DOI:
10.1039/D0RA10671B
(Paper)
RSC Adv., 2021,
11, 9572-9585
Smart edible coating films based on chitosan and beeswax–pollen grains for the postharvest preservation of Le Conte pear
Received
19th December 2020
, Accepted 11th February 2021
First published on 3rd March 2021
Abstract
Smart edible coating films can be used in food packaging. For this application, they must have good physical and mechanical properties. Herein, chitosan–beeswax based film is used to preserve Le Conte pears postharvest. The chitosan–beeswax films were characterized using XRD, FT-IR, and SEM analysis. Moreover, all films showed good self-healing aptitudes ranging from 86.7 to 96.3. The film treated with pollen grains showed an enhanced water contact angle compared with the chitosan film. The chitosan–beeswax/pollen grain film exhibited a two-fold lower WVTR value compared to the chitosan film, and showed the tendency to increase the stiffness of the film. The elongation% at break was reduced from 35.81 to 14.09. Fruit quality parameters were determined in cold storage for 105 days during shelf life after a simulated marketing period of 7 days. All coated fruits successfully showed decrease in weight loss, decay and rate of softening. Therefore, chitosan–beeswax/pollen grains can be considered safe and effective coating for the fruit preservation.
1. Introduction
Currently, biodegradable edible coating alternatives for postharvest fruit preservation are attracting attention from researchers. Fortunately, smart edible coatings are one of these alternatives. Auto-healing coatings that can repair their damage automatically or by means of external stimuli have received attention recently.1–4 Generally, self-healing materials include two main categories: inherent self-healing materials that exhibit dynamic reversibility of covalent and non-covalent bonds,5–8 such as Schiff bases,9 host–guest interaction,10 Diels–Alder,11 and hydrogen bonding,12 and extrinsic self-healing materials that rely on the performance of agents encapsulated into hollow structures, microspheres, and nanospheres.13,14 Inherent self-healing materials have aroused more interest than extrinsic ones because the latter frequently necessitate healing agents to repair the damage that occurs.15
Pear (Pyrus communis, L.) is one of the oldest plants grown in Egypt and is considered to be one of the most consumed fruits in the world. Its fruits are characterized by attractive flavor and aroma16 as well as by high amounts of nutritional value, such as vitamins and minerals.17,18 It is classified as a climacteric fruit, showing a peak in ethylene production and respiration rate during development. Hence, some deterioration is seen in the color, appearance, firmness, sugar content and acidity.19 Due to these factors, pear fruits are relatively perishable and difficult to maintain well for a longer period.20,21 Accordingly, fruits suffer from luster loss, shrink and rotting due to relatively high water transpiration, nutrient loss and infection with microorganisms during storage.22 Thus, in order to extend the shelf life of stored pear fruits, some technological advances must be applied to slow and limit the decline in fruit quality and generation of ethylene23 as well as lengthen the postharvest life and maintain the quality of freshness.24
Edible coatings are non-toxic packaging materials that can regulate metabolic processes, improve the appearance of stored fruits, delay deterioration, and preserve product quality by extending the shelf life of fruits as well as ensure their brightness as an attractive factor for consumers.25–28
Recently, chitosan has received special attention in developing edible coatings due to its bioactivity, antimicrobial, and film forming capability.29–33 Arnon et al.26 fabricated a coating of carboxymethyl cellulose and chitosan layer by layer for the preservation of citrus fruits. Pollen grains are the major sources of protein, fats, vitamins and minerals.34 Wax coatings create a modifying atmosphere around the fruit and provide a semi-permeable barrier for raising carbon dioxide levels and reducing oxygen levels, thereby reducing respiration, water loss, oxidation reaction rates and metabolic activities, especially respiration and transpiration. Therefore, coating fruits increases their resistance to pathogens and increases their storage and marketing capabilities.35,36
The main objective of this study was the preparation and characterization of a self-healing chitosan–beeswax-based film for maintaining quality criteria and extending the storage period of Le Conte pear fruits. Edible hydrophobic components, such as beeswax and pollen grains, were blended with chitosan to improve its physical and barrier properties while maintaining suitable mechanical characteristics. Three modifications were studied to reach these goals: beeswax–pollen grains, chitosan–glycerol/CaCl2·2H2O, and chitosan–beeswax/glycerol/CaCl2·2H2O/pollen grains, coded as (BW/PG), Chito and Chito–BW/PG, respectively, in comparison with water dipping as a control.
2. Experimental
2.1. Materials
2.1.1. Pear orchard. The target fruit sample of this investigation was Le Conte pear trees (Pyrus communis, L.). The trees were twenty years old, approximately uniform and vigorous, and budded on Pyrus communis root stock. They were developed in a loamy clay soil. The space was 5 × 5 m under a flood irrigation system. The healthy, almost uniform trees were almost identical in growth vigor and received the recommended horticultural treatments.
2.1.2. Fruit sample. Harvested mature fruits free from pathogen infection and blemishes at the beginning of August 2018 and 2019 were selected and delivered to the laboratory of Faculty of Agriculture Cairo University (Agriculture Development Systems (ADS) project). Chitosan (medium Mwt) was purchased from Sigma Aldrich. Beeswax (melting point 61–65 °C) was purchased from El Nasr Pharmaceutical Chemicals; CaCl2·2H2O was purchased from Loba Chemie.
2.2. Saponification and acid numbers of beeswax
The saponification and acid numbers of the beeswax were estimated according to the method reported by Elena et al.37 The estimated saponification and acid number values were 542.75 mg KOH/g and 26.64 mg KOH/g, respectively.
2.3. Preparation of the self-healing chitosan–beeswax-based composite coating film
Firstly, beeswax pollen grain emulsion (BW/PG) was prepared by dissolving 0.5 g beeswax, 0.2 g glycerol, 0.5 g pollen grains and 0.2 g tween 80 in 100 mL hot distilled water. The whole mixture of the film-forming solution was homogenized at 70 °C for 2 minutes.
Secondly, the chitosan film-forming solution was prepared as described: chitosan (1 g) was dissolved in acetic acid (100 mL, 1%) and stirred at room temperature. After that, glycerol (0.2 g) and CaCl2·2H2O (30 mL, 0.4%) were added. Then, the solution was stirred for 30 minutes.
Then, the chitosan–beeswax/pollen grain coating composite was prepared, as described previously, in two steps: 0.5 g beeswax and 0.2 g glycerol, followed by 0.5 g pollen grains and 0.2 g tween 80, were added to the chitosan solution. The coating composite formulations were subjected to an ultrasonic bath to ensure complete removal of air bubbles before casting. The film-forming solutions were casted in Teflon casting plates. Then, the dry films were peeled off the plates and washed. The films were desiccated at (20 ± 2 °C, 50% relative humidity) for one week to be ready for analysis.
2.4. Characterization of the self-healing chitosan–beeswax-based composite coating film
2.4.1. Thickness measurements. The thicknesses of the chitosan and chitosan–beeswax-based coating films were measured in accordance with the ISO 534-2005 protocol. A thickness gauge tester (Kalfer GmbH, Germany) accurate to 0.01 mm reading was used. Five replicates were measured per sample.
2.4.2. X-ray diffraction (XRD). The prepared composite materials were assessed via an X-ray Philips diffractometer (PW 1820 goniometer, PW 1930 generator), fixed with Cu Kα radiation (45 kV, 40 mA, with λ = 0.15418 nm). The analysis was scanned in a 2θ range of 5 to 60° with a step size of 0.02 and step time of 1 s.
2.4.3. Fourier transform infrared spectroscopy (FT-IR). The structures of the prepared composites were evaluated using a Bruker Vector 33 FTIR spectrometer (Germany). The infrared spectra were recorded in absorbance units in the 4000–400 cm−1 range.
2.4.4. Scanning electron microscopy (SEM). The surface morphology investigation (SEM) of the chitosan–beeswax based coating film was carried out using an SEM (Quanta 250 FEG) attached with an EDX Unit, with accelerating voltage 30 kV., magnification 14× up to 1
000
000 and resolution for Gun.1n.
2.4.5. Moisture content and degree of swelling%. The moisture contents of the coating films were gravimetrically determined as clarified in eqn (1).38 The degree of swelling was estimated by cutting the films into rectangular shapes (1 × 4 cm2, m1), which were dried at 105 °C for 24 h to achieve the dry mass (m2) as demonstrated in eqn (2). Then, the films were soaked in distilled water at 25 °C for 24 h to obtain a constant mass (m3). Lastly, the films were dried at 105 °C for 24 h (m4). |
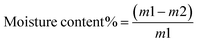 | (1) |
|
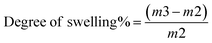 | (2) |
2.4.6. Mechanical properties. The mechanical properties (tensile strength and elongation% at break) were determined by ASTM D638-10.39 All the studied dry films were cut into strips. Five strip samples of each film type were desiccated at 33% RH for 24 hours at ambient temperature. Two replicas of the coating films were cut into rectangular shapes (40 mm × 10 mm). The samples were clamped and subjected to tension until breakage. The strip samples were measured on an Instron Universal Testing Machine at a crosshead speed of 15 mm min−1 and a 0.1 kN force load cell.40
2.4.7. Self-healing efficacies%. The ratio of healed stress or strain at the breaking point to the initial value is known as self-healing efficacy%, as illustrated in eqn (3). The coating films were cut into two identical pieces, and the film was left to heal under ambient temperature for the desired time. The fracture stress or stain of the initial and healed specimens was determined.41 |
 | (3) |
2.4.8. Barrier properties (water vapor and oxygen transmission rate). The water vapor transmission rate (WVTR) was determined using a GBI W303 (B) Water Vapor Permeability Analyzer (China) using the cup method. WVTR was measured as the mass of water vapor transmitted throughout a unit area in a unit time under controlled conditions of temperature (38 °C) and humidity (4%) according to a standard (ASTM E96). Also, the gas transmission rate (OTR) was measured by an N530 Gas Permeability Analyzer (China). According to the standard ASTM D1434-82 (2003).
2.4.9. Water contact angle (WCA). Herein, a Theta Optical Tensiometer (Model OAC, 13EC, DataPhysics Instruments GmbH, Germany) was utilized to detect the water contact angles (WCA). The single liquid dispenser (OAC 13 EC) dispensed a water drop with an exact volume of 1 μl. It was inclined until the drop came in contact with the surface of the film. Then, the automatic dispenser was raised up with the end goal of retaining the water drop at the surface. Pictures were taken by a programmed camera. Then, the images were analyzed using the DataPhysics program, known as the Young fitting method, to perform the contact angle analyses.
2.5. Treatments and storage conditions
Two hundred and forty mature fruits were selected that were free from visual defects (“undamaged, uniform in shape, free from blemishes and pathogen infection”) and were divided into 4 treatment groups. The mature picked fruits were dipped in solutions containing beeswax–pollen grain, chitosan, and chitosan–beeswax/pollen grain coatings for 5 minutes in parallel to water as a control solution. Then, the coated fruits were left to air-dry. The treatment protocol included ten replicates per treatment and each replicate per 6 fruits; every treatment group contained approximately 60 fruits (12 kg), which were packed in fiberboard cartons under storing conditions (0 ± 1 °C and 85–90% RH) for 105 days. After fixed time intervals (15 days), the fruit samples were removed and fruit quality criteria estimations were carried out.
At the end of storage period, a sample (20 fruits) of each treatment group was left at room temperature (23 ± 2 °C and 47% RH) for one week as a marketing period, and quality assessments were performed.
2.6. Fruit quality assessments
2.6.1. Physical characteristics. The weight loss% of the all coated samples were estimated periodically by weight difference. The decay% was evaluated as follows: the decayed fruit samples were counted and discarded. The decay% was calculated based on the total numbers of the fruits. To determine the firmness (lb per inch2), on each sampling, 3 pear fruits from each treatment were analyzed for firmness using an Analogue Portable Fruit Hardness Tester model FT 327 (3–27 lbs) equipped with a 8.0 mm cylindrical probe with a flat surface and height of 2 cm by measuring the penetration on both sides of the fruit of each of 3 fruits from the coated and uncoated groups in a vertical direction; then, the average was taken (6 readings per treatment).
2.6.2. Chemical characteristics. The total soluble solids percentages (TSS%) were determined using a hand refractometer. The total acidity (TA%) was estimated as the percentage of malic acid in fruit juice (AOAC, 2000).The statistical analysis was performed using the computer-based software MS Excel, and the results were analyzed by variance.42 LDS statistical analysis was used to define differences among treatment means at a significance level of 0.05.43
3. Results and discussion
3.1. The structure of the prepared chitosan–BW/PG film
FT-IR spectra of the BW, PG, CH, CH/BW and CH/BW-PG films are presented in Fig. 1. In the chitosan spectrum, a strong band at 3247 cm−1 corresponds to N–H and O–H stretching, along with intramolecular hydrogen bonds. The absorption bands at 2915 and 2848 cm−1 can be recognized as C–H symmetric and asymmetric stretching, respectively. The attendance of residual N-acetyl groups was verified by the bands near 1607 cm−1 (C
O stretching of amide I) and 1322 cm−1 (C–N stretching of amide III), separately. A band at 1536 cm−1 indexes with the N–H bending of the primary amine.44 The CH2 bending and CH3 symmetrical deformations were detected by the incidences of the bands at approximately 1416 and 1380 cm−1, respectively.45,46
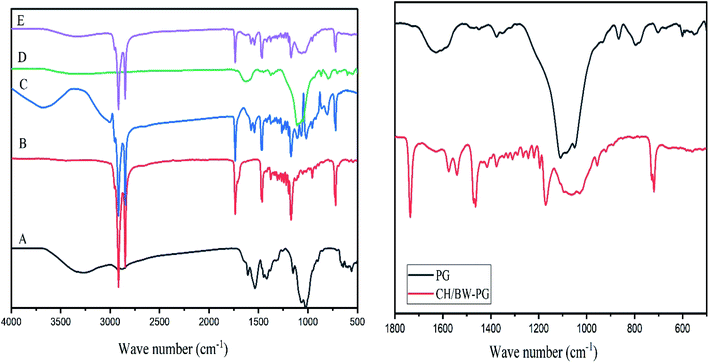 |
| Fig. 1 FT-IR spectra of (A) CH, (B) BW, (C) CH-BW, (D) PG, and (E) CH/BW-PG. | |
The beeswax spectrum displayed noticeable infrared absorption peaks at 3417, 2954, 2915, 2848, 1735, 1462, 1375, 1328, 1219, 1170, 729, and 719 cm−1. The peak at 3417 cm−1 implies the existence of the stretching vibrations of hydroxyl groups of alcohols, the peaks at 2915 and 2848 cm−1 point to the presence of fatty acid chains (caused by the stretching vibrations of C–H groups), the peak at 1735 cm−1 is caused by the stretching vibrations of the carbonyls of esters, the peaks at 2954 and 1462 cm−1 are caused by hydrocarbon vibrations, and the peaks at 1328, 1219–1170 and 729 cm−1 account for non-planar amide groups.47–49 These results are similar to those of Dinker and Agarwal.50 Intense absorption bands at 2954, 2915, 2848, 1735, 1462, 1375, 1328, 1219, 1170, 729, and 719 cm−1 appear in the FT-IR spectra of the chitosan/beeswax-based films, indicating the successful incorporation of beeswax into chitosan.
The FT-IR spectrum of the pollen grains shows an absorption band at 1628 cm−1 (amide I: C
O stretch) of proteins, which interferes with the carbonyl groups in the chitosan/BW-PG film. The presence of aromatic rings was observed at the absorption band of 1449 cm−1. In the spectral region of 1500–866 cm−1, a prominent band around the value of 1375 cm−1 (COO-stretch and CH2 and CH3 deformation) appeared, which is attributed to the presence of lipids and triglycerides. The characteristic region of 1500–866 cm−1 that occurred in the FT-IR spectrum of the chitosan/BW-PG film indicates the presence of incorporated pollen grains. The most intense band in this spectral region is at 1109 cm−1 (C–OH skeletal; C–O–C). Carbohydrate molecules can also be observed around the band of 866 cm−1 (C–O–C).51
3.2. X-Ray diffraction (XRD) pattern of the prepared chitosan–BW/PG film
XRD is an additional quick analytical practice for identification of the crystallinity of materials, and it is generally used for structural and compositional studies in material science. To support the FT-IR data and explore the crystallinity of the chitosan-based beeswax films, X-ray diffraction (XRD) analysis was executed on samples of natural beeswax, chitosan, pollen grains, and chitosan-based wax and pollen grain films. As shown in Fig. 2, the diffractogram of beeswax shows the seven most intense peaks at 2θ = 10.3° (d-spacing 8.5979 nm), 21.53° (d-spacing 4.1232 nm), 23.90° (d-spacing 3.7199 nm), 29.98° (d-spacing 2.9785 nm), 36.11° (d-spacing 2.4849 nm), 40.31° (d-spacing 2.2354 nm), and 42.97° (d-spacing 2.1029 nm), which are specific to the orthorhombic crystal structure in beeswax.52,53
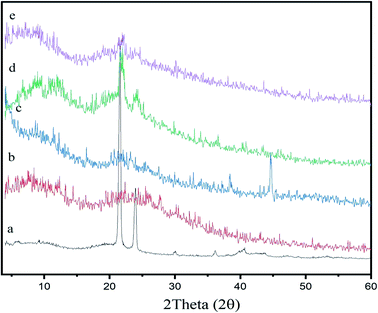 |
| Fig. 2 XRD patterns of the (a) BW, (b) chitosan, (c) PG (d) chitosan–BW, and, (e) and chitosan/BW-PG films. | |
The XRD analysis is indicative of the high purity and high crystallinity of the beeswax. As shown in Fig. 2, the diffractogram of chitosan shows two distinctive peaks appearing at 2θ = 12.13° and 22.10°. These two peaks were observed in the diffractograms of the chitosan–BW and chitosan/BW-PG films with decreased intensities, indicating good incorporation of beeswax into the chitosan films. The pollen grains show five characteristic peaks observed at 2θ = 9.0°, 21.0°, 23.0°, 38.39° and 44.62°. In the diffractograms of the chitosan/BW-PG films, detectable pollen grain peaks were observed, but with small intensities; these peaks may be overlapped with the noise of the chitosan/BW-PG film.54
3.3. SEM images of the self-healing chitosan–beeswax films
The topographical and texture characterizations of the self-healing films comprising chitosan/beeswax/pollen grains (chitosan–BW/PG) cross-linked with CaCl2·2H2O are shown in Fig. 3. The SEM images clarified that the chitosan–BW/PG film has a fairly rough surface morphology. These features of the surface morphology were probably affected by the crosslinking process, whereas the hydrogel has a fitted, rough, and dense structure, indicating good toughness and high surface area, which promotes its mechanical properties and water diffusion. The image showed uniform and homogeneous distribution of beeswax plus pollen grains with a lamellar appearance.
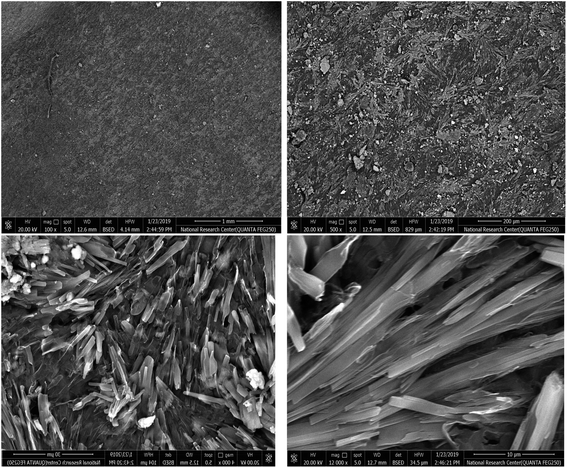 |
| Fig. 3 SEM images of the self-healing chitosan–beeswax/pollen grains film at diverse magnification scales. | |
3.4. Contact angles of the chitosan–beeswax-based film
To predict the prospective interactions of the packaging materials with food, the water contact angle criterion was measured. The water contact angles of the chitosan and chitosan–BW/PG films are presented in Fig. 4. The contact angle for the chitosan film is 79.8° because of the occurrence of hydroxyl groups in the chitosan/glycerol blend; therefore, the surface is partially hydrophobic. The contact angle value increased upon adding the beeswax and pollen grains to reach to 109.7°, which displays the enhancement of the hydrophobic character of the prepared film. Due to the surface hydrophobicity of the film, the spreading of water droplets was limited.
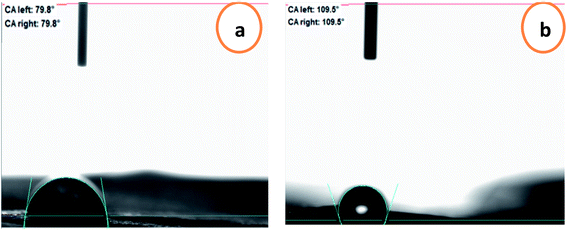 |
| Fig. 4 Contact angle values of the chitosan (a) and chitosan–BW/PG (b) films. | |
3.5. Physical and mechanical properties of the chitosan–beeswax-based composite film
Experimentally, beeswax/pollen grains have no capability to form well-matched coating films by normal casting procedures. Thus, this formulation was used in a comparative study with the chitosan and chitosan–beeswax based coating films, as shown in Table 1.
Table 1 Physical and mechanical parameters of the self-healing chitosan–beeswax composite film
Test type |
BW/PG |
Chitosan |
Chitosan–BW/PG |
Thickness, (μm) |
— |
34.00 ± 1.50 |
38.9 ± 1.60 |
Moisture content, % |
— |
26.44 ± 0.02 |
25.44 ± 0.03 |
Degree of swelling, % |
— |
2172.09 ± 4.20 |
458.81 ± 4.00 |
WVTR, (g per M2 per day) |
— |
2065.23 ± 10.80 |
1038.07 ± 5.78 |
OTR, (cm3 per M2 per day) |
— |
0.034 ± 0.004 |
0.13 ± 0.03 |
CO2, GTR (cm3 per M2 per day) |
— |
10.40 ± 0.25 |
25.85 ± 0.45 |
Tensile at break, (MPa) |
— |
0.77 ± 0.10 |
0.99 ± 0.10 |
Elongation at break, % |
— |
35.81 ± 0.30 |
14.09 ± 0.27 |
Young`s modulus, (MPa) |
— |
12.70 ± 0.20 |
22.56 ± 0.60 |
Self-healing efficiency, % |
— |
86.70 ± 11.00 |
96.30 ± 0.14 |
3.6. Moisture content studies of the prepared chitosan–beeswax-based composite coating films
The moisture content of coating films governs the plasticizing parameter of these films. This leads to increased flexibility, and the produced films can resist cracking. The moisture content% was greatly affected by the presence of beeswax in the chitosan-based composites, as shown in Table 1. In all the chitosan-based composite formulations, the plasticizing agents were water and glycerol. The coating films containing beeswax have lower moisture contents than the chitosan coating films. This behavior has been reported widely for films blended with beeswax. These results were reported by Elena Velickova et al.37 Unlike other lipids, beeswax has noticeable resistance to moisture transport.
3.7. Swelling studies of the chitosan–beeswax-based composite coating film
The swelling degrees of the self-healing chitosan and chitosan–BW/PG-based composite coating films are mostly remarkable for postharvest preservation of fresh fruits. A self-healing chitosan–BW/PG-based film was fabricated by cross-linking with calcium chloride dihydrate. Generally, cross-linking leads to more reduction of the mesh size of the coating film, limiting the amount of water diffusion into the polymer matrix and cross-links.55 The blending of beeswax and pollen grains into the chitosan formulation has a critical effect on the swelling degree% of the coating films. The chitosan formulation comprising beeswax has a reasonably lower swelling degree% than the chitosan coating formulation without beeswax. The swelling degree% of the self-healing chitosan–beeswax/PG-based film was 458.81 ± 4.0% compared with that of the chitosan coating film (2172.09 ± 4.2%), as shown in Table 1.
3.8. Permeability studies of the chitosan–beeswax based composite coating film
WVTR is a required element for appropriateness of packaging materials that are used for food packaging applications. Correspondingly, the path of water vapor (WV) from the neighboring air into food products or the loss of moisture from the foodstuff to the adjacent atmosphere meaningfully influences the stability and quality of the packaged food throughout the storage period. The barrier properties of the film contribute to giving a better indication of the applications of food packaging films, whereas the coating films are used to prevent dehydration of fruits.56 The barrier properties of the self-healing chitosan and chitosan–beeswax/PG-based composite coating films are shown in Table 1. The existence of beeswax and pollen grains appears to increase the thickness of the coating films. The increasing thickness of the films may be due to the higher solid content. The beeswax is a key parameter that deeply affects the WVTR readings of the self-healing chitosan and chitosan–beeswax/PG-based films, which resulted in a sharp decrease in WVTR values compared with the chitosan formulation without beeswax and pollen grains. The self-healing chitosan–beeswax/PG-based composites exhibited a lower WVTR value of up to 1038.07 ± 5.78 g per m2 per day compared with that of the chitosan coating film (2065.23 ± 10.8 g per m2 per day).
Moreover, the oxygen transmission rates (GTR) of the self-healing chitosan and chitosan–beeswax/PG based films were generally markedly low. This parameter is probably related to the nature of the coating film, and the permeate (oxygen gas) affects its transfer through the film.57 This is because polar molecules diffuse faster than non-polar molecules, especially through polar films. This is due to the increased solubility of polar molecules in polar films. It is clear that the self-healing chitosan–beeswax/PG-based coating film exhibited higher GTR than the chitosan film. The GTR value for the self-healing chitosan–beeswax/PG-based coating films was detected and compared to that of the chitosan coating film, reaching values as high as 0.13 ± 0.033 cm3 per M2 per day compared with chitosan (0.034 ± 0.004 cm3 per M2 per day). The addition of the highly hydrophobic beeswax as a component of the coating composite is responsible for the increasing film hydrophobicity; also, the oxygen gas diffusion increased, and thus the GTR increased. Also, Table 1 shows that the GTR of carbon dioxide (CO2) of the fabricated chitosan–beeswax/PG based composites improves upon loading beeswax/PG in the chitosan–beeswax/PG composites. This is owing to the specific structures of beeswax/PG in the prepared composite. Therefore, the permeability of CO2 of the prepared chitosan–beeswax/PG based composites was enhanced from 10.40 ± 0.25 to 25.85 (cm3 per M2 per day) compared with chitosan.
3.9. Mechanical properties and self-healing efficiency%
The incorporation of long chain hydrocarbon beeswax into self-healing chitosan-based composite films creates a considerably less flexible coating film. The reduction in film elasticity may be a result of the lower absorbed water content in the self-healing chitosan composites containing beeswax.58 The increased toughness of the films is evidenced by the increasing Young's modulus, tensile strength, and reduced elongation% at break, as demonstrated in Table 1. The chitosan–BW/PG film is a considerably less flexible film. A two-fold decrease of the elongation% at break was observed for the chitosan–BW/PG film (14.09 ± 0.266%) compared with the chitosan film (35.81 ± 0.3%). Also, the Young's modulus showed a twofold increase for the chitosan–BW/PG film (22.56 ± 0.6 MPa) compared with the chitosan film (12.7 ± 0.2 MPa). The chitosan and chitosan–beeswax coating films exhibit perfect self-healing modes; they tend to auto-heal under the ambient temperature without any external effects.
The self-healing efficiency% is presented in Table 1. The results indicated that the PEM-SH coating films have high self-healing efficiency% within the range of 83.03 ± 9.3% to 92.00 ± 1.9%. The real reasons for the auto-healing mode of the films may be dynamic supramolecular interactions that comprise electrostatic interactions and hydrogen bonding. In our work, the auto-healing aspects may be due to presence of Ca+ ions, which diffuse along the interface of the cut points and tend to re-build the supramolecular interactions at the connection points. Due to the inherently dynamic nature of the polymer, the hydrogen bonds would be re-constructed again between the two cut fragments.59
3.10. Fruit quality assessments after storage
3.10.1. Fruit physical characteristics.
3.10.1.1. Fruit weight loss percentage. The data in Fig. 5(a and b) show that the weight loss (%) of the fruits shows a slight but significant increase as the storage periods progress, ranging from 0.20 to 7.10 in the 1st season and from 0.56 to 7.97 in the 2nd season. These findings suggest that all the coated samples have a high tendency to reduce the weight loss percentage of Le Conte pear fruits compared with that of uncoated fruits under cold storage conditions. The most efficient coating treatment is BW/PG, followed by chitosan and chitosan–BW/PG, respectively, compared with the control; the trend exhibits a descending order, but there is no significant difference among them. The actual reasons for these results may be water evaporation, transpiration and dry matter by respiration. Using the edible coatings may create a thin film that surrounds the fruit peel and induces a modification of the atmosphere around the fruits. The film is semipermeable against CO2, O2, water vapor molecules and solute transport. Therefore, the water loss, oxidation and respiration rates would be reduced.60
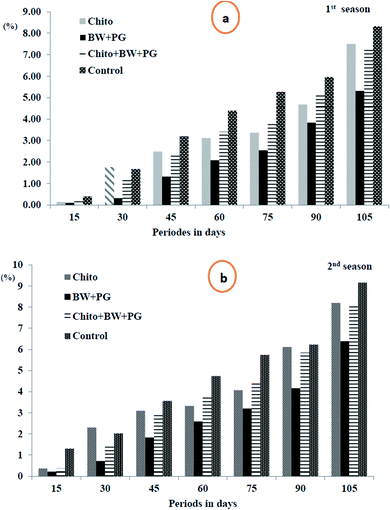 |
| Fig. 5 (a and b): Weight loss% of pear fruits coated with chitosan (Chito), beeswax/pollen grains (BW + PG), chitosan/beeswax/pollen grains (Chito + BW + PG) and the control sample (water) in the first and second seasons. | |
Comparable results were reported for previous studies on beeswax alone by Bashir and Abu-Goukh61 in guava by and El-Anany et al.62 in Anna apple, for chitosan alone by Kerch63 in several fruits and vegetables and for combined coats (beeswax + chitosan) by Asgar et al.64 on papaya fruits. The weight loss criterion is an important issue to consumers. Therefore, the use of edible coatings is considered to be an excellent option to limit weight loss.65
3.10.1.2. Fruit decay percentage. Looking at Fig. 6(a and b), it is obvious that the fruit decay% increased steadily and gradually as the storage period progressed up to 105 days at 0 ± 1 °C in a significant way in the two seasons, except that this increment was fixed up to 60 days in the first season and up to 45 days in the second season. In both seasons, after 15 days of storage, all the studied samples exhibited fruits that were healthy and free from decay, in accordance with the interaction between different treatments and storage periods. Although in the 1st season, the fruit decay% was steady up to 60 days (7.15%) in all treatment groups, it continued steady up to 75 days in both treatment groups (BW/PG) and chitosan–BW/PG, which recorded 7.15%, and in fruit coated with chitosan up to 90 days, which reached 7.15%. Meanwhile, in the second season, the fruit decay% was fixed for up to 45 days in uncoated fruits and up to 75 days in fruits coated with chitosan, which recorded 7.15%. Meanwhile, fruit coated with chitosan–BW/PG did not decay up to 75 days; however, fruits coated with BW/PG remained healthy and free of decay for up to 90 days. The highest significant% of decay was obtained at 105 days in uncoated fruits (42.90% and 50.05% sequentially in the two seasons).
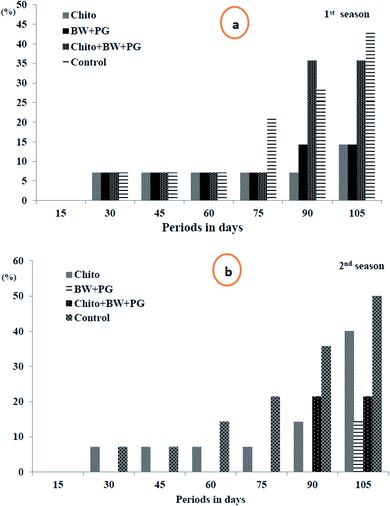 |
| Fig. 6 (a and b): Decay% of pear fruits coated with chitosan (Chito), beeswax/pollen grains (BW + PG), chitosan/beeswax/pollen grains (Chito + BW + PG), and the control sample (water) in the first and second seasons. | |
Regarding the effect of treatments on decay%, the lowest values of decay were obtained from fruits coated with chitosan, which recorded (7.15%), followed by fruits coated by BW/PG (8.17), then chitosan–BW/PG-coated fruits (14.3%) in the 1st season. Otherwise, lower decay% were revealed from fruits coated with BW/PG (2.04%), chitosan–BW/PG (6.13%) and chitosan (11.85%), respectively, in the 2nd season. The highest decay% was shown by the uncoated fruits (16.34% and 19.41%) consecutively in both seasons, and no significant differences were detected between all treatments in the two seasons. The reduction in fruit decay% by the application of an edible coating was due to the partial restriction of gas exchange through the peel; also, later, ethylene gas generation was inhibited. Due to this inhibitory mechanism, the postharvest fruits are more resistant to decay. The water loss may cause minor wounds on the peel, and in that way, the decay% will be reduced. Owing to the off-flavor which results from fruit rotting,66 in other studies, chitosan has been used extensively as an inhibiting elicitor for postharvest senescence and diseases in many fruits, such as pear, citrus, kiwifruit, apple peach, strawberry and sweet cherry.63 These results were in agreement with Ahmed et al.;67 they mentioned that treatment with chitosan has the potential to inhibit decay and hence prolong the cold storage life of navel orange fruits.
3.10.1.3. Fruit firmness (lb per inch2). Fruit firmness is one of the most important physical considerations to evaluate the progress of ripening. Fruit firmness is an indicator of ripeness degree because it is recognized as the force required to break the flesh tissues. It is associated with various ripening stages. The data in Fig. 7(a and b) display clearly that the flesh firmness of pear fruits significantly decreased with advancing storage period for all treatments during cold storage at 0 ± 1 °C, and the minimum was revealed at up to 105 days, which was recorded as 11.73 and 11.34 lb per inch2, respectively, in both seasons. Coated pear fruits showed higher effects in reducing the rate of fruit softening. The combined coated fruits with chitosan–BW/PG achieved low softness after up to 105 days of cold storage (13.25 and 12.75 lb per inch2), followed in the same period by BW + PG-coated fruit (11.75 and 12.10 lb per inch2), sequentially, in the two seasons. Chitosan-coated fruit came next in this respect. The control fruits showed more significant softness than those with the other treatments.
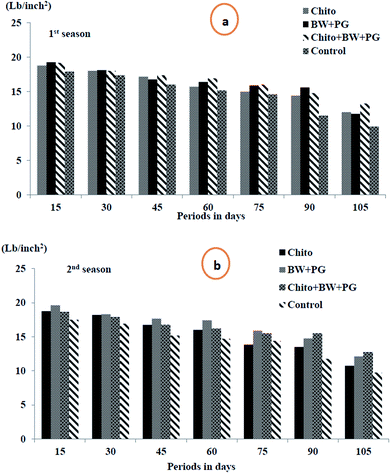 |
| Fig. 7 (a and b): Firmness (lb per inch2) of pear fruits coated with chitosan (Chito), beeswax/pollen grains (BW + PG), chitosan/beeswax/pollen grains (Chito + BW + PG) and control sample (water) in the first and second seasons. | |
Pears ripened to a soft melting texture are considered ‘ready to eat’. As with other fruit species, the cell wall extracted from pear fruit showed considerable increase in swelling and high pectin solubilization during ripening. This phenomenon can be explained by previous research that indicated that disassembly of the primary cell wall and middle lamella structures of fruit flesh could contribute to changes in fruit texture during storage. Firmness during ripening in climacteric fruit, such as pears, is generally attributed to degradation of the cell wall and loss of turgor pressure in the cells reduced by water loss.68 The reduction in fruit softness by application of an edible coating (wax and/or chitosan coating alone or combined) may be due to the inhibition of water loss and the activities of pectin-degrading enzymes closely related to fruit softening by reducing the rate of metabolic processes during senescence. These results are similar to those of other authors for the preservation of different varieties of pears, such as “Flor de Invierno”,69 “Huanghua”,70 “d’Anjou”71 and “Le Conte”.66
3.10.2. Physical–chemical characteristics of the fruit.
3.10.2.1. Total soluble solids percentage (TSS%). The results in Fig. 8(a and b) indicate that the TSS% increased significantly throughout the progress of the storage periods at 0 ± 1 °C, and the maximum was obtained for up to 105 days by all treatments in the two seasons. All the coated fruits showed positive increases in TSS% when compared with uncoated fruits. The best treatment in this regard was fruit coated with chitosan–BW/PG, which was more effective statistically in increasing TSS% in both seasons. This was followed by chitosan-coated fruit in the 1st season, in contrast with BW/PG-coated fruit in the 2nd season. The lowest significant levels of TSS% were detected for the uncoated fruits in both seasons.
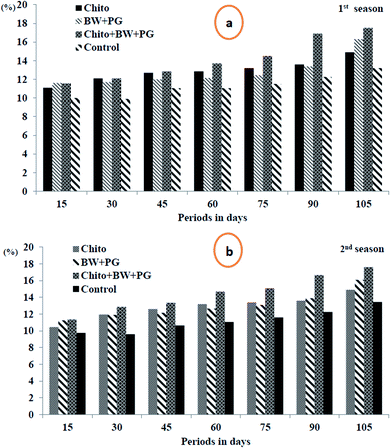 |
| Fig. 8 (a and b): TSS% of pear fruits coated with chitosan (Chito), beeswax/pollen grains (BW + PG), chitosan/beeswax/pollen grains (Chito + BW + PG) and the control sample (water) in the first and second seasons. | |
The increase in total soluble solids in fruits is directly correlated to the hydrolytic activities of starch, the increased activity of enzymes responsible for the hydrolysis of starch to soluble sugars, and the conversion of starch to sugar, which indicates that the fruits are in the ripening process.66 Similar findings were observed in some fruits treated with edible coatings, such as those reported previously for Raafat et al.25
3.10.2.2. Total acidity percentage (TA%). The results in Fig. 9 demonstrate that there was a gradual decline in the fruit juice TA% of Le Conte pear with advancing storage period in cold storage up to 75 days, followed by a slight increase up to 105 days, in the two seasons. Fruits coated with chitosan–BW/PG stored for 15 days scored the highest values of this parameter compared with the other different storage periods in both seasons. In general, all pear fruits, coated or uncoated, gave values of TA% with no significant differences among them in both seasons. Fruit acidity is another important factor affecting consumer acceptance. The main acid present in pear is malic acid, and its level decreases during ripening. As the ripening of the fruits develops, a reduction in titratable acidity is observed. The decrease in acid content is caused by the use of acids in the fruit as a source of energy and the conversion of organic acids to form sugar.72
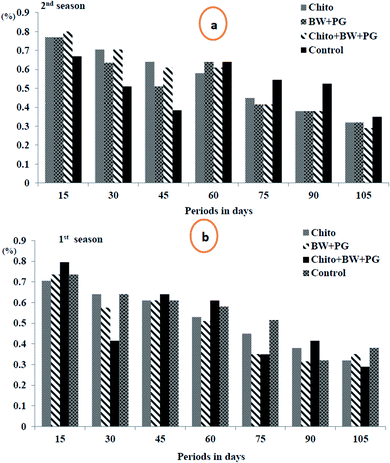 |
| Fig. 9 (a and b): TA% of pear fruits coated with chitosan (Chito), beeswax/pollen grains (BW + PG), chitosan/beeswax/pollen grains (Chito + BW + PG) and the control sample (water) in the first and second seasons. | |
The reduction in TA% of the coated fruits may be due to a decrease in the respiration rate, while the increasing TA% at the end of the storage periods may be due to absorption of amino acids and their transformation to organic acids throughout metabolic activities, especially respiration and transpiration. Our results are in harmony with those obtained by Kou et al.73
3.11. Fruit quality assessments after shelf life (marketing period)
The data in Table 2 demonstrate that all test treatments in this study showed approximately the same trend of the influence on the physical and chemical characteristics of Le Conte fruits throughout cold storage (0 ± 1 °C) for 105 days + 7 days at room temperature (23 ± 2 °C) as a simulated marketing period (shelf-life), and this was confirmed in both seasons. Moreover, all edible coatings were more effective in improving Le Conte fruit quality when compared with uncoated fruits. The Chitosan–BW/PG-coated fruits were more pronounced in reducing their percentages of weight loss (0.80% and 3.13%) and decay (0.0% and 10.0%) as well as progress in increasing firmness (11.75 and 12.35 lb per inch2) and TSS% (11.25% and 11.65%) in the 1st and the 2nd seasons, respectively.
Table 2 Effects of BW/PG, chitosan and chitosan–BW/PG on quality assessments of Le Conte pear fruits stored at 0 ± 1 °C for 105 days + one week at 23 ± 2 °C during the 2018 and 2019 seasons
Treatments |
Physical characteristics |
Weight loss (%) |
Decay (%) |
Firmness (lb per inch2) |
1st season |
2nd season |
1st season |
2nd season |
1st season |
2nd season |
Chitosan |
2.03 |
3.66 |
10.00 |
20.00 |
10.75 |
11.00 |
BWPG |
1.81 |
3.19 |
10.00 |
20.00 |
11.10 |
11.75 |
Chitosan–BW/PG |
0.80 |
3.13 |
00.00 |
10.00 |
11.75 |
12.35 |
Control (water) |
3.89 |
6.51 |
40.00 |
40.00 |
8.50 |
8.50 |
LSD0.05 |
0.14 |
0.1 |
10.90 |
7.80 |
0.13 |
0.83 |
Treatments |
Chemical characteristics |
Total soluble solids (%) |
Total acidity (%) |
1st season |
2nd season |
1st season |
2nd season |
Chitosan |
11.10 |
11.20 |
0.25 |
0.25 |
BW/PG |
11.20 |
11.45 |
0.32 |
0.32 |
Chitosan–BW/PG |
11.25 |
11.65 |
0.37 |
0.37 |
Control (water) |
9.10 |
9.60 |
0.32 |
0.32 |
LSD0.05 |
0.50 |
0.39 |
0.03 |
0.07 |
The fruits coated only with BW/PG and chitosan came next in this regard. Concerning the effects of these coating treatments on TA%, the fruits coated with combined chitosan–BW/PG (0.37% and 0.37%) came after those coated with chitosan alone (0.25% and 0.25%), BW/PG (0.32 and 0.32) and the control (0.32% and 0.32%), respectively, with little change. In contrast, the control treatment obtained the highest significant values of weight loss (3.89% and 6.51%) and decay (40.0% and 40.0%) and also recorded the lowest significant values of firmness (8.50 and 8.50 lb per inch2) and total soluble solids (9.10% and 9.60%), sequentially, in both seasons.
The above results are in line with findings by Virgilio et al.66 that showed that the application of an edible coating (wax) will partially restrict gas exchange through the fruit peel and inhibit the action of ethylene. This inhibitory action can provide better protection against postharvest decay in fruits. In addition, the water loss may cure minor wounds on the peel of the fruits and thereby reduce fruit rot, which results in the development of off-flavour. The influence on the respiration rate and ethylene by the coatings is specific to each fruit and variety. Thus, theses parameters are important when coating and packaging are studied postharvest.
Coating is therefore necessary for storage and marketing. Also, Yage et al.74 indicated that a chitosan coating is beneficial to maintain the storage quality and prolong the shelf life of postharvest fruits; it is always used as the carrier film for antimicrobial agents. Moreover, Ahmed et al.67 demonstrated that treatment with chitosan prolongs postharvest shelf life during shipping and marketing and is also safe for human health and the environment. Fig. 10 displays that the chitosan–BW/PG-coated fruit showed the best parameter values to preserve the fruits (better appearance, bright, attractive, less weight loss and decay, high firmness and TSS); thus, it is the best treatment to improve the quality and prolong the shelf life and marketing period of Le Conte pears. The previous results are in harmony with the findings of Gol et al.,75 Kerch,63 Virgilio et al.66 and Nair et al.76
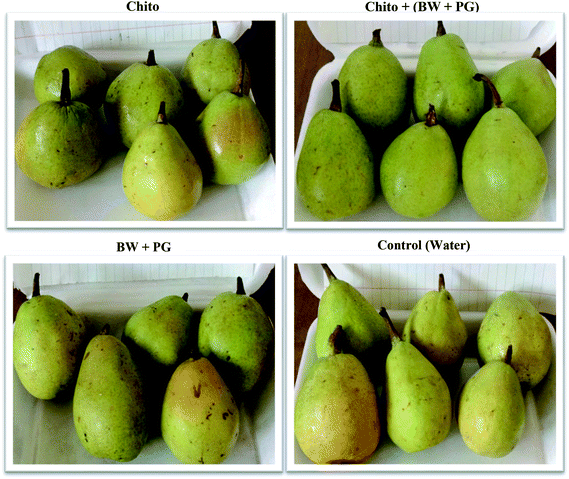 |
| Fig. 10 Effects of BW/PG, chitosan and chitosan–BW/PG on the appearance of Le Conte pear fruits stored at 0 ± 1 °C for 105 days + one week at 23 ± 2 °C as a simulated marketing period during the 2018 and 2019 seasons. | |
4. Conclusions
Smart biodegradable and edible coatings may be good alternative candidates for postharvest preservation of fruits. Opportunely, inherently auto-healing edible coatings can autonomously re-build their integral networks. Our observations in this study demonstrated that coating fruit with combined chitosan–BW/PG was the best treatment to maintain the quality of Le Conte pears during storage life and marketing periods. Thus, it is considered to be a safe and effective method as well as an environmentally friendly technology that is safe for human health.
Conflicts of interest
There are no conflicts to declare.
Acknowledgements
The authors are highly grateful to Dr/Mamdouh Mohamed Nageib professors of Pomology, National Research Center (NRC) for providing us his private farm located in Kalubia Governorate, Egypt to achieve this study.
References
- B. J. Blaiszik, S. L. Kramer, S. C. Olugebefola, J. S. Moore, N. R. Sottos and S. R. White, Self-healing polymers and composites, Annu. Rev. Mater. Res., 2010, 40, 179–211 CrossRef CAS.
- B. K. Ahn, D. W. Lee, J. N. Israelachvili and J. H. Waite, Surface-initiated self-healing of polymers in aqueous media, Nat. Mater., 2014, 13(9), 867 CrossRef CAS PubMed.
- N. A. Al-Tayyar, A. M. Youssef and R. R. Al-Hindi, Antimicrobial packaging efficiency of ZnO-SiO2 nanocomposites infused into PVA/CS film for enhancing the shelf life of food products, Food Packaging and Shelf Life, 2020, 25, 100523 CrossRef.
- Y. Yang and M. W. Urban, Self-healing polymeric materials, Chem. Soc. Rev., 2013, 42(17), 7446–7467 RSC.
- O. R. Cromwell, J. Chung and Z. Guan, Malleable and self-healing covalent polymer networks through tunable dynamic boronic ester bonds, J. Am. Chem. Soc., 2015, 137(20), 6492–6495 CrossRef CAS PubMed.
- N. Kuhl, S. Bode, R. K. Bose, J. Vitz, A. Seifert, S. Hoeppener, S. J. Garcia, S. Spange, S. van der Zwaag, M. D. Hager and U. S. Schubert, Acylhydrazones as reversible covalent crosslinkers for self-healing polymers, Adv. Funct. Mater., 2015, 25(22), 3295–3301 CrossRef CAS.
- H. Chen, X. Ma, S. Wu and H. Tian, A rapidly self-healing supramolecular polymer hydrogel with photostimulated room-temperature phosphorescence responsiveness, Angew. Chem., Int. Ed., 2014, 53(51), 14149–14152 CrossRef CAS PubMed.
- H. S. Kang, H. T. Kim, J. K. Park and S. Lee, Light-Powered Healing of a Wearable Electrical Conductor, Adv. Funct. Mater., 2014, 24(46), 7273–7283 CrossRef CAS.
- B. Huang, Y. Wang, S. Zhan and J. Ye, One-step electrochemical deposition of Schiff base cobalt complex as effective water oxidation catalyst, Appl. Surf. Sci., 2017, 396, 121–128 CrossRef CAS.
- Y. Zhao, Y. Wu, L. Wang, M. Zhang, X. Chen, M. Liu, J. Fan, J. Liu, F. Zhou and Z. Wang, Bio-inspired reversible underwater adhesive, Nat. Commun., 2017, 8(1), 1–8 CrossRef CAS.
- K. K. Oehlenschlaeger, J. O. Mueller, J. Brandt, S. Hilf, A. Lederer, M. Wilhelm, R. Graf, M. L. Coote, F. G. Schmidt and C. Barner-Kowollik, Adaptable hetero Diels–Alder networks for fast self-healing under mild conditions, Adv. Mater., 2014, 26(21), 3561–3566 CrossRef CAS PubMed.
- T. P. Russell, Surface-responsive materials, Science, 2002, 297(5583), 964–967 CrossRef CAS PubMed.
- X. J. Ye, J. L. Zhang, Y. Zhu, M. Z. Rong, M. Q. Zhang, Y. X. Song and H. X. Zhang, Ultrafast self-healing of polymer toward strength restoration, ACS Appl. Mater. Interfaces, 2014, 6(5), 3661–3670 CrossRef CAS PubMed.
- F. Ahangaran, M. Hayaty and A. H. Navarchian, Morphological study of polymethyl methacrylate microcapsules filled with self-healing agents, Appl. Surf. Sci., 2017, 399, 721–731 CrossRef CAS.
- L. F. Fan, M. Z. Rong, M. Q. Zhang and X. D. Chen, Repeated intrinsic self-healing of wider cracks in polymer via dynamic reversible covalent bonding molecularly combined with a two-way shape memory effect, ACS Appl. Mater. Interfaces, 2018, 10(44), 38538–38546 CrossRef CAS PubMed.
- M. Omaima, H. Hamouda and M. A. Abd-El-Mageed, Effect of calcium and some antioxidants treatments on storability of Le Conte pear fruits and its volatile components, Science, 2010, 8(5), 109–126 Search PubMed.
- Y. Xing, Q. Xu, X. Li, C. Chen, L. Ma, S. Li, Z. Che and H. Lin, Chitosan-based coating with antimicrobial agents: preparation, property, mechanism, and application effectiveness on fruits and vegetables, Int. J. Polym. Sci., 2016, 4851730, DOI:10.1155/2016/4851730.
- Y. Xing, Q. Xu, L. Jiang, D. Cao, H. Lin, Z. Che, Y. Ma, X. Li and Y. Cai, Effect of different coating materials on the biological characteristics and stability of microencapsulated Lactobacillus acidophilus, RSC Adv., 2015, 5(29), 22825–22837 RSC.
- C. M. Oliveira, A. Alpalhão, C. Neto, L. Goulão, J. Clemente, J. Henriques and I. Lourenço, Efeito do 1-metilciclopropeno em pera'Rocha'em diferentes estados de maturação armazenadas em atmosfera normal, Simpósio Nacional de Fruticultura, 2006, 1, 232–238 Search PubMed.
- D. Jianglian and Z. Shaoying, Application of chitosan based coating in fruit and vegetable preservation: a review, J. Food Process. Technol., 2013, 4(5), 227 CrossRef.
- Y. Yu and Y. Ren, Effect of chitosan coating on preserving character of post-harvest fruit and vegetable: a review, J. Food Process. Technol., 2013, 4(8) Search PubMed.
- X. Meng, J. Han, Q. Wang and S. Tian, Changes in physiology and quality of peach fruits treated by methyl jasmonate under low temperature stress, Food Chem., 2009, 114(3), 1028–1035 CrossRef CAS.
- J. F. Thompson, Pre-cooling and storage facilities, The Commercial Storage of Fruits, Vegetables, and Florist and Nursery Stocks, 2004, vol. 11 Search PubMed.
- M. A. Saleh, N. S. Zaied, M. A. Maksoud and O. M. Hafez, Application of Arabic gum and essential oils as the postharvest treatments of Le Conte pear fruits during cold storage, Asian Journal of Agricultural and Horticultural Research, 2019, 1–11 CrossRef.
- D. Raafat, K. Von Bargen, A. Haas and H. G. Sahl, Insights into the mode of action of chitosan as an antibacterial compound, Appl. Environ. Microbiol., 2008, 74(12), 3764–3773 CrossRef CAS PubMed.
- H. Arnon, Y. Zaitsev, R. Porat and E. Poverenov, Effects of carboxymethyl cellulose and chitosan bilayer edible coating on postharvest quality of citrus fruit, Postharvest Biol. Technol., 2014, 87, 21–26 CrossRef CAS.
- G. R. Pl&acido, R. M. da Silva, C. Cagnin, M. D. Cavalcante, M. A. da Silva, M. A. Caliari, M. S. de Lima and L. E. do Nascimento, Effect of chitosan-based coating on postharvest quality of tangerines (Citrus deliciosa Tenore): Identification of physical, chemical, and kinetic parameters during storage, Afr. J. Agric. Res., 2016, 11(24), 2185–2192 Search PubMed.
- O. M. Sharaf, M. S. Al-Gamal, G. A. Ibrahim, N. M. Dabiza, S. S. Salem, M. F. El-Ssayad and A. M. Youssef, Evaluation and characterization of some protective culture metabolites in free and nano-chitosan-loaded forms against common contaminants of Egyptian cheese, Carbohydr. Polym., 2019, 223, 115094 CrossRef CAS PubMed.
- A. M. Youssef, M. S. Hasanin, M. E. Abd El-Aziz and G. M. Turky, Conducting chitosan/hydroxylethyl cellulose/polyaniline bionanocomposites hydrogel based on graphene oxide doped with Ag-NPs, Int. J. Biol. Macromol., 2021, 167, 1435–1444 CrossRef CAS PubMed.
- H. S. El-Sayed, S. M. El-Sayed, A. M. Mabrouk, G. A. Nawwar and A. M. Youssef, Development of Eco-friendly Probiotic Edible Coatings Based on Chitosan, Alginate and Carboxymethyl Cellulose for Improving the Shelf Life of UF Soft Cheese, J. Polym. Environ., 2021, 1–13, DOI:10.1007/s10924-020-02003-3.
- N. A. Al-Tayyar, A. M. Youssef and R. R. Al-Hindi, Edible coatings and antimicrobial nanoemulsions for enhancing shelf life and reducing foodborne pathogens of fruits and vegetables: a review, Sustainable Mater. Technol., 2020a, 26, e00215 Search PubMed.
- M. Hesham, S. M. El-Sayed and A. M. Youssef, Synergistic impact of cumin essential oil on enhancing of UV-blocking and antibacterial activity of biodegradable poly (butylene adipate-co-terephthalate)/clay platelets nanocomposites, J. Thermoplast. Compos. Mater., 2021 DOI:10.1177/0892705721989771.
- H. F. Youssef, M. E. El-Naggar, F. K. Fouda and A. M. Youssef, Antimicrobial packaging film based on biodegradable CMC/PVA-zeolite doped with noble metal cations, Food Packaging and Shelf Life, 2019, 22, 100378 CrossRef.
- H. A. Mesbah, N. A. El-Sayed, N. K. Hassona, K. Abdel-Hameed and H. A. Abdel-Sattar, The common types of pollen grains collected by honey bee workers Apis mellifera, L.(Hymenoptera: Apidae) in El-Sabheia region, Alexandria Governorate, Egypt, Alexandria Sci. Exch. J., 2017, 38(October-December), 913–920 CrossRef.
- R. Kaur and N. R. Kumar, Pollen foraging activity of Apis mellifera during autumn season in Chandigarh, Halteres, 2013, 4, 12–14 Search PubMed.
- S. Ghayempour, M. Montazer and M. M. Rad, Tragacanth gum as a natural polymeric wall for producing antimicrobial nanocapsules loaded with plant extract, Int. J. Biol. Macromol., 2015, 81, 514–520 CrossRef CAS PubMed.
- E. Velickova, E. Winkelhausen, S. Kuzmanova, V. D. Alves and M. Moldão-Martins, Impact of chitosan-beeswax edible coatings on the quality of fresh strawberries (Fragaria ananassa cv Camarosa) under commercial storage conditions, LWT–Food Sci. Technol., 2013, 52(2), 80–92 CrossRef CAS.
- D. C. González Sandoval, B. Luna Sosa, G. C. Martínez-Ávila, H. Rodríguez Fuentes, V. H. Avendaño Abarca and R. Rojas, Formulation and characterization of edible films based on organic mucilage from Mexican Opuntia ficus-indica, Coatings, 2019, 9(8), 506 CrossRef.
- C. R. Raajeshkrishna and P. Chandramohan, Effect of reinforcements and processing method on mechanical properties of glass and basalt epoxy composites, SN Appl. Sci., 2020, 2(5), 1–6 Search PubMed.
- C. M. Müller, J. B. Laurindo and F. Yamashita, Effect of nanoclay incorporation method on mechanical and water vapor barrier properties of starch-based films, Ind. Crops Prod., 2011, 33(3), 605–610 CrossRef.
- Y. M. Chen, L. Sun, S. A. Yang, L. Shi, W. J. Zheng, Z. Wei and C. Hu, Self-healing and photoluminescent carboxymethyl cellulose-based hydrogels, Eur. Polym. J., 2017, 94, 501–510 CrossRef CAS.
- G. W. Snedecor and G. William, Statistical methods, 1989 Search PubMed.
- R. A. Waller and D. B. Duncan, A Bayes rule for the symmetric multiple comparisons problem, J. Am. Stat. Assoc., 1969, 64(328), 1484–1503 Search PubMed.
- S. H. Lim and S. M. Hudson, Synthesis and antimicrobial activity of a water-soluble chitosan derivative with a fiber-reactive group, Carbohydrate research, 2004, 339(2), 313–319 CrossRef CAS PubMed; S. H. Lim and S. M. Hudson, Synthesis and antimicrobial activity of a water-soluble chitosan derivative with a fiber-reactive group, Carbohydrate research, 2004, 339(2), 313–319 CrossRef PubMed.
- A. B. Vino, P. Ramasamy, V. Shanmugam and A. Shanmugam, Extraction, characterization and in vitro antioxidative potential of chitosan and sulfated chitosan from Cuttlebone of Sepia aculeata Orbigny, 1848, Asian Pac. J. Trop. Biomed., 2012, 2(1), S334–S341 CrossRef.
- M. Fernandes Queiroz and K. R. T. Melo, et al., Does the use of chitosan contribute to oxalate kidney stone formation?, Mar. Drugs, 2015, 13(1), 141–158 CrossRef PubMed.
- B. Zimnicka and A. Hacura, An Investigation of molecular structure and dynamics of crude beeswax by Vibrational Spectroscopy, Pol. J. Environ. Stud., 2006, 15(4), 112–114 Search PubMed.
- J. Baeten and K. Romanus, et al., Application of a multi-analytical toolset to a 16th century ointment: identification as lead plaster mixed with beeswax, Microchem. J., 2010, 95(2), 227–234 CrossRef CAS.
- A. Rodríguez, K. Eremin, N. Khandekar, J. Stenger, R. Newman, F. Bazeta and M. T. Escohotado, Characterization of calcium sulfate grounds and fillings of applied tin-relief brocades by Raman spectroscopy, Fourier transform infrared spectroscopy, and scanning electron microscopy, J. Raman Spectrosc., 2010, 41(11), 1517–1524 CrossRef.
- A. Dinker, M. Agarwal and G. D. Agarwal, Preparation, characterization, and performance study of beeswax/expanded graphite composite as thermal storage material, Exp. Heat Transfer, 2017, 30(2), 139–150 CrossRef CAS.
- E. Buta and M. Cantor, et al., FT-IR characterization of pollen biochemistry, viability, and germination capacity in Saintpaulia H. Wendl. Genotypes, J. Spectrosc., 2015, 2015 Search PubMed.
- N. M. Ranjha, H. Khan and S. Naseem, Encapsulation and characterization of controlled release flurbiprofen loaded microspheres using beeswax as an encapsulating agent, J. Mater. Sci.: Mater. Med., 2010, 21(5), 1621–1630 CrossRef CAS PubMed.
- W. Luo, T. Li, C. Wang and F. Huang, Discovery of Beeswax as binding agent on a 6th-century BC Chinese Turquoise-inlaid Bronze sword, J. Archaeol. Sci., 2012, 39(5), 1227–1237 CrossRef CAS.
- J. Ji, S. Hao, D. Wu, R. Huang and Y. Xu, Preparation, characterization and in vitro release of chitosan nanoparticles loaded with gentamicin and salicylic acid, Carbohydr. Polym., 2011, 85(4), 803–808 CrossRef CAS.
- T. Canal and N. A. Peppas, Correlation between mesh size and equilibrium degree of swelling of polymeric networks, J. Biomed. Mater. Res., 1989, 23(10), 1183–1193 CrossRef CAS PubMed.
- S. M. El-Sayed, H. S. El-Sayed, O. A. Ibrahim and A. M. Youssef, Rational design of chitosan/guar gum/zinc oxide bionanocomposites based on Roselle calyx extract for Ras cheese coating, Carbohydr. Polym., 2020, 239, 116234 CrossRef CAS PubMed.
- M. R. De Moura, R. J. Avena-Bustillos, T. H. McHugh, D. F. Wood, C. G. Otoni and L. H. Mattoso, Miniaturization of cellulose fibers and effect of addition on the mechanical and barrier properties of hydroxypropyl methylcellulose films, J. Food Eng., 2011, 104(1), 154–160 CrossRef CAS.
- C. G. Otoni, R. J. Avena-Bustillos, C. W. Olsen, C. Bilbao-Sáinz and T. H. McHugh, Mechanical and water barrier properties of isolated soy protein composite edible films as affected by carvacrol and cinnamaldehyde micro and nanoemulsions, Food Hydrocolloids, 2016, 57, 72–79 CrossRef CAS.
- O. M. Hafez, Enhancement yield and fruit quality of Washington Navel orange by application of spraying potassium microencapsulated biodegradable polylactic acid, Agricultural Engineering International: CIGR Journal, 2018, 19(5), 101–110 Search PubMed.
- D. Martínez-Romero, N. Alburquerque, J. M. Valverde, F. Guillén, S. Castillo, D. Valero and M. Serrano, Postharvest sweet cherry quality and safety maintenance by Aloe vera treatment: a new edible coating, Postharvest Biol. Technol., 2006, 39(1), 93–100 CrossRef.
- H. A. Bashir and A.-B. A. Abu-Goukh, Compositional changes during guava fruit ripening, Food Chem., 2003, 80(4), 557–563 CrossRef CAS.
- A. M. El-Anany, G. F. Hassan and F. R. Ali, Effects of edible coatings on the shelf-life and quality of Anna apple (Malus domestica Borkh) during cold storage, Journal of Food Technology, 2009, 7(1), 5–11 CAS.
- G. Kerch, Chitosan films and coatings prevent losses of fresh fruit nutritional quality: A review, Trends Food Sci. Technol., 2015, 46(2), 159–166 CrossRef CAS.
- A. Ali, M. T. Muhammad, K. Sijam and Y. Siddiqui, Effect of chitosan coatings on the physicochemical characteristics of Eksotika II papaya (Carica papaya L.) fruit during cold storage, Food Chemistry, 2011, 124(2), 620–626 CrossRef CAS.
- G. Gonzales-Aguilar, I. Monroy-Garcinia, F. Goycoolea-Valencia, M. Diaz-Cinco and J. Ayala-Zavala, in Covers edible chitosan. An alternative to prevent microbial spoilage and preserve quality of fresh-cut papaya, Proceedings of the Symposium on “New Technologies for Preservation and Packaging of Fruits and Vegetables Fresh Cut Vegetables, 2005, pp. 121–133 Search PubMed.
- V. Cruz, R. Rojas, S. Saucedo-Pompa, D. G. Martínez, A. F. Aguilera-Carbó, O. B. Alvarez, R. Rodríguez, J. Ruiz and C. N. Aguilar, Improvement of shelf life and sensory quality of pears using a specialized edible coating, Journal of Chemistry, 2015, 2015 Search PubMed.
- A. H. H. Ahmed, M. R. A.-E. Nesiem, H. A. Allam and A. F. El-Wakil, Effect of pre-harvest chitosan foliar application on growth, yield and chemical composition of Washington navel orange trees grown in two different regions, Afr. J. Biochem. Res., 2016, 10(7), 59–69 CrossRef CAS.
- R. J. Redgwell, M. Fischer, E. Kendal and E. A. MacRae, Galactose loss and fruit ripening: high-molecular-weight arabinogalactans in the pectic polysaccharides of fruit cell walls, Planta, 1997, 203(2), 174–181 CrossRef.
- G. Oms-Oliu, R. Soliva-Fortuny and O. Martín-Belloso, Edible coatings with antibrowning agents to maintain sensory quality and antioxidant properties of fresh-cut pears, Postharvest Biol. Technol., 2008, 50(1), 87–94 CrossRef CAS.
- R. Zhou, Y. Li, L. Yan and J. Xie, Effect of edible coatings on enzymes, cell-membrane integrity, and cell-wall constituents in relation to brittleness and firmness of Huanghua pears (Pyrus pyrifolia Nakai, cv. Huanghua) during storage, Food Chem., 2011, 124(2), 569–575 CrossRef CAS.
- Z. Xiao, Y. Luo, Y. Luo and Q. Wang, Combined effects of sodium chlorite dip treatment and chitosan coatings on the quality of fresh-cut d'Anjou pears, Postharvest Biol. Technol., 2011, 62(3), 319–326 CrossRef CAS.
- R. Wills, B. McGlasson, D. Graham and D. Joyce, An introduction to the physiology and handling of fruit, vegetables and ornamentals, UNSW Press, Desiccation trial CATIE: the seeds were desiccated to, 1998, vol. 26, ch. 21.3, pp. 10–15 Search PubMed.
- X. H. Kou, W. L. Guo, R. Z. Guo, X. Y. Li and Z. H. Xue, Effects of chitosan, calcium chloride, and pullulan coating treatments on antioxidant activity in pear cv.“Huang guan” during storage, Food Bioprocess Technol., 2014, 7(3), 671–681 CrossRef CAS.
- Y. Xing, Q. Xu, X. Li, C. Chen, L. Ma, S. Li, Z. Che and H. Lin, Chitosan-based coating with antimicrobial agents: preparation, property, mechanism, and application effectiveness on fruits and vegetables, Int. J. Polym. Sci., 2016, 2016 Search PubMed.
- N. B. Gol, P. R. Patel and T. R. Rao, Improvement of quality and shelf-life of strawberries with edible coatings enriched with chitosan, Postharvest Biol. Technol., 2013, 85, 185–195 CrossRef CAS.
- M. S. Nair, A. Saxena and C. Kaur, Characterization and antifungal activity of pomegranate peel extract and its use in polysaccharide-based edible coatings to extend the shelf-life of capsicum (Capsicum annuum L.), Food Bioprocess Technol., 2018, 11(7), 1317–1327 CrossRef CAS; M. Nakahata, Y. Takashima, H. Yamaguchi and A. Harada, Redox-responsive self-healing materials formed from host–guest polymers, Nat. Commun., 2011, 2, 511 CrossRef PubMed.
|
This journal is © The Royal Society of Chemistry 2021 |