change greater than 1.5 or less than 0.67.32 Identified proteins were annotated with their biological functions according to KEGG (http://www.genome.jp/kegg/) and the literature. Information on the DAPs was obtained from the Universal Protein Resource (http://www.uniprot.org/). Pathway enrichment analysis was performed using KOBAS 3.0 (http://kobas.cbi.pku.edu.cn/).
2.9. Statistical analysis
All data are expressed as mean values ± standard deviations of tests on three replicate independent broccoli sprouts. The data obtained were statistically analysed by one-way ANOVA and Tukey's test, and a p-value of less than 0.05 was considered significant. Relative gene expression was analysed by the 2−ΔΔCt method.33
3. Results and discussion
3.1. Effect of melatonin on growth performance, sprout length, fresh weight, malondialdehyde content, and electrolyte leakage of broccoli sprouts
Compared with the control, ZnSO4 treatment significantly inhibited the growth and development of broccoli sprouts (Fig. 1I), decreasing the sprout length and fresh weight (Fig. 1II and III). As indicators of membrane damage, electrolyte leakage and MDA content dramatically enhanced in broccoli sprouts under ZnSO4 treatment (p < 0.05) (Fig. 1IV and V). Fig. 1I shows that the growth of broccoli sprouts was better after exogenous melatonin was applied than under ZnSO4 stress alone. After germinating for 4 and 6 days under ZnSO4 plus melatonin treatment, the sprout length and fresh weight showed increases while electrolyte leakage and MDA content decreased significantly (p < 0.05) compared with ZnSO4 treatment alone (Fig. 1II–V). The above facts provide evidence that melatonin counters the adverse effects of ZnSO4 stress in broccoli sprouts.
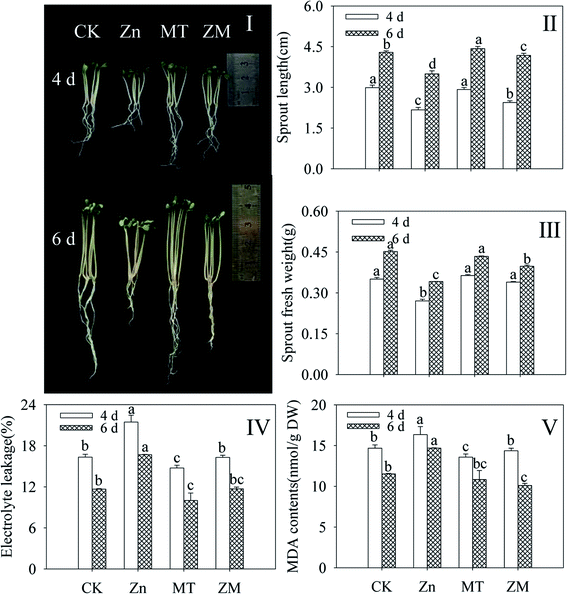 |
| Fig. 1 Effect of exogenous melatonin on growth performance (I), sprout length (II), fresh weight (III), electrolyte leakage (IV), and MDA content (V) of broccoli sprouts under ZnSO4 treatment during germination. Each data point represents the average of three independent biological replications (average ± SD). Lower case letters reflect the significance of the differences in indexes between treatments at the given germination times (p < 0.05). CK: control; Zn: ZnSO4; MT: melatonin; ZM: ZnSO4 + melatonin. | |
3.2. Effect of melatonin on glucosinolate content, isothiocyanate content, sulforaphane content, and myrosinase activity of broccoli sprouts
In plants, GLSs can be hydrolysed by MYR into ITCs. As shown in Fig. 2, compared with the control, the content of ITCs, sulforaphane and GLSs, and the MYR activity in broccoli sprouts all increased significantly during germination under ZnSO4 and ZnSO4 plus melatonin treatments (p < 0.05). Melatonin significantly enhanced the GLS and sulforaphane content after germination for 4 days (p < 0.05) compared with the ZnSO4 treatment alone (Fig. 2II and III). There was no significant difference (p > 0.05) in ITC content or MYR activity under ZnSO4 plus melatonin treatment in 4 day-old broccoli sprouts compared with those treated with ZnSO4 alone.
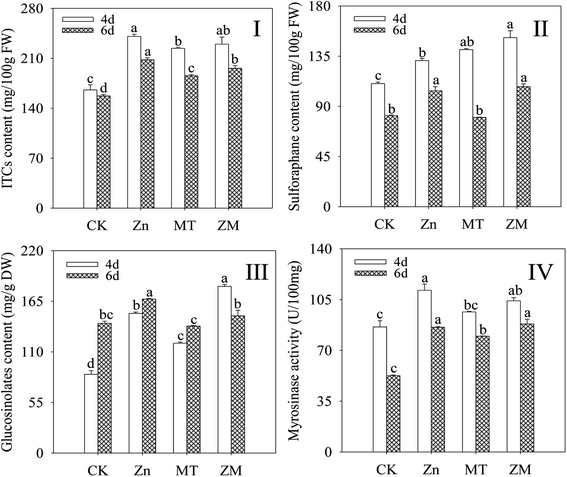 |
| Fig. 2 Effect of exogenous melatonin on ITC content (I), sulforaphane content (II), GLS content (III), and MYR activity (IV) of broccoli sprouts under ZnSO4 treatment during germination. Each data point represents the average of three independent biological replications (average ± SD). Lower case letters reflect the significance of the differences in indexes between treatments at the given germination times (p < 0.05). CK: control; Zn: ZnSO4; MT: melatonin; ZM: ZnSO4 + melatonin. | |
3.3. Effect of melatonin on total antioxidant capacity and peroxidase activity of broccoli sprouts
T-AOC and POD activity were dramatically increased (p < 0.05) in broccoli sprouts exposed to ZnSO4 treatment during germination (Fig. 3). However, the T-AOC and POD activity of 4 day-old broccoli sprouts under ZnSO4 plus melatonin treatment were significantly lower than those treated with ZnSO4 alone (p < 0.05).
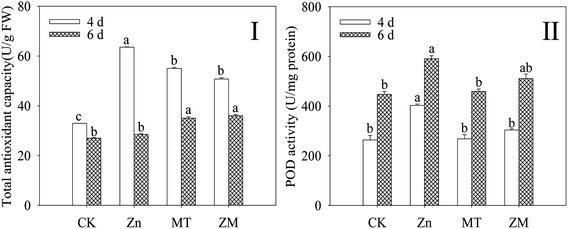 |
| Fig. 3 Effect of exogenous melatonin on T-AOC (I) and POD activity (I) of broccoli sprouts under ZnSO4 treatment during germination. Each data point represents the average of three independent biological replications (average ± SD). Lower case letters reflect the significance of the differences in indexes between treatments at the given germination times (p < 0.05). CK: control; Zn: ZnSO4; MT: melatonin; ZM: ZnSO4 + melatonin. | |
3.4. Effect of melatonin on intracellular free calcium of broccoli sprouts
Root tips of broccoli sprouts cultured in vermiculite for 4 days were cut into 4 mm lengths and then put into an HBSS buffer solution in the presence (Fig. 4B–E) or absence of Fluo-4 AM (Fig. 4A). The root tips were observed after incubation. The cell wall and cytoplasm of the broccoli sprout root tip without the Fluo-4 AM treatment both showed no spontaneous fluorescence. However, under the ZnSO4 stress and ZnSO4 plus melatonin treatments, the fluorescence intensities of the root tip cell walls treated with Fluo-4 AM were significantly higher than that of the control, exhibiting a brighter green fluorescence.
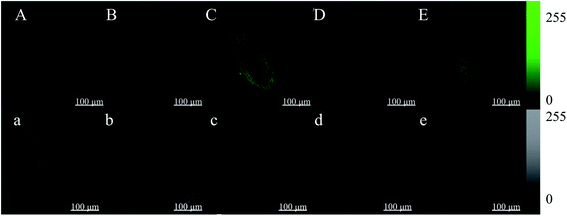 |
| Fig. 4 Changes in the calcium ion content of sprout root tip cells after different treatments for 4 days. (B) Control; (C) ZnSO4; (D) melatonin; (E) ZnSO4 + melatonin. (A) Root tip cells treated with distilled water without the addition of probe before observation under the laser scanning confocal microscope. (A–E) show pseudocolor images of Ca2+ in the root tip, and (a–e) show the corresponding pictures taken under a bright field. The color bars on the right side show the minimum (0) and maximum intensities (255). Scale bar = 100 μm. | |
3.5. Changes in gene expression of key enzymes in ITC synthesis in broccoli sprouts
As shown in Fig. 5, the expression of OX1 was induced by ZnSO4, melatonin and their combined treatment in 4 day-old broccoli sprouts while the expression of ST5b was reduced (p < 0.05) (Fig. 5II and III). ZnSO4 treatment also significantly reduced the expression of UGT74B1, while the other treatments had no significant effect (Fig. 5I). ZnSO4 plus melatonin treatment significantly increased the expression of MYB28 compared with the control (Fig. 5V). In ZnSO4 and ZnSO4 plus melatonin treated 4 day-old sprouts, the respective expression levels of AOP2 were 3.79- and 2.74-fold that of the control, respectively (Fig. 5VIII). ZnSO4 plus melatonin treatment significantly increased the expression of CYP83A1 and BOHMT1, while the other two treatments had no significant effect on them (Fig. 5VI and X). Compared with the control, other treatments all increased the expression of CYP83A1 (Fig. 5VI). ZnSO4 plus melatonin treatment increased the expression of BOHMT1 to 1.82 times that of the control (Fig. 5X). Compared with the control, ZnSO4 plus melatonin significantly increased the expression level of BOSAT1, while ZnSO4 treatment gave no significant change (p > 0.05) (Fig. 5IX).
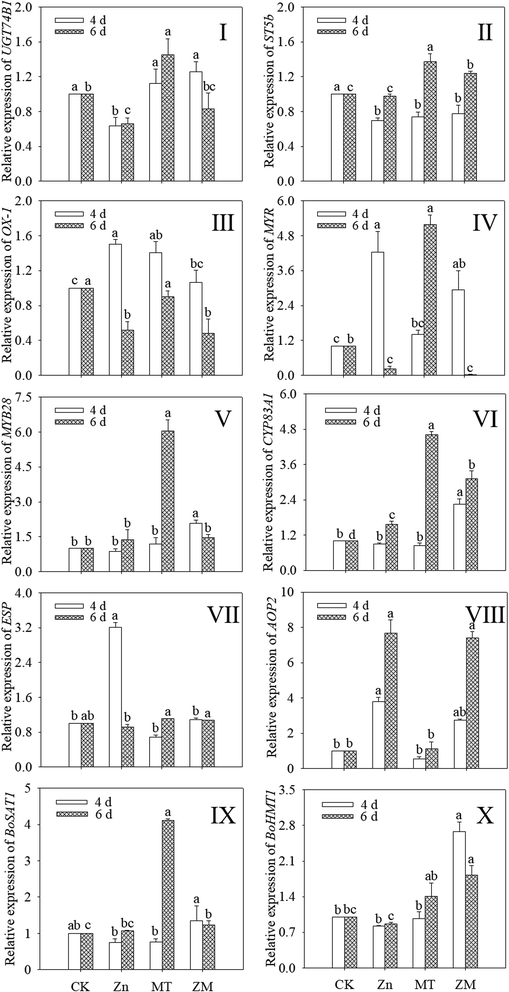 |
| Fig. 5 Changes in relative expression levels of UGT74B1 (I), ST5b (II), OX-1 (III), MYR (IV), MYB28 (V), CYP83A1 (VI), ESP (VII), AOP2 (VIII), BOSAT1 (IX), and BOHMT1 (X) in broccoli sprouts under different treatments during germination. Lower case letters reflect the significance of the differences in indexes between the treatments at the given germination times (p < 0.05). CK: control; Zn: ZnSO4; MT: melatonin; ZM: ZnSO4 + melatonin. | |
3.6. iTRAQ analysis and identification of differentially abundant proteins
In the present study, a total of 466 DAPs were identified from all replicates and different treatments used (ESI Table S3†). A total of 152 DAPs were identified in the Zn vs. CK samples, consisting of 150 up-regulated and 2 down-regulated proteins, 108 DAPs were identified in the MT vs. CK samples with 100 up-regulated and 8 down-regulated, 145 DAPs were identified in the ZM vs. Zn samples with 135 up-regulated and 10 down-regulated, and 165 DAPs were identified in the ZM vs. MT samples with 117 up-regulated and 48 down-regulated (Fig. 6 and 7). A hierarchical clustering analysis of the DAPs in the four comparison groups revealed different expression patterns. The ZM vs. Zn and Zn vs. CK groups had nine common DAPs; while 12 DAPs were found in both ZM vs. Zn and MT vs. CK, and 27 common DAPs were found in Zn vs. CK and ZM vs. MT. Only one DAP overlapped in all four comparison groups. A total of 92, 56, 115 and 114 DAPs were independently expressed in Zn vs. CK, MT vs. CK, ZM vs. Zn, and ZM vs. MT, respectively (Fig. 6).
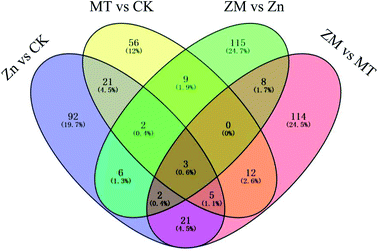 |
| Fig. 6 Venn diagram showing the numbers of DAPs in broccoli sprouts dependent on the different treatments. CK: control; Zn: ZnSO4; MT: melatonin; ZM: ZnSO4 + melatonin. | |
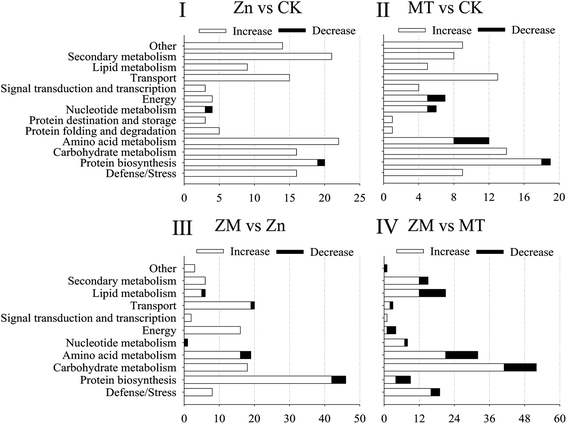 |
| Fig. 7 The abundance of DAPs in each functional classification for the comparison groups. The x-axis represents the numbers of DAPs enriched in each functional classification; the y-axis specifies the functional classifications. CK: control; Zn: ZnSO4; MT: melatonin; ZM: ZnSO4 + melatonin. | |
According to the molecular functions listed on the UniProt and KEGG websites, these DAPs could be divided into 13 functional classes, i.e., defense/stress, protein biosynthesis, carbohydrate metabolism, amino acid metabolism, protein folding and degradation, protein destination and storage, nucleotide metabolism, energy, signal transduction and transcription, transport, lipid metabolism, secondary metabolism, and other (Fig. 7). After germinating for 4 days under ZnSO4 plus melatonin, the abundance of all of the DAPS in the defence/stress, secondary metabolism, signal transduction and transcription, energy, and carbohydrate metabolism classes increased significantly, while the nucleotide metabolism-related DAPs decreased compared with the levels in the sprouts under ZnSO4 stress alone (Fig. 7).
These DAPs were analysed using bioinformatics approaches to obtain relevant pathway information. All of the identified peptides and DAPs under the different treatments were classified into three major gene ontology (GO) categories: biological processes (BP), cellular components (CC), and molecular functions (MF). The most common biological processes were oxidation–reduction processes; the most common molecular functions related to structural constituents of the ribosome and metal ion binding; and the most common cellular components were in the cytosol, cytoplasm and chloroplasts (Fig. 8).
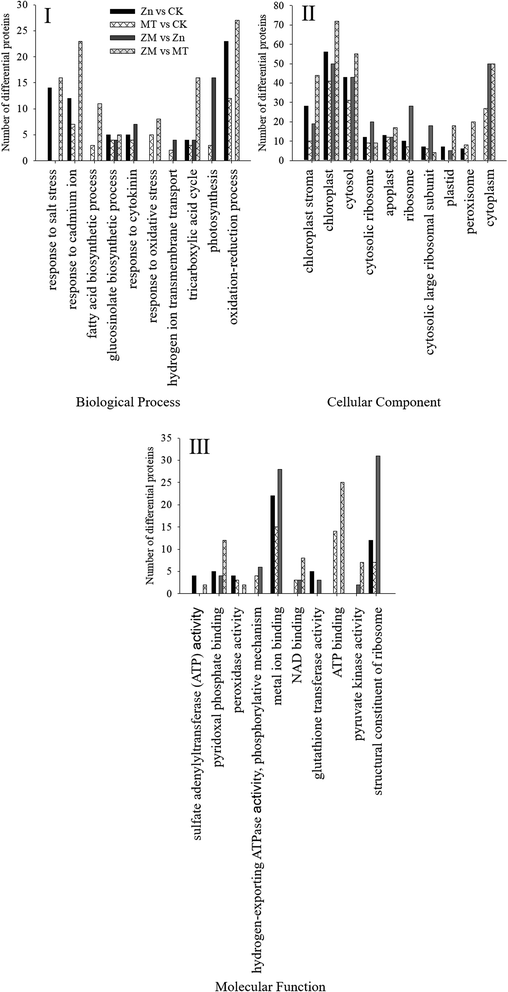 |
| Fig. 8 GO classification of all DAPs found in broccoli sprouts under the different treatments. (I) Number of proteins in pathways of the biological process classification. (II) Number of proteins in different cellular components. (III) Number of proteins in pathways of the molecular function classification. CK: control; Zn: ZnSO4; MT: melatonin; ZM: ZnSO4 + melatonin. | |
In order to analyse the affected metabolic pathways, the DAPs were further researched using the KEGG database. The pathway enrichment analysis identified 17, 16, 14 and 15 KEGG pathways for the Zn vs. CK, MT vs. CK, ZM vs. Zn and ZM vs. MT groups, respectively, taking a p-value of less than 0.05 as the threshold (Table 1). They participated in pathways involving for example carbon metabolism, biosynthesis of secondary metabolites and selenocompound metabolism.
Table 1 Primary reagent pathway enrichment analysis of DAPs in broccoli sprouts
Pathway ID |
Pathway |
Input number |
p-Value |
Zn vs. CK |
MT vs. CK |
ZM vs. Zn |
ZM vs. MT |
Zn vs. CK |
MT vs. CK |
ZM vs. Zn |
ZM vs. MT |
ath01100 |
Metabolic pathways |
64 |
36 |
61 |
106 |
1.70 × 10−43 |
2.62 × 10−20 |
9.31 × 10−37 |
4.79 × 10−101 |
ath01110 |
Biosynthesis of secondary metabolites |
36 |
19 |
23 |
88 |
3.89 × 10−24 |
6.92 × 10−11 |
1.34 × 10−10 |
5.62 × 10−95 |
ath00920 |
Sulfur metabolism |
12 |
2 |
1 |
7 |
7.29 × 10−19 |
0.0061 |
1.0000 |
1.70 × 10−9 |
ath00450 |
Selenocompound metabolism |
9 |
2 |
1 |
4 |
1.84 × 10−16 |
0.0011 |
1.0000 |
1.88 × 10−6 |
ath01200 |
Carbon metabolism |
16 |
9 |
20 |
61 |
3.84 × 10−14 |
1.09 × 10−7 |
1.47 × 10−18 |
1.32 × 10−84 |
ath01230 |
Biosynthesis of amino acids |
13 |
3 |
10 |
47 |
3.60 × 10−11 |
0.0344 |
1.75 × 10−7 |
6.66 × 10−61 |
ath00966 |
Glucosinolate biosynthesis |
6 |
2 |
4 |
5 |
1.63 × 10−9 |
0.0024 |
5.93 × 10−6 |
2.06 × 10−7 |
ath00380 |
Tryptophan metabolism |
7 |
4 |
2 |
5 |
6.78 × 10−9 |
2.83 × 10−5 |
0.0279 |
1.13 × 10−5 |
ath00190 |
Oxidative phosphorylation |
9 |
8 |
10 |
1 |
7.09 × 10−8 |
6.27 × 10−8 |
1.06 × 10−8 |
1.0000 |
ath03010 |
Ribosome |
11 |
6 |
28 |
4 |
1.56 × 10−6 |
0.0015 |
1.27 × 10−24 |
0.1501 |
ath00020 |
Citrate cycle (TCA cycle) |
4 |
3 |
2 |
20 |
0.0001 |
0.0007 |
0.0306 |
7.39 × 10−30 |
ath01212 |
Fatty acid metabolism |
3 |
3 |
1 |
14 |
0.0019 |
0.0007 |
0.2308 |
2.21 × 10−19 |
ath00195 |
Photosynthesis |
3 |
3 |
7 |
1 |
0.0055 |
0.0021 |
1.54 × 10−7 |
1.0000 |
ath04146 |
Peroxisome |
2 |
4 |
1 |
13 |
0.0375 |
7.17 × 10−5 |
1.0000 |
1.59 × 10−16 |
ath00620 |
Pyruvate metabolism |
3 |
2 |
9 |
24 |
0.0057 |
0.0267 |
3.32 × 10−10 |
4.33 × 10−34 |
ath00061 |
Fatty acid biosynthesis |
3 |
1 |
1 |
8 |
0.0004 |
0.0952 |
0.1418 |
1.55 × 10−11 |
ath00710 |
Carbon fixation in photosynthetic organisms |
2 |
1 |
8 |
15 |
0.0342 |
1.0000 |
1.62 × 10−9 |
4.52 × 10−20 |
3.7. Discussion
Stressful conditions can strongly enhance the ITC content of plants.34 In the present study, under ZnSO4 treatment, the ITC content in broccoli sprouts significantly increased (Fig. 2I), indicating that broccoli sprouts can be used as a good source of functional health components. However, ZnSO4 stress also inhibited the growth and development of the sprouts (Fig. 1I–III). Stress triggers reactive oxygen species (ROS) accumulation and breaks down the balance between ROS generation and detoxification in plants.35 The accumulation of ROS can induce lipid peroxidation and chlorophyll degradation, and cause the loss of cell membrane integrity.36 Compared with the control, electrolyte leakage and MDA content increased markedly in broccoli sprouts under ZnSO4 treatment (Fig. 1IV and V).
As an abiotic anti-stressor, melatonin mediates multiple physiological processes in plants such as growth, development, and enhanced tolerance of abiotic stress.15 After germinating under ZnSO4 plus melatonin treatment, the sprouts' lengths and fresh weights increased while electrolyte leakage and MDA content decreased significantly (p < 0.05) compared to those germinated with ZnSO4 treatment alone (Fig. 1). The results confirmed that exogenous melatonin could not only alleviate the adverse effects of ZnSO4 stress on sprout growth and development, but also increase the sulforaphane content in broccoli sprouts (Fig. 1 and 2).
As a plant cell signaling ion, Ca2+ plays a key role in adjusting plant growth and development under biotic and abiotic stress. External stimulation can increase intracellular Ca2+, which moderates transcription through the bioregulation of transcription factors. In the present study, it was observed that ZnSO4 stress treatment and ZnSO4 plus melatonin could significantly induce Ca2+ influx into sprout root tip cells (Fig. 4), similar to the increase seen in Ca2+ in barley root tip cells under salt stress.37
The transcription levels of key genes including MYB28, UGT74B1, ST5b, OX1, MYR, ESP, CYP83A, AOP2, BOSAT1 and BOHMT1 under the different treatments in broccoli sprouts were analysed using qRT-PCR (Fig. 5). MYB28 is a major gene which regulates aliphatic-glucosinolate synthesis;38 UGT74B1 and ST5b are involved in the formation of the core glucosinolate biosynthesis;39 FMOGS-OX1 is involved in side-chain modification of glucosinolate; and MYR and ESP can regulate the hydrolysis of glucosinolates to ITCs.13 In the present study, the expression of ITC synthesis-related genes showed different changes under the treatments of ZnSO4 and ZnSO4 plus melatonin. This phenomenon has also been observed in heat shock and hypoxia treatments.30 ZnSO4 up-regulated the gene expression of MYR, ESP, FMOGS-OX1, and AOP2 to increase the content of glucosinolates, thereby enriching ITC content. The increase in MYR activity might be related to its gene expression. Yang et al.13 found that ZnSO4 stimulated the formation of ITCs by enhancing the gene expression and activity of MYR, and the gene expression and glucosinolate content in broccoli sprouts. After germination for 4 days, the reduction of glucosinolate content under melatonin treatment was not related to the expression of MYR, ESP, AOP2 or ST5b. Moreover, MYR activity was not consistent with its gene expression level.
Methylthioalkylmalate synthase 1 (MAM1), isopropylmalate isomerase 2 (IPMI2), 3-isopropylmalate dehydratase large subunit (IIL1), 3-isopropylmalate dehydrogenase (IMD1), branched-chain-amino-acid aminotransferase 3 (BCAT3), cytosolic sulfotransferase 16 (STO16), cytosolic sulfotransferase 17 (SOT17), cytosolic sulfotransferase 18 (SOT18), cytochrome P450 83B1 (CYP83B1), myrosinase 1 (MYR1), myrosinase 2 (MYR2), epithiospecifier protein (ESP) and nitrile-specifier protein 2 (NSP2) play an important role in the formation of ICTs.40 In the present study, from the iTRAQ data, IPMI2 (A0A178VZE1), IIL1 (Q94AR8), IMD1 (Q5XF32), STO16 (Q9C9D0), SOT17 (Q9FZ80) and CYP83B1 (O65782) involved in the metabolism of aliphatic glucosinolates differed markedly in abundance under the different treatments, while MAM1 (Q9FG67), BCAT3 (Q9M401) and SOT18 (Q9C9C9) involved in the metabolism of indole glucosinolate metabolism were not significantly changed (ESI Table S1†). The ZnSO4 and ZnSO4 plus melatonin treatments positively regulated the metabolism of aliphatic glucosinolates by increasing the relative abundance of IPMI2, IMD1, STO16 and SOT17. The results indicate that the up-regulation of these proteins had a positive regulatory effect on the metabolism of aliphatic thiocyanates, and thus increased the ITC content. In the present study, some enzymes (CYP79F1, UGT74B1, FMOGS-OX1, AOP2), involved in the formation of the core structure of the aliphatic glucosinolates in broccoli sprouts were not detected. It might be that the abundance of these proteins was too low to be detected in this test, or that these enzymes in broccoli were less compatible with those in the Arabidopsis thaliana database; these proteins were also not detected in the previous study.30 MYR1 (P37702), MYR2 (Q9C5C2), ESP (Q8RY71) and NSP2 (O49326) were detected; Koroleva41 and Guo et al.30 also detected MYR1 and MYR2. Under ZnSO4 treatment, the abundance of MYR1, MYR2 and ESP in broccoli sprouts increased significantly compared with the control, while the abundance of NSP2 did not change significantly. MYR activity was consistent with protein expression, and the expression of the ESP gene was consistent with that of the protein, showing that ZnSO4 enriches ITCs in broccoli sprouts by increasing the gene expression and protein expression of glucosinolates, and increasing MYR activity and the abundance of MYR1 and MYR2. Compared with ZnSO4 treatment, the abundance of MYR1, MYR2 and ESP did not significantly differ under ZnSO4 plus melatonin treatment, while MYR activity and MYR gene expression increased compared with the control, indicating that ZnSO4 plus melatonin improves MYR activity and induces MYR1 and MYR2 protein abundance, therefore enriching ITCs.
In the present study, under ZnSO4 stress, antioxidant enzyme-related DAPs were stimulated, such as superoxide dismutase (F4J504) and peroxidase (F4IQ05). Compared with ZnSO4 treatment, ZnSO4 plus melatonin treatment enhanced the antioxidant capacity of broccoli sprouts by increasing the F4IQ05 abundance, while the F4J504 protein abundance did not change significantly.
According to the hierarchical clustering analysis of DAPs, the four comparison groups showed different expression patterns (ESI Fig. S1†). Carbohydrate catabolism and amino acid metabolism produce the energy for many processes, and also provide basic carbon skeletons for the biosynthesis of secondary metabolites.42 In the current study, one of the most remarkable changes associated with melatonin application was the up-regulation of many proteins related to photosynthesis and carbohydrate metabolism in response to ZnSO4 stress. During photosynthesis, the reaction-center subunit of the photosystem serves as the key site in plants that can be damaged by stress such as that of ZnSO4. ZnSO4 stress increased photosynthetic proteins in broccoli sprouts (P56778, Q8HT11), while melatonin treatment decreased photosynthetic proteins (P56778). These results indicate that ZnSO4 stress inhibits photosynthesis in seedlings, whereas melatonin can enhance energy conversion during ZnSO4 stress.
Ribosomes are large macromolecular ribosomal proteins (r-proteins) responsible for catalysing protein synthesis in the cell and play a crucial role in regulating cell growth, differentiation, and development.43 In the present study, the majority of r-proteins were up-regulated significantly under ZnSO4 stress. However, plants treated with melatonin showed significant increases in r-proteins compared to untreated plants under ZnSO4 stress alone. For instance, nine 40S r-proteins (A0A178UFG0, A0A178VBG6, F4K5C7, P59224, Q8VYK6, Q93VH9, Q9M0E0, Q9SIK2 and Q9SIW5), 14 60S r-proteins (A0A178V6A2, A8MQA1, P38666, P41127, P51420, P59231, P60040, Q42064, Q42347, Q8VZB9, Q93VI3, Q9FF90, Q9M0E2 and Q9SF53), two 30S r-proteins (A0A1B1W4U6, A0A1B1W4X5) and one 50S r-protein (Q9SYL9) were dramatically induced by ZnSO4 stress. A previous study has also shown that melatonin enhances heat resistance by promoting protein biosynthesis in tomato plants.44 These results indicate that the promotion of protein biosynthesis might be a very important response of broccoli sprouts under melatonin plus ZnSO4 treatment.
4. Conclusions
Physiological and comparative proteomic analyses have revealed the putative molecular mechanism of exogenous melatonin treatment on induced ZnSO4 stress responses (Fig. 9). Exogenous melatonin reduced electrolyte leakage and MDA content, induced the levels of peroxidase, ribosomal and defence-related proteins, and thus improved the growth and development of broccoli sprouts and alleviated the negative effects caused by ZnSO4 stress. Meanwhile, compared with the control, exogenous melatonin contributed to glucoraphanin biosynthesis and the hydrolysis of glucoraphanin to form ITCs in broccoli sprouts under ZnSO4 stress via up-regulating the expression levels of glucoraphanin biosynthesis genes, increasing glucosinolate content and myrosinase activity, and increasing the abundance of myrosinase and epithiospecifier proteins. These results provide novel insights into the accumulation of ITCs in broccoli sprouts and provide new evidence to support the multiple roles of melatonin in moderating ZnSO4 stress.
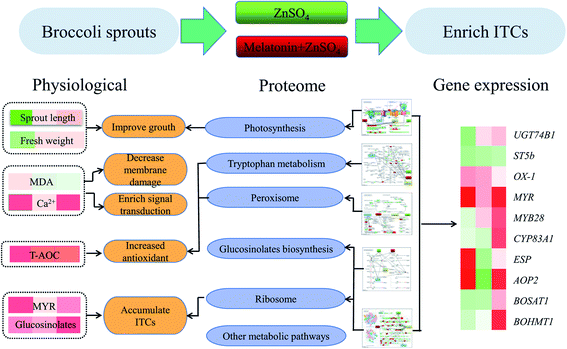 |
| Fig. 9 Putative molecular mechanism of exogenous melatonin treatment on induced ZnSO4 stress responses in 4 day-old broccoli sprouts. | |
Conflicts of interest
There are no conflicts of interest to declare.
Acknowledgements
This work was financially supported by the China Postdoctoral Science Foundation (funded project 2019M651978) and the Qing Lan Project of Yangzhou University.
References
- M. Nestle, Broccoli sprouts in cancer prevention, Nutr. Rev., 1998, 56, 127–130 CrossRef CAS.
- L. Campbell, Brassicas: Cooking the world's healthiest vegetables; kale, cauliflower, broccoli, brussels sprouts and more, Libr. J., 2014, 139, 124–125 Search PubMed.
- D. Pisuttharachai, W. Nalinanon and N. Areechon, Effects of dietary supplementation with broccoli sprouts (Brassica oleracea) on the hematology and immunological responses of nile tilapia (Oreochromis niloticus), J. Fisheries and Environ., 2020, 44, 65–75 Search PubMed.
- M. Mitsiogianni, G. Koutsidis, N. Mavroudis, D. T. Trafalis, S. Botaitis, R. Franco, V. Zoumpourlis, T. Amery, A. Galanis, A. Pappa and M. I. Panayiotidis, The role of isothiocyanates as cancer chemo-preventive, chemo-therapeutic and anti-melanoma agents, Antioxidants, 2019, 8, 35–39 CrossRef PubMed.
- S. N. T. Ngo and D. B. Williams, Protective effect of isothiocyanates from cruciferous vegetables on breast cancer: epidemiological and preclinical perspectives, Anti-Cancer Agents Med. Chem., 2020, 9, 13–19 Search PubMed.
- F. Burcul, I. G. Mekinic, M. Radan, P. Rollin and I. Blazevic, Isothiocyanates: cholinesterase inhibiting, antioxidant, and anti-inflammatory activity, J. Enzyme Inhib. Med. Chem., 2018, 33, 577–582 CrossRef CAS.
- S. Mohanty, A. K. Sahoo, V. B. Konkimalla, A. Pal and S. C. Si, Naringin in combination with isothiocyanates as liposomal formulations potentiates the anti-inflammatory activity in different acute and chronic animal models of rheumatoid arthritis, ACS Omega, 2020, 5, 28319–28332 CrossRef CAS PubMed.
- Y. Bai, X. L. Wang, S. Zhao, C. Y. Ma, J. W. Cui and Y. Zheng, Sulforaphane protects against cardiovascular disease via Nrf2 activation, Oxid. Med. Cell. Longevity, 2015, 2015, 13 Search PubMed.
- J. S. Hooper, S. H. Hadley, K. F. Morris, J. W. Breslin, J. B. Dean and T. E. Taylor-Clark, Characterization of cardiovascular reflexes evoked by airway stimulation with allylisothiocyanate, capsaicin, and ATP in sprague-dawley rats, J. Appl. Physiol., 2016, 120, 580–591 CrossRef CAS PubMed.
- M. Esteve, Mechanisms underlying biological effects of cruciferous glucosinolate-derived isothiocyanates/indoles: a focus on metabolic syndrome, Front. Nutr., 2020, 7, 1–9 CrossRef PubMed.
- A. Esfandiari, A. Saei, M. J. McKenzie, A. J. Matich, M. Babalar and D. A. Hunter, Preferentially enhancing anti-cancer isothiocyanates over glucosinolates in broccoli sprouts: how NaCl and salicylic acid affect their formation, Plant Physiol. Biochem., 2017, 115, 343–353 CrossRef CAS PubMed.
- R. Guo, G. Yuan and Q. Wang, Effect of NaCl treatments on glucosinolate metabolism in broccoli sprouts, J. Zhejiang Univ., Sci., B, 2013, 14, 124–131 CrossRef CAS PubMed.
- R. Q. Yang, L. P. Guo, X. L. Jin, C. Shen, Y. L. Zhou and Z. X. Gu, Enhancement of glucosinolate and sulforaphane formation of broccoli sprouts by zinc sulphate via its stress effect, J. Funct. Foods, 2015, 13, 345–349 CrossRef CAS.
- T. A. Khan, Q. Fariduddin, F. Nazir and M. Saleem, Melatonin in business with abiotic stresses in plants, Physiol. Mol. Biol. Plants, 2020, 26, 1931–1944 CrossRef CAS.
- M. Moustafa-Farag, A. Almoneafy, A. Mahmoud, A. Elkelish, M. B. Arnao, L. F. Li and S. Y. Ai, Melatonin and its protective role against biotic stress impacts on plants, Biomolecules, 2020, 10, 12–19 Search PubMed.
- A. Khan, M. Numan, A. L. Khan, I. J. Lee, M. Imran, S. Asaf and A. Al-Harrasi, Melatonin: awakening the defense mechanisms during plant oxidative stress, Plants, 2020, 9, 22–28 Search PubMed.
- E. Michard and A. A. Simon, Melatonin's antioxidant properties protect plants under salt stress, Plant, Cell Environ., 2020, 43, 2587–2590 CrossRef CAS PubMed.
- R. Wang, M. L. Cheng, H. N. Liu, D. Q. Zhao and J. Tao, Effects of melatonin on flavonoids content and related gene expression levels of gardenia under dark conditions, Bull. Bot. Res., 2018, 38, 559–567 Search PubMed.
- S. W. Gao, W. Y. Ma, X. N. Lyu, X. L. Cao and Y. X. Yao, Melatonin may increase disease resistance and flavonoid biosynthesis through effects on DNA methylation and gene expression in grape berries, BMC Plant Biol., 2020, 20, 15–21 CrossRef PubMed.
- X. Li, G. J. Ahammed, X. N. Zhang, L. Zhang, P. Yan, L. P. Zhang, J. Y. Fu and W. Y. Han, Melatonin-mediated regulation of anthocyanin biosynthesis and antioxidant defense confer tolerance to arsenic stress in camellia sinensis L, J. Hazard. Mater., 2021, 403, 123–132 Search PubMed.
- L. Chen, J. Tian, S. F. Wang, T. T. Song, J. Zhang and Y. C. Yao, Application of melatonin promotes anthocyanin accumulation in crabapple leaves, Plant Physiol. Biochem., 2019, 142, 332–341 CrossRef CAS PubMed.
- Q. Sun, L. Liu, L. Zhang, H. Lv, Q. He, L. Guo, X. Zhang, H. He, S. Ren, N. Zhang, B. Zhao and Y. D. Guo, Melatonin promotes carotenoid biosynthesis in an ethylene-dependent manner in tomato fruits, Plant Sci., 2020, 298, 1–11 CrossRef.
- L. Cao, X. Jin, Y. Zhang, M. Zhang and Y. Wang, Transcriptomic and metabolomic profiling of melatonin treated soybean (Glycine max L.) under drought stress during grain filling period through regulation of secondary metabolite biosynthesis pathways, PLoS One, 2020, 15, 1–9 Search PubMed.
- L. Wei, C. Liu, H. Zheng and L. Zheng, Melatonin treatment affects the glucoraphanin-sulforaphane system in postharvest fresh-cut broccoli (Brassica oleracea L.), Food Chem., 2020, 307, 1–7 CrossRef PubMed.
- K. V. M. Rao and T. V. S. Sresty, Antioxidative parameters in the seedlings of pigeonpea (Cajanus cajan (L.) Millspaugh) in response to Zn and Ni stresses, Plant Sci., 2000, 157, 113–128 CrossRef.
- M. L. Dionisio-Sese and S. Tobita, Antioxidant responses of rice seedlings to salinity stress, Plant Sci., 1998, 135, 1–9 CrossRef CAS.
- M. Burow, A. Losansky, R. Muller, A. Plock, D. J. Kliebenstein and U. Wittstock, The genetic basis of constitutive and herbivore-induced ESP-independent nitrile formation in arabidopsis, Plant Physiol., 2009, 149, 561–574 CrossRef CAS PubMed.
- L. Guo, R. Yang, Z. Wang, Q. Guo and Z. Gu, Glucoraphanin, sulforaphane and myrosinase activity in germinating broccoli sprouts as affected by growth temperature and plant organs, J. Funct. Foods, 2014, 9, 70–77 CrossRef CAS.
- D. Jiao, M. C. Yu, J. H. Hankin, S. H. Low and F. L. Chung, Total isothiocyanate contents in cooked vegetables frequently consumed in Singapore, J. Agric. Food Chem., 1998, 46, 1055–1058 CrossRef CAS.
- L. P. Guo, Z. X. Gu, X. L. Jin and R. Q. Yang, iTRAQ - based proteomic and physiological analyses of broccoli sprouts in response to the stresses of heat, hypoxia and heat plus hypoxia, Plant Soil, 2017, 414, 355–377 CrossRef CAS.
- C. Cheng, Y. Liu, W. M. Fang, J. Tao, Z. F. Yang and Y. Q. Yin, iTRAQ-based proteomic and physiological analyses of mustard sprouts in response to heat stress, RSC Adv., 2020, 10, 6052–6062 RSC.
- Y. Q. Yin, F. Qi, L. Gao, S. Q. Rao, Z. Q. Yang and W. M. Fang, iTRAQ-based quantitative proteomic analysis of dark-germinated soybeans in response to salt stress, RSC Adv., 2018, 8, 17905–17913 RSC.
- K. J. Livak and T. D. Schmittgen, Analysis of relative gene expression data using real-time quantitative PCR and the 2(T)(-Delta Delta C) method, Methods, 2001, 25, 402–408 CrossRef CAS PubMed.
- A. Vanduchova, P. Anzenbacher and E. Anzenbacherova, Isothiocyanate from broccoli, sulforaphane, and its properties, J. Med. Food, 2019, 22, 121–126 CrossRef CAS PubMed.
- G. Miller, N. Suzuki, S. Ciftci-Yilmaz and R. Mittler, Reactive oxygen species homeostasis and signalling during drought and salinity stresses, Plant, Cell Environ., 2010, 33, 453–467 CrossRef CAS.
- J. Larkindale and B. Huang, Thermotolerance and antioxidant systems in Agrostis stolonifera: Involvement of salicylic acid, abscisic acid, calcium, hydrogen peroxide, and ethylene, J. Plant Physiol., 2004, 161, 405–413 CrossRef CAS PubMed.
- Y. Ma, P. Wang, T. Zhou, Z. J. Chen, Z. X. Gu and R. Q. Yang, Role of Ca2+ in phenolic compound metabolism of barley (Hordeum vulgare L.) sprouts under NaCl stress, J. Sci. Food Agric., 2019, 99, 5176–5186 CrossRef CAS PubMed.
- L. Zhuang, K. Xu, Y. Zhu, F. Wang, J. Xiao and L. Guo, Calcium affects glucoraphanin metabolism in broccoli sprouts under ZnSO4 stress, Food Chem., 2021, 334 DOI:10.1016/j.foodchem.2020.127520.
- M. Tian, Y. Yang, F. W. Avila, T. Fish, H. Yuan, M. X. Hui, S. Y. Pan, T. W. Thannhauser and L. Li, Effects of selenium supplementation on glucosinolate biosynthesis in broccoli, J. Agric. Food Chem., 2018, 66, 8036–8044 CrossRef CAS.
- I. E. Sonderby, F. Geu-Flores and B. A. Halkier, Biosynthesis of glucosinolates-gene discovery and beyond, Trends Plant Sci., 2010, 15, 283–290 CrossRef PubMed.
- O. A. Koroleva and R. Cramer, Single-cell proteomic analysis of glucosinolate-rich S-cells in Arabidopsis thaliana, Methods, 2011, 54, 413–423 CrossRef CAS PubMed.
- Y. Z. Chen, Q. Y. Pang, S. J. Dai, Y. Wang, S. X. Chen and X. F. Yan, Proteomic identification of differentially expressed proteins in Arabidopsis in response to methyl jasmonate, J. Plant Physiol., 2011, 168, 995–1008 CrossRef CAS PubMed.
- I. F. Chang, K. Szick-Miranda, S. Q. Pan and J. Bailey-Serres, Proteomic characterization of evolutionarily conserved and variable proteins of arabidopsis cytosolic ribosomes, Plant Physiol., 2005, 137, 848–862 CrossRef CAS.
- W. Xu, S. Y. Cai, Y. Zhang, Y. Wang, G. J. Ahammed, X. J. Xia, K. Shi, Y. H. Zhou, J. Q. Yu, R. J. Reiter and J. Zhou, Melatonin enhances thermotolerance by promoting cellular protein protection in tomato plants, J. Pineal Res., 2016, 61, 457–469 CrossRef CAS PubMed.
Footnote |
† Electronic supplementary information (ESI) available. See DOI: 10.1039/d1ra00696g |
|
This journal is © The Royal Society of Chemistry 2021 |
Click here to see how this site uses Cookies. View our privacy policy here.