DOI:
10.1039/D0RA10576G
(Paper)
RSC Adv., 2021,
11, 5724-5728
I2/DMSO-catalyzed one-pot approach for the synthesis of 1,3,4-selenadiazoles†
Received
16th December 2020
, Accepted 23rd January 2021
First published on 2nd February 2021
Abstract
A three-component cascade reaction for the synthesis of 1,3,4-selenadiazoles and their derivatives from arylaldehydes, hydrazine, and elemental selenium by using molecular iodine is reported. This strategy is operationally simple, well-suited to a wide range of functional groups, and provides the desired products in moderate to excellent yields. The proposed mechanism predicts that the reaction tolerated a radical process.
Introduction
Selenium, is an element which plays a crucial role in biology and technology. It belongs to the chalcogen family, and is an essential element for living organisms as a component of selenomethionine, an amino acid occurring in the active centres of some enzymes, for example, glutathione peroxidase. Due to these significant properties, selenium-containing compounds such as biologically active molecules have been attractive to researchers. Organic and inorganic compounds of selenium also show unique electronic properties and are used as semi- and superconductors in electro-optic devices and sensors. The first organoselenium compound diethyl selenide was synthesized in 1836.1 1,3,4-Selenadiazoles and their derivatives are closely related to the 1,3,4-thiadiazole compounds but their properties are quite different from the 1,3,4-thiadiazole compounds. 1,3,4-Selenadiazoles and their derivatives are applied widely in pharmaceutical, agricultural, and materials chemistry. 1,3,4-Selenadiazoles are important heterocycles with an “N–C–Se” linkage which can work as the active center, chelate certain metal ions in vivo, and show good tissue permeability. The lower toxicity and in vivo stability of the selenadiazole nucleus is attributed to its aromaticity. In particular, the 1,3,4-selenadiazoles have displayed a broad spectrum of biological activities including antibacterial, analgesic, antitumor, anticonvulsant, and anti-inflammatory drugs, pesticides and fungicides.2–4 Furthermore, some of them have been used as thermotropic liquid crystals, corrosion and oxidation inhibitors, or as dyes or metal ion complexation reagents.5–10 1,3,4-Selenadiazole-containing kidney-type glutaminase inhibitors showed improved cellular uptake and antitumor activity.11 However, little is known about the 2,5-disubstituted 1,3,4-selenadiazoles.12
Several methods have been reported for their preparation, which include a ring-closure reaction of selenobenzamides with hydrazine hydrate,13 reacting dimethylformamide azine with hydrogen selenide,14 treatment of 1,2-diacetylhydrazine with phosphorus pentaselenide,15 reaction of isoselenocyanates with selenosemicarbazides16 or a carboxylic acid with selenosemicarbazide and phosphoryl chloride (Fig. 1).17 However, the examples of these compounds have some shortcomings in the literature are limited due to either lack of starting materials or very low yield. Therefore, developing an effective and low-cost method for synthesizing two-substituted 1,3,4-selenathiazoles is still highly warranted. In recent times, the iodine/DMSO combination has received considerable attention, in synthetic organic chemistry, as an effective and eco-friendly oxidative system as it has affected numerous organic transformations.18–22 As our continuous work on the development of a new method for the synthesis of various heterocyclic biologically active molecules,23–43 we herein report iodine/DMSO catalysed a three-component reaction for the preparation of 1,3,4-selenathiazoles from the easily available, aryl aldehyde, hydrazine and selenium powder.
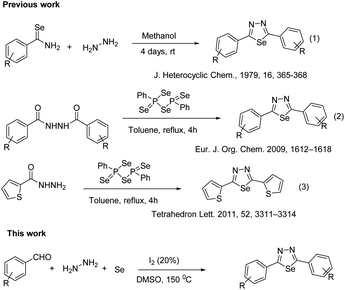 |
| Fig. 1 Methods for the synthesis of 1,3,4-selenadiazoles. | |
Results and discussion
Initially, our studies commenced using benzaldehyde 1a, hydrazine 2 and elemental selenium as a model substrate and the results are presented in Table 1. Firstly, 1a, 2 and selenium were taken in DMSO and the mixture was heated at 150 °C for 4 hours, however, the reaction failed to proceed and no product was detected (Table 1, entry 1). Due to the failure of this reaction, confirming the importance of catalyst in this multicomponent reaction. Therefore, we focused on sequential additions and accordingly, we attempted a reaction with 1a, 2, selenium and TBAI in DMSO, and heated at 150 °C for 4 hours. Encouragingly, the expected product 3a was obtained in an isolated yield of 51% (Table 1, entry 2). Accordingly, we focused our studies on to improve the yield, the reaction was attempted with KI and NH4I affording 3a in 42% and 35% yield respectively (Table 1, entries 3 and 4). Finally, I2 was shown to be the best performing catalyst as the yield significantly increased to 88% (Table 1, entry 7). Many synthetic procedures use varying amounts of I2 and reaction temperatures depending on the reaction conditions employed to produce the desired product in maximum yield. Thus, to complete the study, attempts were made to decrease the amount of iodine to 5% and 10%, however, lowered the yield of the desired product to 71% and 82% respectively (Table 1, entries 5 and 6). Furthermore, attempt was made to increase the amount of iodine to 30%, however, lowered the yield of the desired product to 79% (Table 1, entry 8). Further, the attempt was made to decrease the temperature to 120 °C, however, lowered the yield of the desired product to 76% (Table 1, entry 9). Finally, the attempt was made to increase the temperature to 170 °C, however, lowered the yield of the desired product to 76% (Table 1, entry 10). To conclude, the conditions described in entry 7 were found to be optimal, allowing for maximum conversion to the desired product 3a.
Table 1 Optimization of the reaction conditionsa

|
Entry |
Catalyst (%) |
Solvent |
Temp. (0 °C) |
Yield (%) |
1a (0.5 mmol), 2 (0.25 mmol), Se (0.25 mmol), catalyst (20 mol%), and solvent (3 mL) under air at 150 °C for 4 h. |
1 |
— |
DMSO |
150 |
Trace |
2 |
TBAI (20) |
DMSO |
150 |
51 |
3 |
NH4I (20) |
DMSO |
150 |
35 |
4 |
KI (20) |
DMSO |
150 |
42 |
5 |
I2 (5) |
DMSO |
150 |
71 |
6 |
I2 (10) |
DMSO |
150 |
82 |
7 |
I2 (20) |
DMSO |
150 |
88 |
8 |
I2 (30) |
DMSO |
150 |
79 |
9 |
I2 (20) |
DMSO |
120 |
76 |
10 |
I2 (20) |
DMSO |
170 |
71 |
With the optimized reaction conditions in hand (Table 1, entry 7), we subsequently investigated the substrate scope of this transformation (Schemes 1 and 2). Benzaldehyde bearing neutral, electron-donating, and electron-withdrawing substituents at the o/m/p-positions of the aromatic ring reacted smoothly under the optimized reaction conditions, affording the corresponding product in high yields (73–92%). Besides different benzaldehydes, other benzothiophene, benzofuran, indole aldehydes also yields 65–74%.
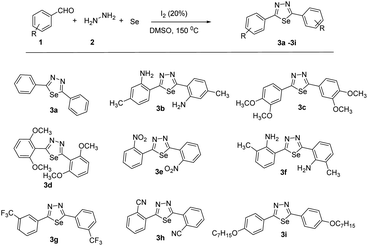 |
| Scheme 1 Synthesis of 2,5-diaryl-1,3,4-selenadiazoles. | |
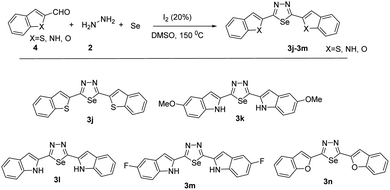 |
| Scheme 2 Synthesis of 2,5-diheteroaryl-1,3,4-selenadiazoles. | |
Moreover, the reaction also tolerated several functional groups, such as nitrile, methoxy, iodo and amino. All reactions proceeded smoothly to give the desired products in moderate to good yields. The reactivities of phenyl rings with strongly electron-withdrawing substituents (–CF3, –NO2) were higher than those of phenyl rings with electron-donating substituents. This suggests that electron-withdrawing groups enhance the reaction efficiency (3e and 3g). Substrates with substituents at the ortho or meta positions were also compatible with. Notably, the position of the substituent did not affect the reaction productivities and yields (3c, 3d and 3b, 3f). After replacing the phenyl group with heteroaryl, such as benzothiophene, benzofuran, indole aldehydes. All reactions proceeded smoothly to give the desired products in moderate to good yields. Compared with indole aldehydes, benzothiophene, benzofuran showed a lower reactivity in the reaction.
We have performed the several control experiments to interpret the reaction mechanism (Scheme 3). The first control experiment was carried out with selenium powder and synthetic diimine, it could be converted into the corresponding desired product 3a in 92% yield. This reaction completed in 1 h. This is because the process is one-step less than the standard reaction. Later, when we replaced Se with KSeCN, desired product 3a was obtained in moderate yield. Finally, radical inhibitors tetramethyl-piperidin-1-oxyl (TEMPO) and butylated hydroxytoluene (BHT) were used resulted in the inhibition of the reaction. These results suggested that this reaction proceeded through a radical pathway. We performed the scale up reactions 5 mmol and 10 mmol and obtained the desired product in 90% and 85% yields, respectively.
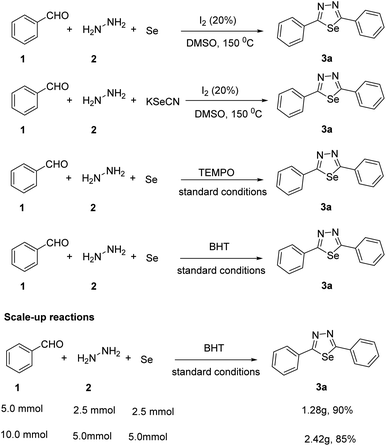 |
| Scheme 3 Controlled experiments. | |
From the above results, a proposed mechanism is presented in Scheme 4. Initially, di-imine generated from benzaldehyde 1a and hydrazine 2. Iodine radicals produced upon heating the molecular iodine. Then, iodine radicals get a proton from A to obtain the radical intermediate B and HI. HI reacts with DMSO to generate iodine, and the cycle continues.44,45 Later, radical intermediate B reacts with selenium powder to afford the selenium free-radical intermediate C.46 Again iodine radicals get a proton from C to obtain the radical intermediate D and HI. Finally, intermediate D undergoes cyclization to furnish the desired product 3a.
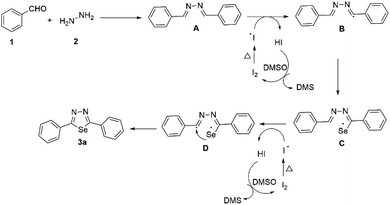 |
| Scheme 4 Proposed mechanism. | |
Conclusion
We have successfully developed a practical way for the synthesis of 1,3,4-selenadiazole through I2/DMSO promoted cascade cyclization process. We reported a mild method by the multicomponent reaction of different aldehydes, elemental selenium, and hydrazine. The present methodology has the advantages of being free of metal, the use of simple operation.
Experimental section
General procedure for the synthesis of 2,5-diaryl-1,3,4-selenadiazoles
Aldehyde (0.5 mmol), elemental selenium (0.25 mmol), hydrazine (0.25 mmol), iodine (20%), and DMSO (3 mL) were added to the reaction vial. The mixture was stirred at 150 °C for 4 h. After completion of reaction (confirmed by TLC), water was added and extracted with ethyl acetate. The combined organic layers were dried over magnesium sulfate. Evaporation of the solvent under reduced pressure provided the crude product, which was purified by column chromatography on silica gel (1–3% MeOH in dichloromethane as eluent) to give the corresponding target product.
2,5-Diphenyl-1,3,4-selenadiazole (3a)
White solid; Yield = 88%; mp 115–117 °C (lit. mp 116–118 °C);47 1
NMR (400 MHz, DMSO-d6): δ 7.85 (d, J = 7.8 Hz, 4H), 7.21–7.22 (m, 6H); 13
NMR (400 MHz, DMSO-d6): δ 170.75, 140.41, 129.51, 129.44, 129.14, 127.47, 109.55; HRMS (ESI) m/z calcd for C14H10N2Se (M + H)+ 287.0269, found 287.0300; elemental analysis: C, 58.96; H, 3.53; N, 9.82; Se, 27.69, found: C, 58.86; H, 3.62; N, 9.87; Se, 27.65.
6,6′-(1,3,4-Selenadiazole-2,5-diyl)bis(3-methylaniline) (3b)
Brown solid; yield = 73%; mp 165–167 °C; 1
NMR (400 MHz, CDCl3): δ 7.79 (d, J = 8.0 Hz, 2H), 6.47 (s, 2H), 6.44 (brs, 2H), 2.25 (s, 6H); 13
NMR (400 MHz, CDCl3): 173.58, 151.03, 145.91, 132.03, 118.04, 116.86, 107.49, 21.67; HRMS (ESI) m/z calcd for C16H16N4Se (M + H)+ 345.0581, found 345.0565; elemental analysis: C, 55.98; H, 4.70; N, 16.32; Se, 23.00, found C, 55.91; H, 4.72; N, 16.4; Se, 22.97.
2,5-Bis(3,4-dimethoxyphenyl)-1,3,4-selenadiazole (3c)
White solid; yield = 84%; mp 210–212 °C; 1
NMR (400 MHz, CDCl3): δ 7.74 (d, J = 8.0 Hz, 2H), 7.74 (s, 2H), 6.9 (d, J = 8.0 Hz, 2H), 3.93 (s, 6H), 3.92 (s, 6H); 13
NMR (400 MHz, CDCl3): 172.04, 153.71, 148.64, 124.58, 121.67, 112.26, 110.28, 56.05, 55.95; HRMS (ESI) m/z calcd for C18H18N2O4Se (M + H)+ 407.0155, found 407.0141; elemental analysis: C, 53.34; H, 4.48; N, 6.91; O, 15.79; Se, 19.48, found C, 53.31; H, 4.50; N, 6.92; O, 15.68; Se, 19.59.
2,5-Bis(2,6-dimethoxyphenyl)-1,3,4-selenadiazole (3d)
White solid; yield = 85%; mp 223–225 °C; 1
NMR (400 MHz, CDCl3): δ 7.33 (d, J = 8.0 Hz, 2H), 6.55 (d, J = 8.0 Hz, 4H), 3.85 (s, 12H); 13
NMR (400 MHz, CDCl3): 170.69, 157.81, 131.82, 111.59, 104.1, 56.16, 56.14; HRMS (ESI) m/z calcd for C18H18N2O4Se (M + H)+ 407.0155, found 407.0141; elemental analysis: C, 53.34; H, 4.48; N, 6.91; O, 15.79; Se, 19.48, found C, 53.29; H, 4.51; N, 6.89; O, 15.81; Se, 19.50.
2,5-Bis(2-nitrophenyl)-1,3,4-selenadiazole (3e)
Pale yellow solid; yield = 90%; mp 265–267 °C; 1
NMR (400 MHz, CDCl3): δ 8.06–8.00 (m, 4H), 7.45 (t, J = 8.0 Hz, 2H), 7.21 (d, J = 7.6 Hz, 2H); 13
NMR (400 MHz, CDCl3): 171.31, 141.98, 133.57, 133.09, 132.1, 128.02, 94.71; HRMS (ESI) m/z calcd for C14H8N4O4Se (M + H)+ 376.9842, found 376.9870; elemental analysis: C, 44.82; H, 2.15; N, 14.93; O, 17.06; Se, 21.04, found C, 44.75; H, 2.18; N, 14.89; O, 17.10; Se, 21.08.
6,6′-(1,3,4-Selenadiazole-2,5-diyl)bis(2-methylaniline) (3f)
Light brown solid; yield = 78%; mp 188–190 °C; 1
NMR (400 MHz, DMSO-d6): δ 7.63 (d, J = 8.0 Hz, 2H), 7.16 (d, J = 7.2 Hz, 2H), 6.48 (t, J = 8.0 Hz, 2H), 2.09 (s, 6H); 13
NMR (400 MHz, DMSO-d6): 172.69, 152.34, 137.04, 131.66, 125.6, 116.94, 112.01, 20.13; HRMS (ESI) m/z calcd for C16H16N4Se (M + H)+ 345.0481, found 345.0490; elemental analysis: C, 55.98; H, 4.70; N, 16.32; Se, 23.00, found C, 55.92; H, 4.71; N, 16.4; Se, 22.97.
2,5-Bis(3-(trifluoromethyl)phenyl)-1,3,4-selenadiazole (3g)
White solid; yield = 92%; mp 155–157 °C; 1
NMR (400 MHz, DMSO-d6): δ 8.23 (s, 2H), 8.17 (d, J = 7.6 Hz, 2H), 8.03 (d, J = 7.6 Hz, 2H), 7.67 (t, J = 8.0 Hz, 2H); 13
NMR (400 MHz, DMSO-d6): 166.10, 136.62, 134.16, 133.17, 132.48, 130.43, 118.48, 112.34; HRMS (ESI) m/z calcd for C16H8F6N2Se (M + H)+ 422.9569, found 422.9550; elemental analysis: C, 45.62; H, 1.91; F, 27.06; N, 6.65; Se, 18.75, found C, 45.58; H, 1.88; F, 27.04; N, 6.71; Se, 18.79.
2,2′-(1,3,4-Selenadiazole-2,5-diyl)dibenzonitrile (3h)
Off-white solid; yield = 80%; mp 159–160 °C; 1
NMR (400 MHz, DMSO-d6): δ 8.05 (d, J = 8.0 Hz, 2H), 8.02 (d, J = 6.8 Hz, 2H), 7.76–7.72 (m, 4H); 13
NMR (400 MHz, DMSO-d6): 165.68, 134.42, 133.76, 133.56, 133.32, 131.33, 118.16, 112.07; HRMS (ESI) m/z calcd for C16H8N4Se (M + H)+ 336.9953, found 336.9972; elemental analysis: C, 57.33; H, 2.41; N, 16.71; Se, 23.55, found: C, 57.30; H, 2.44; N, 16.76; Se, 23.50.
2,5-Bis(4-(heptyloxy)phenyl)-1,3,4-selenadiazole (3i)
White solid; yield = 81%; mp 95–98 °C; 1
NMR (400 MHz, DMSO-d6): δ 7.84 (d, J = 8.8 Hz, 4H), 6.96 (d, J = 8.8 Hz, 4H), 3.99 (t, J = 6.4 Hz, 4H), 1.69–1.65 (m, 4H), 1.35–1.22 (m, 16H), 0.83 (t, J = 6.8 Hz, 6H); 13
NMR (400 MHz, DMSO-d6): 167.43, 162.72, 131.78, 123.19, 114.62, 68.17, 31.65, 28.96, 28.83, 25.83, 22.47, 14.36; HRMS (ESI) m/z calcd for C28H38N2O2Se (M + H)+ 515.2109, found 515.2095; elemental analysis: C, 65.48; H, 7.46; N, 5.45; O, 6.23; Se, 15.37, found: C, 65.54; H, 7.44; N, 5.41; O, 6.19; Se, 15.42.
2,5-Di(benzofuran-2-yl)-1,3,4-selenadiazole (3j)
White solid; yield = 70%; mp 270–272 °C; 1
NMR (400 MHz, DMSO-d6): δ 7.75 (d, J = 8.0 Hz, 2H), 7.66 (d, J = 8.0 Hz, 2H), 7.62 (s, 2H), 7.47 (t, J = 8.4 Hz, 2H), 7.32 (t, J = 8.0 Hz, 2H); 13
NMR (400 MHz, DMSO-d6): 160.53, 155.4, 146.61, 127.98, 127.28, 124.24, 123.52, 113.95, 113.88, 112.49; HRMS (ESI) m/z calcd for C18H10N2O2Se (M + H)+ 366.9939, found 366.9916; elemental analysis: C, 59.19; H, 2.76; N, 7.67; O, 8.76; Se, 21.62, found: C, 59.15; H, 2.82; N, 7.65; O, 8.71; Se, 21.67.
2,5-Bis(benzo[b]thiophen-2-yl)-1,3,4-selenadiazole (3k)
White solid; yield = 65%; mp 265–268 °C; 1
NMR (400 MHz, DMSO-d6): δ 8.07 (s, 2H), 8.0–7.95 (m, 4H), 7.46–7.41 (m, 4H); 13
NMR (400 MHz, DMSO-d6): 163.96, 141.74, 139.15, 135.15, 130.7, 130.65, 127.44, 126.13, 125.49, 123.38; HRMS (ESI) m/z calcd for C18H10N2S2Se (M + H)+ 399.9454, found 399.9444; elemental analysis: C, 54.41; H, 2.54; N, 7.05; S, 16.14; Se, 19.87, found: C, 54.39; H, 2.51; N, 7.11; S, 16.10; Se, 19.89.
2,5-Di(1H-indol-2-yl)-1,3,4-selenadiazole (3l)
White solid; yield = 73%; mp 289–290 °C; 1
NMR (400 MHz, DMSO-d6): δ 11.71 (s, 2H), 7.61 (d, J = 8.4 Hz, 2H), 7.41 (d, J = 8.4 Hz, 2H), 7.21 (t, J = 8.4 Hz, 2H), 7.06 (s, 2H), 7.05 (t, J = 8.0 Hz, 2H); 13
NMR (400 MHz, DMSO-d6): δ 163.25, 137.66, 128.83, 127.28, 124.7, 122.34, 120.38, 112.91, 107.72; HRMS (ESI) m/z calcd for C18H12N4Se (M + H)+ 365.0279, found 365.0296; elemental analysis: C, 59.51; H, 3.33; N, 15.42; Se, 21.74, found: C, 59.47; H, 3.32; N, 15.39; Se, 21.82.
2,5-Bis(5-methoxy-1H-indol-2-yl)-1,3,4-selenadiazole (3m)
White solid; yield = 74%; mp 289–290 °C; 1
NMR (400 MHz, DMSO-d6): δ 11.57 (s, 2H), 7.3 (d, J = 8.8 Hz, 2H), 7.05 (s, 2H), 6.96 (s, 2H), 6.87 (dd, J = 8.8 Hz, J = 2.4 Hz, 2H), 3.71 (s, 6H),; 13
NMR (400 MHz, DMSO-d6): 161.17, 154.24, 132.99, 129.03, 127.57, 116.21, 113.78, 107.36, 102.38, 55.66; HRMS (ESI) m/z calcd for C20H16N4O2Se (M + H)+ 425.0442, found 425.0458; elemental analysis: C, 56.74; H, 3.81; N, 13.23; O, 7.56; Se, 18.65, found: C, 56.69; H, 3.82; N, 13.27; O, 7.63; Se, 18.59.
2,5-Bis(5-fluoro-1H-indol-2-yl)-1,3,4-selenadiazole (3n)
White solid; yield = 71%; mp 268–270 °C; 1
NMR (400 MHz, DMSO-d6): δ 11.83 (s, 2H), 7.42–7.34 (m, 4H), 7.08–7.03 (m, 2H), 7.03 (s, 2H); 13
NMR (400 MHz, DMSO-d6): 162.94, 158.74, 156.42, 134.39, 130.54, 127.36, 127.25, 114.21, 113.70, 113.44, 107.65, 106.51, 106.43, 106.29, 106.2; HRMS (ESI) m/z calcd for C18H10F2N4Se (M + H)+ 401.0111, found 401.0097; elemental analysis: C, 54.15; H, 2.52; F, 9.52; N, 14.03; Se, 19.78, found: C, 54.21; H, 2.48; F, 9.53; N, 14.06; Se, 19.72.
Conflicts of interest
The authors declare no conflicts of interest.
Acknowledgements
We are grateful to the Council of Scientific and Industrial Research (CSIR), New Delhi, India.
References
- C. J. Lowig, Pogg. Ann., 1836, 37, 552 Search PubMed.
- H. N. Dogan, A. Duran, S. Rollas, G. Sener, M. K. Uysalb and D. Gulenc, Bioorg. Med. Chem., 2020, 10, 2893–2896 CrossRef.
- S. Schenone, O. Bruno, A. Ranise, F. Bondavalli, W. Filippelli, G. Falcone, L. Giordano and M. R. Vitelli, Bioorg. Med. Chem., 2001, 9, 2149–2153 CrossRef CAS.
- J. Y. Chou, X. Y. Lai, S. L. Pan, G. M. Jow, J. W. Chen and J. H. Guh, Biochem. Pharmacol., 2003, 66, 115–117 CrossRef CAS.
- M. Saro, T. Kamita, K. Nakadera and K. I. Mukaida, Eur. Polym. J., 1995, 31, 395–400 CrossRef.
- F. Bentiss, M. Lagrenee, J. P. Wignacourt and E. M. Holt, Polyhedron, 2002, 21, 403–408 CrossRef CAS.
- J. D. E. T. Wilton-Ely, A. Schier and H. Schmidbaur, Organometallics, 2001, 20, 1895–1897 CrossRef CAS.
- F. Bentiss, M. Traisel and M. Lagrenee, J. Appl. Electrochem., 2001, 31, 41–48 CrossRef CAS.
- F. Bentiss, M. Lebrini, H. Vezin and M. Lagrenee, Mater. Chem. Phys., 2004, 87, 18–23 CrossRef CAS.
- B. Sybo, P. Bradley, A. Grubb, S. Miller, K. J. W. Proctor, L. Clowes, M. R. Lawrie, P. Sampson and A. J. Seed, J. Mater. Chem., 2007, 17, 3406–3410 RSC.
- Z. Chen, D. Li, N. Xu, J. Fang, Y. Yu, W. Hou, Ha. Ruan, P. Zhu, R. Ma, S. Lu, D. Cao, R. Wu, M. Ni, W. Zhang, W. Su and B. H. Ruan, J. Med. Chem., 2019, 62, 589–603 CrossRef CAS.
- A. Shafiee, I. Lalezari, S. Yazdany and A. Pournorouz, J. Pharm. Sci., 1973, 62, 839–842 CrossRef CAS.
- I. V. Cohen, J. Heterocycl. Chem., 1979, 16, 806–807 Search PubMed.
- R. V. Kendall and R. A. Olofson, J. Org. Chem., 1970, 35, 806–808 CrossRef CAS.
- R. Stolle and L. Gutmann, J. Prakt. Chem., 1904, 69, 509 CrossRef CAS.
- E. Bulka and D. Ehlers, J. Prakt. Chem., 1973, 315, 155–163 CrossRef CAS.
- I. Lalezari and A. Shafiee, J. Heterocycl. Chem., 1971, 8, 835–837 CrossRef CAS.
- J. Jayram and V. Jeena, RSC Adv., 2018, 8, 37557–37563 RSC.
- W. w. Li, J. Zhang, J. He, L. Xu, L. Vaccaro, P. Liu and Y. Gu, Front. Chem., 2020, 8, 466–475 CrossRef CAS.
- S. V. Gaikwad, D. N. Nadimetla, M. A. Kobaisi, M. Devkate, R. Joshi, R. G. Shinde and P. D. Lokhande, ChemistrySelect, 2019, 4, 10054–10059 CrossRef CAS.
- C. Xie, Z. Zhang, B. Yang, G. Song, H. Gao, L. Wen and C. Ma, Tetrahedron, 2015, 71, 1831–1837 CrossRef CAS.
- W. Ge, X. Zhu and Y. Wei, RSC Adv., 2013, 3, 10817–10822 RSC.
- B. S. Kuarm, Y. T. Reddy, J. V. Madhav, P. A. Crooks and B. Rajitha, Bioorg. Med. Chem. Lett., 2011, 21, 524 CrossRef CAS.
- S. V. Laxmi, Y. T. Reddy, B. S. Kuarm, P. N. Reddy, P. A. Crooks and B. Rajitha, Bioorg. Med. Chem. Lett., 2011, 21, 4329 CrossRef.
- B. K. Suresh, J. Venu Madhav, S. L. Laxmi, B. Rajitha, Y. Thirupathi Reddy, P. Narsimha Reddy and P. A. Crooks, Synth. Commun., 2010, 40, 3358 CrossRef.
- B. S. Kuarm, J. Venu Madhav, S. L. Laxmi, B. Rajitha, Y. Thirupathi Reddy, P. Narsimha Reddy and P. A. Crooks, Synth. Commun., 2011, 41, 1719 CrossRef CAS.
- B. K. Suresh and B. Rajitha, Synth. Commun., 2012, 42, 2382 CrossRef.
- B. S. Kuarm, J. Venu Madhav and B. Rajitha, Lett. Org. Chem., 2011, 8, 549 Search PubMed.
- B. K. Suresh, B. Janardan and B. Rajitha, Chin. J. Chem., 2012, 30, 947 CrossRef.
- J. V. Madhav, Y. Thirupathi Reddy and P. A. Crooks, Synth. Commun., 2008, 38, 3215 CrossRef CAS.
- B. Rajitha, Arkivoc, 2008, Xiii, 145 Search PubMed.
- B. Rajitha, Y. Thirupathi Reddy and P. A. Crooks, J. Chem. Res., 2008, 232 Search PubMed.
- B. S. Kuarm, V. N. Kumar, J. V. Madhav and B. Rajitha, Green Chem. Lett. Rev., 2011, 4, 97 Search PubMed.
- J. Venu Madhav and B. Rajitha, Arkivoc, 2008, Xii, 204 Search PubMed.
- S. V. Laxmi, B. S. Kuarm and B. Rajitha, Med. Chem. Res., 2013, 22, 768 Search PubMed.
- B. S. Kuarm, P. A. Crooks and B. Rajitha, Green Chem. Lett. Rev., 2013, 6, 228 Search PubMed.
- J. V. Madhav, B. S. Kuarm and B. Rajitha, Synth. Commun., 2008, 38, 3514 CrossRef CAS.
- B. S. Kuarm, J. V. Madhav and B. Rajitha, J. Heterocycl. Chem., 2013, 50, 1337 CrossRef CAS.
- J. Venu Madhav, Y. Thirupathi Reddy, P. Narsimha Reddy, M. Nikhil Reddy, S. Kuarm, P. A. Crooks and B. Rajitha, J. Mol. Catal. A: Chem., 2009, 304, 85 CrossRef CAS.
- B. K. Suresh, J. Venu Madhav and B. Rajitha, Synth. Commun., 2012, 42, 1770 CrossRef.
- B. K. Suresh, Y. Thirupathi Reddy, P. Narsimha Reddy and P. A. Crooks, Synth. Commun., 2011, 41, 662 CrossRef.
- B. S. Kuarm, J. Venu Madhav, S. V. Laxmi and B. Rajitha, Synth. Commun., 2012, 42, 1211 CrossRef CAS.
- B. S. Kuarm, M. Nirjal, A. Srinivas, P. Narsimha, K. Sesha and S. Kakraba, Molecules, 2020, 25, 3610 CrossRef.
- J. Jayram and V. Jeena, RSC Adv., 2018, 8, 37557 RSC.
- M. Wang, B.-C. Tang, J.-T. Ma, Z.-X. Wang, J.-C. Xiang, Y.-D. Wu, J.-G. Wang and A.-X. Wu, Org. Biomol. Chem., 2019, 17, 1535 RSC.
- P. Ni, J. Tan, W. Zhao, H. Huang, F. Xiao and G. A. Deng, Org. Lett., 2019, 21, 3518 CrossRef CAS.
- G. Hua, Y. Li, A. L. Fuller, A. M. Z. Slawin and J. Derek Woollins, Eur. J. Org. Chem., 2009, 10, 1612 CrossRef.
Footnote |
† Electronic supplementary information (ESI) available. See DOI: 10.1039/d0ra10576g |
|
This journal is © The Royal Society of Chemistry 2021 |
Click here to see how this site uses Cookies. View our privacy policy here.