DOI:
10.1039/D0RA10451E
(Paper)
RSC Adv., 2021,
11, 13292-13296
Cascade synthesis of 2,4-disulfonylpyrroles by the sulfonylation/[2 + 3]-cycloaddition reactions of gem-dibromoalkenes with arylsulfonyl methyl isocyanides†
Received
11th December 2020
, Accepted 16th March 2021
First published on 12th April 2021
Abstract
An efficient cascade reaction involving sulfonylation and [2 + 3]-cycloaddition reactions of gem-dibromoalkenes with arylsulfonyl methyl isocyanides was described for the synthesis of 3-aryl-2,4-disulfonyl-1H-pyrroles. The main highlight of this study is the introduction of a new dual-functional reactivity of arylsulfonyl methyl isocyanides as the sulfonyl source as well as a 1,3-dipolar reagent in the same reaction. This system is facilitated by Cs2CO3 mediation in DMSO and 100 °C conditions.
Introduction
Since its first isolation from bone pyrolysis in 1857, the pyrrole unit was identified as a fundamental substructure of numerous important natural and bioactive unnatural products.1 Lamellarin R as an anti-cancer and anti-HIV alkaloid,2 and other active pyrrolizidine alkaloids, chlorophyll, cobalamin (vitamin B12), heme and bile pigments are some of the well-known naturally occurring pyrrole derivatives.3 Hence, numerous syntheses for substituted pyrroles have been reported including classical Hantzsch,4 Knorr5 and Paal–Knorr6 methods and some alternative procedures such as aza-Wittig, coupling, condensation and annulation reactions.7 Owing to the practicality and commercial accessibility of their compounds, isocyanides and π systems have been demonstrated to be attractive functionalities for heterocyclic cycloaddition reactions that due to appropriate atom-economy are considered as an ideal approach for the direct synthesis of substituted pyrroles.8
In particular, recently, activated isocyanides, including α-acidic isocyanides with additional functionalities owing to their 1,3-dipolar nature and efficient cooperation in [3 + 2] cycloaddition reactions have attracted considerable attention of numerous synthetic researchers.9 Amongst these structures, arylsulfonyl methyl isocyanides (ASMIC), particularly p-toluenesulfonylmethyl isocyanide (TosMIC), are one of the most impressive reagents from both the stability and reactivity point of views.10 TosMIC, as an odorless derivative of isocyanides can afford a multitude of reactions via its three different functionalities of α-acidic carbon, isocyanide and sulfonyl groups.11 Two features of less sterically congested α-C–H bonds and enhanced electrophilicity as a consequence of additional sulfonyl functionality distinguish this reactive reagent from other alkyl isocyanides (Fig. 1).12,13
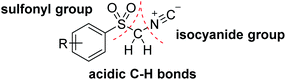 |
| Fig. 1 Structure of TosMIC. | |
Moreover, sulfonyl function as a leaving group can offer TosMIC an efficient source of sulfonyl group, whereby it can act as a dual reagent and/or as a sulfonylation reagent.12 In this regard, Bi et al. introduced the dual role of TosMIC in a cascade reaction with propargylic alcohols, wherein TosMIC acted as a reagent for allenylative and sulfonylation in the presence of Ag2CO3 to give (E)-vinyl sulfones (Scheme 1, eqn (1)).14 Also, recently, our group investigated the behavior of TosMIC in treatment by 2-chloroquinoline-3-carbaldehydes, and the result was in tandem with the van Leusen/ring-closing procedure and tosylation to create 5-(2-tosylquinolin-3-yl)oxazoles, which testified to the dual-functional reactivity of mentioned isocyanide (Scheme 1, eqn (2)).15
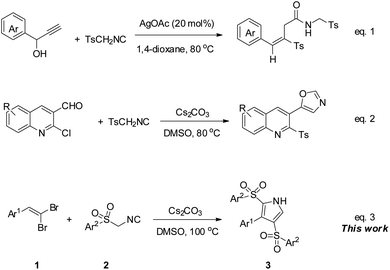 |
| Scheme 1 ASMIC as a dual-functional reactive starting material in the same reaction. | |
As our ongoing effort to explore the novel synthetic application of 1,1-dibromoalkenes, we have previously investigated the reaction of this alkenes with two reagents of alkyl isocyanides and sodium sulfinates, which in the former, 3-(hetero)arylpropynamides furnished from a palladium-catalyzed cross-coupling reaction,16 and the latter involved a sulfonylation to yield the desired 1-bromo-1-sulfonylalkenes.17 gem-Dibromo-1-alkenes, which can be easily generated from the treatment of aldehydes or ketones with CBr4/PPh3,18 can act as an alternative resource of activated alkene and alkyne moieties.19 Encouraged by these findings, we envisaged that arylsulfonyl methyl isocyanides might react with 1,1-dibromoalkenes as a dual functional reagent, both as a [1,3]-dipolar and as a sulfonyl source in a cascade process. To our delight, 3-aryl-2,4-disulfonyl-1H-pyrroles were isolated up to 90% yield from the mentioned reaction under Cs2CO3 condition at 100 °C (Scheme 1, eqn (3)).
Results and discussion
Inspired by our previously developed conditions,17 we started our survey by the cycloaddition of 1-bromo-2-(2,2-dibromovinyl)benzene (1a)20 and TosMIC (2a) under Cs2CO3 condition (2 equiv.) in DMSO at 100 °C. Two different routes were envisageable for the reaction (Scheme 2). What actually rationalizes the formation of 3a product (route B) is the occurrence of an additional step of the tosylation procedure, which affirms the dual role of TosMIC. Noteworthily, in this transformation, no by-product outcome from the direct [2 + 3]-cycloaddition of acetylene bromide (route A) was observed.
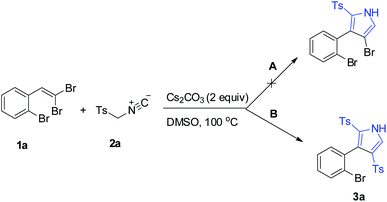 |
| Scheme 2 Formation of 2,4-ditosyl-1H-pyrrole instead of 4-bromo-2-tosyl-1H-pyrrole. | |
Our initial study focused on the exploration of optimal reaction conditions. As summarized in Table 1, to first evaluate the base efficiency, the reaction was conducted in a base-free media whereby no desired product was detected (Table 1, entry 1). This result encouraged us to screen numerous bases involving CsF, Cs2CO3, Et3N, K2CO3, Na2CO3, NaOAc, tBuONa, and tBuOK at 100 °C (Table 1, entries 2–9) and the best yield of 3a was obtained using Cs2CO3 in 78% yield (Table 1, entry 3). Notably, the counterion effect of the basic anions was compared, and the results showed its effective role in proceeding the reaction to high yields as the counterion size increased (Na, K to Cs). Also, when the amount of Cs2CO3 was decreased, the result reduced to 50% yield (Table 1, entry 10). Changing the reaction media to other solvents such as DMF, toluene or CH3CN, was not very successful to improve the product yield (Table 1, entries 11–13). In fact, the reaction failed in toluene (Table 1, entry 12). Implementing the reaction in ambient temperature offered no conversion, proving the distinct critical role of temperatures for the reaction to proceed (Table 1, entry 14). Summarizing the observations, we found that 2 equivalents of Cs2CO3 in DMSO at 100 °C were the best conditions listed in entry 3 and were chosen for the following explorations.
Table 1 Optimization of reaction conditiona
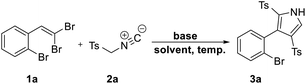
|
Entry |
Base (equiv.) |
Solvent |
Temp. (°C) |
Yieldb (%) |
Reaction conditions: 1a (1.0 mmol), 2a (2.0 mmol), base (2 equiv.), solvent (4.0 mL), temp. (100 °C) (bath temperature), 3–6 h. Isolated yields. |
1 |
— |
DMSO |
100 |
— |
2 |
CsF (2) |
DMSO |
100 |
20 |
3 |
Cs2CO3 (2) |
DMSO |
100 |
78 |
4 |
Et3N (2) |
DMSO |
100 |
Trace |
5 |
K2CO3 (2) |
DMSO |
100 |
15 |
6 |
Na2CO3 (2) |
DMSO |
100 |
12 |
7 |
NaOAc (2) |
DMSO |
100 |
40 |
8 |
tBuONa (2) |
DMSO |
100 |
56 |
9 |
tBuOK (2) |
DMSO |
100 |
65 |
10 |
Cs2CO3 (1) |
DMSO |
100 |
50 |
11 |
Cs2CO3 (2) |
DMF |
100 |
75 |
12 |
Cs2CO3 (2) |
Toluene |
Reflux |
Trace |
13 |
Cs2CO3 (2) |
CH3CN |
Reflux |
25 |
14 |
Cs2CO3 (2) |
DMSO |
rt |
— |
Under the determined optimal conditions, we perused the scope of reaction by numerous available starting materials. As illustrated in Table 2, the process was readily extended to a range of substituted aromatic and heteroaromatic gem-dibromoalkenes (1) with TosMIC (2a). The steric effects derived from ortho-substitutions and quinoline rings attached to the alkene had nearly no influence on these transformations (3a–3m). Similar to TosMIC, phenylsulfonylmethyl isocyanide (2b) also participated in the cascade reaction with 2,6-dichloro-3-(2,2-dibromovinyl)quinoline and 1-(2,2-dibromovinyl)-4-nitrobenzene without any difficulties, giving rise to the corresponding products in 80% and 87% yields, respectively (3n and 3o). Notably, the presence of a Me or an OMe group on the aryl ring of the gem-dibromoalkene did not afford the expected results.
Table 2 Scope of the synthesis of numerous 3-aryl-2,4-disulfonyl-1H-pyrroles 3a
Reaction conditions: 1 (1.0 mmol), 2 (2.0 mmol), Cs2CO3 (2 equiv.), DMSO (4.0 mL), temp. (100 °C) (bath temperature), 3–6 h. |
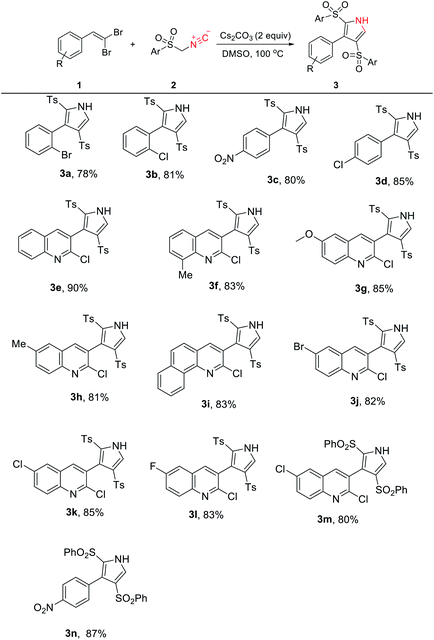 |
Further experiments were performed based on a designed series of control experiments to provide some insights into the reaction profile, as depicted in Scheme 3. Initially, the conversion of 1-(2,2-dibromovinyl)-4-nitrobenzene (1c) as the only starting material under the established condition was investigated, leading to the detection of desired acetylene bromide A. By assuming the following sulfonylation, we treated the resulting acetylene with sodium p-toluenesulfinate (TsNa) as the tosyl source based on our previously related study.17 Similarly, we found vinyl sulfone B as the only product, which in continuation, reacted with TosMIC in optimal conditions furnishing compound 3c in 80% yield.
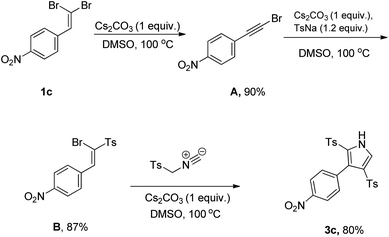 |
| Scheme 3 A designed sequences of control experiments for mechanistic investigation. | |
Regarding the above-described findings, we suggested a plausible mechanism for this cascade reaction initiated by the HBr elimination process of 1,1-dibromoalkene 1 in an alkali media to form acetylene bromide A (Scheme 4). Subsequently, in a domino manner, TosMIC 2a acts as a sulfonyl source to render intermediate B, which undergoes the second HBr elimination to generate the sulfonyl acetylene C by Cs2CO3 assistance. Eventually, the [2 + 3]-cycloaddition of acetylene C with another TosMIC furnishes the desired product 3.
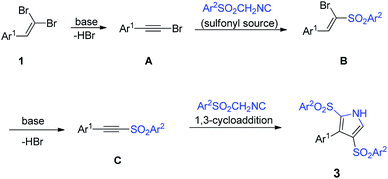 |
| Scheme 4 A plausible reaction mechanism. | |
Conclusions
We introduced a novel and straightforward strategy for the preparation of 3-aryl-2,4-disulfonyl-1H-pyrroles in high efficiency, which took advantage of a dual functional reactivity of arylsulfonyl methyl isocyanides against 1,1-dibromoalkenes under basic conditions. This process was facilitated in the presence of Cs2CO3, and its extensibility was evaluated for numerous starting materials. Furthermore, based on preliminary investigations of the reaction profile, a possible mechanism was proposed involving acetylenic intermediates. This report can be helpful to boost the practical developments of arylsulfonyl methyl isocyanides chemistry.
Conflicts of interest
There are no conflict to declare.
Acknowledgements
Alzahra University and INSF (Iran National Science Foundation) are gratefully acknowledged for their support.
References
-
(a) V. Estevez, M. Villacampa and J. C. Menendez, Multicomponent reactions for the synthesis of pyrroles, Chem. Soc. Rev., 2010, 39, 4402 RSC;
(b) S. D. Joshi, U. A. More, V. H. Kulkarni and T. M. Aminabhavi, Pyrrole: chemical synthesis, microwave assisted synthesis, reactions and applications: a review, Curr. Org. Chem., 2013, 17, 2279 CrossRef CAS;
(c) J. T. Gupton, Pyrrole natural products with antitumor properties, in Heterocyclic Antitumor Antibiotics, Springer, 2006, pp. 53–92 Search PubMed;
(d) S. S. Gholap, Pyrrole: an emerging scaffold for construction of valuable therapeutic agents, Eur. J. Med. Chem., 2016, 110, 13 CrossRef CAS PubMed;
(e) V. Bhardwaj, D. Gumber, V. Abbot, S. Dhiman and P. Sharma, Pyrrole: a resourceful small molecule in key medicinal hetero-aromatics, RSC Adv., 2015, 5, 15233 RSC;
(f) S. Ahmad, O. Alam, M. J. Naim, M. Shaquiquzzaman, M. M. Alam and M. Iqbal, Pyrrole: an insight into recent pharmacological advances with structure activity relationship, Eur. J. Med. Chem., 2018, 157, 527 CrossRef CAS PubMed.
- M. Iwao, T. Fukuda and F. Ishibashi, Synthesis and biological activity of lamellarin alkaloids: an overview, Heterocycles, 2011, 83, 491 CrossRef.
-
(a) V. Estévez, M. Villacampa and J. C. Menéndez, Recent advances in the synthesis of pyrroles by multicomponent reactions, Chem. Soc. Rev., 2014, 43, 4633 RSC;
(b) J. R. Liddell, Pyrrolizidine alkaloids, Nat. Prod. Rep., 2001, 18, 441 RSC;
(c) B. Forte, B. Malgesini, C. Piutti, F. Quartieri, A. Scolaro and G. Papeo, The pyrrole-imidazole alkaloids, Mar. Drugs, 2009, 7, 705 CrossRef CAS PubMed;
(d) P. W. Le Quesne, Y. Dong and T. A. Blythe, Recent Research on Pyrrole Alkaloids, Pergamon Press, Oxford, 1999, vol. 13 Search PubMed;
(e) V. F. Ferreira, M. C. B. V. de Souza, A. C. Cunha, L. O. R. Pereira and M. L. G. Ferreira, Recent advances in the synthesis of pyrroles, Org. Prep. Proced. Int., 2001, 33, 411 CrossRef CAS.
- A. Hantzsch, Ber. Dtsch. Chem. Ges., 1890, 23, 1474 CrossRef.
- L. Knorr, Synthese von pyrrolderivaten, Ber. Dtsch. Chem. Ges., 1884, 17, 1635 CrossRef.
- C. Paal, Synthese von Thiophen-und Pyrrolderivaten, Ber. Dtsch. Chem. Ges., 1885, 18, 367 CrossRef.
-
(a) A. R. Katritzky, J. Jiang and P. J. Steel, 1-Aza-1,3-bis(triphenylphosphoranylidene)propane: a novel: CHCH2N: synthon, J. Org. Chem., 1994, 59, 4551 CrossRef CAS;
(b) E. Baltazzi and L. I. Krimen, Recent Advances in the Chemistry of Pyrrole, Chem. Rev., 1963, 63, 511 CrossRef CAS;
(c) B. A. Trofimov, I. M. Al'bina, E. Y. Schmidt and L. N. Sobenina, Chemistry of Pyrroles, CRC press, 2014 CrossRef;
(d) F. J. Leeper and J. M. Kelly, Synthesis of 3,4-disubstituted pyrroles. A review, Org. Prep. Proced. Int., 2013, 45, 171 CrossRef CAS.
-
(a) A. V. Lygin and A. de Meijere, Isocyanides in the synthesis of nitrogen heterocycles, Angew. Chem., Int. Ed., 2010, 49, 9094 CrossRef CAS PubMed;
(b) S. Sadjadi, M. M. Heravi and N. Nazari, Isocyanide-based multicomponent reactions in the synthesis of heterocycles, RSC Adv., 2016, 6, 53203 RSC;
(c) M. Shiri, N. Farajinia-Lehi, P. Salehi and Z. Tanbakouchian, Transition Metal and Inner Transition Metal Catalyzed Amide Derivatives Formation through Isocyanide Chemistry, Synthesis, 2020, 52, 3162 CrossRef CAS.
-
(a) I. V. Efimov, L. N. Kulikova, D. I. Zhilyaev and L. G. Voskressensky, Recent Advances in the Chemistry of Isocyanides with Activated Methylene Group, Eur. J. Org. Chem., 2020, 7284 CrossRef CAS;
(b) J.-Y. Liao, P.-L. Shao and Y. Zhao, Catalytic divergent synthesis of 3H or 1H pyrroles by [3 + 2] cyclization of allenoates with activated isocyanides, J. Am. Chem. Soc., 2015, 137, 628 CrossRef CAS PubMed;
(c) M. Gao, C. He, H. Chen, R. Bai, B. Cheng and A. Lei, Synthesis of Pyrroles by Click Reaction: Silver-Catalyzed Cycloaddition of Terminal Alkynes with Isocyanides, Angew. Chem., Int. Ed., 2013, 52, 6958 CrossRef CAS PubMed;
(d) J. Cai, H. Bai, Y. Wang, X. Xu, H. Xie and J. Liu, Base-mediated regioselective [3 + 2] annulation of ketenimines and isocyanides: efficient synthesis of 1,4,5-trisubstituted imidazoles, Chem. Commun., 2019, 55, 3821 RSC;
(e) X. Meng, P. Liao, J. Liu and X. Bi, Silver-catalyzed cyclization of 2-pyridyl alkynyl carbinols with isocyanides: divergent synthesis of indolizines and pyrroles, Chem. Commun., 2014, 50, 11837 RSC.
-
(a) A. D. Mathiyazhagan and G. Anilkumar, Recent advances and applications of p-toluenesulfonylmethyl isocyanide (TosMIC), Org. Biomol. Chem., 2019, 17, 6735 RSC;
(b) D. V. Leusen and A. M. V. Leusen, Organic reactions 2001. Synthetic uses of tosylmethyl isocyanide (TosMIC), Org. React., 2001, 57, 417 Search PubMed;
(c) T. Kaur, P. Wadhwa and A. Sharma, Arylsulfonyl methyl isocyanides: a novel paradigm in organic synthesis, RSC Adv., 2015, 5, 52769 RSC.
- D. V. Aderohunmu, T. T. Odutola, O. O. Ajani and E. F. Adebiyi, TosMIC reagent: an excellent precursor in the synthesis of biologically active heterocycles, Rasayan J. Chem., 2019, 12, 1919 CrossRef CAS.
- K. Kumar, TosMIC: A Powerful Synthon for Cyclization and Sulfonylation, ChemistrySelect, 2020, 5, 10298 CrossRef CAS.
- V. V. R. Reddy, p-Toluenesulfonylmethyl isocyanide (TosMIC), Synlett, 2005, 363 CrossRef CAS.
- H. Bounar, Z. Liu, L. Zhang, X. Guan, Z. Yang, P. Liao, X. Bi and X. Li, Silver-catalyzed cascade reaction of tosylmethyl isocyanide (TosMIC) with propargylic alcohols to (E)-vinyl sulfones: dual roles of TosMIC, Org. Biomol. Chem., 2015, 13, 8723 RSC.
- Z. Yasaei, Z. Mohammadpour, M. Shiri, Z. Tanbakouchian and S. Fazelzadeh, Isocyanide reactions toward the synthesis of 3-(oxazol-5-yl)quinoline-2-carboxamides and 5-(2-tosylquinolin-3-yl)oxazole, Front. Chem., 2019, 7, 433 CrossRef PubMed.
- P. Salehi and M. Shiri, Palladium-Catalyzed Regioselective Synthesis of 3-(Hetero)Arylpropynamides from gem-Dibromoalkenes and Isocyanides, Adv. Synth. Catal., 2019, 361, 118 CrossRef CAS.
- M. Shiri, P. Salehi, Z. Mohammadpour, P. Salehi and B. Notash, Cs2CO3-Mediated Regio- and Stereoselective Sulfonylation of 1,1-Dibromo-1-alkenes with Sodium Sulfinates, Synthesis, 2021, 53, 1149 CrossRef CAS.
- E. J. Corey and P. L. Fuchs, Synthetic method for conversion of formyl groups into ethynyl groups, Tetrahedron Lett., 1972, 36, 3769 CrossRef.
-
(a) M. M Heravi, S. Asadi, N. Nazari and B. Malekzadeh Lashkariani, Developments of Corey–Fuchs reaction in organic and total synthesis of natural products, Curr. Org. Chem., 2015, 19, 2196 CrossRef;
(b) G. Chelucci, Synthesis and metal-catalyzed reactions of gem-dihalovinyl systems, Chem. Rev., 2012, 112, 1344 CrossRef CAS PubMed.
-
(a) N. B. Desai, N. McKelvie and F. Ramirez, A new synthesis of 1,1-dibromoölefins via phosphine-dibromomethylenes. The reaction of triphenylphosphine with carbon tetrabromide, J. Am. Chem. Soc., 1962, 84, 1745 CrossRef;
(b) D. Nandini, M. Asthana, K. Mishra, R. P. Singh and R. M. Singh, Temperature dependent selective synthesis of linear 2-bromo and 2-alkoxyfuro[2,3-b]quinolines: reaction of 3-(2,2-dibromovinyl-)quinoline-2(1H)-ones with alcoholic KOH, Tetrahedron Lett., 2014, 55, 6257 CrossRef CAS.
Footnote |
† Electronic supplementary information (ESI) available. See DOI: 10.1039/d0ra10451e |
|
This journal is © The Royal Society of Chemistry 2021 |
Click here to see how this site uses Cookies. View our privacy policy here.