DOI:
10.1039/D0RA09234G
(Paper)
RSC Adv., 2021,
11, 4547-4554
Ultrasound-assisted enzymatic extraction of hydroxy-sanshool compounds from the hydrodistillation residue of two Sichuan peppers: optimization, quantification and pungent taste contribution evaluation
Received
29th October 2020
, Accepted 11th January 2021
First published on 22nd January 2021
Abstract
Hydroxy-sanshool compounds were extracted from the hydrodistillation residue of Z. bungeanum Maxim. pericarp (ZBM) and Z. armatum DC. pericarp (ZADC) by using ethanol–water extraction and extractions assisted with (i) enzymes, (ii) ultrasound, and (iii) enzymes and ultrasound. The yields of the hydroxy-sanshool extracts under these four extraction conditions were calculated and compared. The ultrasound-assisted enzymatic extraction was then optimized based on a Box–Behnken design. Furthermore, five hydroxy-sanshool compounds in the extracts were quantified and their pungent taste contribution value (PCV) was calculated. Oral sensory evaluation was finally performed. Compared with that of the hydroxy-sanshool extracted with ethanol–water, the yields of the samples from the extraction assisted with enzymes, ultrasound, and enzymes and ultrasound increased by 4.2–7.6%, 9.3–9.8%, and 21.5–26.2%, respectively. A maximum yield of 7.87% (w/w) was achieved by using ultrasound-assisted enzymatic extraction under the optimal conditions: 3.1% (w/w) amount of enzyme, an incubation temperature of 50.3 °C, and an ultrasound irradiation power of 207 W. The PCV of hydroxy-α-sanshool, hydroxy-β-sanshool, hydroxy-γ-sanshool, hydroxy-ε-sanshool, and hydroxy-γ-isosanshool was 1153, 447, 461, 139, and 51 ml g−1 for the ZBM extract, respectively, while the PCV of these five hydroxyl-sanshools was 659 ml g−1, 575 ml g−1, not detected, 119 ml g−1, and not detected for the ZADC extract, respectively. Tingling and salivation were the main taste sensations of ZBM, whereas numbing and tingling sensations were dominant in ZADC.
1. Introduction
Z. bungeanum Maxim. and Z. armatum DC., two traditional cash crops belonging to the Zanthoxylum genus of the family Rutaceae, are widely cultivated in Asian countries including China, Japan, India, Korea, Nepal, etc. They have attracted increasing attention in recent decades because of their pharmaceutical properties, typical pungency and tingling sensation.1,2 The pericarps of Z. bungeanum Maxim. and Z. armatum DC., ZBM and ZADC for short, are traditionally used in herbal medicine and stir-fried or processed with vinegar and salt-water. More than 140 chemical compounds have been isolated and identified from ZBM, including alkaloids, terpenoids, flavonoids, and free fatty acids. These bioactive constituents are responsible for the high medicinal value of the analgesic,2 anti-inflammatory,3,4 antibacterial,5 antioxidant,6 and anti-tumor activities.7
ZBM and ZADC are also known as Sichuan peppers or Huajiao and are used as condiments in cooking. Notably, the primary pungent and tingling substances in Zanthoxylum fruits have been identified as hydroxy-sanshool compounds such as hydroxy-α-sanshool, hydroxy-β-sanshool, hydroxy-γ-sanshool, hydroxy-ε-sanshool and hydroxy-γ-isosanshool.8,9 Their chemical structure and molecular formula are shown in Table 1. The pungent nature of the hydroxy-sanshool compounds could be mediated via interactions with the transient receptor potential ankyrin 1 (TRPA1) and vanilloid 1, two chemosensory ion channels.10 The cis-configured double bonds in the hydroxy-sanshools were reported to be essential for the activation of TRPA1.11 A structure–pungency activity relationship study revealed that a minimal structure including an N-(2-methyl-2-hydroxypropyl) amide and a (CH
Z
CH–CH2–CH2–CH
E
CH) sequence motif plays an important role in the pungent and tingling sensations.12 Moreover, hydroxy-sanshool and its isomers could show different pungent sensations and intensity. For example, hydroxy-sanshools (α, γ, and ε) caused the oral sensations of tingling and salivation, while hydroxy-β-sanshool and hydroxy-γ-isosanshool produced a numbing sensation.8 To the best of our knowledge, the composition of hydroxy-sanshools and the pungent taste contribution of each hydroxy-sanshool in the Zanthoxylum species have rarely been reported. So, extraction and quantification of these five hydroxy-sanshools and the evaluation of their pungent taste contributions would help to recognize the pungent and tingling characteristics of ZBM and ZADC at the molecular level and to deeply understand the structure–activity relationship between hydroxy-sanshool and pungent taste sensations.
Table 1 Chemical structure and molecular formula of five kinds of hydroxy-sanshool compounds
Compounds |
Chemical structure |
Molecular formula |
Hydroxy-α-sanshool |
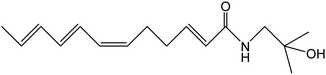 |
C16H25NO2 |
Hydroxy-β-sanshool |
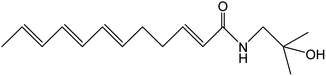 |
C16H25NO2 |
Hydroxy-γ-sanshool |
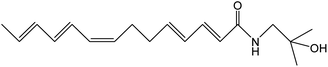 |
C18H27NO2 |
Hydroxy-ε-sanshool |
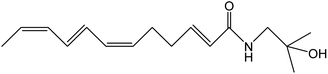 |
C16H25NO2 |
Hydroxy-γ-isosanshool |
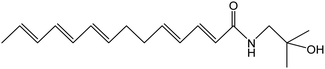 |
C18H27NO2 |
In our previous work, the aromatic essential oil of ZBM and ZADC was obtained by using the hydrodistillation method and a residue was produced.13 If this residue was discarded, it would be a waste of crop resources owing to the fact that the residue is rich in some valuable non-volatile alkylamides such as hydroxy-sanshool compounds.14 So, the hydrodistillation residue of ZBM and ZADC was used as the raw material in this study, and extraction of the hydroxy-sanshool compounds was firstly performed with ethanol–water and then assisted with (i) enzymes, (ii) ultrasound, and (iii) enzymes and ultrasound. The cell destruction of ZBM after these four extractions were observed with scanning electron microscopy. Furthermore, ultrasound-assisted enzymatic extraction of the hydroxy-sanshool compounds was optimized using the Box–Behnken design of response surface methodology. The interactions between three extraction variables (enzyme amount, incubation temperature and ultrasound irradiation power) on the yield were discussed. In addition, five hydroxy-sanshool compounds in the extracts were quantitatively determined and their pungent taste contribution evaluated and the primary hydroxy-sanshool and the typical pungent sensation of ZBM and ZADC were identified. These results were finally verified by oral sensory evaluation.
2. Materials and methods
2.1. Materials and chemicals
ZBM and ZADC were picked in September 2017 from Ya'an, Sichuan Province, China. They were air dried at room temperature, cleaned of impurities and sealed in an aluminum foil bag and stored at −18 °C until use. Their contents (mg g−1) of crude protein, crude fat, crude fiber, total phenols, total flavonoid and moisture were 111.4 ± 10.8 and 117.7 ± 5.3, 38.1 ± 1.6 and 48.7 ± 8.0, 312.8 ± 8.8 and 366.6 ± 10.8, 11.6 ± 0.6 and 6.5 ± 0.1 gallic acid equivalent, 2.9 ± 0.1 and 2.3 ± 0.0 rutin equivalent, and 115.9 ± 1.3 and 135.6 ± 0.6, respectively.
Hydroxy-α-sanshool, hydroxy-β-sanshool, hydroxy-γ-sanshool, hydroxy-ε-sanshool, and hydroxy-γ-isosanshool standards were purchased from Chengdu Mai Desheng Technology Co., Ltd. (Chengdu, China). Gallic acid and rutin standards were purchased from Beijing Suolaibao Biotechnology Co., Ltd. (Beijing, China). Commercial cellulase (EC 3.2.1.4, 5000 U g−1), hemicellulase (EC 3.2.1.78, 5000 U g−1) and pectinase (EC 3.2.1.15, 5000 U g−1) were purchased from Nanning Pangbo Biological Co., Ltd. (Nanning, China). Food grade alcohol was procured from Chron Chemical Reagent Co., Ltd. (Chengdu, China). Other chemical reagents were of analytical grade.
2.2. Preparation of the hydrodistillation residue of ZBM and ZADC
The hydrodistillation residue of ZBM and ZADC was prepared according to the method described in our previous study with minor modifications.13 50 g of dry ZBM (or ZADC) was crushed and sieved through a mesh size 40 and then dispersed into 500 ml water and heated to the boil. After distillation for 1.5 h, the vapor was collected as an aromatic essential oil. The residue was collected and weighed to about 100 g after vacuum filtration. Extraction and quantification of the hydroxy-sanshool compounds in these two residues were performed in the following study, and their pungent taste contributions were evaluated.
2.3. Extraction of hydroxy-sanshool compounds with four different conditions
The hydrodistillation residue of 20 g of ZBM (or ZADC) was soaked in 180 ml water for 3 h under the conditions of 50 °C and pH 4.8. The pH level was adjusted using 0.05 M citric acid. Food grade alcohol (200 ml) was then added and extraction was performed at 50 °C for 3 h. The hydroxy-sanshool crude extract was obtained after filtration and vacuum distillation. After addition of 100 ml food grade alcohol, the mixture was centrifuged at 4000 rpm for 15 min, and the supernatant was collected. Finally, the hydroxy-sanshool extract of ZBM, identified as HSE-ZBM, was obtained after vacuum distillation and weighed as M1. In addition, the ethanol–water extractions assisted with (i) enzymes, (ii) ultrasound, and (iii) enzymes and ultrasound were investigated. For the enzyme-assisted ethanol–water extraction, 2.5% (w/w) mixed enzymes (the dosage ratio of cellulose
:
hemicellulose
:
pectinase was 1
:
1
:
1) was added during soaking for the hydroxy-sanshool extraction, and the other steps were the same as mentioned above. For the ultrasound-assisted ethanol–water extraction, ultrasonic irradiation at 150 W was carried out for 30 min and the other steps were the same as the above. For the ethanol–water extraction assisted with enzymes and ultrasound, 2.5% (w/w) mixed enzymes were added during soaking, and the extraction was assisted with ultrasound at 150 W for 30 min. The other steps were the same as above. The yield (%, w/w) of HSE-ZBM (or HSE-ZADC) was calculated as (M1/20) × 100.
2.4. Optimization of the ultrasound-assisted enzymatic extraction by the Box–Behnken design
Based on the single extraction factor results in our preliminary experiments (data not shown), three factors, i.e., enzyme amount (X1), incubation temperature (X2) and ultrasound irradiation power (X3), had a stronger influence on the yields of hydroxy-sanshool extract and were chosen as the independent variables. The yield of hydroxy-sanshool extract was the response value. Optimization of the ultrasound-assisted enzymatic extraction from the ZBM residue was performed using a Box–Behnken design. The analysis of experimental data was performed using analysis of variance (ANOVA). Design-Expert software was employed for experiment design, regression and graphical analysis of the results obtained.
2.5. Scanning electron microscopy (SEM)
Cell destruction of ZBM after the four extraction conditions mentioned in Section 2.3 was observed using scanning electron microscopy (JSM-7500F, JEOL, Japan). The ZBM residue was fixed with glutaraldehyde solution, dehydrated with ethanol solutions, dried by a critical point drying process, fixed on adhesive tape and then sputtered with gold using a sputter coater (JFC-1600, JEOL, Japan) prior to observation.
2.6. Quantification and pungent taste contribution evaluation
2.6.1. High performance liquid chromatography (HPLC). The contents of hydroxy-α-sanshool, hydroxy-β-sanshool, hydroxy-γ-sanshool, hydroxy-ε-sanshool, and hydroxy-γ-isosanshool in HSE-ZBM (or HSE-ZADC) were determined using an HPLC system (HPLC-6AD, Shimadzu, Japan) equipped with a 5 μm (250 × 4.6 mm i.d.) ZORBAX Eclipse Plus C18 column (Agilent, United States). For the sample preparation, 1 g HSE-ZBM (or HSE-ZADC) was dissolved in methanol and filtrated through an alkaline alumina column. After vacuum distillation, the remaining substance was collected and re-dissolved in methanol to 10 ml. For the sample determination, the injection volume was 20 μl and the detection wavelength was 270 nm. Ultrapure water was used as solvent A and acetonitrile was solvent B. The solvents were delivered at a total flow rate of 0.5 ml min−1. The solvent gradient was from 45% B to 50% B linearly in 10 min, a linear increase to 65% B in 20 min then to 100% B in 1 min, followed by 9 min isocratic elution, and then a return to 45% B in 5 min. The five hydroxy-sanshools in the samples were identified by comparing their retention time with that of the reference standards and quantified with an external standard method.
2.6.2. Determination of the pungent taste contribution value (PCV). To recognize the pungent taste contribution and characteristic sensation of each hydroxy-sanshool in ZBM and ZADC, the PCV (ml g−1) was introduced and calculated as C × SPU × 10−3, where C is the content of each hydroxy-sanshool or its isomer in HSE-ZBM or HSE-ZADC. Scoville Pungency Unit (SPU, ml g−1) was the dilution factor per gram of substance, in which its pungent taste could be simply perceived. The SPUs of hydroxy-α-sanshool, hydroxy-β-sanshool, hydroxy-γ-sanshool, hydroxy-ε-sanshool, and hydroxy-γ-isosanshool were 2.31 × 104 ml g−1, 4.86 × 104 ml g−1, 2.78 × 104 ml g−1, 4.63 × 104 ml g−1, and 2.78 × 104 ml g−1, respectively.15
2.6.3. Oral sensory evaluation of ZBM and ZADC powder. Sensation testing was performed according to the literature protocol.16 Quantitative descriptive analysis was used to evaluate the performance of the sensory pungency characteristics of the ZBM and ZADC samples. Ten assessors (aged 23–27 years old) were invited and trained. The attributes and intensities of three pungency sensations, i.e., numbing, tingling and salivation, were perceived quantitatively by using a standard solution containing hydroxy-α-sanshool and hydroxy-β-sanshool with a ratio of 1
:
1. Six pungency intensities of the solution including hydroxy-sanshool contents (mg ml−1) of 0, 0.02, 0.08, 0.14, 0.2, 0.4, were scored at 0 (no sensation), 1 (very weak), 2 (weak), 3 (moderate), 4 (strong) and 5 (very strong), respectively. Assessors were requested not to eat and drink for at least 1 h before the test. Firstly, they were instructed to rinse their oral cavity with 5 ml of water and 7.25 mg ZBM or ZADC powder was taken inside the mouth for 10 s. Secondly, the assessors were asked to spit and to wait for 2 min before gargling with 5 ml of water and scoring the sensation intensity. The assessors repeated the steps described above and the test interval between each sample was 5 min. The tests were conducted in triplicate and the sensory score was expressed as an average value.
2.7. Statistical analysis
Design-Expert software version 8.0 (Stat-Ease company, USA) for the Box–Behnken design was used to determine the optimum extracting process. ANOVA and Duncan's multiple range tests were used to determine the significant differences (p < 0.05) between the means. All the experimental results were expressed as mean ± standard deviation.
3. Results and discussion
3.1. Effects of the extraction conditions on the yield of hydroxy-sanshool extract
ZBM and ZADC, generally known as “honghuajiao” and “qinghuajiao”, are popular spices used worldwide, especially in Asia. They possess different aroma and pungent characteristics, which could be correlated with the composition and content of the volatile and non-volatile flavor compounds. In our previous work, the essential oil of volatile aroma components of ZBM and ZADC was extracted with hydrodistillation, and the potent odorants and typical aroma were identified.13 Meanwhile, the hydrodistillation residue of ZBM and ZADC was produced, and some valuable active compounds, such as hydroxy-sanshools, were retained. So, the hydroxy-sanshool compounds were extracted from the residue by using four conditions, and their extraction yields are shown in Table 2. The content of hydroxy-sanshool compounds in ZBM was much higher than that in ZADC, suggesting a stronger pungent intensity of ZBM. In addition, compared with that of the hydroxy-sanshool extracted with ethanol–water (5.12 ± 0.19% for HSE-ZBM and 3.35 ± 0.04% for HSE-ZADC), the yields of the samples for which the extraction was assisted with enzymes, ultrasound, and enzymes and ultrasound increased by 4.2–7.6%, 9.3–9.8%, and 21.5–26.2%, respectively. Ultrasound-assisted enzymatic extraction achieved a higher yield than the other three extraction conditions (p < 0.05). This result could be attributed to the synergistic effects between mixed enzymes and ultrasound. The mixture of cellulase, hemicellulase and pectinase could disrupt and degrade the cell wall and release the intracellular constituents.17 Sonication not only improved the affinity between the enzyme and substrate, but also enhanced the conversion of the enzyme/substrate complex into the product.18
Table 2 The yields of HSE-ZBM and HSE-ZADC by using four different extraction conditions
Methods |
Yields (%, w/w) |
HSE-ZBM |
HSE-ZADC |
A, B, and C means that different letters within a column were significantly different (P < 0.05) in HSE-ZBM; a, b, and c means that different letters within a column were significantly different (P < 0.05) in HSE-ZADC. |
Ethanol–water extraction |
5.12 ± 0.19Aa |
3.35 ± 0.04a |
Enzyme-assisted ethanol–water extraction |
5.51 ± 0.28AB |
3.49 ± 0.05a |
Ultrasound-assisted ethanol–water extraction |
5.62 ± 0.05BC |
3.66 ± 0.07b |
Ultrasound-assisted enzymatic ethanol–water extraction |
6.46 ± 0.21C |
4.07 ± 0.06c |
Several literatures reported the extraction of alkylamides from Zanthoxylum. Yang and co-workers reported 6% (w/w) yield of the alkylamide extract with hexane/ethyl acetate from the dried pericarps of Z. bungeanum and Z. schinifolium.19 Bhatt et al. obtained 15.1% (w/w) methanol extract of flavonoids, lignans, coumarin and amides from the dried leaves of Z. armatum.20 Nevertheless, the yields of alkylamides or other active compounds depend not only on the extraction methods, but also on the Zanthoxylum growing area, variety, climate, cultivation conditions and sample preparation processes.21,22 Some solvents, including methanol, ethanol, chloroform, petroleum ether, diethyl ether, vegetable oil and water, have been applied for the extraction of pungent and tingling compounds from Zanthoxylum.23,24 Ethanol and water are generally considered as safe and eco-friendly options, whereas organic solvent is not used at an industrial scale due to the toxicity associated with the solvent residue.
3.2. SEM analysis
To investigate the influence of extraction conditions on the matrix, SEM was employed to observe the microscopic structure changes of the ZBM tissues. The cell wall breakage of the sample treated with four extraction conditions is shown in Fig. 1. After ethanol–water extraction (Fig. 1A), the cell shape of the sample remained unchanged and intact, although the cell wall surface was rough and wrinkled and some cell fragments were observed. In the case of the enzyme-assisted ethanol–water extraction (Fig. 1B), huge perforations in the internal surface of the sample cell were observed, suggesting the disruption of the cell wall structure under the combined effects of cellulase, hemicellulase and pectinase. The shape of the sample cells became irregular. Notably, after ultrasound-assisted ethanol–water extraction (Fig. 1C), a jagged edge appeared on the cell wall and the cell structure was partly damaged, which indicated that the cell walls were attacked by the cavitation effects of the ultrasound. As for the extraction assisted with enzymes and ultrasound (Fig. 1D), compared with the other three treatments, the cell walls incurred more serious damage. The cell structure almost disappeared and a mass of cellular fragments was produced. It revealed that the combined effects of enzymes and ultrasound could greatly enhance the disruption of the ZBM cell structure, which facilitated the release of hydroxy-sanshools. This finding was also in good agreement with the high yield of HSE-ZBM extracted with enzyme and ultrasound mentioned above.
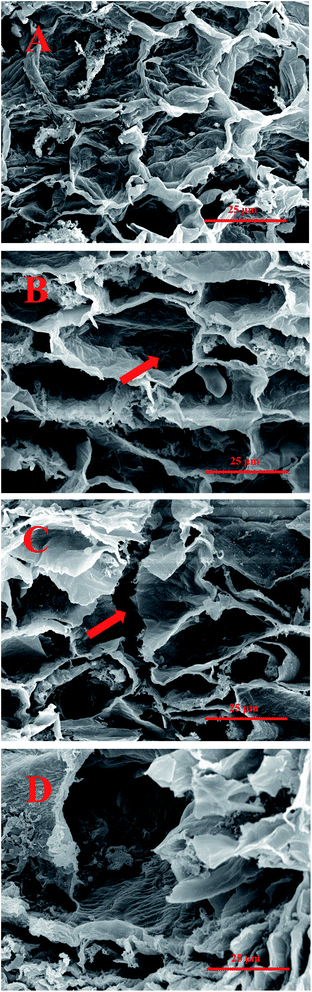 |
| Fig. 1 Electron microscopy of ZBM cells with four extraction conditions. Ethanol–water extraction (A) and extraction assisted with enzymes (B), ultrasound (C), and enzymes and ultrasound (D). | |
3.3. Optimization of the ultrasound-assisted enzymatic extraction of hydroxy-sanshool compounds
In our preliminary experiments, significant variables in the hydroxy-sanshool extraction from the ZBM residue were firstly selected from seven variables, including enzyme amount, incubation pH, incubation temperature, incubation time, ultrasound irradiation power, liquid–solid ratio and ultrasound irradiation time, based on a Plackett–Burman design (data not shown). Three variables, i.e., enzyme amount (X1), incubation temperature (X2) and ultrasound irradiation power (X3), played important roles in the hydroxy-sanshool extraction and were selected for further optimization of the extraction process. A total of 17 experimental conditions were trialed and the results were applied to determine the influence of the variables, as shown in Table 3. The model developed had a p-value of <0.01 and a non-significant lack of fit value of 0.36. The regression equation from the ANOVA had a determination coefficient R2 of 0.9922, which is an estimate of the data variation of the model. “Adequacy precision” measures the signal to noise ratio. A ratio greater than 4 is desirable, and the ratio of 33.44 shows an adequate signal. These model characteristics indicated that the model was significant and could be used to efficiently determine the optimal conditions. Sufficient input levels were used to fit a third-order polynomial equation. The hydroxy-sanshool extract yield (Y) was calculated as follows:
Y = 7.82 + 0.14X1 + 0.09X2 − 0.06X3 − 0.14X1X2 + 0.09X2X3 − 0.38X12 − 0.55X22 − 0.25X32 + 0.25 X1X22 |
Table 3 Experimental design matrix to screen for the variable that determines the hydroxy-sanshool extract yield from the ZBM and ANOVA results
No. |
Box–Behnken design |
ANOVA |
X1 |
X2 |
X3 |
Y (%) |
Source |
Sum of squares |
Degree of freedom |
Mean square |
F-Value |
p-Value |
Actual |
Predicted |
X1 is the amount of enzyme (%), X2 is the incubation temperature (°C), X3 is the ultrasound irradiation power (W), and Y is the total yield. Significant at p < 0.05. |
1 |
3.0 |
45 |
180 |
7.08 |
7.07 |
Modela |
3.22 |
9 |
0.36 |
98.5 |
<0.01b |
2 |
3.0 |
45 |
240 |
6.74 |
6.79 |
X1 |
0.08 |
1 |
0.08 |
20.82 |
<0.01 |
3 |
3.0 |
50 |
210 |
7.80 |
7.82 |
X2 |
0.07 |
1 |
0.07 |
18.34 |
<0.01 |
4 |
2.5 |
50 |
240 |
7.05 |
7.00 |
X3 |
0.03 |
1 |
0.03 |
6.97 |
0.03 |
5 |
3.0 |
50 |
210 |
7.76 |
7.82 |
X1X2 |
0.08 |
1 |
0.08 |
22.36 |
<0.01 |
6 |
3.0 |
50 |
210 |
7.89 |
7.82 |
X2X3 |
0.03 |
1 |
0.03 |
7.96 |
0.03 |
7 |
2.5 |
50 |
180 |
7.06 |
7.11 |
X12 |
0.61 |
1 |
0.61 |
168.24 |
<0.01 |
8 |
3.0 |
50 |
210 |
7.79 |
7.82 |
X22 |
1.27 |
1 |
1.27 |
348.69 |
<0.01 |
9 |
3.5 |
55 |
210 |
7.20 |
7.22 |
X32 |
0.26 |
1 |
0.26 |
71.57 |
<0.01 |
10 |
2.5 |
45 |
210 |
6.30 |
6.28 |
X1X22 |
0.12 |
1 |
0.12 |
33.05 |
<0.01 |
11 |
3.0 |
50 |
210 |
7.87 |
7.82 |
Residual |
0.03 |
7 |
0.01 |
|
|
12 |
3.5 |
50 |
180 |
7.38 |
7.39 |
Lack of fit |
0.02 |
3 |
0.01 |
1.43 |
0.36 |
13 |
3.5 |
45 |
210 |
7.35 |
7.33 |
Pure error |
0.01 |
4 |
0.01 |
|
|
14 |
3.0 |
55 |
180 |
7.14 |
7.09 |
Corrected total |
3.25 |
16 |
|
|
|
15 |
3.5 |
50 |
240 |
7.28 |
7.27 |
Credibility analysis of the regression equations |
16 |
2.5 |
55 |
210 |
6.72 |
6.74 |
Standard deviation |
Mean |
Coefficient of variation |
R2 |
Adequacy precision |
17 |
3.0 |
55 |
240 |
7.14 |
7.15 |
0.06 |
7.27 |
0.83% |
0.9922 |
33.44 |
The ANOVA indicated that the model items X1, X2, X3, X1X2, X2X3, X12, X22, X32, and X1X22 are statistically significant (p < 0.05). The interactions between variables (enzyme amount vs. incubation temperature and incubation temperature vs. ultrasound irradiation power) had a significant influence on the extraction yield, and their contour and response surface plots are shown in Fig. 2. The optimal conditions to maximize hydroxy-sanshool yields could be found from these interactions. The optimum extraction conditions according to the response surface model are: 3.1% (w/w) amount of enzyme, an incubation temperature of 50.3 °C, and an ultrasound irradiation power of 207 W. The predicted highest yield under this condition is 7.84%. In order to verify the reliability of the theoretical model, an experiment was performed using the optimal conditions. The obtained yield of 7.87 ± 0.32 (%, w/w) is almost consistent with the value predicted by the regression model. So, the results predicted by the model were reliable and practical.
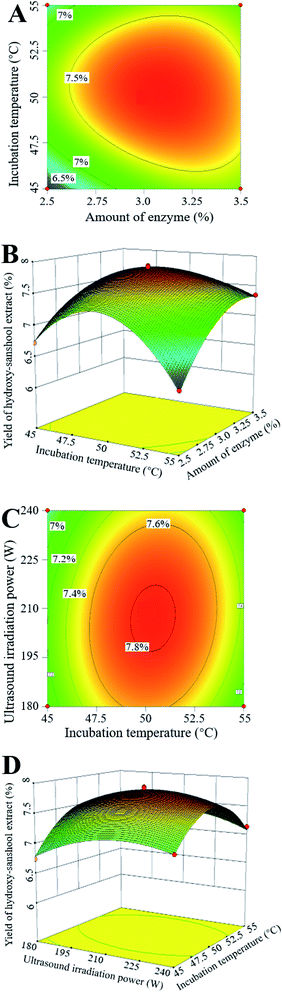 |
| Fig. 2 Contour and response surface plots for the hydroxy-sanshool extraction yield from ZBM showing variable interactions. Contour plot between enzyme amount and incubation temperature (A), response surface plot between enzyme amount and incubation temperature (B), contour plot between incubation temperature and ultrasound irradiation power (C), and response surface plot between incubation temperature and ultrasound irradiation power (D). | |
3.4. Quantification and pungent taste contribution evaluation
Pungency and tingling are the characteristic taste sensations of Sichuan pepper as a spice. Hydroxy-sanshools were considered as the primary pungent active compounds and possessed a potent influence on the pungent taste of ZBM and ZADC.9,15 Five hydroxy-sanshool compounds in both HSE-ZBM and HSE-ZADC were quantitatively measured and their pungent taste contribution value was calculated according to the method described in Section 2.6.2. As shown in Fig. 3 and Table 4, hydroxy-α-sanshool was determined as the main pungent taste component in ZBM with the highest content of 49.92 ± 0.10 mg g−1 and a PCV of 1153 ml g−1, followed by hydroxy-γ-sanshool (16.57 ± 0.58 mg g−1, 461 ml g−1) and hydroxy-β-sanshool (9.19 ± 0.43 mg g−1, 447 ml g−1). With regard to ZADC, hydroxy-α-sanshool (28.53 ± 0.46 mg g−1, 659 ml g−1) and hydroxy-β-sanshool (11.84 ± 0.44 mg g−1, 575 ml g−1) were the main pungent taste components. Hydroxy-ε-sanshool and hydroxy-γ-isosanshool seemed to play a less important role and had a much lower content and PCV in both ZBM and ZADC. According to the oral sensations of five hydroxy-sanshools reported in the literature,8,25 the characteristic pungent sensations of ZBM and ZADC were predicted. ZBM had the major sensations of tingling and salivation, for which the total PCV (1753 ml g−1) was more than thrice that of the numbing sensation (498 ml g−1). In comparison, ZADC shared similar PCVs for the tingling, salivation (778 ml g−1), and numbing (575 ml g−1) sensation.
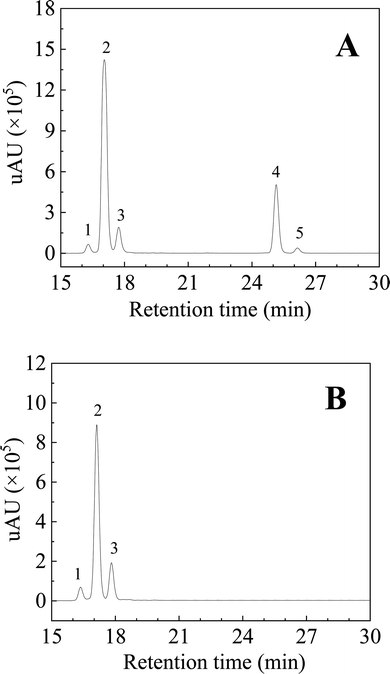 |
| Fig. 3 HPLC chromatograms of HSE-ZBM solution (A) and HSE-ZADC solution (B) at 270 nm. The peaks of 1, 2, 3, 4 and 5 were identified as hydroxy-ε-sanshool, hydroxy-α-sanshool, hydroxy-β-sanshool, hydroxy-γ-sanshool, and hydroxy-γ-isosanshool. | |
Table 4 Quantification and pungent taste contribution value (PCV) evaluation of five hydroxy-sanshool compounds in HSE-ZBM and HSE-ZADC
Compounds |
Content (mg g−1) |
PCV (ml g−1) |
Oral sensationa |
HSE-ZBM |
HSE-ZADC |
HSE-ZBM |
HSE-ZADC |
The data of oral sensation is from the reference by Bader et al. (2014). ND represents that the level is lower than the limit of detection. |
Hydroxy-α-sanshool |
49.92 ± 0.10 |
28.53 ± 0.46 |
1153 |
659 |
Tingling, salivation |
Hydroxy-β-sanshool |
9.19 ± 0.43 |
11.84 ± 0.44 |
447 |
575 |
Numbing |
Hydroxy-γ-sanshool |
16.57 ± 0.58 |
NDb |
461 |
ND |
Tingling, salivation |
Hydroxy-ε-sanshool |
2.30 ± 0.02 |
2.56 ± 0.04 |
139 |
119 |
Tingling, salivation |
Hydroxy-γ-isosanshool |
1.85 ± 0.06 |
ND |
51 |
ND |
Numbing |
The characteristic taste sensations were verified using an oral sensory evaluation for ZBM and ZADC powder, and the results are summarized in Fig. 4. The numbing score of ZBM (3.35) is nearly equal to that of ZADC (3.45) with no significant difference (p > 0.05). The salivation and tingling scores of ZBM were 4.00 and 4.55, respectively, which were significantly higher than those of ZADC (p < 0.05). This result implied that ZBM was inclined to the sensations of tingling and salivation and ZADC had nearly equal shares in tingling and numbing sensations. This finding was in accordance with the PCV analysis mentioned above.
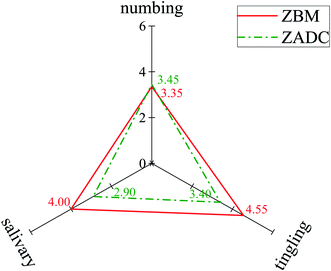 |
| Fig. 4 Oral sensory evaluations of ZBM and ZADC. | |
4. Conclusions
An efficient approach for the extraction of hydroxy-sanshool compounds from the hydrodistillation residue of ZBM and ZADC was developed and optimized by Box–Behnken design. The optimal ultrasound-assisted enzymatic extraction conditions were as follows: 3.1% (w/w) amount of enzyme, an incubation temperature of 50.3 °C, and an ultrasound irradiation power of 207 W. The yield of HSE-ZBM was 7.87 ± 0.32% (w/w) under the optimal conditions. Furthermore, five hydroxy-sanshools in HSE-ZBM and HSE-ZADC were quantified and their pungent taste contribution was recognized. ZBM had the primary pungent taste components of hydroxy-α-sanshool and hydroxy-γ-sanshool, giving the main oral sensations of tingling and salivation. On the other hand, ZADC possessed the dominant pungent compounds of hydroxy-α-sanshool and hydroxy-β-sanshool, exhibiting nearly equal shares in tingling and numbing sensations.
Conflicts of interest
The authors state no conflict of interest.
Acknowledgements
This work was supported by the Key Research and Development Projects of Sichuan Province (no. 2020YFN0151 and no. 2019YFS0063). We would like to acknowledge the volunteers for actively participating in the sensory tests and Dr Qixian Zhang for the helpful discussion.
References
- L. Ranawat, J. Bhatt and J. Patel, J. Ethnopharmacol., 2010, 127, 770–780 CrossRef.
- M. M. Zhang, J. L. Wang, L. Zhu, T. Li, W. D. Jiang, J. Zhou, W. Peng and C. J. Wu, Int. J. Mol. Sci., 2017, 18, 2172 CrossRef.
- Y. Lan, Q. Wu, Y. Q. Mao, Q. Wang, J. An, Y. Y. Chen, W. P. Wang, B. C. Zhao, N. Liu and Y. W. Zhang, J. Zhejiang Univ., Sci., B, 2014, 15, 153–164 CrossRef CAS.
- X. L. Lei, S. R. Cheng, H. L. Peng, Q. He, H. Y. Zhu, M. Q. Xu, Q. Q. Wang, L. Liu, C. S. Zhang, Q. Z. Zhou, F. Zeng, J. Ying, H. Y. Yin and S. G. Yu, Afr. J. Tradit., Complementary Altern. Med., 2016, 13, 45–52 CrossRef CAS.
- H. Lei, J. Wu, Q. Wang, A. X. Su, M. Xue, Q. Liu and Q. H. Hu, Food Funct., 2017, 8, 1569–1576 RSC.
- L. C. Yang, R. Li, J. Tan and Z. T. Jiang, J. Agric. Food Chem., 2013, 61, 1772–1778 CrossRef CAS.
- K. Y. Li, R. Zhou, W. W. Jia, Z. Li, J. Z. Li, P. F. Zhang and T. C. Xiao, J. Ethnopharmacol., 2016, 186, 351–361 CrossRef CAS.
- M. Bader, T. D. Stark, C. Dawid, S. Losch and T. Hofmann, J. Agric. Food Chem., 2014, 62, 2479–2488 CrossRef CAS.
- Z. F. Zhao, R. X. Zhu, K. Zhong, Q. He, A. M. Luo and H. Gao, J. Food Sci., 2013, 78, C1516–C1522 CrossRef CAS.
- S. Murakami, Y. Sudo, K. Miyano, H. Nishimura, M. Matoba, S. Shiraishi, H. Konno and Y. Uezono, J. Pharmacol. Sci., 2016, 130, 72–77 CrossRef CAS.
- C. E. Riera, C. Menozzi-Smarrito, M. Affolter, S. Michlig, C. Munari, F. Robert, H. Vogel, S. A. Simon and J. le Coutre, Br. J. Pharmacol., 2009, 157, 1398–1409 CrossRef CAS.
- C. C. Galopin, S. M. Furrer and A. Goeke, Challenges in taste chemistry and biology, 2004, 867, pp. 139–152 Search PubMed.
- R. X. Zhu, K. Zhong, W. C. Zeng, X. Y. He, X. Q. Gu, Z. F. Zhao and H. Gao, Afr. J. Microbiol. Res., 2011, 5, 4631–4637 CAS.
- S. Wang, J. C. Xie, W. Yang and B. G. Sun, J. Liq. Chromatogr. Relat. Technol., 2011, 34, 2640–2652 CrossRef CAS.
- L. L. Zhang, L. Zhao, H. Y. Wang, B. L. Shi, L. Y. Liu and Z. X. Chen, J. Sci. Food Agric., 2019, 99, 1475–1483 CrossRef CAS.
- L. L. Zhang, S. S. Xu, B. L. Shi, H. Y. Wang, L. Y. Liu, K. Zhong, L. Zhao and Z. X. Chen, J. Sens. Stud., 2018, 33, e12465 CrossRef.
- K. X. Hou, X. B. Yang, M. L. Bao, F. L. Chen, H. Tian and L. Yang, Ind. Crops Prod., 2018, 117, 205–215 CrossRef CAS.
- S. Singh, M. Agarwal, A. Bhatt, A. Goyal and V. S. Moholkar, Bioresour. Technol., 2015, 192, 636–645 CrossRef CAS.
- X. G. Yang, J. Agric. Food Chem., 2008, 56, 1689–1696 CrossRef CAS.
- V. Bhatt, S. Sharma, N. Kumar, U. Sharma and B. Singh, J. Pharm. Biomed. Anal., 2017, 132, 46–55 CrossRef CAS.
- X. H. Wang, S. C. Liang, D. M. Ma, C. Xu, H. H. Liu, Z. Z. Han, W. F. Wei and Q. S. Guo, Ind. Crops Prod., 2020, 150, 112–345 Search PubMed.
- Z. C. Wu, W. Wang, L. W. Sun, A. Z. Wei and D. M. Wang, Ind. Crops Prod., 2020, 145, 111998 CrossRef CAS.
- Y. Ma, X. Li, L. X. Hou and A. Z. Wei, Ind. Crops Prod., 2019, 142, 111872 CrossRef CAS.
- C. H. Wijaya, F. I. Napitupulu, V. Karnady and S. Indariani, Food Rev. Int., 2019, 35, 1–19 CrossRef CAS.
- E. Sugai, Y. Morimitsu, Y. Iwasaki, A. Morita, T. Watanabe and K. Kubota, Biosci., Biotechnol., Biochem., 2005, 69, 1951–1957 CrossRef CAS.
|
This journal is © The Royal Society of Chemistry 2021 |
Click here to see how this site uses Cookies. View our privacy policy here.