DOI:
10.1039/D0QO00837K
(Research Article)
Org. Chem. Front., 2021,
8, 18-24
Copper-catalyzed alkynylation/annulation cascades of N-allyl ynamides: regioselective access to medium-sized N-heterocycles†
Received
23rd July 2020
, Accepted 29th September 2020
First published on 30th September 2020
Abstract
A synthetic strategy based on sequential application of aza-Claisen rearrangement, C–H functionalization, C–N coupling and cyclization as key steps has been developed for the synthesis of various medium-sized N-heterocycles of pharmaceutical relevance. This efficient new method exhibits a broad scope and provides a highly efficient synthesis of N-heterocycles of different ring sizes (6-, 7-, 8-, and 9-membered rings) in moderate to good yields.
N-Heterocycles continue to receive widespread attention due to their bioactivities and pharmaceutical applications. Among N-heterocycles, the 7- and 8-membered N-heterocycles are probably one of the most common structural motifs spread across natural products and drugs (Fig. 1).1 Therefore, the development of facile and efficient methods for the selective and diverse construction of various medium-sized (7- and 8-membered rings) N-heterocycles is still required.
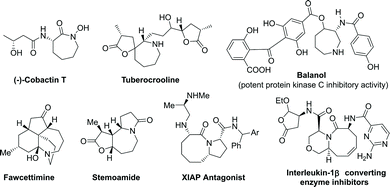 |
| Fig. 1 Examples of important medium-sized N-heterocycles. | |
However, the synthesis of medium-sized (7- and 8-membered rings) N-heterocycles has proved to be highly challenging, mainly due to the unfavorable enthalpic (the strain in many medium-sized rings) and entropic (probability of the chain ends meeting) reasons.2 Therefore, traditional ring closure approaches for 5-and 6-membered rings are problematic for medium-sized rings.3 Furthermore, methodologies to form medium-sized N-heterocycles require the consideration of a raft of compatibility and selectivity issues.
Recently, the ynamide chemistry4 has become a powerful entry to build N-heterocycles.5 In 2019, we have reported CuII-catalyzed annulation cascades of N-allyl-N-((2-bromoaryl)ethynyl)amides with terminal alkynes or 1,3-dicarbonyls for accessing 2,3-difunctionalized indoles. Such methods provide significant benefits toward the synthesis of a series of indole derivatives (Scheme 1a).6 While this reaction has been very successful in 5-membered ring synthesis, its potential for medium-sized ring formation has not been explored.7
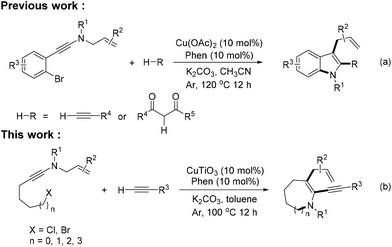 |
| Scheme 1 Synthesis of N-heterocycles. | |
Herein we report on the effective participation of ynamides with terminal alkynes in related processes that have provided a means for the rapid construction of a diverse range of medium-sized N-heterocyclic systems (Scheme 1b). Through the design of the substrate, the relative position of the coupling group and the ynamide group in the molecule is controlled, and N-heterocycles of different ring sizes (6-, 7-, 8- and 9-membered rings) are synthesized selectively.
To initiate our study, the reaction conditions were screened for the formation of 6-allyl-7-(phenylethynyl)-1-tosyl-2,3,4,5-tetrahydro-1H-azepine (3aa) with N-allyl-N-(6-chlorohex-1-yn-1-yl)-4-methylbenzenesulfonamide (1a) and phenylacetylene (2a) as model substrates (Table 1). The results demonstrated that a combination of CuTiO3 with the Phen ligand plays an important role in the reaction (Table 1, entries 1–3). In a typical procedure, a mixture of 1a (0.2 mmol, 1 equiv.), 2a (3 equiv.), CuTiO3 (10 mol%), 1,10-phenanthroline (Phen, 10 mol%) and K2CO3 (2 equiv.) in 2 mL of toluene was stirred under argon at 100 °C. Gratifyingly, the reaction proceeded smoothly to give the azepine 3aa in 62% isolated yield (Table 1, entry 1). The reaction failed to afford 3aa without a copper catalyst or Phen ligand (Table 1, entries 2 and 3). Notably, the amounts of CuTiO3 and Phen affected the reaction, and a combination of 10 mol% CuTiO3 with 10 mol% Phen was preferred (Table 1, entries 1, 4 and 5). Other copper catalysts, such as Cu(OAc)2, CuCl2, and Cu(OTf)2, resulted in 3aa in 31%, 27% and 34% yields, respectively (Table 1, entries 6–8). In order to improve the yield of 3aa, other ligands such as 2,2′-bipyridine and N,N,N′,N′-tetramethylethane-1,2-diamine (TMEDA) were added to the reaction system. Unfortunately, no azepine products were obtained (Table 1, entries 9 and 10). We found that the yield of 3aa decreased from 62% to 43% when using Cs2CO3 instead of K2CO3 (Table 1, entry 11). However, the reaction failed to proceed without bases (Table 1, entry 12). Subsequently, we screened several solvents and found that acetonitrile (MeCN), tetrahydrofuran (THF), and N,N-dimethylformamide (DMF) did not give better results (Table 1, entries 13–15). The reaction in DMF even failed to afford 3aa. Finally, the screening of the reaction temperature effect revealed that either a lower (80 °C) or a higher (120 °C) temperature had a deleterious effect on the reaction (Table 1, entries 16 and 17). Therefore, the combinations listed in entry 1 were the optimal reaction conditions for this tandem cyclization.
Table 1 Optimization of reaction conditionsa

|
Entry |
Variation from the standard conditions |
Isolated yield [%] |
Reaction conditions: 1a (0.2 mmol), 2a (0.6 mmol), CuTiO3 (10 mol%), Phen (10 mol%), K2CO3 (2 equiv.), toluene (2 mL), argon, 100 °C and 12 h.
|
1 |
None |
62 |
2 |
Without Phen |
0 |
3 |
Without CuTiO3 |
0 |
4 |
CuTiO3 (5 mol%) and Phen (5 mol%) |
45 |
5 |
CuTiO3 (20 mol%) and Phen (20 mol%) |
66 |
6 |
Cu(OAc)2 instead of CuTiO3 |
31 |
7 |
CuCl2 instead of CuTiO3 |
27 |
8 |
Cu(OTf)2 instead of CuTiO3 |
34 |
9 |
2,2′-Bipyridine instead of Phen |
0 |
10 |
TMEDA instead of Phen |
0 |
11 |
Cs2CO3 instead of K2CO3 |
43 |
12 |
Without K2CO3 |
0 |
13 |
MeCN instead of toluene |
46 |
14 |
THF instead of toluene |
39 |
15 |
DMF instead of toluene |
0 |
16 |
At 80 °C |
26 |
17 |
At 120 °C |
57 |
With the optimized reaction conditions in hand, the applicability to different ynamides was investigated (Scheme 2). A variety of ynamides transformed smoothly into medium-sized N-heterocycles in this copper/Phen catalytic system and displayed good tolerance. The substrates leading to 6-, 8- and 9-membered N-heterocycles were first examined and gave the desired cyclization products in 72%, 47% and 32% yields, respectively (3ba–3da).8 Unfortunately, the current reaction was not viable for 12-membered N-heterocycles (3ea). Notably, substrates 1f and 1g, containing an N-(2-methylallyl) group or an N-(but-2-en-1-yl) group, underwent the tandem cyclization, giving the corresponding aza-Claisen rearrangement products 3fa and 3ga in moderate yields. We found this transformation to be efficient for a wide range of substrates, which allowed for the synthesis of sulfonylated azepines in moderate yields. It was found that a variety of groups, including alkyl sulfonyl (3ha and 3ia), aryl sulfonyl (3ka–3na), and heterocyclic sulfonyl (3oa), were perfectly tolerated under the tandem cyclization conditions. Tandem cyclization of para-substituted aryl sulfonyl possessing electron-withdrawing (3la and 3ma) substituents proceeded with less efficiency. Unfortunately, the use of an ynamide with a large steric hindrance such as N-allyl-N-(6-chlorohex-1-yn-1-yl)-2,4,6-trimethylbenzenesulfonamide (1j) did not give the desired azepine (3ja).
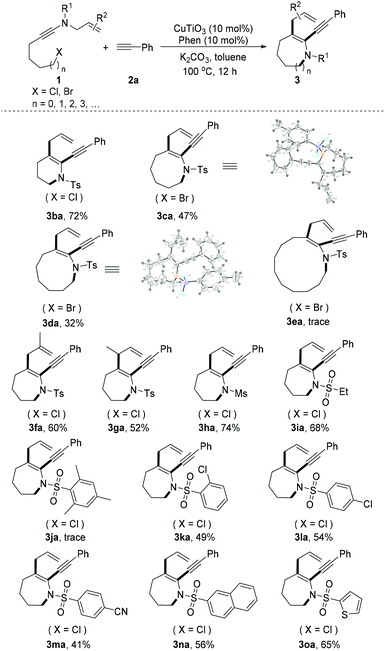 |
| Scheme 2 Scope of ynamides. Reaction conditions: 1 (0.2 mmol), 2 (0.6 mmol), CuTiO3 (10 mol%), Phen (10 mol%), K2CO3 (2 equiv.), toluene (2 mL), argon, 100 °C and 12 h. | |
This copper/Phen catalytic system was further expanded to a range of substituted alkynes (Scheme 3). The results demonstrated that tandem cyclization was compatible with a wide array of arylalkynes and several aryl groups. For instance, 4-MeC6H4, 4-MeOC6H4, 4-ClC6H4, 4-FC6H4, 4-CNC6H4, and 4-NO2C6H4 at the terminal alkyne were well tolerated (3ab–3ag). Moreover, the electron effect had an influence on their reactivity. Arylalkynes bearing an electron-donating group were converted to azepines in good yields (3ab and 3ac), whereas arylalkynes with an electron-withdrawing group delivered azepines in lower yields (3ad–3ag). The use of para- or ortho-substituted arylalkynes afforded azepines 3ac and 3ah, respectively, in high yields, but bulky ortho-substituted arylalkyne generated azepine 3ai with a diminished yield. Notably, the optimal conditions were applicable to polysubstituted arylalkynes, thus producing 3aj in 67% yield. For alkyne derivatives of various cycles, such as naphthalene, pyridine, cyclohexene, and cyclopropane, the corresponding azepines (3ak–3ao) were efficiently constructed in 44–70% yields. Gratifyingly, the optimal conditions were applicable to an aliphatic alkyne, thus producing 3ap in 53% yield. Finally, trimethylsilicone acetylene also displayed moderate reactivity, furnishing the azepine (3aq) with 45% yield.
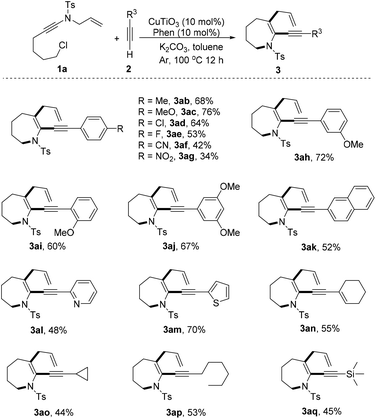 |
| Scheme 3 Scope of terminal alkynes. Reaction conditions: 1 (0.2 mmol), 2 (0.6 mmol), CuTiO3 (10 mol%), Phen (10 mol%), K2CO3 (2 equiv.), toluene (2 mL), argon, 100 °C and 12 h. | |
It was worth pointing out that CuTiO3 is a type of copper ion insertion-type material, in whose open channels, copper ions can reversibly insert in the common vacant sites of the orthorhombic Ti–O framework.9 In the reaction, the copper ion from CuTiO3 can transfer easily from the T–O framework to the solution and combine with the reagents to catalyze the reaction, while the copper ions in other homogeneous copper sources such as Cu(OAc)2 are fixed by the counterpart ions and constricted in the lattice node. Therefore, the reaction almost occurs in solution, and the heterogeneous catalyst CuTiO3 is more efficient than that the other homogeneous copper sources used.
To understand the current tandem cyclization and the essential role of the copper catalyst and the ligand in the reaction, DFT calculations were performed assuming the reaction occurred in solution, and the energy profiles are shown in Scheme 4. According to the experimental results and previous literature reports,4j,10 aza-Claisen rearrangement of the substrate 1a readily takes place under heating to produce the ketenimine intermediate A, which sequentially undergoes coordination with CuII(Phen)CCPh to form intermediate B. The resulting complex B undergoes a fairly nucleophilic addition of the alkynyl group to the C
N bond to form compound C with N–Cu bonding. The migration of CuII(Phen) from anionic nitrogen to the chlorine atom results in the formation of intermediate D, and the resulting structure D undergoes an intramolecular SN2 process assisted by the Lewis acidic CuII group and base to give the final product 3aa and regenerate the active CuII(Phen) species.
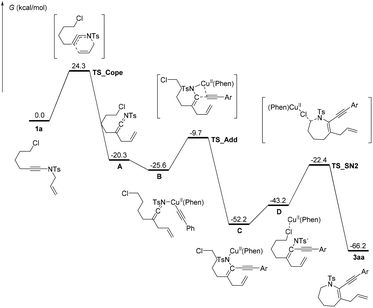 |
| Scheme 4 Energy profiles for the pathways of Cu(II)-catalyzed 1a leading to 3aa. | |
Conclusions
We have successfully developed a novel, efficient and facile method for the synthesis of 6-, 7-, 8-, 9-membered N-heterocycles, a longstanding challenging topic in organic synthesis. The key to success is the ketenimine intermediate generation and its chemoselective cross-coupling to the halogenated group. This protocol features a broad substrate scope and excellent functional group tolerance under copper-catalyzed reaction conditions. Efforts to expand the applications of this cascade strategy in cycle synthesis are currently underway in our laboratory. Further synthetic applications of this efficient transformation in the synthesis of natural products and pharmaceutical agents are currently in progress.
Conflicts of interest
There are no conflicts to declare.
Acknowledgements
We thank the National Natural Science Foundation of China (No. 21901255 and No. 21601204), Hunan Provincial Natural Science Foundation of China (No. 2018JJ3594) and Foundation of National University of Defense Technology (No. ZK17-03-52) for financial support. We also thank Dr Ye Zhang (National University of Defense Technology) for CuTiO3 and very useful discussions.
Notes and references
-
(a) D. L. Klayman, J. P. Scovill, J. F. Bartosevich and C. J. Mason, 2-Acetylpyridine thiosemicarbazones. 2. N4,N4-Disubstituted derivatives as potential antimalarial agents, J. Med. Chem., 1979, 22, 1367–1373 CrossRef CAS;
(b) Y. Ishihara, K. Hirai, M. Miyamoto and G. Goto, Central Cholinergic Agents. 6. Synthesis and Evaluation of 3-[1-(Phenylmethyl)-4-piperidinyl]-1-(2,3,4,5-tetrahydro-1H-1-benzazepin-8-yl)- 1-propanones and Their Analogs as Central Selective Acetylcholinesterase Inhibitors, J. Med. Chem., 1994, 37, 2292–2299 CrossRef CAS;
(c) H. Xu, J. He, J. Shi, L. Tan, D. Qiu, X. Luo and Y. Li, Domino Aryne Annulation via a Nucleophilic-Ene Process, J. Am. Chem. Soc., 2018, 140, 3555–3559 CrossRef CAS;
(d) G. L. Grunewald, V. H. Dahanukar, P. Ching and K. R. Criscione, Effect of Ring Size or an Additional Heteroatom on the Potency and Selectivity of Bicyclic Benzylamine-Type Inhibitors of Phenylethanolamine N-Methyltransferase, J. Med. Chem., 1996, 39, 3539–3546 CrossRef CAS;
(e) B. Basil, E. C. J. Coffee, D. L. Gell, D. R. Maxwell, D. J. Sheffield and K. R. H. Wooldridge, New class of sympathetic .beta.-receptor blocking agents. 3,4-Dihydro-3-hydroxy-1,5-benzoxazocines, J. Med. Chem., 1970, 13, 403–406 CrossRef CAS;
(f) D. Ma, G. Tang and A. P. Kozikowski, Synthesis of 7-Substituted Benzolactam-V8s and Their Selectivity for Protein Kinase C Isozymes, Org. Lett., 2002, 4, 2377–2380 CrossRef CAS;
(g) S. Li, Z. Li and J. Wu, Synthesis of Benzoindolines via a Copper-Catalyzed Reaction of 1-Bromoethynyl-2-(cyclopropylidenemethyl)arenes with N-Allylsulfonamide, Adv. Synth. Catal., 2012, 354, 3087–3094 CrossRef CAS;
(h) E. Vedejs, R. J. Galante and P. G. Goekjian, A Thio-Diels-Alder Route to the Azocine Ring System. Total Synthesis of (±)-Otonecine, J. Am. Chem. Soc., 1998, 120, 3613–3622 CrossRef CAS.
-
(a) C. Hu, R.-J. Song, M. Hu, Y. Yang, J.-H. Li and S. Luo, [5 + 2] Cycloaddition of 2-(2-Aminoethyl)oxiranes with Alkynes via Epoxide Ring-Opening: A Facile Access to Azepines, Angew. Chem., Int. Ed., 2016, 55, 10423–10426 CrossRef CAS;
(b) G. R. Cook, P. S. Shanker and S. L. Peterson, Asymmetric Synthesis of the Balanol Heterocycle via a Palladium-Mediated Epimerization and Olefin Metathesis, Org. Lett., 1999, 1, 615–618 CrossRef CAS;
(c) M. S. Visser, N. M. Heron, M. T. Didiuk, J. F. Sagal and A. H. Hoveyda, Catalytic and Enantioselective Route to Medium-Ring Heterocycles. Asymmetric Zirconium-Catalyzed Ethylmagnesation of Seven- and Eight-Membered Rings, J. Am. Chem. Soc., 1996, 118, 4291–4298 CrossRef CAS;
(d) S. J. Miller, S.-H. Kim, Z.-R. Chen and R. H. Grubbs, Catalytic Ring-Closing Metathesis of Dienes: Application to the Synthesis of Eight-Membered Rings, J. Am. Chem. Soc., 1995, 117, 2108–2109 CrossRef CAS;
(e) J.-J. Feng, T.-Y. Lin, C.-Z. Zhu, H. Wang, H.-H. Wu and J. Zhang, The Divergent Synthesis of Nitrogen Heterocycles by Rhodium(I)-Catalyzed Intermolecular Cycloadditions of Vinyl Aziridines and Alkynes, J. Am. Chem. Soc., 2016, 138, 2178–2181 CrossRef CAS;
(f) H. Ohno, H. Hamaguchi, M. Ohata, S. Kosaka and T. Tanaka, Palladium(0)-Catalyzed Synthesis of Medium-Sized Heterocycles by Using Bromoallenes as an Allyl Dication Equivalent, J. Am. Chem. Soc., 2004, 126, 8744–8754 CrossRef CAS;
(g) M. E. Maier, Synthesis of Medium-Sized Rings by the Ring-Closing Metathesis Reaction, Angew. Chem., Int. Ed., 2000, 39, 2073–2077 CrossRef CAS;
(h) J.-J. Feng, T.-Y. Lin, H.-H. Wu and J. Zhang, Transfer of Chirality in the Rhodium-Catalyzed Intramolecular Formal Hetero-[5 + 2] Cycloaddition of Vinyl Aziridines and Alkynes: Stereoselective Synthesis of Fused Azepine Derivatives, J. Am. Chem. Soc., 2015, 137, 3787–3790 CrossRef CAS.
-
(a) T. L. Wootton, J. A. Porter, K. S. Grewal, P. G. Chirila, S. Forbes, S. J. Coles, P. N. Horton, A. Hamilton and C. J. Whiteoak, Merging Cu-catalysed C-H functionalisation and intramolecular annulations: computational and experimental studies on an expedient construction of complex fused heterocycles, Org. Chem. Front., 2020, 7, 1235–1242 RSC;
(b) J. S. S. Neto and G. Zeni, Recent advances in the synthesis of indoles from alkynes and nitrogen sources, Org. Chem. Front., 2020, 7, 155–210 RSC;
(c) T. Mita, M. Uchiyama and Y. Sato, Catalytic Intramolecular Coupling of Ketoalkenes by Allylic C(sp3)–H Bond Cleavage: Synthesis of Five- and Six-Membered Carbocyclic Compounds, Adv. Synth. Catal., 2020, 362, 1275–1280 CrossRef CAS;
(d) P. H. Gilmartin and M. C. Kozlowski, Vanadium-Catalyzed Oxidative Intramolecular Coupling of Tethered Phenols: Formation of Phenol-Dienone Products, Org. Lett., 2020, 22, 2914–2919 CrossRef CAS;
(e) X. Bao, W. Jiang, J. Liang and C. Huo, One-electron oxidative dehydrogenative annulation and cyclization reactions, Org. Chem. Front., 2020, 7, 2107–2144 RSC;
(f) M. Zhang, Q. Wang, Y. Peng, Z. Chen, C. Wan, J. Chen, Y. Zhao, R. Zhang and A. Q. Zhang, Transition metal-catalyzed sp3 C-H activation and intramolecular C-N coupling to construct nitrogen heterocyclic scaffolds, Chem. Commun., 2019, 55, 13048–13065 RSC;
(g) W. Guo, M. Zhao, W. Tan, L. Zheng, K. Tao and X. Fan, Developments towards synthesis of N-heterocycles from amidines via C-N/C-C bond formation, Org. Chem. Front., 2019, 6, 2120–2141 RSC;
(h) P.-Q. Huang, Y.-H. Huang and S.-R. Wang, One-pot synthesis of N-heterocycles and enimino carbocycles by tandem dehydrative coupling-reductive cyclization of halo-sec-amides and dehydrative cyclization of olefinic sec-amides, Org. Chem. Front., 2017, 4, 431–444 RSC;
(i) J. Yuan, C. Liu and A. Lei, Construction of N-containing heterocycles via oxidative intramolecular N-H/X-H coupling, Chem. Commun., 2015, 51, 1394–1409 RSC;
(j) Y. Quan and Z. Xie, Palladium-Catalyzed Regioselective Intramolecular Coupling of o-Carborane with Aromatics via Direct Cage B-H Activation, J. Am. Chem. Soc., 2015, 137, 3502–3505 CrossRef CAS;
(k) J.-B. Zhu, P. Wang, S. Liao and Y. Tang, PPh3-mediated intramolecular conjugation of alkyl halides with electron-deficient olefins: facile synthesis of chromans and relevant analogues, Chem. Commun., 2013, 49, 4570–4572 RSC;
(l) Y. Li, J. Zhu, H. Xie, S. Li, D. Peng, Z. Li, Y. Wu and Y. Gong, Rh-catalyzed intramolecular sp2 C-H bond difluoromethylenation, Chem. Commun., 2012, 48, 3136–3138 RSC;
(m) Y. Fang and C. Li,
O-Arylation versus C-Arylation: Copper-Catalyzed Intramolecular Coupling of Aryl Bromides with 1,3-Dicarbonyls, J. Org. Chem., 2006, 71, 6427–6431 CrossRef CAS;
(n) D. Solé, X. Urbaneja and J. Bonjoch, Palladium-Catalyzed Intramolecular Coupling of Amino-Tethered Vinyl Halides with Ketones, Esters, and Nitriles Using Potassium Phenoxide as the Base, Adv. Synth. Catal., 2004, 346, 1646–1650 CrossRef;
(o) D. Solé, E. Peidró and J. Bonjoch, Palladium-Catalyzed Intramolecular Coupling of Vinyl Halides and Ketone Enolates. Synthesis of Bridged Azabicyclic Compounds, Org. Lett., 2000, 2, 2225–2228 CrossRef;
(p) D. D. Hennings, S. Iwasa and V. H. Rawal, Anion-Accelerated Palladium-Catalyzed Intramolecular Coupling of Phenols with Aryl Halides, J. Org. Chem., 1997, 62, 2–3 CrossRef CAS.
-
(a) K. A. DeKorver, X.-N. Wang, M. C. Walton and R. P. Hsung, Carbocyclization Cascades of Allyl Ketenimines via Aza-Claisen Rearrangements of N-Phosphoryl-N-allyl-ynamides, Org. Lett., 2012, 14, 1768–1771 CrossRef CAS;
(b) F. Pan, X.-L. Li, X.-M. Chen, C. Shu, P.-P. Ruan, C.-H. Shen, X. Lu and L.-W. Ye, Catalytic Ynamide Oxidation Strategy for the Preparation of α-Functionalized Amides, ACS Catal., 2016, 6, 6055–6062 CrossRef CAS;
(c) G. Duret, V. L. Fouler, P. Bisseret, V. Bizet and N. Blanchard, Diels-Alder and Formal Diels-Alder Cycloaddition Reactions of Ynamines and Ynamides, Eur. J. Org. Chem., 2017, 2017, 6816–6830 CrossRef CAS;
(d) K. A. DeKorver, R. P. Hsung, A. G. Lohse and Y. Zhang, A Divergent Mechanistic Course of Pd(0)-Catalyzed Aza-Claisen Rearrangement and Aza-Rautenstrauch-Type Cyclization of N-Allyl Ynamides, Org. Lett., 2010, 12, 1840–1843 CrossRef CAS;
(e) K. A. DeKorver, T. D. North and R. P. Hsung, An Efficient Synthesis of de novo Imidates via Aza-Claisen Rearrangements of N-Allyl Ynamides, Synlett, 2010, 2397–2402 CAS;
(f) J. R. Alexander and M. J. Cook, Formation of Ketenimines via the Palladium-Catalyzed Decarboxylative π-Allylic Rearrangement of N-Alloc Ynamides, Org. Lett., 2017, 19, 5822–5825 CrossRef CAS;
(g) W. Wu and H. Jiang, Haloalkynes: A Powerful and Versatile Building Block in Organic Synthesis, Acc. Chem. Res., 2014, 47, 2483–2504 CrossRef CAS;
(h) K. A. DeKorver, R. P. Hsung, W.-Z. Song, X.-N. Wang and M. C. Walton, An Intramolecular [2 + 2] Cycloaddition of Ketenimines via Palladium-Catalyzed Rearrangements of N-Allyl-Ynamides, Org. Lett., 2012, 14, 3214–3217 CrossRef CAS;
(i) K. A. DeKorver, M. C. Walton, T. D. North and R. P. Hsung, Introducing a New Class of N-Phosphoryl Ynamides via Cu(I)-Catalyzed Amidations of Alkynyl Bromides, Org. Lett., 2011, 13, 4862–4865 CrossRef CAS;
(j) R. H. Dodd and K. Cariou, Ketenimines Generated from Ynamides: Versatile Building Blocks for Nitrogen-Containing Scaffolds, Chem. – Eur. J., 2018, 24, 2297–2304 CrossRef CAS;
(k) K. A. DeKorver, W. L. Johnson, Y. Zhang, R. P. Hsung, H. Dai, J. Deng, A. G. Lohse and Y.-S. Zhang,
N-Allyl-N-sulfonyl Ynamides as Synthetic Precursors to Amidines and Vinylogous Amidines. An Unexpected N-to-C 1,3-Sulfonyl Shift in Nitrile Synthesis, J. Org. Chem., 2011, 76, 5092–5103 CrossRef CAS;
(l) V. Dwivedi, M. Hari Babu, R. Kant and M. Sridhar Reddy, N-Substitution dependent stereoselectivity switch in palladium catalyzed hydroalkynylation of ynamides: a regio and stereoselective synthesis of ynenamides, Chem. Commun., 2015, 51, 14996–14999 RSC;
(m) K.-B. Wang, R.-Q. Ran, S.-D. Xiu and C.-Y. Li, Synthesis of 3-Aza-bicyclo[3.1.0]hexan-2-one Derivatives via Gold-Catalyzed Oxidative Cyclopropanation of N-Allylynamides, Org. Lett., 2013, 15, 2374–2377 CrossRef CAS;
(n) Y. Zhang, K. A. DeKorver, A. G. Lohse, Y.-S. Zhang, J. Huang and R. P. Hsung, Synthesis of Amidines Using N-Allyl Ynamides. A Palladium-Catalyzed Allyl Transfer through an Ynamido-π-Allyl Complex, Org. Lett., 2009, 11, 899–902 CrossRef CAS;
(o) X.-N. Wang, G. N. Winston-McPherson, M. C. Walton, Y. Zhang, R. P. Hsung and K. A. DeKorver, Synthesis of Cyclopentenimines from N-Allyl Ynamides via a Tandem Aza-Claisen Rearrangement–Carbocyclization Sequence, J. Org. Chem., 2013, 78, 6233–6244 CrossRef CAS;
(p) A. M. Cook and C. Wolf, Terminal ynamides: synthesis, coupling reactions, and additions to common electrophiles, Tetrahedron Lett., 2015, 56, 2377–2392 CrossRef CAS;
(q) K. A. DeKorver, H. Li, A. G. Lohse, R. Hayashi, Z. Lu, Y. Zhang and R. P. Hsung, Ynamides: A Modern Functional Group for the New Millennium, Chem. Rev., 2010, 110, 5064–5106 CrossRef CAS;
(r) G. Evano, A. Coste and K. Jouvin, Ynamides: Versatile Tools in Organic Synthesis, Angew. Chem., Int. Ed., 2010, 49, 2840–2859 CrossRef CAS.
-
(a) R. Li, X. Chen, X.-R. Song, H. Ding, P. Wang, Q. Xiao and Y.-M. Liang, Copper-Catalyzed Cascade Cyclization of 2-Propynolphenols: Access to 4-Phosphorylated 2H-Chromenes, Adv. Synth. Catal., 2017, 359, 3962–3967 CrossRef CAS;
(b) M. Witt, H. A. Oketch-Rabah and J. W. Jaroszewski, A New Class of Spermidine-Derived Alkaloids, Org. Lett., 2003, 5, 2793–2796 CrossRef;
(c) J. Li, M. Hu, C. Li, C. Li, J. Li, W. Wu and H. Jiang, Palladium-Catalyzed Cascade Cyclization/Alkynylation and Alkenylation of Alkynone O-Methyloximes with Terminal Alkynes, Adv. Synth. Catal., 2018, 360, 2707–2719 CrossRef CAS;
(d) C. F. Heinrich, I. Fabre and L. Miesch, Silver-Catalyzed 7-exo-dig Cyclization of Silylenolether-ynesulfonamides, Angew. Chem., 2016, 128, 5256–5260 CrossRef;
(e) M. Mori, T. Kitamura, N. Sakakibara and Y. Sato, Synthesis of Eight-Membered Ring Compounds Using Enyne Metathesis, Org. Lett., 2000, 2, 543–545 CrossRef CAS;
(f) G. C. Fu and R. H. Grubbs, The synthesis of nitrogen heterocycles via catalytic ring-closing metathesis of dienes, J. Am. Chem. Soc., 1992, 114, 7324–7325 CrossRef CAS;
(g) X.-N. Wang, H.-S. Yeom, L.-C. Fang, S. He, Z.-X. Ma, B. L. Kedrowski and R. P. Hsung, Ynamides in Ring Forming Transformations, Acc. Chem. Res., 2014, 47, 560–578 CrossRef CAS.
- R. Su, X.-H. Yang, M. Hu, Q.-A. Wang and J.-H. Li, Annulation Cascades of N-Allyl-N-((2-bromoaryl)ethynyl)amides Involving C-H Functionalization, Org. Lett., 2019, 21, 2786–2789 CrossRef CAS.
-
(a) H. Hamaguchi, S. Kosaka, H. Ohno and T. Tanaka, Bromoallenes as Allyl Dication Equivalents in the Absence of Palladium(0): Synthesis of Bicyclic Sulfamides by Tandem Cyclization of Bromoallenes, Angew. Chem., Int. Ed., 2005, 44, 1513–1517 CrossRef CAS;
(b) B. Niu, R. Liu, Y. Wei and M. Shi, Catalyst-controlled synthesis of 4-amino-isoquinolin-1(2H)-one and oxazole derivatives, Org. Chem. Front., 2018, 5, 1466–1470 RSC;
(c) M. Ito, R. Kawasaki, K. S. Kanyiva and T. Shibata, Catalytic Dearomative Spirocyclization via Gold Carbene Species Derived from Ynamides: Efficient Synthesis of 2-Azaspiro[4.5]decan-3-ones, Chem. – Eur. J., 2018, 24, 3721–3724 CrossRef CAS;
(d) C. Theunissen, B. Métayer, M. Lecomte, N. Henry, H.-C. Chan, G. Compain, P. Gérard, C. Bachmann, N. Mokhtari, J. Marrot, A. Martin-Mingot, S. Thibaudeau and G. Evano, Cationic polycyclization of ynamides: building up molecular complexity, Org. Biomol. Chem., 2017, 15, 4399–4416 RSC;
(e) H. V. Adcock, E. Chatzopoulou and P. W. Davies, Divergent C-H Insertion-Cyclization Cascades of N-Allyl Ynamides, Angew. Chem., Int. Ed., 2015, 54, 15525–15529 CrossRef CAS;
(f) R. Liu, G. N. Winston-McPherson, Z.-Y. Yang, X. Zhou, W. Song, I. A. Guzei, X. Xu and W. Tang, Generation of Rhodium(I) Carbenes from Ynamides and Their Reactions with Alkynes and Alkenes, J. Am. Chem. Soc., 2013, 135, 8201–8204 CrossRef CAS;
(g) W.-B. Shen, B. Zhou, Z.-X. Zhang, H. Yuan, W. Fang and L.-W. Ye, Gold-catalyzed cascade cyclization of N-propargyl ynamides: rapid access to functionalized indeno[1,2-c]pyrroles, Org. Chem. Front., 2018, 5, 2468–2472 RSC;
(h) C. Theunissen, B. Métayer, N. Henry, G. Compain, J. Marrot, A. Martin-Mingot, S. Thibaudeau and G. Evano, Keteniminium Ion-Initiated Cascade Cationic Polycyclization, J. Am. Chem. Soc., 2014, 136, 12528–12531 CrossRef CAS;
(i) Y. Wang, L.-J. Song, X. Zhang and J. Sun, Metal-Free [2 + 2 + 2] Cycloaddition of Ynamides and Nitriles: Mild and Regioselective Synthesis of Fully Substituted Pyridines, Angew. Chem., Int. Ed., 2016, 55, 9704–9708 CrossRef CAS;
(j) M. Anitha, M. Shankar and K. C. Kumara Swamy, Reactivity of epoxy-ynamides with metal halides: nucleophile (Br/Cl/OH)-assisted tandem intramolecular 5-exo-dig or 6-endo-dig cyclisation and AgF2-promoted oxidation, Org. Chem. Front., 2019, 6, 1133–1139 RSC;
(k) G. He, Y. Li, Z. Yu, Z. Chen, Y. Tang, G. Song and T.-P. Loh, Selectfluor™-catalyzed oxidative cyclization of ynamides enables facile synthesis of oxazolidine-2,4-diones, Org. Chem. Front., 2019, 6, 3644–3648 RSC;
(l) P. Han, Z.-Y. Mao, C.-M. Si, Z. Zhou, B.-G. Wei and G.-Q. Lin, Stereoselective Synthesis of Pyrido- and Pyrrolo[1,2-c,][1,3]oxazin-1-ones via a Nucleophilic Addition-Cyclization Process of N,O-Acetal with Ynamides, J. Org. Chem., 2019, 84, 914–923 CrossRef CAS;
(m) Y. Pan, G.-W. Chen, C.-H. Shen, W. He and L.-W. Ye, Synthesis of fused isoquinolines via gold-catalyzed tandem alkyne amination/intramolecular O-H insertion, Org. Chem. Front., 2016, 3, 491–495 RSC;
(n) T. Okitsu, A. Namura, S. Kondo, S. Tada, M. Yanagida and A. Wada, Ynamides enabled 6-, 7-, and 8-endo-dig iodocyclization of ethoxyethyl ethers: rapid construction of medium-sized oxacycles at room temperature, Org. Chem. Front., 2020, 7, 879–884 RSC.
- CCDC 1910929 (3aa), CCDC 1910930 (3ca) and CCDC 1910931 (3da) contain the supplementary crystallographic data for this paper.†.
-
(a) S. Ghosh, Y. Kee, S. Okada and P. Barpanda, Energy-savvy solid-state and sonochemical synthesis of lithium sodium titanate as an anode active material for Li-ion batteries, J. Power Sources., 2015, 296, 276–281 CrossRef CAS;
(b) D. Dambournet, I. Belharouak and K. Amine, MLi2Ti6O14 (M = Sr, Ba, 2(Na) Lithium Insertion Titanate Materials: A Comparative Study, Inorg. Chem., 2010, 49, 2822–2826 CrossRef CAS;
(c) S. Y. Yin, L. Song, X. Y. Wang, Y. H. Huang, K. L. Zhang and Y. X. Zhang, Reversible lithium storage in Na2Li2Ti6O14 as anode for lithium ion batteries, Electrochem. Commun., 2009, 11, 1251–1254 CrossRef CAS;
(d) A. P. Ramirez, M. A. Subramanian, M. Gardel, G. Blumberg, D. Li, T. Vogt and S. M. Shapiro, Giant dielectric constant response in a copper-titanate, Solid State Commun., 2000, 115, 217–220 CrossRef CAS.
-
(a) L. Xu, P. Yang and L. Wang, Direct functionalization of benzylic and non-benzylic C(sp3)-H bonds via keteniminium ion initiated cascade [1,5]-hydrogen transfer/cyclization, Org. Chem. Front., 2018, 5, 1854–1858 RSC;
(b) N. S. V. M. R. Mangina, R. Guduru and G. V. Karunakar, Synthesis of medium-sized aryl-fused nitrogenous heterocycles via sequential aryne aza-Claisen rearrangement/ring-closing metathesis, Org. Biomol. Chem., 2018, 16, 2134–2142 RSC;
(c) D. Zhang, I. Nakamura and M. Terada, Copper-Catalyzed Cascade Transformation of O-Propargylic Oximes with Sulfonyl Azides to α,β-Unsaturated N-Acylamidines, Org. Lett., 2014, 16, 5184–5187 CrossRef CAS;
(d) Y. Kiran Kumar, G. Ranjith Kumar and M. Sridhar Reddy, Cu-Catalyzed Conversion of Propargyl Acetates to E-α,β-Unsaturated Amides via Ketenimine Formation with Sulfonyl Azides, J. Org. Chem., 2014, 79, 823–828 CrossRef CAS;
(e) P. Lu and Y. Wang, The thriving chemistry of ketenimines, Chem. Soc. Rev., 2012, 41, 5687–5705 RSC.
Footnote |
† Electronic supplementary information (ESI) available: General experimental information, experimental procedure for product synthesis, full characterization data, 1H and 13C NMR spectra of all products. CCDC 1910929, 1910930 and 1910931. For ESI and crystallographic data in CIF or other electronic format see DOI: 10.1039/d0qo00837k |
|
This journal is © the Partner Organisations 2021 |
Click here to see how this site uses Cookies. View our privacy policy here.