DOI:
10.1039/D0RA10170B
(Paper)
RSC Adv., 2021,
11, 4829-4841
In vivo acute toxicity and mutagenic analysis of crude saponins from Chenopodium quinoa Willd husks
Received
2nd December 2020
, Accepted 17th January 2021
First published on 26th January 2021
Abstract
Background: As a functional food factor, quinoa saponins are valuable as additives and in medical care, pharmaceutical development, cosmetics and other fields. However, few studies have investigated the toxicity of saponins. The main purpose of this study was to evaluate the toxicity of crude saponins extracted from quinoa husks. Thus, acute toxicity and excretion experiments were carried out in rats. The Ames test, micronucleus test and mouse sperm aberration test were carried out in mice. Results: In the acute toxicity study, the obtained LD50 was more than 10 g per kg per bw for both sexes, the food intake of all rats decreased over a period of time, and some rats developed diarrhea. In the case of large-dose gavage, the saponin excretion time in rats was approximately four days. When the dosage was 10 mg kg−1, quinoa saponins were hydrolyzed into aglycone within 24 hours and excreted out of the body. The results of the mutagenicity experiment showed that saponins had no mutagenicity in mice. Conclusion: This work has demonstrated that quinoa saponins have limited acute toxicity effects, which provides a theoretical basis for their rational utilization.
Introduction
Quinoa is a traditional food in the Andes.1 Recently, there has been a large amount of interest in quinoa, which contains a very high content of protein, minerals, vitamins and phytochemicals such as phenolic acids, flavonoids and saponins.2 Due to the lack of gluten, some people classify this pseudocereal as a “superfood”.3,4 In addition to its high nutritional value, quinoa can be grown on marginal lands because it adapts to different climate and soil conditions.5 As saponins affect the taste of quinoa, some varieties of sweet quinoa were invented. However, these varieties contain few saponins (<0.11% free saponins) and tend to have a low resistance to insects and birds.6,7 Quinoa can increase endurance, promote health and prevent and treat obesity, cardiovascular diseases, diabetes, cancer and other diseases.8 In the late 1970s, quinoa production started to experience a renaissance within South America, not only for domestic consumption but also for export.9 Quinoa cultivation is in the process of rapid expansion outside its traditional cultivated areas with good yields. To date, quinoa is presently cultivated or tested in 95 countries worldwide.10,11 However, the processing of quinoa for food can produce approximately 8–12% husks, which are usually discarded because of their bitter saponins. Moreover, quinoa husks are usually burned or discarded without being utilized.12
The saponins in quinoa are triterpenoid glucoside compounds and are distributed throughout the plant, mainly in the mammary cells of the outermost layer (husks), with a total content of approximately 2–6% of the grain weight.13–15 They have a bitter and anti-nutritional effect, impeding consumption, and in order to improve sensory quality and consumer acceptance, the amount of this substance is usually reduced or removed from the outside of grain by grinding or washing.16,17
Most of the reported bioactive compounds of quinoa are polysaccharides and phenolic acids.18–20 In recent years, many studies have characterized saponins from various perspectives (e.g., bacteriostatic, anti-inflammatory, antidiabetic, antioxidant, antiobesity, etc.).21–24 In addition, Ruiz and other researchers made a molluscicide from quinoa saponins, which had good effects.25 What's more, Ruiz did some basic toxicological studies including acute oral/dermal/inhalation toxicity of saponin powders in rats, acute eye/dermal irritation/corrosion effects of saponin powder in rabbits, skin sensitization of saponin powder in guinea pigs and other acute toxicity tests for fish/algae/microcrustaceans.25 However, few studies on the toxicity and excretion of quinoa saponin have been performed,23,25 no information is available on the mutagenic, teratogenicity and sub-chronic (90 day) oral toxicity of quinoa saponin. Regardless of discarding quinoa husk or removing saponins, a large amount of saponins will be exposed to the living environment. At present, saponins have been proved to have a large number of biological active functions, and eating saponins is beneficial to human health. However, the consequences of long term consumption of saponins for human health are still unknown. Therefore, it is necessary to study the safety evaluation of saponins. We isolated crude saponins from quinoa, identified their chemical properties, and selected 4 tests to evaluate their safety. The present study was undertaken to assess the potential toxicity of quinoa saponin and better understand the safety of quinoa saponins and to research whether quinoa saponins are harmful to human health. The work flow of this study are shown in Fig. 1.
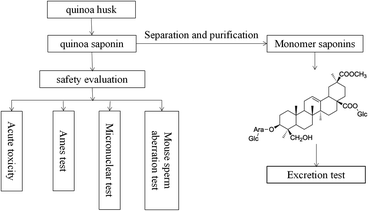 |
| Fig. 1 A work flow of this study. | |
Materials and methods
Drug and chemicals
Chenopodium quinoa Willd was obtained from Jingle Yilong Quinoa Co., Ltd. Quinoa saponin Q3 (3-O-β-D-glucopyranosyl-(1→3)-α-L-arabinopyranosyl-phytolaccagenic acid 28-O-β-D-glucopyranosyl) was prepared in the laboratory with >98% purity. Chromatographic grade methanol and acetonitrile were obtained from Fisher (America). All other reagents were analytical grade. The Ames kit was obtained by Huizhi Taikang Biochemical Co., Ltd.
Extraction of the crude saponins
Saponins were extracted from quinoa husks according to a previously described method.26 Briefly, quinoa husk powder was ultrasonically extracted twice with 75% ethanol (1
:
8 and 1
:
6) for 1 h and for 30 min, respectively, and the supernatants were combined. Rotary evaporation was conducted and condensed at 60 °C. The concentrate was extracted with ethylacetate (3×), petroleum (3×), and n-butanol (3×) until colorless. The n-butanol fraction was collected, evaporated and lyophilized by freeze-drying and stored at −20 °C until usage to obtain the crude quinoa saponin.
The chromatographic conditions
HPLC analysis was performed, the SHIMADZU Prominence LC-20A HPLC instrument (Shimadzu Corporation) and a YMC-ODS Pack column (4.6 mm × 250 mm, YMC Co., Ltd.) was used. The parameters of the HPLC were conducted according to the methods of Xue Peng.16 The detection wavelength was set at 202 nm and the column oven was maintained at 25 °C. Solvent A was water and solvent B was acetonitrile. The linear UPLC gradient elution programs were used as follows: 5 min 10% B; 10 min 15% B; 15 min 20% B; 35 min 28% B; 50 min 40% B; 60 min 60% B; 70 min 70% B and 75 min 10% B. The separation was operated at a flow rate was of 1 mL min−1 and the injection volume was 10 μL.
LC-MS analysis
Chromatographic analysis was performed on Thermo Scientific Ultimate Standard system (Thermo, America) equipped with a binary pump, an auto plate-sampler, an online degasser, and a thermostatically controlled column compartment. Sample separation was achieved on a Hypersil GOLD™ column (Thermo Scientific, 1.9 μ particles, 100 mm × 2.1 mm) with a constant flow rate of 0.3 mL min−1. The mobile phase was composed of acetonitrile (solvent A) and water (solvent C), using a gradient elution of 90–54% C at 0–10 min, 54–26% C at 10–15 min, 26–0% C at 15–22 min, 0–90% C at 22–30 min. The sample volume injected was set at 4 μL.
Detection was performed by a Q-Exactive mass spectrometer (Thermo Scientific, America) with HESI source. The operating parameters were optimized as follows: sheath gas flow rate, 30 L min−1; aux gas flow rate, 8 L min−1; spray voltage, 3.2 KV; capillary temperature, 320 °C and aux gas heater temp, 310 °C. Mass spectra were recorded across the range m/z 150–2000 in negative modes.
Animals and treatment
Male and female specific pathogen-free (SPF) adult Wistar rats (140–180 g) for acute toxicity and SPF Kunming mice (20–30 g) for the mouse sperm aberration test and micronuclear experiment were obtained from Jinan Pengyue Experimental Animal Breeding Co., Ltd. under license number SCXK (LU) 20140007.
Animal rooms were maintained at a temperature of 25 ± 3 °C and a relative humidity of 40%, and animals had free access to tap water and a standard diet. An automatic air circulation system was used for air exchange to ensure the normal breathing of rats, and disinfection was regularly performed. All rats were acclimatized for one week before starting the experiments, and then they were kept individually in cages.
Acute toxicity study
Experimental design. To determine the toxicity of quinoa saponins and to identify the LD50, experiments were conducted according to the methods of GB/T 21757-2008 and Li.27 After a few days of quarantine and acclimation, fifty SPF-grade Wistar rats were randomly divided into five groups, with 10 rats in each group (5 females and 5 males), and reared in cages. Saponins were dissolved in purified water and administered by oral gavage once a day at the doses of low-dose group (1.00 g kg−1), medium-dose group (2.15 g kg−1), high-dose group (4.64 g kg−1) and ex high-dose group (10.0 g kg−1) after fasting for 24 h. The control group was given the same volume of water. Clinical signs were monitored continuously for 4 h after dosing. Each rat was observed for 14 days, and clinical signs, mortality and the body weight of each rat were measured with an electronic scale. The LD50 was calculated according to Horn's method.28 If the LD50 was not detected, no further testing was required because the concentration of the highest dose group reached 10.0 g kg−1, which is far greater than the pharmacologically relevant equivalent dose. According to toxicological toxicity classification standards, it is already practically nontoxic. The classification standards are shown in Table 1.
Table 1 Toxicity grading standards
Toxicity classification |
One oral (mg kg−1) |
Level 6, very poisonous |
<1 |
Level 5, highly toxic |
1–50 |
Level 4, moderate poison |
51–500 |
Level 3, low toxicity |
501–5000 |
Level 2, practically non-toxic |
5001–15 000 |
Level 1, non-toxic |
>15 000 |
Clinical observation. Animals were observed for any moribundity, general health status and signs of toxicity. Clinical observations were carried out, including monitoring eating and drinking behaviors, skin, fur, gait, posture, abnormal sensitivity to external stimuli, abnormal urine and feces, shortness of breath, stereotyped or bizarre behavior and other toxic reactions or death. The food conversion efficiency (weight gain/100 g food consumed) during the 14 days of the study was also calculated.
Hematology, serum biochemistry and histopathological examinations. At the end of the experiment, all remaining rats were euthanized by anesthesia, and blood samples were collected from the retroorbital plexus for hematology and serum biochemistry analysis. The hematological evaluations included red blood cell count (RBC), neutrophil count (NEUT), lymphocyte count (LYMPH) and white blood cell count (WBC). Serum chemistry parameters included total protein (TP), albumin (ALB), alanine aminotransferase (ALT) and aspartate aminotransferase (AST). A complete necropsy was performed for each animal. During the necropsies, the organs of each animal, including the kidneys, liver and spleen, were weighed separately (Mettler-Toledo PL203; USA), and the viscera coefficient was calculated. Kidney, liver, spleen and intestine were preserved in 10% oxymethylene. After routine processing, 5 μm paraffin sections of the above organs were cut and stained with eosin for microscopic examination.
Ames test. The assays were modified according to the plate incorporation procedure described by Maron and Ames.29 The following solution concentrations were used: 4, 2, 1 and 0.5 mg mL−1. Experiments with all strains were conducted with and without the addition of S9 mix. Negative and positive controls were performed in parallel in each experiment. The positive control contained only the standard mutagen without the test substance, the solvent control included all the reagents except the test substance and the standard mutagen, and the negative control contained only the bacterial solution. The plate was mixed well and poured into the plate, and the result was observed after incubation at 37 °C for 48 h. The experiment was repeated three times, and the average was obtained. The test substance was considered mutagenic if it reproducibly induced a two-fold increase in the number of mutants with at least one concentration in strains TA97, TA98, TA100 and TA102, both compared to the corresponding negative controls. A dose-effect relationship was also considered as an indication of mutagenic potential.
Micronuclear test. Fifty SPF-grade Kunming mice were randomly divided into five groups, with 10 mice in each group (20–30 g), and reared in cages. Saponins were dissolved in purified water for the three experimental groups, the high-dose group (5.00 g kg−1), medium-dose group (2.50 g kg−1) and low-dose group (1.25 g kg−1), which received an 20 mL per kg per bw oral gavage. The negative control group was given the same volume of water by oral gavage, and the positive control group was given cyclophosphamide (CTX) at 40 mg kg−1 by intraperitoneal injection. The bone marrow was squeezed into one end of the glass slide with bovine serum. Then, another clean glass slide was used to completely mix the serum and bone marrow, the slide was quickly pushed and air dried. The slides were fixed with methanol for 15 min, stained with Giemsa for 15 min and washed with distilled water. The number of micronucleated polychromatic erythrocytes (MNPCEs) per 1000 polychromatic erythrocytes (PCEs) was analyzed by an automatic micronucleus analyzer. The cytotoxic index [PCEs/(PCEs + NCEs) (normochromatic erythrocytes)] of quinoa saponins was calculated based on the evaluation of 200 erythrocytes per animal.30
Mouse sperm aberration test. The mouse sperm aberration test was conducted according to OECD genetic toxicology guidance and Wang literature,31 and some steps were modified. Forty male mice were randomly divided into five groups by the random number method. The dose, gavage volume, and negative and positive controls were the same as in section acute toxicity study, and the animals were continuously exposed for 5 days. On the 30th day after exposure, the mice were sacrificed. After exposing the testicles, the mouse abdominal cavity was cut open. The bilateral epididymides of mice were collected and placed into a culture dish containing physiological saline, and then the epididymides were cut vertically by using ophthalmological scissors once or twice. The sperm suspension was squeezed onto a glass slide and pushed by another slide, air dried and fixed in methanol for 15 min. After air drying, the slide was stained with 1% eosin for 1 h and washed with distilled water. A microscope was used to observe the sperm after staining, and the number of sperm with no hook, a fat head, a folded tail, two heads, or two tails or that appeared banana-shaped or amorphous, which were considered abnormal sperm, per 1000 sperm, were counted, and the incidence of sperm deformity was calculated and analyzed by appropriate statistical methods.32 The criteria were a sperm deformity rate of the test substance that was a multiple of the negative control or a significant difference after statistical analysis and a dose–response relationship.
Study on the transformation of saponins in the rat intestine. Four Wistar rats (2 females and 2 males) were reared in each cage. Q3 (3-O-β-D-glucopyranosyl-(1→3)-α-L-arabinopyranosyl-phytolaccagenic acid 28-O-β-D-glucopyranosyl) was dissolved in purified water and orally administered at a dose of 10 mg kg−1 after acclimatization. Fecal samples were collected 0 h, 6 h, 24 h, 48 h, 72 h and 96 h after intragastric administration and dried at 60 °C. While conducting acute toxicity tests, fecal samples were collected twice daily at 14
:
00 and 20
:
00 on days 1–4 and stored at −40 °C until analysis.33 Fifty milligrams of the fecal sample was dissolved in 5 mL of 70% methanol in a centrifuge tube. The tube was kept in an ultrasonic for 30 min until dissolution and centrifuged for 10 min at 4000 rpm. Then, 5 mL of solution was placed into the test tube and blown with nitrogen at 60 °C until dried. After dissolving with 1 mL of methanol, the solution was filtered into the sample vial with a 0.45 μm filter membrane. The analysis was carried out according to the chromatographic conditions of reference,34 and the injection volume was 10 μL.
Statistical analysis. All data were analyzed by SPSS statistics 17.0 software. One-way analysis of variance (ANOVA) was used to compare the difference in population mean among multiple groups of samples with homogeneous variance. Data with heterogeneous variance in the micronuclear experiment and sperm malformation experiment were compared by two independent samples test. P < 0.05 or 0.01 was considered statistically significant. All data are presented as the mean ± standard deviation (std). The structural formulas were drawn with Chem Draw Ultra12.0 software and a nuclear magnetic map with MestReNova software was used for processing analysis.
Results
Separation and identification results
According to previous research, a large amount of crude saponins can be extracted from quinoa husks with a purity of 62.6%. Six kinds of saponins were obtained after separation, purification and HPLC/NMR identification (Fig. 2A): Q1, Q, Q3, Q4, Q5 and Q6. Q3 was mainly used in this experiment because its content was the highest. In addition, Q1 and Q5 were hederagenin saponins, and Q2, Q3, Q4 and Q6 were phytolaccagenic acid saponins. The structure of the Q1–Q6 monomer saponin is shown in Fig. 2B.
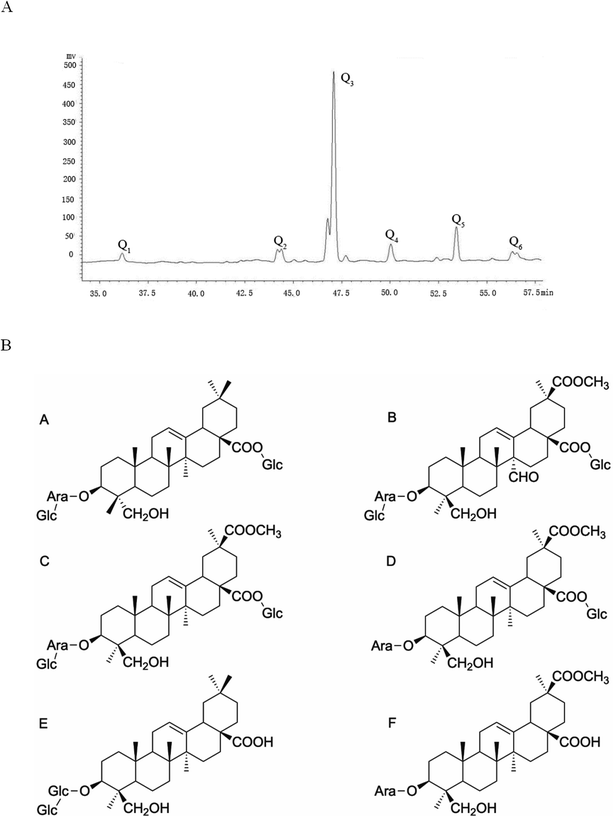 |
| Fig. 2 (A) Liquid phase map of saponins in Chenopodium quinoa husks. (B) (A) Monomer structure formula of Q1. (B) Monomer structure formula of Q2. (C) Monomer structure formula of Q3. (D) Monomer structure formula of Q4. (E) Monomer structure formula of Q5. (F) Monomer structure formula of Q6. | |
Acute toxicity
All male and female rats survived. It can be inferred that the LD50 of saponin was more than 10.0 g kg−1, which is limited acute toxicity effects. These results are in agreement with Verza and Ruiz.23,25 No signs of toxicity or behavioral changes were observed after the treatment with quinoa saponin. Only saponin at the ex high-dose (10.0 g kg−1) caused rough hair, depression and diarrhea. According to Table 2, quinoa saponins had an effect on the dietary amount, weight gain and food utilization rate of rats, which were statistically different compared with the control group.
Table 2 Acute oral toxicity of saponin on the food intake, body weight and food utilization rate in ratsa
Sex |
Saponin dose (g per kg per body weight) |
Food intake (g) |
Body weight (g) |
Food utilization rate (%) |
All data are means ± standard deviation (n = 5 in each group, **Significant difference at P < 0.01 level when compared with the control group, *Significant difference at P < 0.05 level when compared with the control group). |
Male |
0 |
444.00 ± 6.07 |
138.00 ± 10.66 |
31.06 ± 1.84 |
1 |
420.00 ± 7.32** |
106.00 ± 14.78* |
25.35 ± 2.88* |
2.15 |
410.00 ± 6.98** |
95.00 ± 9.66** |
23.14 ± 2.05** |
4.64 |
428.00 ± 7.57** |
108.00 ± 17.78* |
25.28 ± 4.39* |
10 |
375.00 ± 9.29** |
97.00 ± 13.57** |
25.68 ± 2.80* |
Female |
0 |
262.00 ± 4.56 |
67.00 ± 9.27 |
25.54 ± 3.09 |
1 |
247.00 ± 5.01* |
51.00 ± 3.81* |
20.63 ± 1.12* |
2.15 |
233.00 ± 7.68** |
44.00 ± 6.53** |
18.84 ± 2.18** |
4.64 |
242.00 ± 8.52* |
46.00 ± 0.57** |
19.02 ± 0.43** |
10 |
226.00 ± 13.10** |
44.00 ± 10.58** |
19.33 ± 3.57* |
Organ index and histopathological observation
No significant pathological changes in the colors and textures of vital organs, including the intestine, liver, spleen and kidney, were observed via macroscopic examination (Fig. 3). No treatment-related changes were observed in the absolute or relative liver and kidney weights of male or female rats. The spleen index was notably increased in the ex high-dose group compared with the control group for both sexes, and the difference was statistically significant (P < 0.05) (Table 3). However, no obvious changes were found during the histopathological examination. The difference in spleen coefficient may be due to the fact that Chenopodium quinoa saponins can enhance the immune function of animals and then cause immune cell proliferation, but it does not cause substantial damage to the structure of the spleen, so there is no obvious change in spleen sections. However, the difference of spleen coefficient only exists between the high dose group and the control group, and there is no obvious dose-effect relationship, and its biological significance needs to be further verified.
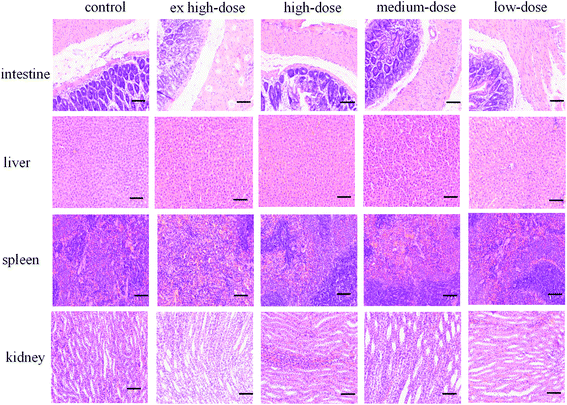 |
| Fig. 3 H&E staining of the intestine, liver, spleen and kidney (200×). Scale bar = 50 μm. | |
Table 3 Effect of quinoa saponin on organ index in rats (mg g−1)a
Gender |
Biochemical parameters (M ± SD) (%) |
Dose level |
Liver index |
Spleen index |
Kidney index |
**Significant difference at P < 0.01 level when compared with the control group. |
Male |
Control |
33.21 ± 1.97 |
1.72 ± 0.09 |
7.56 ± 0.47 |
Low-dose |
34.03 ± 2.65 |
1.69 ± 0.04 |
7.57 ± 0.53 |
Medium-dose |
31.47 ± 3.63 |
1.84 ± 0.13 |
7.45 ± 0.68 |
High-dose |
4.16 ± 2.47 |
1.87 ± 0.06 |
7.54 ± 0.48 |
Ex high-dose |
38.44 ± 2.39 |
2.11 ± 0.17** |
7.74 ± 0.50 |
Female |
Control |
30.47 ± 1091 |
2.33 ± 0.15 |
8.47 ± 0.36 |
Low-dose |
31.39 ± 2.63 |
2.21 ± 0.09 |
8.96 ± 0.42 |
Medium-dose |
29.70 ± 2.08 |
2.15 ± 0.07 |
8.50 ± 0.18 |
High-dose |
30.86 ± 1.63 |
2.20 ± 0.15 |
8.27 ± 0.07 |
Ex high-dose |
33.42 ± 1.80 |
2.78 ± 0.11** |
8.29 ± 0.12 |
Hematological and biochemical analysis
In the hematology analysis, a significant increase in NEUT% was found in female rats treated with different doses compared with control female rats (P < 0.05) (Table 4). Additionally, a significant decrease in LYMPH% was also observed in the female rats in the experimental groups compared with the control group (P < 0.05). No significant changes in hematology were observed between the experimental and control male rats. As shown in Table 5, the examination of the serum biochemistry of rats showed that the difference in ALT between each dose group and the control group was statistically significant for male rats (P < 0.05), while there was no statistically significant difference between each dose group. The AST of female rats administered 1 and 4.64 g kg−1 quinoa saponins was lower than that of the control female rats (P < 0.05) (Table 5).
Table 4 Hematology analysis of ratsa
|
Group |
WBC |
RBC |
NEUT% |
LYMPH% |
Values are presented as the mean ± SD (n = 10). *Significant difference at P < 0.05 level when compared with the control group. |
Male |
Control |
9.76 ± 1.24 |
7.78 ± 0.14 |
7.75 ± 1.51 |
89.46 ± 1.01 |
1 g kg−1 |
10.26 ± 0.94 |
7.41 ± 0.38 |
5.72 ± 0.34 |
90.71 ± 0.55 |
2.15 g kg−1 |
9.57 ± 2.15 |
7.94 ± 0.20 |
8.70 ± 1.05 |
88.62 ± 0.40 |
4.64 g kg−1 |
9.43 ± 1.25 |
7.73 ± 0.12 |
9.53 ± 1.53 |
86.47 ± 1.12 |
10 g kg−1 |
8.03 ± 2.10 |
7.42 ± 0.48 |
7.61 ± 1.84 |
86.58 ± 3.37 |
Female |
Control |
7.32 ± 0.20 |
8.26 ± 0.40 |
14.25 ± 0.95 |
92.52 ± 5.99 |
1 g kg−1 |
8.44 ± 0.49 |
7.82 ± 0.33 |
24.37 ± 1.24* |
76.83 ± 1.30* |
2.15 g kg−1 |
8.13 ± 0.29 |
7.81 ± 0.30 |
26.37 ± 4.98* |
77.84 ± 0.48* |
4.64 g kg−1 |
7.13 ± 0.55 |
8.01 ± 0.26 |
27.40 ± 0.85* |
78.27 ± 0.41* |
10 g kg−1 |
7.80 ± 1.01 |
7.53 ± 0.73 |
23.36 ± 0.15* |
77.74 ± 2.70* |
Table 5 Serum biochemical analysis of ratsa
|
Dose group |
ALT (U L−1) |
AST (U L−1) |
TP (g L−1) |
ALB (g L−1) |
Values are presented as the mean ± SD (n = 10). *Significant difference at P < 0.05 level when compared with the control group. |
Male |
Control |
48.42 ± 1.18 |
90.56 ± 7.98 |
54.53 ± 3.05 |
39.3 ± 2.75 |
1 g kg−1 |
41.27 ± 2.37* |
107.29 ± 14.18 |
51.52 ± 2.25 |
34.40 ± 1.81 |
2.15 g kg−1 |
38.36 ± 7.25* |
84.40 ± 2.05 |
49.36 ± 1.82 |
33.53 ± 1.63 |
4.64 g kg−1 |
39.30 ± 4.35* |
90.90 ± 10.75 |
54.50 ± 3.08 |
36.13 ± 2.24 |
10 g kg−1 |
37.17 ± 2.76* |
96.07 ± 0.87 |
51.97 ± 3.20 |
35.80 ± 2.95 |
Female |
Control |
31.68 ± 2.29 |
165.27 ± 14.74 |
48.37 ± 1.96 |
34.38 ± 2.37 |
1 g kg−1 |
31.41 ± 2.82 |
114.93 ± 10.11* |
48.27 ± 2.01 |
34.46 ± 2.48 |
2.15 g kg−1 |
35.50 ± 1.30 |
160.21 ± 19.82 |
49.40 ± 1.81 |
36.52 ± 2.38 |
4.64 g kg−1 |
32.50 ± 4.35 |
137.33 ± 5.03* |
46.16 ± 0.40 |
39.71 ± 0.72 |
10 g kg−1 |
32.11 ± 6.51 |
174.13 ± 3.36 |
46.37 ± 2.57 |
37.47 ± 1.82 |
Ames test
The test substance was considered mutagenic if it reproducibly induced a two-fold increase in the number of mutants with at least one concentration in strains TA97, TA98, TA100 and TA102, both compared to the corresponding negative controls. The results of the Ames test showed that the four doses of quinoa saponins were not mutagenic for the strains tested even in the presence of excretion activation. The specific results are shown in Fig. 4A. Taking strain TA102 as an example, the bacterial reversion effect is shown in Fig. 4B.
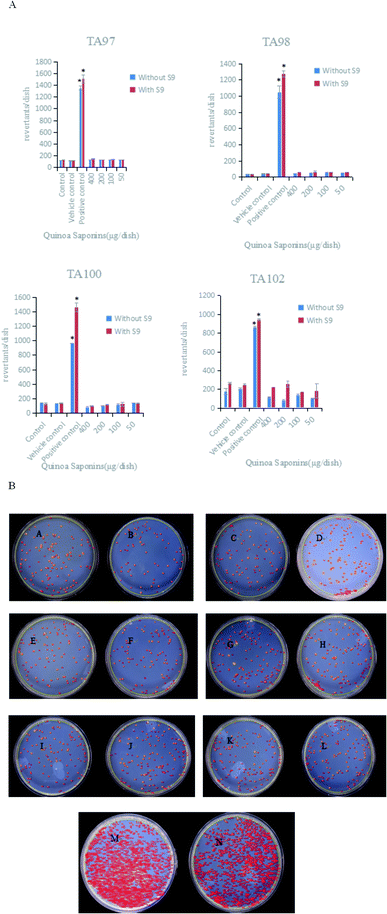 |
| Fig. 4 (A) Mean number of revertants induced by different doses of quinoa saponins and the control groups. TA97, TA98, TA100, TA102 strains of Salmonella typhimurium were used in these experiments. Values are expressed as the mean ± SD.*The number of revertants induced was two or more times higher than the number of revertants observed in the control and vehicle control groups. (B) Bacterial reversion mutation of TA102 strain in different dose groups (μg per dish). (A) 400 + S9; (B) 400 − S9; (C) 200 + S9; (D) 200 − S9; (E) 100 + S9; (F) 100 − S9; (G) 50 + S9; (H) 50 − S9; (I) control + S9; (J) control − S9; (K) vehicle control + S9; (L) vehicle control − S9; (M) positive control + S9; (N) positive control − S9. | |
Micronucleus test
To evaluate the mutagenic potential of quinoa saponins in bone marrow cells derived from Kunming mice, a micronucleus test was performed by determining the frequency of micronucleated polychromatic erythrocytes (MNPCEs) per 1000 polychromatic erythrocytes (PCEs) per animal. As shown in Table 6, there was no significant increase in the frequencies of MNPCEs at all test doses of quinoa saponins compared with the negative control. CTX, a positive control, significantly increased the frequency of MNPCEs, as expected. The cytotoxic index [PCEs/(PCEs + NCEs)] of quinoa saponins ranged from 29% to 42% at all test doses, which was comparable to those of the negative and positive control treatments. Fig. 5 shows the results of mice bone marrow erythrocyte micronucleus study.
Table 6 Summary of the micronucleus test of the quinoa saponins in Kunming mice (mean ± SD)a
Group (mg kg−1) |
PCE/(PCE + NCE) (‰) |
MNPCE/1000 PCEs (%) |
PCE, polychromatic erythrocyte; NCE, normochromatic erythrocyte; MNPCE, micronucleated polychromatic erythrocyte. *P < 0.05 vs. the negative control group. |
1250 |
29.04 ± 0.02 |
5.36 ± 0.86 |
2500 |
39.27 ± 0.01 |
7.67 ± 1.67 |
5000 |
42.96 ± 0.21 |
7.55 ± 2.79 |
Positive control |
39.59 ± 0.23 |
24.30 ± 2.80* |
Negative control |
48.13 ± 0.08 |
6.04 ± 1.19 |
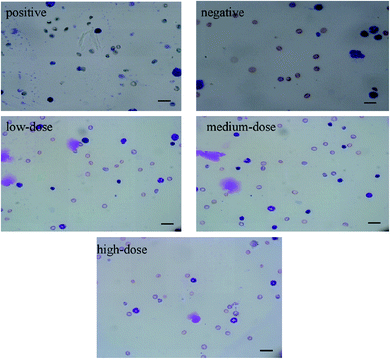 |
| Fig. 5 Polychromatic erythrocytes with micronucleus images in each groups (400×). Scale bar = 50 μm. | |
Mouse sperm aberration test
As shown in Fig. 6A, the difference in the sperm aberration rate between the negative control group and the positive control group (40 mg per kg per bw CTX) was statistically significant (P < 0.01). The sperm aberration rate in all treatment groups and the negative control group was significantly lower than that in the positive control group, indicating that the saponins at the doses above did not result in abnormal sperm morphology. The sperm sections of each dose group are shown in the Fig. 6B.
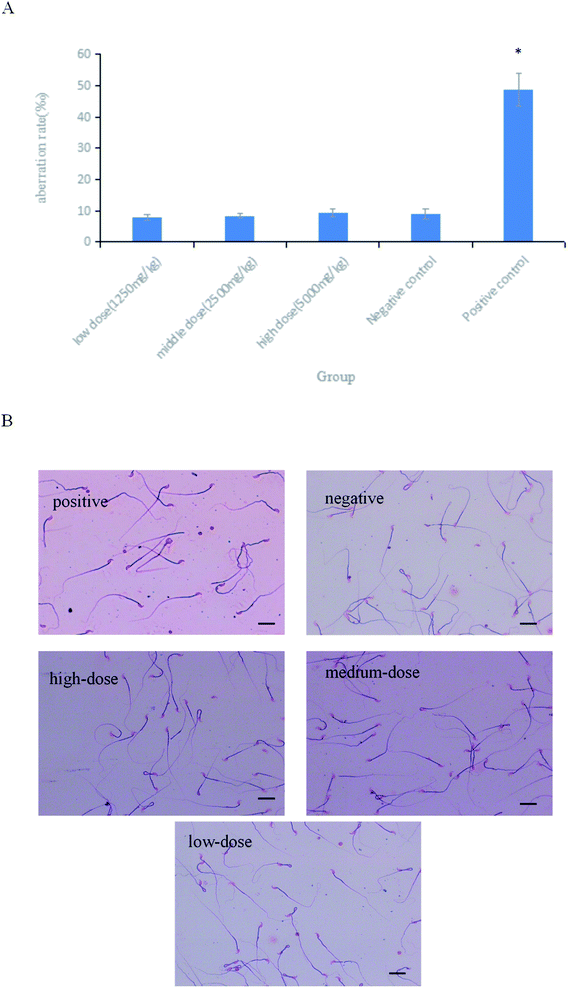 |
| Fig. 6 (A) Results of sperm aberration assay in mice.*P < 0.01 vs. negative control. (B) Micrographs of sperm aberration in each group (400×). Scale bar = 50 μm. | |
Analysis of intestinal excretion products in rats
As shown in Fig. 7, the saponin content increased from days 1–3 in both sexes after intragastric administration. On day 3, saponins reached the highest level in the rats. There was a significant decrease in the saponin content of rats at day 4, and the content of excreted saponins was less than 0.01 μg g−1, which is equivalent to all saponins excreted from the body. As shown in Fig. 8(A and B), the low-dose saponins were absorbed and excreted by rats at 6 h, and almost all saponins were excreted after 24 h (the red part in Fig. 8B shows the peak time of saponins). The initial cytoplasmic ratio (m/z = 971.49 [M − H]−) of Q3 was analyzed by mass spectrometry. After a series of cleavages and absorption in rats, two molecules of glucose and one molecule of arabinose were removed. After removing the sugar chain, this was the mother nuclear structure of phytolaccagenic acid saponins. The m/z values were 516.34 [M], 515.34 [M − H]− and 517.34 [M + H]+ (Fig. 8D). Therefore, the 14.97 min peak was identified as the ion peak of saponins.
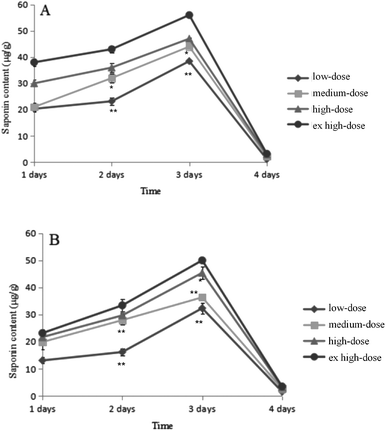 |
| Fig. 7 The changes of saponin content in rats. (A) Male rats; (B) female rats. Data are presented as the mean ± SD; *P < 0.05, **P < 0.01 vs. the low-dose group. | |
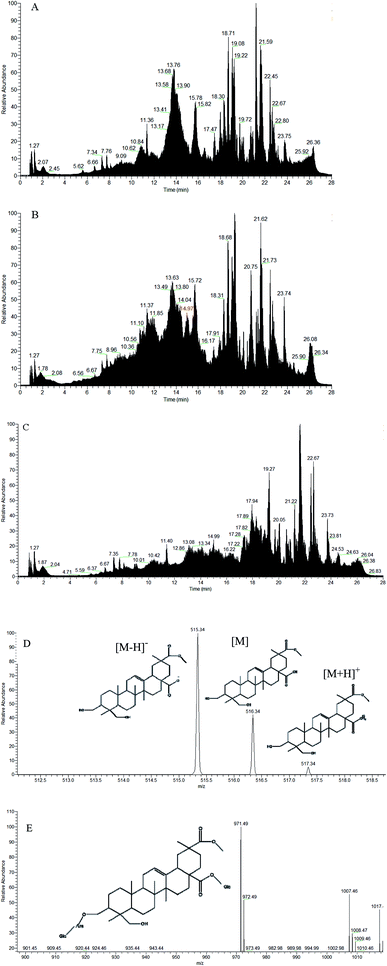 |
| Fig. 8 (A) TIC diagram of the content of saponins in the feces of 0 h rats after intragastric administration; (B) TIC diagram of the content of saponins in the feces of 6 h rats after intragastric administration; (C) TIC diagram of the content of saponins in the feces of 24 h rats after intragastric administration; (D) ion fragments of saponins in 6 h feces in negative mode; (E) ion fragments of Q3 in negative mode. | |
Discussion
As an anti-nutritional substance, saponins can resist the harm of external adverse factors. However, these bitter ingredients may interfere with the digestion and absorption of various nutrients.35 Additionally, saponins have many activities that can be used in the fields of food, pharmaceuticals and cosmetics.36 Recent studies have shown that the saponins in quinoa can inhibit Pomacea maculata, Candida albicans and other organisms.37,38 In addition, some researchers have used quinoa saponin extracts as a biological pesticide to control the reproduction of Oncomelania hupensis.25 In this experiment, the content of crude saponins extracted from quinoa was mainly phytolaccagenic acid-type (PA) saponins. This type of saponin has extreme bactericidal and bacteriostatic effects on Gram-positive bacteria such as S. aureus, B. cereus and S. epidermidis, which mainly damage fungal membrane integrity by connecting steroids of membranes.
In the acute toxicity evaluation, Horn's method was used to estimate the LD50 of quinoa saponins in rats.28 Four different doses of quinoa saponins (1.00, 2.15, 4.64, and 10.0 g per kg per bw) were administered orally to both males and females. It is generally accepted that substances with LD50 values greater than 5.00 g kg−1 by the oral route can be considered practically nontoxic (Table 1). The present experimental results showed that the LD50 of quinoa saponins was more than 10.0 g kg−1, making them limited acute toxicity effects. However, quinoa saponins may affect the daily eating of rats. It was presumed that the reason might be the bitter taste of saponins. After the rats are administered quinoa saponins by gavage, they may eat less in a period due to stomach discomfort. In addition, saponins can cause diarrhea and affect the food and water intake and food utilization of rats (Table 2). Moreover, previous reports showed that soya saponins can induce enteritis in Atlantic salmon.39 We speculated that saponins may have a certain irritating effect on the intestinal tract, so we performed excretion experiments. The results show that saponins were almost completely excreted on the fourth day, and there were very few saponins in vivo (Fig. 7). Combined with the daily observations during the acute toxicity test, these results show that saponins can stimulate the intestinal tract to a certain extent, but not for a long time. As long as the intake of saponins is controlled, saponins will not have a very large effect on the intestinal tract.
Rats were intragastrically administered saponins, and fecal samples were collected. As evidenced by pretreatment and mass spectrometry analysis, the metabolites of saponins were found in feces after 6 h. Combined with mass spectrometry information, it can be judged that the sugar chain was removed from the saponins. Interestingly, it is known that large doses of saponins will remain in the body for 4 days after intragastric administration, while small doses of saponins will be hydrolyzed into sapogenins and excreted from the body (Fig. 7 and Fig. 8). Because saponins are poorly absorbed and reside for a long time in the intestinal tract, when a large amount of saponins enter the body, very little is absorbed by the small intestine; thus, saponins accumulate and slowly degrade in the colon. However, small doses of saponins are converted into sapogenin by intestinal bacteria and metabolic enzymes in the intestine.40,41 Sapogenin is a low polar saponins and is highly irritating to the intestines, so eating large amounts of saponins can lead to diarrhea,42 which is in concordance with previous reports about saponins toxicity.43
The organ coefficient, also known as the relative weight of organs, is an important indicator of animal physiology and pathology.44,45 In the current study, saponins changed the organ index of some rat organs, especially the spleen organ index, which was significantly different in the saponin-treated group compared with the control group (P < 0.01) (Table 3). However, no obvious pathological or histological changes were observed (Fig. 3), indicating that quinoa saponins did not cause substantial damage to the viscera of the rats.
Hematological and serum biochemical examination results can be used to evaluate the damage to organs and tissues, especially to the liver, caused by drugs or chemicals.46 Current research showed that compared with the control condition, quinoa saponin treatment could cause statistically significant changes (P < 0.05) in NEUT% and LYMPH% in female rats (Table 4). However, these variations had no biological significance because the observed values were within the normal range of the species.
The liver is the main organ involved in the metabolism of endogenous and exogenous substances and is the location of cholesterol synthesis and degradation. ALT and AST are sensitive indicators of liver cell damage.47 In the present investigation, the ALT level of male saponin-treated rats and the AST level of female saponin-treated rats at doses of 1 and 4.64 g per kg per bw were significantly different from those of the control groups (P < 0.05) (Table 5). However, there were no abnormalities in liver histopathology, and it was assumed that quinoa saponins did not produce toxicological reactions to liver tissue and liver function.
Assessment of genotoxic potential is very important for food, pharmaceutical and chemical safety since classic genotoxic substances result in carcinogenicity and fertility defects.48,49 In this study, the Ames test, micronucleus test and sperm aberration assay were used to evaluate the genotoxicity of quinoa saponin.
The Ames test is the most widely used method for detecting gene mutations, and it is the first choice in a group of experiments for detecting environmental mutagens.50 This test is usually employed as a preliminary screen to determine the mutagenic potential of new chemicals and drugs, especially their ability to induce point mutations, including the substitution, addition or deletion of one or more DNA base pairs.51 In the current test, the number of revertants in all treatment groups did not reach twice as high as that in the negative control group among the four bacterial strains with and without S9 (Fig. 4A). The results demonstrated that quinoa saponins did not cause gene mutations in the tested bacterial strains. Considering that the study of DNA damage at micronuclei formation is a vital part of genetic toxicity screening, we then performed mouse micronucleus test.52 Chromosome fragments without centromeres or the loss of the whole chromosome due to spindle damage will become micronuclei in the cytoplasm during anaphase in cell division. In this study, in bone marrow cells from the femurs of Kunming mice, there was no significant or dose-related increase in the amount of MNPCE in the quinoa saponin treatment groups at any dose. In addition, the ratio of PCEs/(PCEs + NCEs), a cytotoxic index, was not significantly different between the treatment and control groups (Table 6). The results revealed that quinoa saponins did not induce micronuclei in mice. Some saponins have been demonstrated to be lack of mutagenic effects.53 In the sperm aberration assay in mice, even when the dose of quinoa saponin reached 10 mg per kg per bw, no significant difference in the sperm malformation rate was observed from the negative control. The results showed that quinoa saponins did not induce sperm malformation. To date, there have not been systematic toxicological studies on quinoa saponin. However, the safety of the saponins extracted from the Bamboo rhizoa and tuber of Liriope muscari was evaluated. Both the saponins and steroidal saponins showed limited acute toxicity effects and genetic safety,54,55 which was consistent with the results of this study.
As an anti-nutritional factor, previous studies focused on the removal of saponins and technology to extract saponins and their biological activity. Chenopodium saponins, as triterpenoid saponins with amphiphilic activity, can stimulate the digestive tract at high dosages. In addition, the excretion time of Chenopodium saponins in the body is long. Therefore, people with digestive tract diseases should pay attention to the content of dietary saponins, and adequate safety evaluations should be conducted before the widespread use of quinoa saponins. In this study, the preliminary safety evaluation of Chenopodium saponins showed that it was a limited acute toxicity effects plant active ingredient, and no mutagenesis was found under the current experimental conditions. It can be further developed and utilized to make it a new type of resource. However, other toxicological tests still need to further confirm the safety of long-term intake.
Informed consent
Clinical experiments were not included in this study and there is no informed consent involved.
Conflicts of interest
The authors declare that they have no conflict of interest performed by any of the authors.
Acknowledgements
This work was supported by the Doctoral research start-up funds of Weifang Medical University and project No. ZR2019PH043 supported by Natural Science Foundation of Shandong Province. We would like to thank the Quinoa Saponin Toxicology Research Project from the Institute of Crop Science, Chinese Academy of Agricultural Sciences and Shandong Provincial Youth Innovation Team Development Plan of Colleges and Universities (No. 2019-6-156, Lu-Jiao). We would also like to thank the Public Health Testing Center of the Weifang Medical University and Weifang Key Laboratory for Food Nutrition and Safety for providing us with the experimental platform and technical guidance.
References
- J. G. Lim, H. M. Park and K. S. Yoon, Analysis of saponin composition and comparison of the antioxidant activity of various parts of the quinoa plant (Chenopodium quinoa Willd), Food Sci. Nutr., 2019, 8(1), 694–702 CrossRef.
- M. Pellegrini, R. L. Gonzales, A. Ricci, J. Fontecha, F. J. López and J. P. Alvarez, et al., Chemical, fatty acid, polyphenolic profile, techno-functional and antioxidant properties of flours obtained from quinoa (Chenopodium quinoa Willd) seeds, Ind. Crops Prod., 2018, 111, 38–46 CrossRef CAS.
- E. Pereira, C. E. Zelada, L. Barros, U. G. Barron, V. Cadavez and I. Ferreira, Chemical and nutritional characterization of Chenopodium quinoa Willd (quinoa) grains: a good alternative to nutritious food, Food Chem., 2019, 280, 110–114 CrossRef CAS.
- A. M. Filho, M. R. Pirozi and J. T. Borges, Sant'Ana HM, Chaves JB and Coimbra JS, Quinoa: nutritional, functional, and antinutritional aspects, Crit. Rev. Food Sci., 2017, 57(8), 1618–1630 CrossRef.
- D. E. Jarvis, Y. S. Ho, D. J. Lightfoot, S. M. Schmöckel, B. Li and T. J. Borm, et al., The genome of Chenopodium quinoa, Nature, 2017, 542(7641), 307–312 CrossRef CAS.
- J. F. Jurado, J. Pollier, T. Moses, P. Arend, N. B. Medina and E. Morillo, et al., Saponin determination, expression analysis and functional characterization of saponin biosynthetic genes in Chenopodium quinoa leaves, Plant Sci., 2016, 250, 188–197 CrossRef.
- S. N. Varli and N. Sanlier, Nutritional and health benefits of quinoa (Chenopodium quinoa willd.), J. Cereal Sci., 2016, 69, 371–376 CrossRef.
- M. Ayyash, S. K. Johnson, S. Q. Liu, A. A. Mheiri and A. Abdhelaibi, Cytotoxicity, antihypertensive, antidiabetic and antioxidant activities of solid-state fermented lupin, quinoa and wheat by Bifidobacterium species: in vitro investigations, LWT, 2018, 95, 295–302 CrossRef CAS.
- G. T. Li and F. Zhu, Quinoa starch: structure, properties, and applications, Carbohydr. Polym., 2018, 181, 851–861 CrossRef CAS.
- G. T. Li, S. N. Wang and F. Zhu, Physicochemical properties of quinoa starch, Carbohydr. Polym., 2016, 137, 328–338 CrossRef CAS.
- D. Bazile, S. E. Jacobsen and A. Verniau, The Global Expansion of Quinoa: Trends and Limits, Front. Plant Sci., 2016, 7, 622 Search PubMed.
- X. Y. Sun, X. S. Yang, P. Xue, Z. G. Zhang and G. X. Ren, Improved antibacterial effects of alkali-transformed saponin from quinoa husks against halitosis-related bacteria, BMC Complementary Altern. Med., 2019, 19(1), 46 CrossRef.
- J. Nickel, L. P. Spanier, F. T. Botelho, M. A. Gularte and E. Helbig, Effect of different types of processing on the total phenolic compound content, antioxidant capacity, and saponin content of Chenopodium quinoa Willd grains, Food Chem., 2016, 209, 139–143 CrossRef CAS.
- M. A. Lazo-Vélez, D. Guajardo-Flores, D. Mata-Ramírez, J. A. Gutiérrez-Uribe and S. O. Serna-Saldivar, Characterization and Quantitation of Triterpenoid Saponins in Raw and Sprouted Chenopodium berlandieri spp. (Huauzontle) Grains Subjected to Germination with or without Selenium Stress Conditions, J. Food Sci., 2016, 81(1), C19–C26 CrossRef.
- I. G. Medina-Meza, N. A. Aluwi, S. R. Saunders and G. M. Ganjyal, GC-MS Profiling of Triterpenoid Saponins from 28 Quinoa Varieties (Chenopodium quinoa Willd.) Grown in Washington State, J. Agric. Food Chem., 2016, 64(45), 8583–8591 CrossRef CAS.
- P. Xue, L. Zhao, Y. J. Wang, Z. H. Hou, F. X. Zhang and X. S. Yang, Reducing the damage of quinoa saponins on human gastric mucosal cells by a heating process, Food Sci. Nutr., 2019, 8(1), 500–510 CrossRef.
- Y. M. Han, J. W. Chi, M. W. Zhang, R. F. Zhang, S. H. Fan and L. H. Dong, et al., Changes in saponins, phenolics and antioxidant activity of quinoa (Chenopodium quinoa willd) during milling process, LWT, 2019, 114, 108381 CrossRef CAS.
- F. Abderrahim, E. Huanatico, R. Segura, S. Arribas, M. C. Gonzalez and L. Condezo-Hoyos, Physical features, phenolic compounds, betalains and total antioxidant capacity of coloured quinoa seeds (Chenopodium quinoa Willd.) from Peruvian Altiplano, Food Chem., 2015, 183, 83–90 CrossRef CAS.
- Y. C. Hu, J. M. Zhang, L. Zou, C. M. Fu, P. Li and G. Zhao, Chemical characterization, antioxidant, immune-regulating and anticancer activities of a novel bioactive polysaccharide from Chenopodium quinoa seeds, Int. J. Biol. Macromol., 2017, 99, 622–629 CrossRef CAS.
- S. H. Fan, J. N. Li and B. Q. Bai, Purification, structural elucidation and in vivo immunity-enhancing activity of polysaccharides from quinoa (Chenopodium quinoa Willd.) seeds, Biosci., Biotechnol., Biochem., 2019, 83(12), 2334–2344 CrossRef CAS.
- Y. Yao, Y. Y. Zhu, Y. Gao, Z. X. Shi, Y. B. Hu and G. X. Ren, Suppressive effects of saponin-enriched extracts from quinoa on 3T3-L1 adipocyte differentiation, Food Funct., 2015, 6(10), 3282–3290 RSC.
- Y. Tang and R. Tsao, Phytochemicals in quinoa and amaranth grains and their antioxidant, anti-inflammatory, and potential health beneficial effects: a review, Mol. Nutr. Food Res., 2017, 61(7), 1–39 CrossRef.
- S. G. Verza, F. Silveira, S. Cibulski, S. Kaiser, F. Ferreira and G. Gosmann, et al., Immunoadjuvant activity, toxicity assays, and determination by UPLC/Q-TOF-MS of triterpenic saponins from Chenopodium quinoa seeds, J. Agric. Food Chem., 2012, 60(12), 3113–3118 CrossRef CAS.
- J. Escribano, J. Cabanes, M. Jiménez-Atiénzar, M. Ibañez-Tremolada, L. R. Gómez-Pando and F. García-Carmona, et al., Characterization of betalains, saponins and antioxidant power in differently colored quinoa (Chenopodium quinoa) varieties, Food Chem., 2017, 234, 285–294 CrossRef CAS.
- M. C. Ruiz, H. C. Jones, H. Schlotterbeck, M. A. Lopze, A. Tomas and R. S. Martin, Safety and efficacy of quinoa (Chenopodium quinoa) saponins derived molluscicide to control of pomacea maculata in rice fields in the ebro delta, spain, Crop Prot., 2018, 111, 42–49 CrossRef.
- S. X. Dong, X. S. Yang, L. Zhao, F. X. Zhang, Z. H. Hou and P. Xue, Antibacterial activity and mechanism of action saponins from Chenopodium quinoa Willd. husks against foodborne pathogenic bacteria, Ind. Crops Prod., 2020, 149, 112350 CrossRef CAS.
- F. J. Li, X. L. He, W. Y. Niu, Y. N. Feng, J. Q. Bian and H. B. Xiao, Acute and sub-chronic toxicity study of the ethanol extract from leaves of Aralia elata in rats, J. Ethnopharmacol., 2015, 175, 499–508 CrossRef.
- H. J. Horn, Simplified LD50 (or ED50) calculations, Biometrics, 1956, 12, 311–322 CrossRef.
- D. M. Maron and B. N. Ames, Revised methods for the Salmonella mutagenicity test, Mutat. Res., 1983, 113(3–4), 173–215 CAS.
- L. H. Deng, J. D. Lei, J. He, J. Liu, L. Y. Wang and R. Zhang, et al., Evaluation on genotoxicity and teratogenicity of aqueous extract from Cyclocarya paliurus leaves, Sci. World J., 2014, 498134 Search PubMed.
- J. Z. Wang, F. F. Sun, S. S. Tang, S. X. Zhang, J. Li and X. Y. Cao, Mutagenicity and teratogenicity studies of vitacoxib in rats and mice, Toxicol. Rep., 2018, 5, 827–831 CrossRef CAS.
- J. Z. Wang, F. F. Sun, S. S. Tang, S. X. Zhang and X. Y. Cao, Acute, mutagenicity, teratogenicity and subchronic oral toxicity studies of diaveridine in rodents, Environ. Toxicol. Pharmacol., 2015, 40(2), 660–670 CrossRef CAS.
- E. Bamberg, R. Palme and J. G. Meingassner, Excretion of corticosteroid metabolites in urine and
faeces of rats, Lab. Anim., 2001, 35(4), 307–314 CrossRef CAS.
- L. Zhao, K. Y. Ding, X. Zheng, F. Z. Xu, P. Xue and F. X. Zhang, Bacteriostatic and tyrosinase inhibitory activities of different polar sites from quinoa bran, Sci. Technol. Food Ind., 2019, 40, 82–88 Search PubMed.
- D. Suárez-Estrella, L. Torri, M. A. Pagani and A. Marti, Quinoa bitterness: causes and solutions for improving product acceptability, J. Sci. Food Agric., 2018, 98(11), 4033–4041 CrossRef.
- F. X. Zhang, S. J. Tang, L. Zhao, X. S. Yang, Y. Yao and Z. H. Hou, et al., Stem-leaves of Panax as a rich and sustainable source of less polar ginsenosides: comparison of ginsenosides from Panax ginseng, American ginseng and Panax notoginseng prepared by heating & acid treatment, J. Ginseng Res., 2021, 45(1), 163–175 CrossRef.
- Y. Yang, S. Laval and B. Yu, Chemical synthesis of saponins, Adv. Carbohydr. Chem. Biochem., 2014, 71, 137–226 CrossRef.
- G. M. Woldemichael and M. Wink, Identification and biological activities of triterpenoid saponins from Chenopodium quinoa, J. Agric. Food Chem., 2001, 49(5), 2327–2332 CrossRef CAS.
- A. Krogdahl, K. Gajardo, T. M. Kortner, M. Penn, M. Gu and G. M. Berge, et al., Soya Saponins Induce Enteritis in Atlantic Salmon (Salmo salar L.), J. Agric. Food Chem., 2015, 63(15), 3887–3902 CrossRef CAS.
- W. W. Dong, F. L. Xuan, F. L. Zhong, J. Jiang, S. Wu and D. Li, et al., Comparative Analysis of the Rats' Gut Microbiota Composition in Animals with Different Ginsenosides Metabolizing Activity, J. Agric. Food Chem., 2017, 65(2), 327–337 CrossRef CAS.
- J. Y. Wan, P. Liu, H. Y. Wang, L. W. Qi, C. Z. Wang and P. Li, et al., Biotransformation and metabolic profile of American ginseng saponins with human intestinal microflora by liquid chromatography quadrupole time-of-flight mass spectrometry, J. Chromatogr. A, 2013, 1286, 83–92 CrossRef CAS.
- J. N. D. Hierro, T. Herrera, T. Fornari, G. Fornari and D. Martin, The gastrointestinal behavior of saponins and its significance for their bioavailability and bioactivities, J. Funct. Foods, 2018, 40, 484–497 CrossRef.
- M. Stauss-Grabo, S. Atiye, A. Warnke, R. S. Wedemeyer, F. Donath and H. H. Blume, Observational study on the tolerability and safety of film-coated tabletscontaining ivy extract (Prospa Cough Tablets) in the treatment of colds accompanied by coughing, Phytomedicine, 2011, 18, 433–436 CrossRef CAS.
- P. Raina, C. V. Chandrasekaran, M. Deepak, A. Agarwal and K. G. Ruchika, Evaluation of subacute toxicity of methanolic/aqueous preparation of aerial parts of O. sanctum in Wistar rats: clinical, haematological, biochemical and histopathological studies, J. Ethnopharmacol., 2015, 175, 509–517 CrossRef CAS.
- Y. Zhang, K. Zhu, H. Cui, Y. Liu, Y. F. Lu and H. W. Pan, et al., Toxicological evaluation of Oviductus ranae: acute, sub-acute and genotoxicity studies in mice and rats, J. Ethnopharmacol., 2017, 203, 101–109 CrossRef.
- H. Olson, G. Betton, D. Robinson, K. Thomas, A. Monro and G. Kolaja, et al., Concordance of the toxicity of pharmaceuticals in humans and in animals, Regul. Toxicol. Pharmacol., 2000, 32(1), 56–67 CrossRef CAS.
- R. Ren, Y. M. Ma, W. S. Ma and S. M. Lu, The Assessment of Liver Reserve Function by Spectrophotometry based on Determination of Phenacetin and Paracetamol, Clin. Lab., 2015, 61(12), 1927–1934 CAS.
- N. Singh, B. Manshian, G. J. Jenkins, S. M. Griffiths, P. M. Williams and T. G. Maffeis, et al., NanoGenotoxicology: the DNA damaging potential of engineered nanomaterials, Biomaterials, 2009, 30(23–24), 3891–3914 CrossRef CAS.
- S. Shin, J. M. Yi, N. S. Kim, C. S. Park, S. H. Kim and O. K. Bang, Aqueous extract of Forsythia viridissima fruits: acute oral toxicity and genotoxicity studies, J. Ethnopharmacol., 2020, 249, 112381 CrossRef CAS.
- G. Raitanoa, A. Roncaglionia, A. Manganaroa, M. Honma, L. Sousselier and T. Q. Do, et al., Integrating in silico models for the prediction of mutagenicity (Ames test) ofbotanical ingredients of cosmetics, Comput. Toxicol., 2019, 12, 100108 CrossRef.
- A. Özkara, Assessment of cytotoxicity and mutagenicity of insecticide Demond EC25 in Allium cepa and Ames Test, International Journal of Cytology, Cytosystematics and Cytogenetics, 2019, 72(2), 21–27 Search PubMed.
- J. Ashby, Is there a continuing role for the intraperitoneal injection route of exposure in short-term rodent genotoxicity assays?, Mutat. Res., 1985, 156(3), 239–243 CAS.
- S. Ma, J. Kou and B. Yu, Safety evaluation of steroidal saponin DT-13 isolated from the tuber of Liriope muscari (Decne.) Baily, Food Chem. Toxicol., 2011, 49(9), 2243–2251 CrossRef CAS.
- Y. Zhang, X. Q. Wu, Y. P. Ren, J. Y. Fu and Y. Zhang, Safety evaluation of a triterpenoid-rich extract from bamboo shavings, Food Chem. Toxicol., 2004, 42(11), 1867–1875 CrossRef CAS.
- S. T. Ma, J. P. Kou and B. Y. Yu, Safety evaluation of steroidal saponin DT-13 isolated from the tuber of Liriope muscari (Decne.) Baily, Food Chem. Toxicol., 2011, 49(9), 2243–2251 CrossRef CAS.
Footnote |
† These authors have contributed equally to this work. |
|
This journal is © The Royal Society of Chemistry 2021 |
Click here to see how this site uses Cookies. View our privacy policy here.