DOI:
10.1039/D0RA08822F
(Paper)
RSC Adv., 2021,
11, 3288-3294
Simplification of gold nanoparticle synthesis with low cytotoxicity using a greener approach: opening up new possibilities
Received
16th October 2020
, Accepted 28th December 2020
First published on 14th January 2021
Abstract
Gold nanoparticles (AuNPs) have diverse applications in the diagnosis and treatment of ailments. This study describes an extremely simplified synthesis of AuNPs using antioxidant-rich pollen extract as a local natural source. Ultraviolet-visible (UV-vis) spectroscopy, X-ray diffraction (XRD), Fourier-transform infrared spectroscopy (FTIR) and transmission electron microscopy (TEM) were used to characterize the synthesized AuNPs; strong UV-vis absorption at 534 nm confirmed their formation, the XRD pattern showed the presence of a crystalline structure, and TEM images showed them to be spherical nanoparticles with an average size of 9.3 ± 2.9 nm. As synthesized AuNPs remained stable for up to two months under laboratory conditions without any sedimentation or change in the absorption value, presumably due to the protection afforded by the capping agents from pollen. AuNPs revealed low toxicity effects on MCF-7 and HUVECs cell lines (with an IC50 value of ∼400 μg mL−1 for both the cell lines). The proposed method did not use any hazardous materials or high-energy consuming devices; thus this efficient protocol may be adapted for large-scale production using local resources.
1. Introduction
Metallic nanoparticles (NPs), including gold, iron, nickel, copper and silver, are the subject of many investigations due to their wide range of applications in various scientific disciplines such as medicine, physics and chemistry.1–4 Gold nanoparticles (AuNPs) have been used in biological sciences, pharmaceuticals, catalysis, immunization and cancer therapy.5,6 There are different physical,7 chemical8 and biological9 methods for the synthesis of NPs. NP synthesis by physical and chemical methods has some disadvantages namely multi-stage synthesis processes,10 dependence on specialized equipment,11 energy dependence,12 and use hazardous chemicals13 that pollute the environment.3,14,15 Therefore, direct or indirect biological synthesis using living organisms such as bacteria, fungi and plants has been considered.16,17 On the other hand, due to the potential pathogenicity of some microbial agents and difficulty of cultivation of actinomycetes, bacteria and fungi, and the need for complex and expensive media, nowadays biological synthesis of NPs, especially gold, iron, and silver using different herbs has been considered. Among others, synthesis of gold nanostructures has been reported using a variety of plants such as Aloe vera,18 alfalfa,19 Citrus aurantium L. blossoms and Rose damascena oils,20 plant surfactants and biological approaches.
The deployment of biological methods for NPs synthesis is protective to the environment, as these protocols, in the most extent, entails low-energy consumption, and avoids the use of solvents and toxic chemicals. The aim of this study has been to develop an eco-friendly synthesis of AuNPs using pine pollen (from male pinecones of the pine tree), as a low-cost procedure that exploits the safe bio-resource for the greener synthesis of AuNPs at room temperature without using environmentally harmful substances. Pollen extracts are known to be rich source of antioxidants and anti-inflammatory agents21 and hence can be ideal source for reduction of salts. The synthesized AuNPs are characterized and their toxicity evaluation revealed them to be safe.
2. Materials and methods
2.1. Materials and cells
Hydrogen tetrachloroaurate(III) (HAuCl4) tetrahydrate was purchased from Merck (Germany), 3-(4,5-dimethylthiazol-2-yl)-2,5-diphenylte-tetrazolium bromide (MTT) was procured from Sigma-Aldrich (USA), and dimethyl sulfoxide (DMSO) was obtained from Sequoia Research Products. A human breast cancer cell line of MCF-7 and a human umbilical vein endothelial cell (HUVEC) line were secured from cell bank of Stem Cell Research Center, Kerman University of Medical Sciences. The cells were cultured in RPMI 1640 medium at 37 °C in a 5% CO2 atmosphere with a 95% humidity. Deionized water was used throughout the study.
2.2. Synthesis of AuNPs
Pollen containing male pine cones were picked up from a pine tree in the spring. Male pine cones (2 g) were washed with water for 10 s to remove the surface dust, and then air dries at room temperature. Dried cones (1 g) was added to an Erlenmeyer flask containing 50 mL water, kept at room temperature for 12 h, and then filtered using a Whatman filter paper (no. 40). The filtered extract had pH = 5.6. 2 mL extract was added into 10 mL HAuCl4 of 0.1 mM dissolved in water and manually mixed to complete the reaction within 2 h and the ensuing mixture was used for further evaluations.
2.3. Characterisation of nanoparticles
In order to investigate the physicochemical properties of AuNPs, the reaction mixture was evaluated by the following methods: UV-vis spectroscopy was performed by a spectrophotometer of Scan Drop from Analytic Jena (Germany) in the wavelength range of 400–700 nm. X-ray diffraction (XRD) analysis was performed by an X-ray diffractometer of X PERTPRO, PANalitical (the Netherlands) at a radiation wavelength of 1.54 Å and 2θ range of 20°–85°. For XRD sample preparation, 90 mL of the AuNPs suspension was centrifuged at 15
000 rpm for 10 min and was washed with water three times. The final sediment was dried at 60 °C for 24 h and the resulting powder was used. Fourier-transform infrared spectroscopy (FTIR) was done using a Tensor 27 spectrometer (Germany). For FTIR sample preparation, 10 mL of the extract alone was centrifuged at 6000 rpm for 6 min, and the supernatant solution was dried at 60 °C for 48 h. The resultant precipitate was analyzed by FTIR. Separately, 10 mL of the AuNPs suspension was centrifuged at 6000 rpm for 10 min, and washed with water three times. The final sediment was dried at 60 °C for 24 h and the resulting powder was analyzed by FTIR analysis of AuNPs. The shape, size and size distribution of AuNPs were studied using transmission electron microscopy (TEM) under a Carl ZEISS microscope (Germany) at an accelerating voltage of 80 kV. For TEM sample preparation, 5 mL of the AuNPs suspension was sonicated for 5 min, a droplet of the suspension was placed on a copper grid, and let to dry at room temperature.
2.4. Cytotoxicity assessment of AuNPs
MTT assay was used for evaluation of cytotoxicity of AuNPs toward MCF-7 cell (as a cancerous) and HUVEC (as a normal) lines. The cell lines were exposed to serial concentrations (0.78–800 μg mL−1) of AuNPs for 24 h. After incubation, 10 μL MTT was added into each well of ELISA plates and incubated for 4 h at 37 °C. Finally, DMSO was added and optical density was measured at 590 nm.
3. Results
During the synthesis of AuNPs, the reaction progress could be monitored with the naked eye upon reduction of gold ions to the metallic state; color change of the extract from light yellow to red after addition of gold ions was the first sign of greener synthesis of AuNPs, (Fig. 1). The color change progression, depicting the reaction, occurred in less than an hour with no change in the color of the control extract (without addition of HAuCl4).
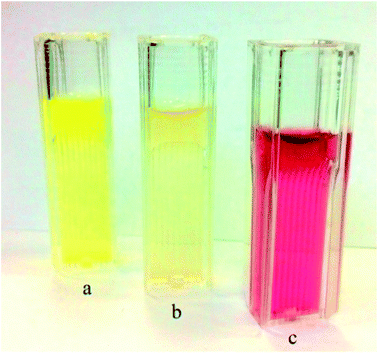 |
| Fig. 1 Colors of HAuCl4 solution (a), pine pollen extract (b), and biosynthesized AuNPs (c). | |
Absorption spectra of the pine pollen extract and the AuNPs dispersion are presented in Fig. 2. While the pine pollen extract had no noticeable absorption, the AuNPs dispersion showed a strong absorption peak at 534 nm indicating that the synthesis of AuNPs was successfully accomplished using the extract. The as-synthesized AuNPs remained stable at least for 2 months at 27 °C in dark without sedimentation or aggregation.
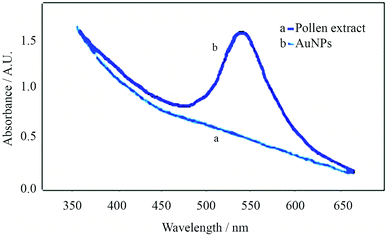 |
| Fig. 2 Absorption spectra of the pine pollen extract (a) and the AuNPs dispersion (b). | |
Fig. 3 depicts an XRD pattern of AuNPs. The diffractogram showed strong peaks at 38°, 44°, 64°, 77° and 82° which are related to crystalline planes of (1 1 1), (2 0 0), (2 2 0), (3 1 1) and (2 2 2), respectively. The XRD pattern also showed the presence of AuNPs crystals in the cubic structure.
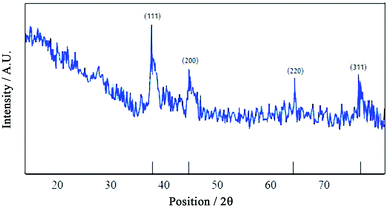 |
| Fig. 3 XRD pattern of AuNPs. | |
Fig. 4 shows the FTIR spectra of the dried pine pollen extract and AuNPs. Spectrum of the extract indicated the presence of different functional groups, such as phenol, amide and carboxylic (Lee et al., 2009), as part of the antioxidants. In the spectrum of AuNPs, bands located at 1628 and 1383 cm−1 could be ascribed to carboxylic (C
O) group and C–O bond, respectively. The strong peak at 1073 cm−1 also corresponded to an amide (N–H) group. Although the exact composition of the extract is unknown and is complex (Lee et al., 2009), it appears that the aforementioned groups would play the major role in the reduction of gold ions, while the constituents bearing amide and carboxylic groups would play the major role of stabilization of AuNPs.
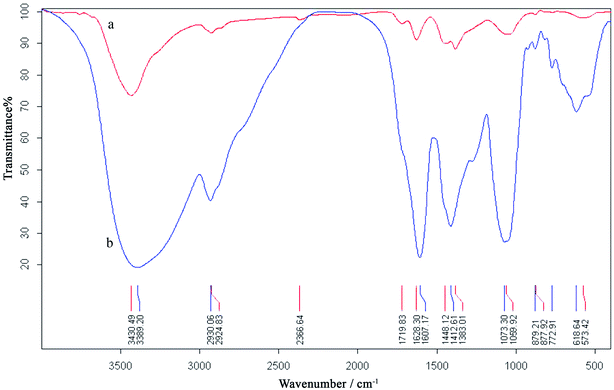 |
| Fig. 4 FTIR spectra of the dried pine pollen extract (a) and AuNPs (b). | |
A TEM image from AuNPs and the corresponding size distribution histogram are shown in Fig. 5. The image showed spherical nanoparticles with an average size of 9.3 ± 2.9 nm.
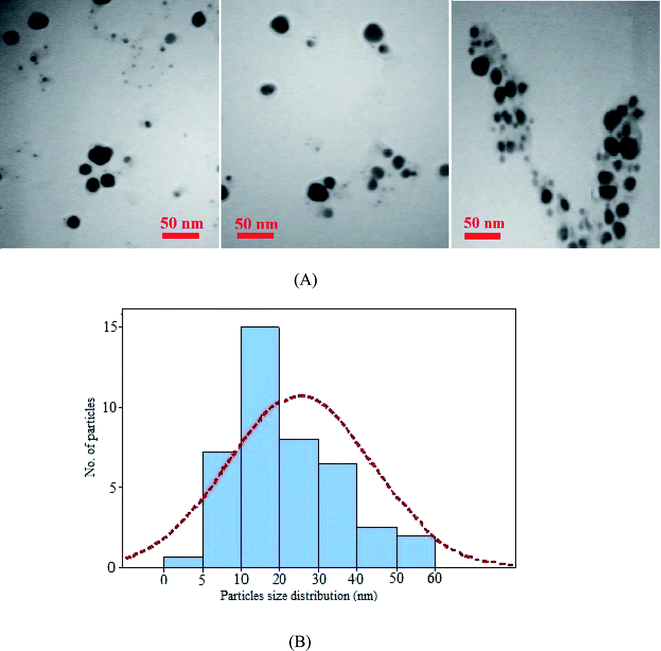 |
| Fig. 5 A TEM image from AuNPs (A) and a size distribution histogram (B). | |
Cytotoxicity of the ensuing AuNPs was evaluated in vitro, and the results, as dependency of the cell viability on the AuNPs concentration, are presented in Fig. 6; an IC50 value of ∼400 μg mL−1 was attained for both the cell lines. Therefore, cytotoxicity of AuNPs toward both the cells was negligible, compared to anticancer drugs such as doxorubicin with an IC50 value of ∼10 μg mL−1.22
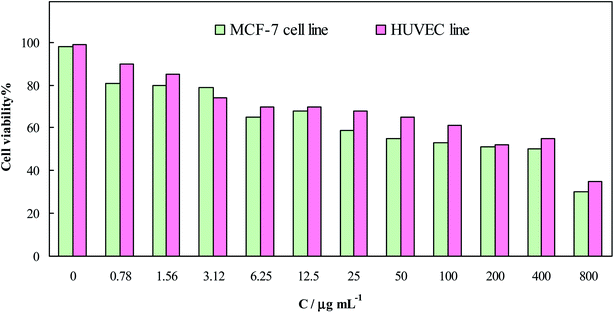 |
| Fig. 6 Dependency of viability of MCF-7 cell and HUVEC lines on the AuNPs concentration. | |
4. Discussion
In the present study, a greener strategy is developed for the synthesis of AuNPs without use of any chemical solvents; pine pollen extract showed displayed potential for the formation and stabilization of AuNPs. Therefore, this low-cost method, exploiting the local resources, has a potential to be used as a biological source for generating water-soluble AuNPs on a larger scale production. UV-vis absorption spectra of AuNPs is similar to those reported for chemically synthesized ones.23 Change in the color of the synthesis mixture and the peak at 534 nm in UV-vis spectrum could be attributed to the surface plasmon resonance of AuNPs arising from the massive oscillations of free electrons upon interaction with light. The FTIR results confirmed stabilization of AuNPs by constituents bearing amide and acid functional groups. Up to now, proteins,24 carbohydrates,25 flavonoids,26 Citrus aurantium L. blossoms and Rose damascena oils20 have been reported as natural compounds for the synthesis of AuNPs. XRD results were quite in line with those reported for chemically synthesized AuNPs.27 TEM image confirmed that the AuNPs size was smaller than those reported previously;28 AuNPs in a size range of 30–60 nm have been produced using Pycnoporus sanguineus.29 In order to inspect the repeatability and reproducibility of the synthesis method, AuNPs were synthesized under different conditions, UV-vis spectra were recorded, and relative standard deviation (RSD) of the absorbance values were obtained. Three independent syntheses were performed over one day (intra-day synthesis) and over three days (inter-day synthesis) using the same precursors (HAuCl4 and the extract); RSD values of 1.5% and 2.0% were obtained for these assays. In addition, three independent extractions were conducted from pine pollen procured from the same tree, and AuNPs were synthesized; a RSD value of 5.7% was attained for the absorbance values. While three independent extractions were performed from pine pollen taken from different trees located at the same geographical region, RSD values for the synthesized AuNPs was 8.1%. Thus, the repeatability and reproducibility of the synthesis method was quite good and satisfactory (Table 1).
Table 1 Provides comparison with the earlier reported methods of plant-mediated AuNPs synthesis in terms of size, organs of the plant, stability, cytotoxic features
Plant |
Organs of the plant |
Size (nm) |
Stability (days) |
Cytotoxic features |
Ref. |
NM: not mentioned. |
Aegle marmelos and Eugenia jambolana |
Fruit |
An average 18–28 |
More than 150 |
IC50 ∼ 163 μg mL−1 |
30 |
Nerium oleander |
Stem bark |
10–100 |
Several months |
IC50 ∼ 74 μg mL−1 |
31 |
Platycodon grandiflorum |
Leaves |
3 to 80 with an average 15 |
NM |
NM |
32 |
Pongamia pinnata |
Leaves |
5–20 |
NM |
NM |
33 |
Dracocephalum kotschyi |
Leaves |
7–22 |
150 |
IC50 ∼ 152 μg mL−1 K 562 and 196 μg mL−1 against HeLa cell line |
34 |
Taxus baccata |
Needles |
Less than 20 |
More than 180 |
The maximum cell mortality of 22.15 and 26.5% were obtained after 72 h |
35 |
Moringa oleifera |
Flower |
3 to 5 with triangular shapes |
NM |
An effect was not observed in the cancerous A549 cells |
36 |
Cryptolepis buchanani |
The whole plant |
An average 11 |
NM |
NM |
37 |
Terminalia catappa |
Leaves |
10 to 35 with average of 21 |
120 |
NM |
38 |
Carica papaya and Catharanthus roseus |
Leaves |
Less than 20 |
NM |
Cell death was about 98% was found at 250 μg mL−1 AuNPs: used against MCF7 and HepG2 cell lines. |
39 |
Jasminum auriculatum |
Leaves |
8–37 |
In PBS was 2 |
IC50 value of 104 μg mL−1 against the human cervical cancer cell line |
40 |
Pine tree |
Pine pollen |
An average 9 |
Up to 60 |
IC50 value of 400 μg mL−1 |
This study |
Although not all the reported methods for synthesis of AuNPs generally require environmentally detrimental chemicals, synthesis using plants is more appropriate than those used fungal or microbial entities due to the need for additional culture and/or separation steps. Furthermore, our method described herein does not need any sophisticated laboratory devices and deploy local resources; Table 2 provides a comparison with the earlier reported methods for AuNPs synthesis from point of view of special devices with energy consumption.
Table 2 A comparison between the reported methods of AuNPs synthesis in terms of use of laboratory devices and energy consumptiona
Bioresource |
Water bath |
Centrifuge |
Mixer |
Heater |
Culture medium |
Laminar air flow |
Incubator |
Ref. |
− No need, + need. |
Streptomyces microflavus |
− |
+ |
− |
+ |
+ |
+ |
+ |
41 |
Sumac |
− |
+ |
+ |
+ |
− |
− |
+ |
42 |
Garcinia mangostana |
− |
− |
+ |
+ |
− |
− |
+ |
43 |
Black cardamom |
− |
+ |
+ |
− |
− |
− |
− |
44 |
Salvia officinalis |
− |
+ |
+ |
+ |
− |
− |
+ |
45 |
Lippia citriodora |
− |
+ |
+ |
+ |
− |
|
+ |
45 |
Peanut skin |
− |
− |
+ |
+ |
− |
+ |
+ |
46 |
Deinococcus radiodurans |
+ |
+ |
− |
+ |
+ |
+ |
+ |
47 |
Dendropanax morbifera |
− |
+ |
− |
+ |
− |
− |
+ |
48 |
leuropterus multiflorus |
− |
+ |
+ |
+ |
− |
− |
+ |
49 |
Ocimum sanctum |
− |
+ |
+ |
+ |
− |
− |
+ |
50 |
Aspergillus niger |
− |
+ |
+ |
+ |
+ |
+ |
+ |
51 |
Aspergillus terreus |
− |
+ |
+ |
+ |
+ |
+ |
+ |
52 |
Aegle marmelos |
+ |
− |
− |
+ |
− |
− |
+ |
53 |
Botrytis cinerea |
+ |
+ |
+ |
+ |
+ |
+ |
+ |
54 |
Olive |
− |
− |
+ |
+ |
− |
− |
+ |
55 |
Melissa officinalis |
− |
− |
+ |
+ |
− |
− |
+ |
56 |
Mentha piperita |
− |
− |
+ |
+ |
− |
− |
+ |
56 |
Salvia officinalis |
− |
− |
+ |
+ |
− |
− |
+ |
56 |
Marine algae |
|
+ |
+ |
+ |
|
|
+ |
57 |
Actinomycetes |
+ |
+ |
+ |
+ |
+ |
+ |
+ |
58 |
Cucurbita pepo |
+ |
− |
+ |
+ |
− |
− |
+ |
59 |
Coffea arabica |
+ |
− |
+ |
+ |
− |
− |
+ |
60 |
Pine pollen |
− |
− |
− |
− |
− |
− |
− |
This study |
In other words, the fact that no mechanical stirring was needed in this study is simply because the volumes used needed no mechanical stirring.
5. Conclusion
In brief, a greener synthesis of AuNPs is described method that occurs under ambient temperature and pressure without using chemical solvents. The ensuing AuNPs exhibited XRD pattern revealing the crystalline structure of AuNPs while the TEM image confirm the formation of spherical NPs with an average size of 9.3 ± 2.9 nm. As synthesized AuNPs remained stable for up to two months under laboratory conditions, without any sedimentation or aggregation they displayed low toxicity indicating the possible applications in different areas of biological research. Due to simple procedure, employing a low-cost extract and high yield of AuNPs preparation, the proposed method can be scaled up for large-scale production of AuNPs and may stimulate further investigations.
Conflicts of interest
There are no conflicts to declare.
Acknowledgements
We would like to thank The Research Council of Shahid Beheshti and Bam University of Medical Sciences (grant F. Borhani and M. Khatami) for supporting this research. We would also thank Dr Iraj Sharifi for his valuable helps.
References
- M. Khatami, H. Q. Alijani, B. Fakheri, M. M. Mobasseri, M. Heydarpour, Z. K. Farahani and A. U. Khan, J. Cleaner Prod., 2019, 208, 1171–1177 CrossRef.
- G. Sargazi, D. Afzali, A. Mostafavi, A. Shadman, B. Rezaee, P. Zarrintaj, M. R. Saeb, S. Ramakrishna and M. Mozafari, Heliyon, 2019, 5, e01527 CrossRef.
- M. Safaei, M. M. Foroughi, N. Ebrahimpoor, S. Jahani, A. Omidi and M. Khatami, TrAC, Trends Anal. Chem., 2019, 118, 401–425 CrossRef CAS.
- R. Nazari-Vanani, N. Sattarahmady, H. Yadegari, M. Khatami and H. Heli, Biosens. Bioelectron., 2019, 142, 111541 CrossRef CAS.
- Z. U. H. Khan, A. Khan, Y. Chen, N. S. Shah, N. Muhammad, A. U. Khan, K. Tahir, F. U. Khan, B. Murtaza, S. U. Hassan, S. A. Qaisrani and P. Wan, J. Photochem. Photobiol., B, 2017, 173, 150–164 CrossRef.
- J. A. Salazar-González, O. González-Ortega and S. Rosales-Mendoza, Expert Rev. Vaccines, 2015, 14, 1197–1211 CrossRef.
- S. Iravani, H. Korbekandi, S. V. Mirmohammadi and B. Zolfaghari, Res. Pharm. Sci., 2014, 9, 385–406 CAS.
- A. Miri, M. Khatami and M. Sarani, J. Inorg. Organomet. Polym. Mater., 2020, 30, 767–774 CrossRef CAS.
- J. Miri, S. Darroudi and S. Sarani, Appl. Organomet. Chem., 2020, 34, 5308–5309 CrossRef.
- S. Ghazal, A. Akbari, H. A. Hosseini, Z. Sabouri, M. Khatami and M. Darroudi, Inorg. Chem. Res., 2021, 5, 37–49 Search PubMed.
- S. Scaramuzza, M. Zerbetto and V. Amendola, J. Phys. Chem. C, 2016, 120, 9453–9463 CrossRef CAS.
- J. Maroušek, S. Hašková, A. Maroušková, K. Myšková, R. Vaníčková, J. Váchal, M. Vochozka, R. Zeman and J. Žák, Energy Sources, Part A, 2015, 37, 1723–1728 CrossRef.
- K. Karthik, S. Dhanuskodi, C. Gobinath, S. Prabukumar and S. Sivaramakrishnan, Mater. Res. Innovations, 2018, 23(5), 1–9 Search PubMed.
- J. Maroušek, V. Stehel, M. Vochozka, A. Maroušková and L. Kolář, J. Cleaner Prod., 2018, 199, 173–176 CrossRef.
- M. Khatami, I. Sharifi, M. A. L. Nobre, N. Zafarnia and M. R. Aflatoonian, Green Chem. Lett. Rev., 2018, 11, 125–134 CrossRef CAS.
- S. Azhdari, R. E. Sarabi, N. Rezaeizade, F. Mosazade, M. Heidari, F. Borhani, M. Abdollahpour-Alitappeh and M. Khatami, RSC Adv., 2020, 10, 29737–29744 RSC.
- A. Alinaghi Langari, S. Soltaninezhad, N. Zafarnia, M. Heidari, R. S. Varma, Z. Ebrahimi, S. Azhdari, F. Borhani and M. Khatami, Bioprocess Biosyst. Eng., 2020, 43, 1–7 CrossRef.
- S. P. Chandran, M. Chaudhary, R. Pasricha, A. Ahmad and M. Sastry, Biotechnol. Prog., 2006, 22, 577–583 CrossRef CAS.
- M. O. Montes, A. Mayoral, F. L. Deepak, J. G. Parsons, M. Jose-Yacamán, J. R. Peralta-Videa and J. L. Gardea-Torresdey, J. Nanopart. Res., 2011, 13, 3113–3121 CrossRef CAS.
- N. Sattarahmady, V. Firoozabadi, R. Nazari-Vanani and N. Azarpira, Int. J. Biol. Macromol., 2018, 112, 703–711 CrossRef CAS.
- F. Schulte, J. Lingott, U. Panne and J. Kneipp, Anal. Chem., 2008, 80, 9551–9556 CrossRef CAS.
- X. Wang, Z. Teng, H. Wang, C. Wang, Y. Liu, Y. Tang, J. Wu, J. Sun, H. Wang, J. Wang and G. Lu, Int. J. Clin. Exp. Pathol., 2014, 7, 1337–1347 Search PubMed.
- M. E. El-Naggar, T. I. Shaheen, M. M. G. Fouda and A. A. Hebeish, Carbohydr. Polym., 2016, 136, 1128–1136 CrossRef CAS.
- P. Ravindra, Mater. Sci. Eng., B, 2009, 163, 93–98 CrossRef CAS.
- E. Filippo, A. Serra, A. Buccolieri and D. Manno, J. Non-Cryst. Solids, 2010, 356, 344–350 CrossRef CAS.
- E. Egorova and A. Revina, Colloids Surf., A, 2000, 168, 87–96 CrossRef CAS.
- C. Engelbrekt, K. H. Sorensen, J. Zhang, A. C. Welinder, P. S. Jensen and J. Ulstrup, J. Mater. Chem., 2009, 19, 7839–7847 RSC.
- K. B. Narayanan and N. Sakthivel, Mater. Lett., 2008, 62, 4588–4590 CrossRef CAS.
- C. Shi, N. Zhu, Y. Cao and P. Wu, Nanoscale Res. Lett., 2015, 10, 147 CrossRef.
- S. Vijayakumar, J. Saudi Chem. Soc., 2019, 23, 753–761 CrossRef.
- A. C. Barai, K. Paul, A. Dey, S. Manna, S. Roy, B. G. Bag and C. Mukhopadhyay, Nano Convergence, 2018, 5, 10 CrossRef.
- P. Anbu, S. C. Gopinath and S. Jayanthi, Nanomater. Nanotechnol., 2020, 10, 1847980420961697 Search PubMed.
- A. Khatua, E. Priyadarshini, P. Rajamani, A. Patel, J. Kumar, A. Naik, M. Saravanan, H. Barabadi, A. Prasad, l. Ghosh, B. Paul and R. Meena, J. Cluster Sci., 2020, 31, 125–131 CrossRef CAS.
- N. Dorosti and F. Jamshidi, J. Appl. Biomed., 2016, 14, 235–245 CrossRef.
- A. A. Kajani, A.-K. Bordbar, S. H. Z. Esfahani and A. Razmjou, RSC Adv., 2016, 6, 63973–63983 RSC.
- K. Anand, R. Gengan, A. Phulukdaree and A. Chuturgoon, J. Ind. Eng. Chem., 2015, 21, 1105–1111 CrossRef CAS.
- K. Wongyai, P. Wintachai, R. Maungchang and P. Rattanakit, J. Nanomater., 2020, 2020, 1320274 CrossRef.
- B. Ankamwar, Eur. J. Chem., 2010, 7, 745120 Search PubMed.
- T. Muthukumar, B. Sambandam, A. Aravinthan, T. P. Sastry and J.-H. Kim, Process Biochem., 2016, 51, 384–391 CrossRef CAS.
- S. Balasubramanian, S. M. J. Kala and T. L. Pushparaj, J. Drug Delivery Sci. Technol., 2020, 57, 101620 CrossRef CAS.
- M. S. Nejad, M. Khatami and G. H. S. Bonjar, IET Nanobiotechnol., 2016, 10, 33–38 CrossRef.
- H. Shabestarian, M. Homayouni-Tabrizi, M. Soltani, F. Namvar, S. Azizi, R. Mohamad and H. Shabestarian, Materials Research, 2017, 20, 264–270 CrossRef CAS.
- K. Xin Lee, K. Shameli, M. Miyake, N. Kuwano, N. B. Bt Ahmad Khairudin, S. E. Bt Mohamad and Y. P. Yew, J. Nanomater., 2016, 2016, 7 Search PubMed.
- A. K. Singh and O. N. Srivastava, Nanoscale Res. Lett., 2015, 10, 353 CrossRef.
- P. Elia, R. Zach, S. Hazan, S. Kolusheva, Z. e. Porat and Y. Zeiri, Int. J. Nanomed., 2014, 9, 4007–4021 Search PubMed.
- A. Pani, T. D. Thanh, N. H. Kim, J. H. Lee and S.-I. Yun, IET Nanobiotechnol., 2016, 10, 431–437 CrossRef.
- J. Li, Q. Li, X. Ma, B. Tian, T. Li, J. Yu, S. Dai, Y. Weng and Y. Hua, Int. J. Nanomed., 2016, 11, 5931–5944 CrossRef CAS.
- C. Wang, R. Mathiyalagan, Y. J. Kim, V. Castro-Aceituno, P. Singh, S. Ahn, D. Wang and D. C. Yang, Int. J. Nanomed., 2016, 11, 3691 CrossRef CAS.
- V. Castro-Aceituno, R. Abbai, S. S. Moon, S. Ahn, R. Mathiyalagan, Y.-J. Kim, Y.-J. Kim and D. C. Yang, Biomed. Pharmacother., 2017, 93, 995–1003 CrossRef CAS.
- S. Y. Lee, S. Krishnamurthy, C.-W. Cho and Y.-S. Yun, ACS Sustainable Chem. Eng., 2016, 4, 2651–2659 CrossRef CAS.
- R. Bhambure, M. Bule, N. Shaligram, M. Kamat and R. Singhal, Chem. Eng. Technol., 2009, 32, 1036–1041 CrossRef CAS.
- E. Priyadarshini, N. Pradhan, L. B. Sukla and P. K. Panda, J. Nanotechnol., 2014, 2014, 9 Search PubMed.
- A. K. Jha and K. Prasad, Int. J. Green Nanotechnol., 2011, 3, 92–97 CrossRef CAS.
- M. E. Castro, L. Cottet and A. Castillo, Mater. Lett., 2014, 115, 42–44 CrossRef CAS.
- M. M. H. Khalil, E. H. Ismail and F. El-Magdoub, Arabian J. Chem., 2012, 5, 431–437 CrossRef CAS.
- A. Dzimitrowicz, P. Jamróz, G. C. diCenzo, I. Sergiel, T. Kozlecki and P. Pohl, Arabian J. Chem., 2019, 12, 4118–4130 CrossRef CAS.
- M. Ramakrishna, D. Rajesh Babu, R. M. Gengan, S. Chandra and G. Nageswara Rao, J. Nanostruct. Chem., 2016, 6, 1–13 CrossRef CAS.
- L. M. Fernando, F. E. Merca and E. S. Paterno, Philipp. Agric. Scientist., 2013, 96, 129–136 Search PubMed.
- C. Gonnelli, C. Giordano, U. Fontani, M. C. Salvatici and S. Ristori, in Advances in Bionanomaterials: Selected Papers from the 2nd Workshop in Bionanomaterials, BIONAM 2016, October 4-7, 2016, Salerno, Italy, ed. S. Piotto, F. Rossi, S. Concilio, E. Reverchon and G. Cattaneo, Springer International Publishing, Cham, 2018, pp. 155–164, DOI:10.1007/978-3-319-62027-5_14.
- N. Bogireddy, U. Pal, L. M. Gomez and V. Agarwal, RSC Adv., 2018, 8, 24819–24826 RSC.
|
This journal is © The Royal Society of Chemistry 2021 |