DOI:
10.1039/D0RA09621K
(Paper)
RSC Adv., 2021,
11, 1654-1661
Amino acid ester-coupled caffeoylquinic acid derivatives as potential hypolipidemic agents: synthesis and biological evaluation†
Received
12th November 2020
, Accepted 21st December 2020
First published on 5th January 2021
Abstract
Pandanus tectorius (L.) Parkins. (PTPs) is rich in caffeoylquinic acids and amino acids, especially some essential amino acids, such as valine, phenylalanine, and so forth. A series of novel amino acid ester-coupled caffeoylquinic acid derivatives have been designed and synthesized. Biological evaluation suggested that some amino acid ester-coupled derivatives exhibited varying degrees of lipid-lowering effects on oleic acid-elicited lipid accumulation in HepG2 liver cells. Particularly, derivatives 6c, 6d, 6e and 6f exhibited comparable potential lipid-lowering effect with the positive control simvastatin and chlorogenic acid. Further studies on the mechanism of 6c, 6d, 6e and 6f revealed that the lipid-lowering effects were related to their regulation of TG levels and mRNA levels of lipometabolic-modulating genes, and merit further investigation.
Introduction
Hyperlipidemia, one of the most common metabolic and endocrine disorder diseases, is characterized by the decreasing concentration of high density lipoprotein cholesterol (HDL-C) and increasing concentration of triglycerides (TG), total cholesterol (TC), and low density lipoprotein cholesterol (LDL-C).1–4 It is well known that hyperlipidemia is one of the key risk factors for cerebral and cardiovascular diseases, and the increasing levels of TG show a significant influence on coronary heart disease (CHD) and atherosclerosis (AS).5–7 Several studies have provided evidence that sterol-regulatory element binding protein 1 (SREBP1) is involved in low-density lipoprotein gene transcription and sterol synthesis, and acetyl-CoA carboxylase (ACC) and fatty acid synthase (FASN) are responsible for fatty acid synthesis; these three genes are all associated with lipid synthesis.8–11 Therefore, it is quite beneficial to research and explore anti-hypolipidemic agents that could modulate the dysregulation of lipid metabolism, and decrease the elevated levels of serum TG, the gene SREBP1, ACC, and FASN.
Pandanus tectorius (L.) Parkins. (PTPs, Fig. 1), shaped like a pineapple, is rich in phenolic acids and amino acids, and often used as a traditional medicine in the folk for treating leprosy, bronchitis, measles, dermatitis and diabetes diseases.12–14 The content of amino acids in PTPs fruit is as high as 10.5 μg g−1, including seven high content of essential amino acids, such as leucine (1.0 μg g−1), valine (0.8 μg g−1), phenylalanine (0.5 μg g−1) and so on. These essential amino acids, which must be supplied by food, participate in many physiological functions of the human body.14 As the ester of caffeic acid and quinic acid, chlorogenic acid (3-O-caffeoylquinic acid, CA, 1, Fig. 1) was also found abundantly in PTPs. CA was reported to have multiple potential activities, including antioxidant, anti-inflammatory, antimicrobial, and anti-hyperglycemic effects.15–18 Our previous researches revealed that the caffeoylquinic acid analogues extracted from PTPs were confirmed to possess obvious anti-hyperlipidemia activity, and could significantly inhibit lipid accumulation and TG levels in the liver.13,19 In addition, we also found that CA derivatives (chlorogenic acid diketal, 2, Fig. 1) could decrease lipid accumulation in HepG2 cells, with the ketal substituent on the 1,4,5-position hydroxyl and carboxyl group.20 Recently, our preliminary SARs of chlorogenic acid suggested that the phenolic hydroxyl, not the carboxyl group, on the caffeic acid moiety is vital for the lipid-lowering activity of CA, and CA amide derivatives (3 and 4, Fig. 1) exhibited more potential lipid-lowering effect than the positive control simvastatin and chlorogenic acid.20,21 However, despite the anticipated pharmacological properties of caffeoylquinic acid amide analogues, synthesis and investigation of the novel amino acid ester-coupled caffeoylquinic acid derivatives lipid-decreasing effects has never been researched yet.
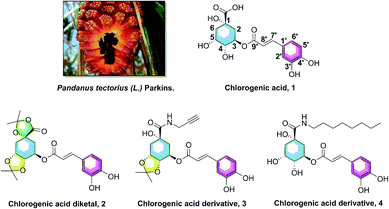 |
| Fig. 1 The Pandanus tectorius (L.) Parkins. fruit and the structures of chlorogenic acid (1), and its active analogues chlorogenic acid diketal (2), derivatives 3 and 4. | |
Considering of the high content of essential amino acids in PTPs fruit and the difference structures of amino acids, herein, we choose six kind of ethyl amino acid esters as the coupling groups (glycine, alanine, valine, leucine, phenylalanine, and tryptophan ethyl esters) which were coupled to the carboxyl group on 1-position of chlorogenic acid, and report the synthesis of twelve novel amino acid ester-coupled caffeoylquinic acid derivatives with various amino acid esters. Meanwhile, in consideration of diketal analogues of chlorogenic acid attenuated lipid accumulation, we design the derivatives with 4,5-position hydroxyl groups substituted by ketal (isopropylidene). Therefore, this research was aim to synthesize novel amino acid ester-coupled caffeoylquinic acid derivatives (5a–f, 6a–f, Fig. 2) and investigate the effects of derivatives on oleic acid-elicited lipid accumulation in HepG2 cells. The underlying lipid-lowering effect mechanisms of the amino acid ester-coupled derivatives were also elucidated by intracellular TG quantification and quantitative real-time PCR.
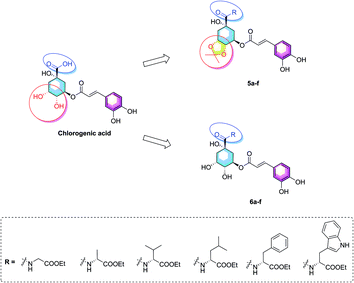 |
| Fig. 2 The structures of amino acid ester-coupled caffeoylquinic acids derivatives. | |
Results and discussion
Chemistry
The synthesis of amino acid ester-coupled caffeoylquinic acids derivatives 5a–f and 6a–f were outlined in Scheme 1. Chlorogenic acid (1) was treated with dimethyoxypropane (DMP) and p-toluenesulfonic acid (TsOH) in dry acetone to gain chlorogenic acid ketal the intermediate 7. Then the target products 5a–f were attained via amidation with various amino acid ethyl esters hydrochloride (glycine ethyl ester hydrochloride, L-alanine ethyl ester hydrochloride, L-valine ethyl ester hydrochloride, L-leucine ethyl ester hydrochloride, L-phenylalanine ethyl ester hydrochloride, L-tryptophan ethyl ester hydrochloride) of carboxyl group on 1-position of intermediate 7 in BOP (benzotriazol-1-yl-oxytris(dimethylamino)phosphoniumhexa-fluorophosphate) and DIEA (dimethyltriethylamine) conditions, and then followed by deprotection of ketal with TFA (trifluoroacetic acid) to obtain another series of target products 6a–f.
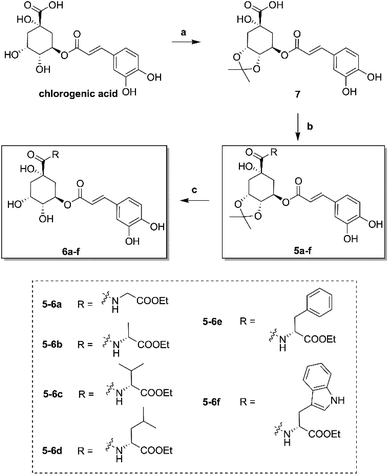 |
| Scheme 1 The synthesis of derivatives 5a–f and 6a–f. Reagents and conditions: (a) DMP, TsOH, dry acetone, 60 min, r.t., 82%; (b) BOP, DIEA, amino acid ester hydrochlorides, 3–8 h, r.t., 86% (5a), 81% (5b), 72% (5c), 78% (5d), 81% (5e), 67% (5f); (c) TFA, DCM, H2O (9 : 1 : 1), 4 h, r.t., 89% (6a), 83% (6b), 79% (6c), 82% (6d), 83% (6e), 80% (6f). | |
Biological results and discussion
Lipid-lowering effects of chlorogenic acid and compounds 5a–f and 6a–f on oleic acid-elicited lipid accumulation in HepG2 liver cells. The lipid-lowering effects of chlorogenic acid and amino acid ester-coupled caffeoylquinic acids derivatives against oleic acid-elicited lipid accumulation in HepG2 liver cells were detected by Oil red O staining assay. MTT assay showed that amino acid ester-coupled caffeoylquinic acids derivatives 5a–5f and 6a–6f (Fig. 3A) in 10 μmol L−1 displayed no cytotoxicity within 24 h to HepG2 liver cells. As shown in Fig. 3B, the preliminary test of compounds 5a–5f and 6a–6f at 10 μmol L−1 revealed that 6c, 6d, 6e and 6f exhibited better regulate effects than chlorogenic acid (CA). The absorbance decreased from 0.3698 (with oleic acid treatment alone) to 0.3547, 0.3552, 0.3483, and 0.3483 after treatment with 10 μmol L−1 compounds 6c, 6d, 6e and 6f, respectively. The Oil red O staining images were showed in Fig. 3C. Among them, derivatives 6c, 6d, 6e and 6f exhibited a more potent regulation effects than the others after treatment for 24 h, which suggests that 6c, 6d, 6e and 6f deserves further evaluation as potential hypolipidemic agents for regulation on oleic acid-elicited lipid accumulation in HepG2 liver cells.
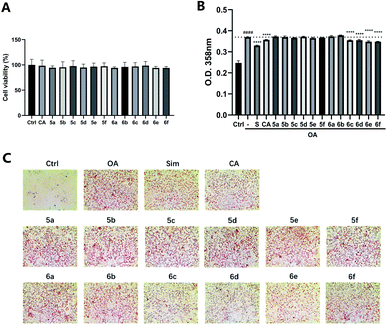 |
| Fig. 3 The nontoxic effects of chlorogenic acid and amino acid ester-coupled caffeoylquinic acids derivatives on cell viability. (A) Cells treated with 10 μmol L−1 compounds 5a–5f and 6a–6f for 24 h respectively. (B) The regulation effects of chlorogenic acid and amino acid ester-coupled caffeoylquinic acids derivatives on oleic acid-elicited lipid accumulation in HepG2 liver cells. HepG2 cells were co-incubated in serum-free DMEM containing 100 μmol L−1 oleic acid (OA) (Model) and co-treated with 10 μmol L−1 of simvastatin (S or Sim) or chlorogenic acid (CA), compounds 5a–5f and 6a–6f for 24 h respectively. (C) Oil red O staining images. The data are expressed as the mean ± SD (n = 8) from three independent experiments. ####p < 0.0001 versus control group (Ctrl); ****p < 0.0001 versus OA-treated cells. | |
Inhibitory effects of compounds 5a–f and 6a–f toward intracellular triglycerides accumulation. To investigate whether amino acid ester-coupled caffeoylquinic acids derivatives could affect TG levels, the inhibitory effects of derivatives 5a–f and 6a–f toward triglycerides were assessed by means of intracellular TG quantification assay. As shown in Fig. 4, it was found that 5d and 6c–f can reduce the TG level of HepG2 cells in the 5a–f and 6a–f tests at 10 μmol L−1. Treatment of HepG2 liver cells with 6c, 6d, 6e and 6f showed a better inhibitory effect than CA. As shown in Fig. 4, the levels of TG in HepG2 liver cells decreased from 100.0% (with OA treatment alone) to 70.4%, 68.4%, 80.6% and 74.5%, after treatment with 10 μmol L−1 compounds 6c, 6d, 6e and 6f. Among them, compounds 6c and 6d showed comparable potential inhibitory effect with the positive control simvastatin (S: 71.4%) and chlorogenic acid (CA: 83.7%). And the efficacy of 6e and 6f in decreasing TG levels was little weaker than that of simvastatin, but better than that of CA. Taken together, these results indicated that derivatives 6c, 6d, 6e and 6f inhibited TG levels markedly on oleic acid-elicited lipid accumulation in HepG2 liver cells.
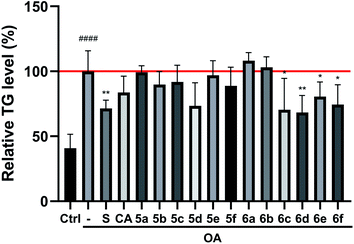 |
| Fig. 4 Inhibitory effects of compound 5a–f and 6a–f toward intracellular triglycerides accumulation in HepG2 cells. Positive control: simvastatin (Sim, 71.4%) and chlorogenic acid (CA, 83.7%); control (Ctrl, 40.8%): DMEM; OA (100.0%): oleic acid. Intracellular levels of triglycerides were measured by kits according to the manufacturer's instructions. Bars depict the means ± SD in triplicate. ####p < 0.0001 versus control group (Ctrl); **p < 0.01 versus OA-treated cells, *p < 0.05 versus OA-treated cells. | |
The preliminary structure–activity relationships (SARs) suggested that, compared to the positive group Sim and CA, the amino acid ester-coupled caffeoylquinic acids derivatives with 4,5-position hydroxyl groups substituted by ketal were weaker potent than those with 4,5-position hydroxyl groups exposed. Beyond that, the derivatives 6c, 6d, 6e and 6f with valine, leucine, phenylalanine, and tryptophan esters groups could better regulate lipid accumulation after oleic acid treatment, indicating that the introduction of valine, leucine, phenylalanine, and tryptophan esters groups could ameliorate lipid accumulation. Among them, derivative 6c and 6d exhibited more potent lipid-lowering effects, compared to 6e and 6f, which means that aliphatic group with on the amino acid is sort of favourable substituent for regulation lipid accumulation than aromatic group in HepG2 liver cells. However, derivatives 6a and 6b, including glycine and alanine esters groups, were inert, suggesting that such unbranched aliphatic glycine and alanine esters groups was negative factor for the derivatives. In addition, the above derivatives showed lipid-lowering effects in Fig. 3 and 4, illustrating that blockage of 4,5-position hydroxyl groups on the chlorogenic acid with isopropylidene may deteriorate regulating lipid effects, such as compound 5c vs. 6c and 5e vs. 6e, and different amino acid esters groups on 1-position carboxyl group influenced lipid-lowering activities obviously.
The regulation of compounds 5a–f and 6a–f on lipometabolic-modulating genes. Lipogenesis and lipid oxidation considered to be two important processes regulate liver lipid metabolism. SREBP1c is one of the most important transcription factors modulating de novo lipogenesis in the liver and primarily regulates genes involved in fatty acid biosynthesis, including acetyl-CoA carboxylase (ACC) and fatty acid synthase (FAS). To investigate the regulation of CA and its amino acid ester-coupled analogues on lipometabolic-modulating genes, 6c, 6d, 6e and 6f that having the preferable potency compounds were selected for further investigation with CA, and their regulation on these lipometabolic involved genes were assessed. As shown in Fig. 5, quantitative real-time PCR results showed that compounds 6c–f exhibited moderate to good regulation effects on lipometabolic-modulating genes. Among them, compound 6c, possessing valine ester group, remarkably stimulated down-regulation of ACC and FAS transcription than CA. Compound 6d with leucine ester moiety significantly reduced the expression of lipogenic genes SREBP1c as well as its down steam genes FAS. These results proved our previous data obtained from oil-red O staining and TG levels, and demonstrated that compound 6c, 6d, 6e and 6f shows more potent regulation effects on these lipogenic and lipid oxidation related genes.
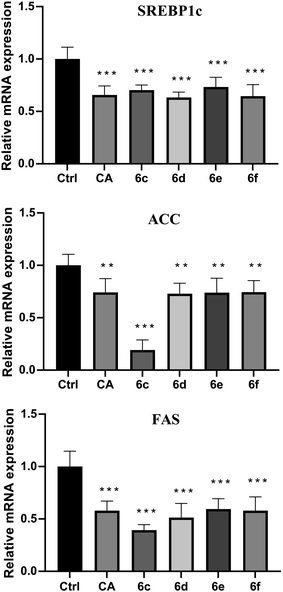 |
| Fig. 5 Effects of CA and its amino acid ester-coupled analogues 6c–f (10 μmol L−1) on the mRNA levels of lipogenic genes SREBP1c, ACC and FAS in OA-elicited HepG2 cells. Real-time quantitative PCR was conducted with gene-specific oligonucleotide primers. The amplification of β-actin served as an internal control (n = 6). Data are depicted as means ± SD in triplicate. **p < 0.01, ***p < 0.001, test groups vs. negative control group. Ctrl: negative control; CA: chlorogenic acid; SREBP1c: sterol response element binding protein 1c; ACC: acetyl-CoA carboxylase; FAS: fatty acid synthase. | |
Experimental
General information
All the reagents were used without further purification unless otherwise specified. Solvents were dried and redistilled before used in the reaction. Analytical TLC was performed using silica gel HF254. Preparative column chromatography was performed with silica gel H. 1H and 13C NMR spectra were recorded on a Bruker Advance III 600 MHz spectrometer. HRMS were obtained on a Thermofisher LTQ-Obitrap XL. Chlorogenic acid, DMP (dimethyoxypropane), TsOH (p-toluenesulfonic acid), BOP (benzotriazol-1-yl-oxytris (dimethylamino)phosphonium-hexa-fluorophosphate), DIEA (dimethyltriethylamine), glycine ethyl ester hydrochloride, L-alanine ethyl ester hydrochloride, L-valine ethyl ester hydrochloride, L-leucine ethyl ester hydrochloride, L-phenylalanine ethyl ester hydrochloride, L-tryptophan ethyl ester hydrochloride, TFA (trifluoroacetic acid), DCM (dichloromethane) were purchased from the InnoChem Company. Dulbecco's Modified Eagle Medium (DMEM), fetal bovine serum were purchased from Corning Inc (CA, USA). Penicillin and streptomycin were procured from Hyclone (Logan, Utah, USA). Simvastatin, oil-red O, oleic acid (OA) and dimethyl sulphoxide DMSO were purchased from Sigma-Aldrich (St. Louis, MO, USA). The kits for triglyceride (TG) were purchased from Jian Cheng Biotechnology Company (Nanjing, China). Total RNA extraction reagent Trizol (Ambion, USA), PrimeScript RT reagent kit and SYBR-Green PCR kit were purchased from Transgene Biotech, Inc. (Beijing, China).
Chemistry
Procedure for the synthesis of intermediate 7. To a suspension of chlorogenic acid (10.5 g, 29.7 mmol) in dry acetone (90 mL) and DMP (60 mL), catalytic amount of TsOH (75 mg, 0.39 mmol) was added. Then the reaction mixture was stirred at room temperature for 60 min. Reaction was monitored by TLC. The crude mixture was neutralized with Na2CO3 powder to pH 6. Then the suspension was filtered out and the filtrate was evaporated and the crude product was subjected to column chromatography (eluent: PE–EtOAc, 1
:
1) to offer pure light yellow solid compound 7 (9.6 g, 82% yield).
General procedure for the synthesis of compounds 5a–f. The compound 7 (1.2 g, 3.1 mmol) was dissolved in dry THF (50 mL), and then BOP (1.4 g, 3.1 mmol) and organic base DIEA (0.8 g, 6.2 mmol) were added and stirred at room temperature under N2 air. After that, various amino acid ester hydrochlorides (glycine ethyl ester hydrochloride, L-alanine ethyl ester hydrochloride, L-valine ethyl ester hydrochloride, L-leucine ethyl ester hydrochloride, L-phenylalanine ethyl ester hydrochloride, L-tryptophan ethyl ester hydrochloride) were putted in and reacted respectively for 3–8 h. When completed by TLC monitor, the reaction solvent was evaporated and the crude product was subjected to column chromatography (eluent: DCM–CH3OH, 50
:
1) to obtain pure compounds 5a–f as white solid.
1-N-Ethoxycarbonylmethyl-4,5-di-O-isopropylidene-chlorogenic acid amide (5a). White powder, 86% yield; 1H-NMR (600 MHz, MeOD) δ: 7.57 (d, J = 15.8 Hz, 1H, H-7′), 7.05 (d, J = 2.0 Hz, 1H, H-2′), 6.95 (dd, J = 8.2 Hz, 2.0 Hz, 1H, H-6′), 6.78 (d, J = 8.2 Hz, 1H, H-5′), 6.28 (d, J = 15.9 Hz, 1H, H-8′), 5.48–5.44 (m, 1H, H-3), 4.56–4.54 (m, 1H, H-4), 4.22–4.16 (m, 3H, H-5, COOC
2CH3), 3.95 (s, 2H, NH–C
2), 2.38–2.35 (m, 1H, H-2), 2.19–2.16 (m, 1H, H-2), 2.02–1.99 (m, 1H, H-6), 1.94–1.89 (m, 1H, H-6), 1.53 (s, 3H, CH3–C–O), 1.35 (s, 3H, C
3–C–O), 1.26 (t, J = 7.1 Hz, 3H, COOCH2C
3); 13C-NMR (150 MHz, MeOD) δ: 178.5 (
ONH), 171.2 (
OOEt), 168.6 (C-9′), 149.6 (C-4′), 147.2 (C-7′), 146.8(C-3′), 127.7 (C-1′), 123.1 (C-6′), 116.5 (C-5′), 115.1 (C-2′), 115.0 (C-8′), 110.4 (O–C–O), 78.6 (C-3), 76.1 (C-1), 75.5 (C-4), 72.5 (C-5), 62.4 (COO
H2CH3), 42.0 (NH
H2), 37.7 (C-2), 35.3 (C-6), 28.4 (
H3–C–O), 26.2 (
H3–C–O), 14.4 (COOCH2
H3); HRMS (ESI): calcd for [M + Na]+ C23H29NNaO10: 502.1689, found 502.1691.
1-N-(α-Ethoxycarbonyl)ethyl-4,5-di-O-isopropylidene-chlorogenic acid amide (5b). White powder, 81% yield; 1H-NMR (600 MHz, MeOD) δ: 7.57 (d, J = 15.8 Hz, 1H, H-7′), 7.05 (d, J = 2.0 Hz, 1H, H-2′), 6.95 (dd, J = 8.2 Hz, 2.0 Hz, 1H, H-6′), 6.78 (d, J = 8.2 Hz, 1H, H-5′), 6.28 (d, J = 15.9 Hz, 1H, H-8′), 5.47–5.43 (m, 1H, H-3), 4.56–4.53 (m, 1H, H-4), 4.37 (q, J = 7.3 Hz, 1H, NHC
CH3), 4.21–4.16 (m, 3H, H-5, COOC
2CH3), 2.35–2.31 (m, 1H, H-2), 2.18–2.15 (m, 1H, H-2), 1.97–1.90 (m, 2H, H-6), 1.53 (s, 3H, CH3–C–O), 1.42 (d, J = 7.3 Hz, 3H, NHCHC
3), 1.35 (s, 3H, C
3–C–O), 1.27 (t, J = 7.2 Hz, COOCH2C
3); 13C-NMR (150 MHz, MeOD) δ: 177.6 (
ONH), 173.9 (
OOEt), 168.6 (C-9′), 149.7 (C-4′), 147.2 (C-7′), 146.8 (C-3′), 127.7 (C-1′), 123.0 (C-6′), 116.5 (C-5′), 115.1 (C-2′), 115.0 (C-8′), 110.4 (O–C–O), 78.6 (C-3), 76.0 (C-1), 75.4 (C-4), 72.5 (C-5), 62.5 (COO
H2CH3), 49.5 (NH
HCH3), 37.6 (C-2), 35.3 (C-6), 28.4 (
H3–C–O), 26.2 (
H3–C–O), 17.5 (NHCH
H3), 14.4 (COOCH2
H3); HRMS (ESI): calcd for [M + Na]+ C24H31NNaO10: 516.1846, found 516.1846.
1-N-(α-Ethoxycarbonyl-β-methyl)propyl-4,5-di-O-isopropylidene-chlorogenic acid amide (5c). White powder, 72% yield; 1H-NMR (600 MHz, MeOD) δ: 7.57 (d, J = 15.9 Hz, 1H, H-7′), 7.04 (d, J = 1.9 Hz, 1H, H-2′), 6.95 (dd, J = 8.2 Hz, 2.0 Hz, 1H, H-6′), 6.77 (d, J = 8.2 Hz, 1H, H-5′), 6.27 (d, J = 16.0 Hz, 1H, H-8′), 5.47–5.43 (m, 1H, H-3), 4.55–4.53 (m, 1H, H-4), 4.29 (d, J = 5.4 Hz, 1H, NHC
), 4.25–4.16 (m, 3H, H-5, COOC
2CH3), 2.36–2.33 (m, 1H, H-2), 2.22–2.14 (m, 2H, H-2, NHCHC
), 1.96–1.91 (m, 2H, H-6), 1.53 (s, 3H, CH3–C–O), 1.34 (s, 3H, C
3–C–O), 1.28 (t, J = 7.1 Hz, COOCH2C
3), 0.97–0.92 (m, 6H, 2 × CH3); 13C-NMR (150 MHz, MeOD) δ: 177.7 (
ONH), 172.7 (
OOEt), 168.6 (C-9′), 149.7 (C-4′), 147.3 (C-7′), 146.9 (C-3′), 127.6 (C-1′), 123.1 (C-6′), 116.5 (C-5′), 115.1 (C-2′), 115.0 (C-8′), 110.4 (O–C–O), 78.6 (C-3), 76.2 (C-1), 75.4 (C-4), 72.4 (C-5), 62.4 (COO
H2CH3), 58.8 (NH
H), 37.9 (C-2), 35.1 (C-6), 32.1 (NHCH
H), 28.4 (
H3–C–O), 26.2 (
H3–C–O), 19.4 (CH
H3), 18.2 (CH
H3), 14.5 (COOCH2
H3); HRMS (ESI): calcd for [M + Na]+ C26H35NNaO10: 544.2159, found 544.2161.
1-N-(α-Ethoxycarbonyl-γ-methyl)butyl-4,5-di-O-isopropylid-ene-chlorogenic acid amide (5d). White powder, 78% yield; 1H-NMR (600 MHz, MeOD) δ: 7.56 (d, J = 15.9 Hz, 1H, H-7′), 7.04 (d, J = 2.0 Hz, 1H, H-2′), 6.95 (dd, J = 8.2 Hz, 2.0 Hz, 1H, H-6′), 6.77 (d, J = 8.2 Hz, 1H, H-5′), 6.27 (d, J = 15.9 Hz, 1H, H-8′), 5.46–5.42 (m, 1H, H-3), 4.55–4.53 (m, 1H, H-4), 4.43–4.41 (m, 1H, NHC
), 4.21–4.14 (m, 3H, H-5, COOC
2CH3), 2.35–2.31 (m, 1H, H-2), 2.18–2.15 (m, 1H, H-2), 1.94–1.92 (m, 2H, H-6), 1.71–1.64 (m, 3H, NHCHC
2C
), 1.53 (s, 3H, CH3–C–O), 1.34 (s, 3H, C
3–C–O), 1.26 (t, J = 7.1 Hz, COOCH2C
3), 0.95–0.94 (m, 3H, CH3), 0.92–0.91 (s, 3H, CH3); 13C-NMR (150 MHz, MeOD) δ: 177.8 (CONH), 173.9 (
OOEt), 168.6 (C-9′), 149.7 (C-4′), 147.3 (C-7′), 146.8 (C-3′), 127.7 (C-1′), 123.1 (C-6′), 116.5 (C-5′), 115.1 (C-2′), 115.0 (C-8′), 110.4 (O–C–O), 78.6 (C-3), 76.1 (C-1), 75.4 (C-4), 72.5 (C-5), 62.4 (COO
H2CH3), 52.1 (NH
H), 41.4 (NHCH
H2), 37.7 (C-2), 35.3 (C-6), 28.4 (
H3–C–O), 26.2 (
H3–C–O), 26.0 (
H(CH3)2), 23.3 (CH
H3), 21.9 (CH
H3), 14.5 (COOCH2
H3); HRMS (ESI): calcd for [M + Na]+ C27H37NNaO10: 558.2315, found 558.2319.
1-N-(α-Ethoxycarbonyl)phenethyl-4,5-di-O-isopropylidene-chlorogenic acid amide (5e). White powder, 81% yield; 1H-NMR (600 MHz, MeOD) δ: 7.58 (d, J = 15.8 Hz, 1H, H-7′), 7.27–7.24 (m, 2H, H–ph′′), 7.19–7.17 (m, 3H, H–ph′′), 7.06 (d, J = 1.9 Hz, 1H, H-2′), 6.95 (dd, J = 8.2 Hz, 1.9 Hz, 1H, H-6′), 6.79 (d, J = 8.2 Hz, 1H, H-5′), 6.28 (d, J = 15.8 Hz, 1H, H-8′), 5.40–5.36 (m, 1H, H-3), 4.67–4.66 (m, 1H, NHC
), 4.52–4.50 (m, 1H, H-4), 4.19–4.14 (m, 3H, H-5, COOC
2CH3), 3.19 (dd, J = 13.8 Hz, 5.6 Hz, 1H, ph′′C
2), 3.06 (dd, J = 13.8 Hz, 8.3 Hz, 1H, ph′′C
2), 2.32–2.29 (m, 1H, H-2), 2.11–2.08 (m, 1H, H-2), 1.76–1.68 (m, 2H, H-6), 1.51 (s, 3H, CH3–C–O), 1.34 (s, 3H, CH3–C–O), 1.24 (t, J = 7.2 Hz, COOCH2C
3); 13C-NMR (150 MHz, MeOD) δ: 177.4 (
ONH), 172.7 (
OOEt), 168.6 (C-9′), 149.7 (C-4′), 147.3 (C-7′), 146.8 (C-3′), 137.7 (C–Ph′′), 130.4 (2C–Ph′′), 129.6 (2C–Ph′′), 128.0 (C–Ph′′), 127.7 (C-1′), 123.1 (C-6′), 116.5 (C-5′), 115.2 (C-2′), 115.1 (C-8′), 110.4 (O–C–O), 78.5 (C-3), 76.0 (C-1), 75.4 (C-4), 72.5 (C-5), 62.6 (COO
H2CH3), 54.8 (NH
HCH2), 38.4 (NHCH
H2), 37.7 (C-2), 35.0 (C-6), 28.4 (
H3–C–O), 26.2 (
H3–C–O), 14.4 (COOCH2
H3); HRMS (ESI): calcd for [M + Na]+ C30H35NNaO10: 592.2159, found 592.2163.
1-N-[(α-Ethoxycarbonyl)-1H-indol-3′-yl]ethyl-4,5-di-O-isopropylidene-chlorogenic acid amide (5f). White powder, 67% yield; 1H-NMR (600 MHz, MeOD) δ: 7.57 (d, J = 15.9 Hz, 1H, H-7′), 7.50–7.49 (m, 1H, H–indole′′), 7.30–7.29 (m, 1H, H–indole′′), 7.08 (s, 1H, H–indole′′), 7.06–7.03 (m, 2H, H-2′, H–indole′′), 7.00–6.96 (m, 2H, H-6′, H–indole′′), 6.78 (d, J = 8.1 Hz, 1H, H-5′), 6.27 (d, J = 15.8 Hz, 1H, H-8′), 5.40–5.36 (m, 1H, H-3), 4.68–4.66 (m, 1H, NHC
), 4.50–4.48 (m, 1H, H-4), 4.15–4.09 (m, 3H, H-5, COOC
2CH3), 2.30–2.29 (m, 2H, indole–C
2), 2.30–2.27 (m, 1H, H-2), 2.10–2.07 (m, 1H, H-2), 1.74–1.73 (m, 2H, H-6), 1.50 (s, 3H, CH3–C–O), 1.31 (s, 3H, CH3–C–O), 1.18 (t, J = 7.3 Hz, COOCH2C
3); 13C-NMR (150 MHz, MeOD) δ: 177.5 (CONH), 173.2 (
OOEt), 168.6 (C-9′), 149.7 (C-4′), 147.2 (C-7′), 146.9 (C-3′), 138.0 (Cindole′′), 128.7 (C–indole′′), 127.7 (C-1′), 124.6 (C–indole′′), 123.1 (C-6′), 122.5 (C–indole′′), 119.9 (C–indole′′), 119.3 (C–indole′′), 116.5 (C-5′), 115.1 (C-2′), 115.0 (C-8′), 112.3 (C–indole′′), 110.4 (O–C–O), 110.2 (C–indole′′), 78.6 (C-3), 76.0 (C-1), 75.4 (C-4), 72.4 (C-5), 62.6 (COO
H2CH3), 54.5 (NH
HCH2), 37.5 (C-2), 35.1 (C-6), 28.4 (
H3–C–O), 28.3 (NHCH
H2), 26.2 (
H3–C–O), 14.3 (COOCH2
H3); HRMS (ESI): calcd for [M + Na]+ C32H36N2NaO10: 631.2268, found 631.2269.
General procedure for the synthesis of compounds 6a–f. The compounds 5a–f (1.0 mmol) was dissolved in TFA–DCM–H2O (8 mL) and reacted for 4 h at room temperature. After reaction finished, the solvent was evaporated and the oily residue was purified by silica gel column chromatography (eluent: DCM–CH3OH, 10
:
1) to gain pure compound 6a–f as white solid.
1-N-Ethoxycarbonylmethyl-chlorogenic acid amide (6a). White powder, 89% yield; 1H-NMR (600 MHz, MeOD) δ: 7.57 (d, J = 15.8 Hz, 1H, H-7′), 7.04 (d, J = 2.0 Hz, 1H, H-2′), 6.95–6.94 (m, 1H, H-6′), 6.77 (d, J = 8.2 Hz, 1H, H-5′), 6.29 (d, J = 15.9 Hz, 1H, H-8′), 5.44–5.39 (m, 1H, H-3), 4.25–4.24 (m, 1H, H-4), 4.19–4.15 (m, 2H, COOC
2CH3), 3.97–3.90 (m, 2H, NH–C
2), 3.72–3.70 (m, 1H, H-5), 2.19–2.17 (m, 1H, H-2), 2.09–2.06 (m, 1H, H-2), 2.02–1.98 (m, 2H, H-6), 1.27–1.24 (m, 3H, COOCH2C
3); 13C-NMR (150 MHz, MeOD) δ: 177.5 (
ONH), 171.2 (
OOEt), 169.1 (C-9′), 149.6 (C-4′), 147.0 (C-7′), 146.8 (C-3′), 127.8 (C-1′), 123.0 (C-6′), 116.5 (C-5′), 115.4 (C-2′), 115.1 (C-8′), 77.8 (C-3), 74.4 (C-1), 72.6 (C-4), 71.9 (C-5), 62.4 (COO
H2CH3), 42.0 (NH
H2), 39.9 (C-2), 38.7 (C-6), 14.4 (COOCH2
H3); HRMS (ESI): calcd for [M + Na]+ C20H25NNaO10: 462.1376, found 462.1378.
1-N-(α-Ethoxycarbonyl)ethyl-chlorogenic acid amide (6b). White powder, 83% yield; 1H-NMR (600 MHz, MeOD) δ: 7.58 (d, J = 15.8 Hz, 1H, H-7′), 7.05 (d, J = 2.0 Hz, 1H, H-2′), 6.95 (m, J = 8.2 Hz, 2.0 Hz, 1H, H-6′), 6.78 (d, J = 8.2 Hz, 1H, H-5′), 6.30 (d, J = 15.9 Hz, 1H, H-8′), 5.43–5.39 (m, 1H, H-3), 4.37 (q, 1H, NHC
), 4.25–4.24 (m, 1H, H-4), 4.21–4.13 (m, 2H, COOC
2CH3), 3.72–3.70 (m, 1H, H-5), 2.15–2.12 (m, 1H, H-2), 2.06–1.99 (m, 3H, H-2, H-6), 1.41 (d, J = 7.3 Hz, 1H, NHCHC
), 1.26 (m, J = 7.3 Hz, 3H, COOCH2C
3); 13C-NMR (150 MHz, MeOD) δ: 176.6 (
ONH), 174.0 (
OOEt), 169.1 (C-9′), 149.6 (C-4′), 147.0 (C-7′), 146.8 (C-3′), 127.8 (C-1′), 123.0 (C-6′), 116.5 (C-5′), 115.3 (C-2′), 115.1 (C-8′), 77.7 (C-3), 74.3 (C-1), 72.6 (C-4), 71.9 (C-5), 62.5 (COO
H2CH3), 49.5 (NH
HCH3), 39.8 (C-2), 38.6 (C-6), 17.5 (NHCH
H3), 14.4 (COOCH2
H3); HRMS (ESI): calcd for [M + Na]+ C21H27NNaO10: 476.1533, found 476.1537.
1-N-(α-Ethoxycarbonyl-β-methyl)propyl-chlorogenic acid amide (6c). White powder, 79% yield; 1H-NMR (600 MHz, MeOD) δ: 7.58 (d, J = 15.8 Hz, 1H, H-7′), 7.04 (d, J = 2.0 Hz, 1H, H-2′), 6.95 (m, J = 8.2 Hz, 2.0 Hz, 1H, H-6′), 6.77 (d, J = 8.2 Hz, 1H, H-5′), 6.30 (d, J = 15.9 Hz, 1H, H-8′), 5.43–5.39 (m, 1H, H-3), 4.30–4.27 (m, 1H, NHC
), 4.25–4.23 (m, 1H, H-4), 4.22–4.17 (m, 2H, COOC
2CH3), 3.72–3.70 (m, 1H, H-5), 2.22–1.96 (m, 5H, H-2, H-6, NHCHC
), 1.28 (t, J = 7.1 Hz, COOCH2C
3), 0.95–0.93 (m, 6H, 2×CH3); 13C-NMR (150 MHz, MeOD) δ: 176.7 (
ONH), 172.7 (
OOEt), 169.0 (C-9′), 149.6 (C-4′), 147.1 (C-7′), 146.8 (C-3′), 127.7 (C-1′), 123.0 (C-6′), 116.5 (C-5′), 115.3 (C-2′), 115.1 (C-8′), 77.9 (C-3), 74.3 (C-1), 72.6 (C-4), 71.8 (C-5), 62.4 (COO
H2CH3), 58.7 (NH
H), 40.2 (C-2), 38.5 (C-6), 32.1 (NHCH
H), 19.4 (CH
H3), 18.2 (CH
H3), 14.5 (COOCH2
H3); HRMS (ESI): calcd for [M + Na]+ C23H31NNaO10: 504.1846, found 504.1849.
1-N-(α-Ethoxycarbonyl-γ-methyl)butyl-chlorogenic acid amide (6d). White powder, 82% yield; 1H-NMR (600 MHz, MeOD) δ: 7.58 (d, J = 15.9 Hz, 1H, H-7′), 7.05 (d, J = 2.0 Hz, 1H, H-2′), 6.95 (m, J = 8.2 Hz, 2.0 Hz, 1H, H-6′), 6.78 (d, J = 8.2 Hz, 1H, H-5′), 6.30 (d, J = 15.9 Hz, 1H, H-8′), 5.43–5.39 (m, 1H, H-3), 4.44–4.41 (m, 1H, NHC
), 4.25–4.23 (m, 1H, H-4), 4.20–4.14 (m, 2H, COOC
2CH3), 3.72–3.70 (m, 1H, H-5), 2.13–2.00 (m, 4H, H-2, H-6), 1.71–1.63 (m, 3H, NHCHC
2C
), 0.95–0.94 (m, 3H, CH3), 0.92–0.91 (s, 3H, CH3); 13C-NMR (150 MHz, MeOD) δ: 176.8 (CONH), 173.9 (
OOEt), 169.1 (C-9′), 149.6 (C-4′), 147.1 (C-7′), 146.8 (C-3′), 127.8 (C-1′), 123.0 (C-6′), 116.5 (C-5′), 115.3 (C-2′), 115.2 (C-8′), 77.8 (C-3), 74.3 (C-1), 72.6 (C-4), 71.9 (C-5), 62.4 (COO
H2CH3), 52.1 (NH
H), 41.4 (NHCH
H2), 39.9 (C-2), 38.6 (C-6), 26.1 (
H3–C–O), 23.3 (CH
H3), 21.9 (CH
H3), 14.5 (COOCH2
H3); HRMS (ESI): calcd for [M + Na]+ C23H31NNaO8: 518.2002, found 518.2009.
1-N-(α-Ethoxycarbonyl)phenethyl-chlorogenic acid amide (6e). White powder, 83% yield; 1H-NMR (600 MHz, MeOD) δ: 7.58 (d, J = 15.9 Hz, 1H, H-7′), 7.27–7.24 (m, 2H, H–ph′′), 7.19–7.17 (m, 3H, H–ph′′), 7.05 (d, J = 2.0 Hz, 1H, H-2′), 6.96 (m, J = 8.2 Hz, 2.0 Hz, 1H, H-6′), 6.78 (d, J = 8.2 Hz, 1H, H-5′), 6.29 (d, J = 15.9 Hz, 1H, H-8′), 5.36–5.32 (m, 1H, H-3), 4.65–4.62 (m, 1H, NHC
), 4.21–4.20 (m, 1H, H-4), 4.18–4.14 (m, 2H, COOC
2CH3), 3.67–3.65 (m, 1H, H-5), 3.19–3.16 (m, 2H, NHCHC
2), 3.07–3.04 (m, 1H, NHCHC
2), 2.02–1.79 (m, 4H, H-2, H-6), 1.23 (m, J = 7.3 Hz, 3H, COOCH2C
3); 13C-NMR (150 MHz, MeOD) δ: 176.5 (
ONH), 172.6 (
OOEt), 169.0 (C-9′), 149.6 (C-4′), 147.0 (C-7′), 146.8 (C-3′), 137.8 (C–Ph′′), 130.4 (2C–Ph′′), 129.5 (2C–Ph′′), 128.0 (C–Ph′′), 127.8 (C-1′), 123.0 (C-6′), 116.5 (C-5′), 115.3 (C-2′), 115.1 (C-8′), 77.7 (C-3), 74.3 (C-1), 72.5 (C-4), 71.8 (C-5), 62.5 (COO
H2CH3), 54.7 (NH
HCH2), 39.9 (NHCH
H2), 38.5 (C-2), 38.3 (C-6), 14.4 (COOCH2
H3); HRMS (ESI): calcd for [M + Na]+ C27H31NNaO10: 552.1846, found 552.1850.
1-N-[(α-Ethoxycarbonyl)-1H-indol-3′-yl]ethyl-chlorogenic acid amide (6f). White powder, 80% yield; 1H-NMR (600 MHz, MeOD) δ: 7.58 (d, J = 15.9 Hz, 1H, H-7′), 7.50–7.49 (m, 1H, H-indole′′), 7.31–7.29 (m, 1H, H–indole′′), 7.07–7.05 (m, 3H, 2H–indole′′, H-2′), 7.01–6.98 (m, 1H, H–indole′′), 6.96 (m, J = 8.2 Hz, 2.0 Hz, 1H, H-6′), 6.78 (d, J = 8.2 Hz, 1H, H-5′), 6.30 (d, J = 15.9 Hz, 1H, H-8′), 5.38–5.33 (m, 1H, H-3), 4.68–4.65 (m, 1H, NHC
), 4.20–4.19 (m, 1H, H-4), 4.13–4.08 (m, 2H, COOC
2CH3), 3.67–3.65 (m, 1H, H-5), 3.30–3.29 (m, 2H, NHCHC
2), 2.01–1.85 (m, 4H, H-2, H-6), 1.17 (m, J = 7.1 Hz, 3H, COOCH2C
3); 13C-NMR (150 MHz, MeOD) δ: 175.1 (CONH), 171.8 (
OOEt), 167.6 (C-9′), 148.2 (C-4′), 145.6 (C-7′), 145.4 (C-3′), 136.6 (C–indole′′), 127.3 (C–indole′′), 126.4 (C-1′), 123.2 (C–indole′′), 121.6 (C-6′), 121.1 (C–indole′′), 118.5 (C–indole′′), 117.9 (C–indole′′), 115.1 (C-5′), 114.0 (C-2′), 113.7 (C-8′), 110.9 (C–indole′′), 108.8 (C–indole′′), 76.3 (C-3), 72.9 (C-1), 71.1 (C-4), 70.4 (C-5), 61.1 (COO
H2CH3), 53.1 (NH
HCH2), 38.4 (C-2), 37.1 (C-6), 26.9 (NHCH
H2), 12.9 (COOCH2
H3); HRMS (ESI): calcd for [M + Na]+ C29H32N2NaO10: 591.1955, found 591.1960.The spectrograms of the derivatives 5a–f and 6a–f were shown in ESI.†
Evaluation of the biological activity
Cell culture and compounds solution preparation. HepG2 cells were from American Type Culture Collection (ATCC) (Manassas, VA, USA) and obtained from the Peking Union Medical College (Beijing, China). HepG2 cells were cultured in Dulbecco's Modified Eagle Medium (DMEM) supplemented with 10% fetal bovine serum, 1% penicillin and streptomycin at 37 °C in 5% CO2 atmosphere with 100% humidity. Compounds were dissolved in dimethyl sulphoxide (DMSO) and the equal volume of it was added in control group. Chlorogenic acid and its derivatives 5a–f, 6a–f were dissovled in dimethyl sulphoxide (DMSO) to a final concentration of 20 mmol L−1 for cellular assay.
Cell viability assay. Cell viability was examined using MTT assay. HepG2 cells in 96-well culture plates were treated with 10 μmol L−1 compounds. The cells were incubated for 24 h, and MTT reagent was added to each well according to the instruction of CellTiter 96® Aqueous One Solution Cell Proliferation Assay Kit (Promega Corperation, Beijing, China). The absorbance at 490 nm was measured using a microplate reader (ThermoFisher Ltd., Shanghai, China).
Oil red O staining. The cells with 70–80% confluence in 96 well plates were incubated in serum-free DMEM + oleic acid (OA) (100 μmol L−1) and 10 μmol L−1 of chlorogenic acid, the derivative compound 5a–f, 6a–f respectively or the positive control simvastatin (10 μmol L−1) for 24 h. Cells were then fixed with 4% w/v paraformaldehyde at room temperature for 30 min and stained with filtered oil-red O staining solution (prepared by mixing two volume of 0.5% oil-red O stock solution with three volume of ddH2O) at room temperature for 15 min. The oil-red O was redissolved with DMSO and measured by a Tecan Infinite M1000Pro Microplate Reader and spectrophotometry at 358 nm.
Intracellular TG quantification. HepG2 cells with 70–80% confluence in 6 well plates were incubated in serum-free DMEM + OA (100 μmol L−1) and 10 μmol L−1 of chlorogenic acid, compound 5a–f, 6a–f respectively or the positive control simvastatin (10 μmol L−1) for 24 h. The cells were subjected to TG quantification as introduced by the protocol of Triglyceride Quantification Kit. Each experiment was performed in duplicates with three wells for each compound.
RNA isolation and quantitative real-time PCR. The total RNA of each sample was extracted with Trizol (Invitrogen). cDNA was synthesized using EasyScript® One-Step gDNA Removal and cDNA Synthesis SuperMix (Transgen Biotech), and quantitative real-time PCR was performed with ABI Prism7500 Instrument, using TransStart® Green qPCR SuperMix (Transgen Biotech). The specificity of primer was confirmed via agarose gel electrophoresis and melting-curve analysis. A housekeeping gene (β-actin) was used as an internal standard. The relative expression or amount of specific genes was quantitated with the 2−ΔΔCt calculation.The primers used are shown in the ESI Table 1.†
Statistical analysis. Data are expressed as mean ± SEM. One-way ANOVA was used to determine significant differences among groups, after which the modified Students t-test with the Bonferroni correction was used for comparison between individual groups. All statistical analyses were performed with SPSS 17.0 software (SPSS Inc., Chicago, IL, USA). A value of P value < 0.05 was considered statistically significant.
Conclusions
The results of the present research indicated that amino acid ester-coupled caffeoylquinic acids derivatives 6c, 6d, 6e and 6f exerts a profound lipid-lowering activity against oleic acid-elicited lipid accumulation for HepG2 liver cells through regulation of TG levels and mRNA levels of lipometabolic-modulating genes. Preliminary SAR analysis has shown that the aliphatic group on the amino acid ester-coupled derivatives had a good impact on the protective effect. Further studies are in progress as the promising results demonstrate that these amino acid ester-coupled caffeoylquinic acids derivatives merit further investigation as potential hypolipidemic agents.
Conflicts of interest
There are no conflicts to declare.
Acknowledgements
This work was supported by Beijing Natural Science Foundation (grant no. 7192129), the National Natural Sciences Foundation of China (grant no. 81302656), and the CAMS Innovation Fund for Medical Science (CIFMS) (grant no. 2016-I2M-1-012).
Notes and references
- T. J. Lan, Q. F. Li, M. Chang, C. L. Yin, D. Zhu, Z. Wu, X. L. Li, W. Q. Zhang, B. W. Yue, J. L. Shi, H. B. Yuan, Z. H. Su and H. W. Guo, J. Ethnopharmacol., 2020, 260, 112989 CrossRef CAS.
- H. L. Gao, Y. Z. Long, X. Q. Jiang, Z. C. Liu, D. C. Wang, Y. Zhang, D. W. Li and B. L. Sun, Exp. Gerontol., 2013, 48, 572–578 CrossRef CAS.
- S. Gao, G. S. Hu, D. Li, M. Z. Sun and D. H. Mou, J. Funct. Foods, 2020, 66, 103837 CrossRef CAS.
- M. J. Hao, Z. J. Guan, Y. Gao, J. L. Xing, X. X. Zhou, C. Y. Wang, J. Xu and W. M. Li, Phytomedicine, 2020, 78, 153292 CrossRef CAS.
- T. T. Zeng, D. J. Tang, Y. X. Yuan, J. Su and H. Jiang, Gene, 2017, 626, 319–325 CrossRef CAS.
- V. Bittner, L. Q. Deng, R. S. Rosenson, B. Taylor, S. P. Glasser, S. T. Kent, M. E. Farkouh and P. Maul, J. Am. Coll. Cardiol., 2015, 66, 1864–1872 CrossRef.
- Y. X. Zhang, Y. Y. Gu, Y. B. Chen, Z. Y. Huang, M. Li, W. H. Jiang, J. H. Chen, W. T. Rao, S. F. Luo, Y. Y. Chen, J. Q. Chen, L. J. Li, Y. H. Jia, M. H. Liu and F. H. Zhou, J. Ethnopharmacol., 2021, 266, 113436 CrossRef CAS.
- J. Bordoloia, D. Ozahc, T. Borac, J. Kalitaa and P. Mannaa, J. Nutr. Biochem., 2019, 70, 174–184 CrossRef.
- Z. S. Xie, X. M. Wan, L. J. Zhong, H. Yang, P. Li and X. J. Xu, J. Funct. Foods, 2017, 31, 217–228 CrossRef CAS.
- M. W. Martin, L. D. R. Jr, H. B. Li, S. E. R. Schiller, A. V. Toms, Z. G. Wang, K. W. Bair, J. Castro, S. Fessler, D. Gotur, S. E. Hubbs, G. S. Kauffman, M. Kershaw, G. P. Luke, C. McKinnon, L. Yao, W. Lu and D. S. Millan, Bioorg. Med. Chem. Lett., 2019, 29, 1001–1006 CrossRef CAS.
- L. Q. Han, T. Y. Gao, G. Y. Yang and J. J. Loor, J. Dairy Sci., 2018, 101, 6523–6531 CrossRef CAS.
- Y. Andriani, N. M. Ramli, D. F. Syamsumir, M. N. I. Kassim, J. Jaafar, N. A. Aziz, L. Marlina, N. S. Musa and H. Mohamad, Arabian J. Chem., 2019, 12, 3555–3564 CrossRef CAS.
- C. M. Wu, X. P. Zhang, X. Zhang, H. Luan, G. B. Sun, X. B. Sun, X. L. Wang, P. Guo and X. D. Xu, J. Nutr. Biochem., 2014, 25, 412–419 CrossRef CAS.
- L. H. Peng, J. W. Liu, J. K. Zheng, J. L. Cheng and M. Y. Xian, Chin. J. Ethnomed. Ethnopharm., 2012, 5, 24–25 Search PubMed.
- I. Tomaca, M. Šerugaa and J. Labudab, Food Chem., 2020, 325, 126787 CrossRef.
- V. Francisco, G. Costa, A. Figueirinha, C. Marques, P. Pereira, B. M. Neves, M. C. Lopes, M. T. Cruz and M. T. Batista, J. Ethnopharmacol., 2013, 148, 126–134 CrossRef CAS.
- D. Liu, S. Meng, Z. H. Xiang, N. J. He and G. W. Yang, Phytochemistry, 2019, 163, 1–10 CrossRef CAS.
- D. Y. Wang, X. M. Zhao and Y. L. Liu, Int. J. Biol. Macromol., 2017, 102, 396–404 CrossRef CAS.
- X. P. Zhang, C. M. Wu, H. F. Wu, L. H. Sheng, Y. Su, X. Zhang, H. Luan, G. B. Sun, X. B. Sun, Y. Tian, Y. B. Ji, P. Guo and X. D. Xu, PLoS One, 2013, 8, 61922 CrossRef.
- X. X. Cao, C. M. Wu, Y. Tian and P. Guo, RSC Adv., 2019, 9, 12247–12254 RSC.
- Y. Tian, X. X. Cao, H. Shang, C. M. Wu, X. Zhang, P. Guo, X. P. Zhang and X. D. Xu, Molecules, 2019, 24, 964 CrossRef.
Footnotes |
† Electronic supplementary information (ESI) available. See DOI: 10.1039/d0ra09621k |
‡ These two authors contributed equally to this paper. |
|
This journal is © The Royal Society of Chemistry 2021 |