DOI:
10.1039/D0QI01495H
(Research Article)
Inorg. Chem. Front., 2021,
8, 1804-1809
A new twist on an old ligand: a [Mn16] double square wheel and a [Mn10] contorted wheel†
Received
21st December 2020
, Accepted 26th January 2021
First published on 3rd February 2021
Abstract
Ligand design remains key to the synthesis of coordination compounds possessing specific topologies, nuclearities and symmetries that direct targeted physical properties. N,O-chelates based on ethanolamine have been particularly prolific in constructing a variety of paramagnetic 3d transition metal complexes with fascinating magnetic properties. Here, we show that combining three ethanolamine moieties within the same organic framework in the form of the pro-ligand 1,3,5-tri(2-hydroxyethyl)-1,3,5-triazacyclohexane (LH3) leads to the formation of two highly unusual Mn wheels. Reaction of Mn(NO3)2·6H2O with LH3 in basic methanolic solutions leads to the formation of [MnIII12MnII4(μ3-O)6(μ-OH)4(μ3-OMe)2(μ-OMe)2(L)4(LH)2(H2O)10](NO3)6(OH)2 (1) and [MnIII10(μ3-O)4(μ-OH)4(μ-OMe)4(L)4(H2O)4](NO3)2 (2), the only difference in the synthesis being the ratio of metal:ligand employed. The structure of the former describes two offset [MnIII6MnII2] square wheels, linked through a common centre, and the latter a single [MnIII10] wheel twisted at its centre, such that the top half is orientated perpendicular to the bottom half. In both cases the L3−/LH2− ligands dictate the orientation of the Jahn-Teller axes of the MnIII ions which lie perpendicular to the triazacyclohexane plane. Direct current magnetic susceptibility and magnetisation data reveal the presence of competing exchange interactions in 1 and strong antiferromagnetic interactions in 2. Given the simplicity of the reactions employed and the paucity of previous work, the formation of these two compounds suggests that LH3 will prove to be a profitable ligand for the synthesis of a multitude of novel 3d transition metal complexes.
Introduction
The development of magneto-structural relationships in molecular coordination compounds can be traced back to measurements of copper(II) acetate and the basic metal(III) carboxylates, wherefrom their dinuclear and trinuclear structures, solved later, were predicted.1,2 Detailed, quantitative analyses of a variety of di-, tri- and tetranuclear 3d transition metal complexes followed, often revealing a complex relationship between exchange interactions/magnetic anisotropy, and the identity of the ligand, metal-metal distances, metal-ligand bond lengths, bond angles, torsions angles, and metal geometry – correlations now benefitting from detailed theoretical input.3–5 Interest in the magnetochemistry of Mn compounds in particular was boosted by the discovery of single-molecule magnets (SMMs), the first of which was a [Mn12] complex6 whose structure was reported some years earlier.7 Magneto-structural studies were aided and abetted by magnetic measurements on the large library of low-nuclearity Mn compounds initially established as metalloenzyme model complexes, particularly those pertaining to the water oxidation centre in PSII.8 These proved vital in both the development of novel synthetic methodologies for the construction of new Mn compounds whose nuclearities now reach eighty four9 and in understanding the origin of the slow magnetisation relaxation dynamics.10
Central to these studies has been the design of ligands capable of bridging between paramagnetic metal ions in a particular manner, be that within a rigid or flexible framework. One very successful class of ligands in the latter category are N,O-chelates including 2-(hydroxymethyl)pyridine (hmpH),11 2,6-pyridinemethanol (pdmH2),12 di- (R-deaH2)13 and triethanolamine (teaH3)14 which are all characterised by possessing one or more linked ethanolamine (eaH)15 moieties (Fig. 1). Herein we extend this body of work to include the pro-ligand 1,3,5-tri(2-hydroxyethyl)-1,3,5-triazacyclohexane (LH3), which contains three linked eaH units. A search of the Cambridge Structural Database (CSD) reveals just two hits in 3d transition metal chemistry. The first,16 in 1999, was the monomer [Cr(CO)3(LH3)] and the second, in 2019, an aesthetically pleasing torus-like [Mn16] complex, [MnII2MnIII14(trz)14L4(μ3-O)8(H2O)10](ClO4)6 (Htzr = 1,2,3-triazole), in which the ligand was generated serendipitously in situ, upon the reaction of (2-hydroxymethyl)-1,2,3-triazole and 2-aminoethanol in the presence of manganese perchlorate.17
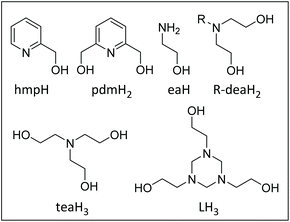 |
| Fig. 1 The N,O-chelates 2-(hydroxymethyl)pyridine (hmpH), 2,6-pyridinedimethanol (pdmH2), di- (R-deaH2) and triethanolamine (teaH3), and 1,3,5-tri(2-hydroxyethyl)-1,3,5-triazacyclohexane (LH3), all of which contain one or more linked ethanolamine (eaH) moieties. | |
Experimental
General methods
All chemicals were obtained from commercial suppliers (Sigma-Aldrich) and were used without further purification/treatment.
Synthesis
LH3 was prepared as previously described.16
[MnIII12MnII4(μ3-O)6(μ-OH)4(μ3-OMe)2(μ-OMe)2(L)4(LH)2(H2O)10](NO3)6(OH)2 (1).
Mn(NO3)2·6H2O (143.5 mg, 0.5 mmol) and LH3 (103.58 mg, 0.5 mmol) were stirred in MeOH for 30 minutes in the presence of NEt3 (1 mmol). The solution was then filtered and allowed to stand. Crystals of 1 formed in ∼5 days in a yield of 20%. Elemental analysis (%) calcd for C62H178N24O76 (1): C 22.82, H 4.89, N 11.01; found: C 22.93, H 4.71, N 11.13.
[MnIII10(μ3-O)4(μ-OH)4(μ-OMe)4(L)4(H2O)4](NO3)2 (2).
Mn(NO3)2·6H2O (143.5 mg, 0.5 mmol) and LH3 (207.16 mg, 1.00 mmol) were stirred in MeOH for 30 minutes in the presence of NEt3 (1 mmol). The solution was then filtered and allowed to stand. Crystals of 2 formed in ∼3 days in a yield of 25%. Elemental analysis (%) calcd for C40H96Mn10N14O34 (2): C 25.74, H 5.18, N 10.51; found: C 25.62, H 5.06, N 10.42.
Crystallography
Using Olex2,18 the structures were solved with the SHELXT19 structure solution program using Intrinsic Phasing and refined with the SHELXL20 refinement package using Least Squares minimisation. Crystal data for 1 (CCDC 2042257†): C58H148Mn16N24O62 (M = 3053.04 g mol−1), monoclinic, space group P21/n (no. 14), a = 15.3417(7) Å, b = 22.1812(11) Å, c = 18.9436(8) Å, β = 92.046(2)°, V = 6442.3(5) Å3, Z = 2, T = 200.0 K, Bruker Apex II diffractometer, μ(CuKα) = 13.098 mm−1, Dcalc = 1.574 g cm−3, 160
106 reflections measured (6.138° ≤ 2Θ ≤ 131.672°), 11
061 unique (Rint = 0.0991, Rsigma = 0.0384) which were used in all calculations. The final R1 was 0.0743 (I > 2σ(I)) and wR2 was 0.2285 (all data). Crystal data for 2 (CCDC 2042258†): C40H96Mn10N14O34 (M = 1866.70 g mol−1), tetragonal, space group I41/a (no. 88), a = 18.2751(8) Å, c = 32.445(3) Å, V = 10
836.1(13) Å3, Z = 4, T = 100.0 K, Bruker D8 Venture diffractometer, μ(CuKα) = 9.687 mm−1, Dcalc = 1.144 g cm−3, 182
310 reflections measured (8.748° ≤ 2Θ ≤ 149.676°), 5547 unique (Rint = 0.0634, Rsigma = 0.0144) which were used in all calculations. The final R1 was 0.0297 (I > 2σ(I)) and wR2 was 0.0927 (all data). Neighbouring clusters in the structure of 2 pack so as to form large solvent/anion occupied spaces that are extremely disordered as evidenced by the presence of diffuse electron density. The presence of nitrate counterions in the large voids in the structure of 2 was confirmed by IR spectroscopy. Given the diffuse nature of the density in the difference map it is not possible (or sensible) to try and model this.
Magnetometry
Variable-temperature and variable-field magnetic measurements were carried out using a MPMS-XL Quantum Design magnetometer equipped with a 5 T magnet. Diamagnetic corrections were applied using Pascal's constants.
Powder-XRD measurements
Powder XRD measurements were collected on freshly prepared samples of the complexes on a PANanalytical X'Pert Pro MPD diffractometer.
Infra-red spectroscopy
FTIR–ATR (Fourier-transform infrared–attenuated total reflectance) spectra were recorded on a PerkinElmer FTIR Spectrum BX spectrometer.
Results and discussion
The 1
:
1 reaction of LH3 with Mn(NO3)2·6H2O in a basic MeOH solution produces dark brown crystals of [MnIII12MnII4(μ3-O)6(μ-OH)4(μ3-OMe)2(μ-OMe)2(L)4(LH)2(H2O)10](NO3)6(OH)2 (1; Fig. 2) after 5 days. 1 crystallises in a monoclinic cell and structure solution was performed in the space group P21/n. The asymmetric unit of 1 contains half the cluster complex and three nitrate/one hydroxide counter ions. The metallic skeleton of the asymmetric unit contains a non-planar, asymmetric [MnIII6MnII2] square wheel (Fig. 2, top) of corner sharing [MnIII3] (Mn2–Mn4, Mn6–Mn8) and [MnIII2MnII] triangles in which the two MnII ions (Mn1, Mn5) are opposed. The three μ3-O2− ions (O13, O14, O16) occupy three of the four positions on the inside of the square wheel (Mn2, Mn4, Mn6, Mn8) further bridging to MnIII ions (Mn3, Mn7) or a MnII ion (Mn1) in the [MnIII3] and [MnIII2MnII] triangles, respectively. The fourth side of the inner wheel (Mn4, Mn6) is occupied by a μ-OH− ion (O15) which is H-bonded to O13 (O⋯O, 2.806 Å). This does not bridge to the third Mn ion in its triangle, this job being performed by the sole μ3-MeO− ion (O11) present. The remaining μ-OH/OMe ions bridge between neighbouring MnIII ions around the outside of the wheel (Mn3–O9(H)–Mn3, Mn6–O12(H)–Mn7, Mn3–O10(Me)–Mn4). The three 1,3,5-tri(2-hydroxyethyl)-1,3,5-triazacyclohexane ligands are of two types, two are fully deprotonated (L3−) and one is doubly deprotonated (LH2−). One μ5-bridging L3− ion directs the formation of a [MnIII3] triangle (Mn2–4) through N,O-chelation, with two of its three O-atoms (O1, O3) further bridging to the neighbouring MnII ions. The third O-atom (O2) remains terminally coordinated. The second μ6-L3− ion bridges in a similar fashion, but with the third O-atom now bridging between the two [Mn8] wheels (Fig. 2, middle). The μ5-LH2− ligand N,O-chelates to the Mn ions in the ‘lower’ [MnIII2MnII] triangle (Mn1, Mn2, Mn8). The deprotonated O-atoms further bridge to neighbouring MnIII ions, while the protonated arm remains terminally coordinated to Mn1. The MnIII ions are all six-coordinate and in Jahn-Teller (JT) distorted octahedral geometries. In each case the JT axis is directed by the Mn–N(L) bonds. The coordination of Mn3 is completed with a single H2O molecule. The MnII ions are also six coordinate and in regular octahedral geometries, with Mn1 having one and Mn5 having three coordinated H2O molecules.
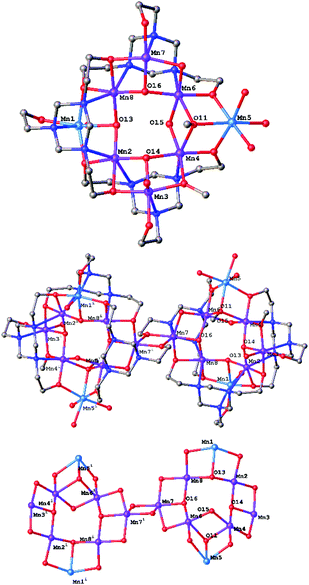 |
| Fig. 2 The asymmetric unit found in 1 (top) and its symmetry expanded structure (middle). The metallic core of 1, showing the connection of the two {Mn8} units (bottom). Colour code: MnIII = purple, MnII = light blue, O = red, N = blue, C = grey. H-atoms and counter anions have been omitted for clarity. | |
There are several short intermolecular interactions. The H2O molecule (O0) and terminally bonded O(L) atom (O2) on Mn3 are H-bonded to their symmetry equivalent atoms on neighbouring molecules (O⋯O, ∼2.63 Å) creating 1D chains of wheels down the c-axis of the crystal. (O0) is also H-bonded to a NO3− counter anion (O0⋯O20, ∼2.86 Å) which is further H-bonded to both H2O solvent of crystallisation (O21⋯O31, ∼2.80 Å) and to the H2O molecule (O22⋯O29, ∼2.63 Å) and terminal O(L) on Mn1 (O7⋯O21, ∼2.75 Å). The NO3− counter anions are also H-bonded to the H2O molecules on Mn5 (O17⋯O25, ∼2.78 Å; O18⋯O23, ∼2.72 Å; O19⋯O27, ∼2.87 Å) and the μ-OH− ion bridging between Mn6–Mn7. The result is a complicated network of interactions in all three dimensions.
Repeating the reaction that produces 1, but increasing the Mn
:
LH3 ratio to 1
:
2 produces the complex [MnIII10(μ3-O)4(μ-OH)4(μ-OMe)4(L)4(H2O)4](NO3)2 (2). 2 crystallises in the tetragonal space group I41/a (Fig. 3, top) with three MnIII ions, one O2− (O5), one OMe− (O4) and one OH− (O7) ion in the assymetric unit. The metallic skeleton of 2 describes a rather contorted [MnIII10] square wheel of corner sharing [MnIII3O] triangles, twisted at its centre such that the top half is orientated perpendicular to the bottom half (Fig. 3, bottom). There are two corner sharing [MnIII3O] triangles in each [Mn5] half, each with a μ3-O2− at its centre and a μ-OMe− along the Mn1–Mn3 edge (Mn1–O4–Mn01, 96.9°). The two halves of the molecule are connected via four μ-OH− ions (Mn01–O7–Mn01, ∼138°), which are H-bonded to the μ3-O2− ions (O7⋯O5, 2.895 Å). There are two L3− ligands in each [Mn5] half of the molecule bonding in an identical μ4-fashion, N,O-chelating to the MnIII ions with just one of the three arms (O2) further bridging to a neighbouring metal centre. The MnIII ions are all in JT distorted octahedral geometries, again dictated by the Mn–N(L) bonds. The remaining coordination site on Mn1 is occupied by a H2O molecule (O6) which, alongside O1(L), H-bond to the symmetry equivalent atoms on neighbouring molecules (O6⋯O1, 2.588 Å). The result is that the [Mn10] clusters pack in an aesthetically pleasing brickwork-like fashion, forming large solvent filled channels (Fig. 4). O6 also forms an internal H-bond to one of the terminally bonded O(L) atoms (O6⋯O3, 2.579 Å).
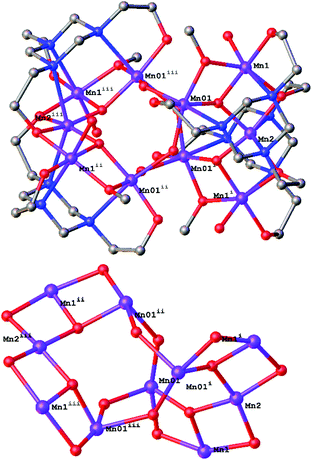 |
| Fig. 3 The crystal structure of 2 (top) and its metallic core (bottom). Colour code: MnIII = purple, O = red, N = blue, C = grey. H-atoms and counter anions have been omitted for clarity. | |
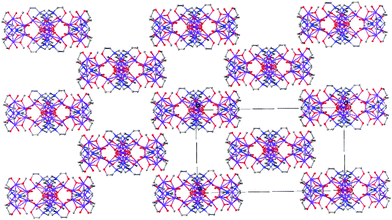 |
| Fig. 4 The brickwall-like crystal packing of 2 in the ac plane. Colour code: MnIII = purple, O = red, N = blue, C = grey. H-atoms and counter anions have been omitted for clarity. | |
There are several structural similarities between 1 and 2. Both are square wheels composed of corner-sharing [Mn3O]n+ triangles as directed by the L3− and LH2− ligands. The N-atoms of the ligands also dictate the orientation of the JT axes (and hence the dz2 orbital) of the MnIII ions, which has important design consequences for tuning magnetic exchange and magnetic anisotropy.21 Both compounds possess terminally bonded H2O molecules which mediate similar intermolecular interactions in the extended structure. Perhaps the biggest differences between the two compounds, despite the very similar synthetic procedures, is the high symmetry of 2versus the asymmetry of 1, and the dimerization of wheels in 1versus the single wheel in 2. The intricacies involved in driving these differences are unknown and will require a larger library of clusters to be synthesised and characterised. Given that 1 and 2 are just the second and third Mn complexes made with LH3, it would seem likely that many more species await discovery. It also suggests that other homo- and heterometallic 3d and 4f cluster compounds will be readily accessible. A search of the Cambridge structural database reveals that, bar [MnII2MnIII14(trz)14L4(μ3-O)8(H2O)10](ClO4)6, there are no [Mn16] or [Mn10] molecules in the literature with similar topologies to 1 and 2.
Magnetic properties
The direct current (dc) molar magnetic susceptibility, χ, of freshly prepared polycrystalline samples of 1 and 2 were measured in an applied field, B, of 0.1 T, over the 2–300 K temperature, T, range. The purity of the samples was verified by means of PXRD comparison with the simulated data from the single-crystal structure (Fig. S3†). The experimental results are showed in Fig. 5, in the form of the χT product, where χ = M/B, and M is the magnetisation of the sample. At room temperature the χT products of 1 (36.0 cm3 K mol−1) and 2 (15.4 cm3 K mol−1) are lower than the sum of the Curie constants expected for non-interacting [MnIII12MnII4] (53.5 cm3 K mol−1) and [MnIII10] (30 cm3 K mol−1) units, respectively. As temperature decreases, the χT product for both complexes decreases rapidly and for 2 reaches a value close to 0 cm3 K mol−1 at T = 2 K, clearly indicative of strong antiferromagnetic exchange and a diamagnetic ground state. For 1, there is a plateau in the value of χT ≈ 24 cm3 K mol−1 between T = 15–25 K, before it decreases rapidly to a value of 10 cm3 K mol−1 at T = 2 K. The plateau in χT is suggestive of the presence of competing ferro- and antiferromagnetic interactions which may, or may not, be related to the dimeric nature of the structure. Low-temperature variable-temperature-and-variable-field magnetisation data were measured in the temperature range 2–7 K, in magnetic fields up to 5.0 T (Fig. 6). At the lowest temperature and highest field measured, M reaches a value of ∼22.2 μB and ∼1.1 μB for 1 and 2, respectively. The nuclearity of the two compounds (and the structural complexity of 1) precludes any quantitative analysis. We note that the magnetic behaviour of the wheel-like complex [MnII2MnIII14(trz)14L4(μ3-O)8(H2O)10](ClO4)6 is also dominated by AF exchange interactions.17
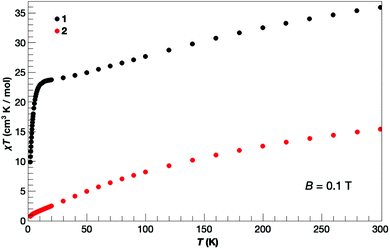 |
| Fig. 5 Temperature dependence of the χT product, where χ is the dc molar magnetic susceptibility, for 1 and 2, as labelled, collected for an applied magnetic field of B = 0.1 T. | |
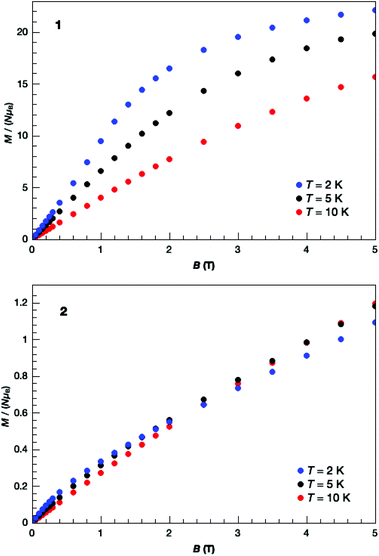 |
| Fig. 6 Isothermal molar magnetisation M versus applied magnetic field, for 1 (top) and 2 (bottom), collected for T = 2, 5 and 10 K, as labelled. | |
Conclusions
The first concerted effort at examining the coordination chemistry of LH3 with Mn has afforded two large and unusual cages: a [Mn16] double square wheel and a [Mn10] contorted square wheel. Both are constructed from corner sharing [Mn3O]n+ triangles dictated by the presence of N,O-chelating L3− and LH2− ligands, which also direct the JT axes of the MnIII ions along the Mn–N(L) bonds. While 1 describes two linked, offset [MnIII6MnII2] wheels, 2 is a single wheel but one in which the upper half is oriented perpendicular to the lower half. Magnetic measurements reveal the presence of strong antiferromagnetic interactions and a diamagnetic ground state in 2 and strong, competing exchange interactions in 1.
The simplicity of the synthetic procedures that produce 1 and 2 suggests that many more Mn coordination compounds constructed with LH3 await discovery. Variation in metal salt, oxidation state, base, solvent, co-ligands, temperature and pressure have proved enormously successful in the coordination chemistry of ethanolamine-based ligands with Mn to date.11–15 Building a library of such species is the first step to understanding what controls the self-assembly process, which, in turn, aids interpretation and exploitation of magneto-structural parameters. We also note that there is no coordination chemistry of this ligand with any other paramagnetic 3d or 4f metal ions. There therefore remains much synthetic chemistry to be explored.
Conflicts of interest
There are no conflicts to declare.
Acknowledgements
CJM and TGT thank the Hellenic Foundation for Research and Innovation (H.F.R.I.) under the “First Call for H.F.R.I. Research Projects to support Faculty members and Researchers and the procurement of high-cost research equipment grant” (Project Number: 400). EKB thanks the EPSRC for financial support under grant reference numbers EP/I03255X/1 and EP/I031421/1. GL and ME thank the Ministerio de Ciencia e Innovación (RTI2018-094909-J-I00) and CSIC (PIE 201960E002).
Notes and references
- B. Bleaney and K. D. Bowers, Anomalous paramagnetism of copper acetate, Proc. R. Soc. London, 1952, A214, 451 Search PubMed.
- K. Kambe, On the paramagnetic susceptibilities of some polynuclear complex salts, J. Phys. Soc. Jpn., 1950, 5, 48 CrossRef CAS.
- J. Glerup, D. J. Hodgson and E. Petersen, A novel correlation between magnetism and structural parameters in superexchange coupled chromium(III) dimer, Acta Chem. Scand., 1983, A37, 161 CrossRef CAS.
- H. Weihe and H.-U. Güdel, Angular and distance dependence of the magnetic properties of oxo-bridged iron(III) dimer, J. Am. Chem. Soc., 1997, 119, 6539 CrossRef CAS.
- T. Cauchy, E. Ruiz and S. Alvarez, Magnetostructural correlations in polynuclear complexes: The Fe4 butterflies, J. Am. Chem. Soc., 2006, 128, 15722 CrossRef CAS PubMed.
- A. Caneschi, D. Gatteschi, R. Sessoli, A.-L. Barra, L.-C. Brunel and M. J. Guillot, Alternating current susceptibility, high field magnetization, and millimeter band EPR evidence for a ground S=10 state in [Mn12O12(CH3COO)16 (H2O)4]·2CH3COOH·4H2O, J. Am. Chem. Soc., 1991, 113, 5873 CrossRef CAS; R. Sessoli, H.-L. Tsai, A. R. Schake, S. Wang, J. B. Vincent, K. Folting, D. Gatteschi, G. Christou and D. N. Hendrickson, High-spin molecules: [Mn12O12(O2CR)16(H2O)4], J. Am. Chem. Soc., 1993, 115, 1804 CrossRef; R. Sessoli, D. Gatteschi, A. Caneschi and M. A. Novak, Magnetic bistability in a metal-ion cluster, Nature, 1993, 365, 141 CrossRef; G. Christou, D. Gatteschi, D. N. Hendrickson and R. Sessoli, Single-molecule magnets, MRS Bull., 2000, 25, 66 CrossRef.
- T. Lis, Preparation, structure, and magnetic properties of a dodecanuclear mixed-valence manganese carboxylate, Acta Crystallogr., 1980, B36, 2042 CrossRef CAS.
- J. B. Vincent, C. Christmas, J. C. Huffman, G. Christou, H.-R. Chang and D. N. Hendrickson, Modelling the photosynthetic water oxidation centre: Synthesis, structure, and magnetic properties of [Mn4O2(OAc)7(bipy)2](ClO4)·3H2O (bipy=2,2′-Bipyridine), J. Chem. Soc., Chem. Commun., 1987, 236 RSC; J. S. Bashkin, H.-R. Chang, W. E. Streib, J. C. Huffman, D. N. Hendrickson and G. Christou, Modelling the photosynthetic water oxidation center: preparation and physical properties of a tetranuclear oxide bridged Mn complex corresponding to the native S2 state, J. Am. Chem. Soc., 1987, 109, 6502 CrossRef CAS.
- A. J. Tasiopoulos, A. Vinslava, W. Wernsdorfer, K. A. Abboud and G. Christou, Giant single–molecule magnets: A {Mn84} torus and its supramolecular nanotubes, Angew. Chem., Int. Ed., 2004, 116, 2117 CrossRef PubMed.
- G. Aromí and E. K. Brechin, Synthesis of 3d metallic single-molecule magnets, Struct. Bonding, 2006, 122, 1 CrossRef; C. J. Milios and R. E. P. Winpenny, Cluster-based single-molecule magnets, Struct. Bonding, 2014, 164, 1 CrossRef.
- M. A. Bolcar, S. M. J. Aubin, K. Folting, D. N. Hendrickson and G. Christou, A new manganese cluster topology capable of yielding high-spin species: Mixed-valence [Mn7(OH)3Cl3(hmp)9]2+ with S ≥ 10, Chem. Commun., 1997, 1485 RSC.
- E. K. Brechin, J. C. Huffman, G. Christou, J. Yoo, M. Nakano and D. N. Hendrickson, A new class of single-molecule magnets: mixed-valent [Mn4(O2CMe)2(Hpdm)6][ClO4]2 with an S=8 ground state, Chem. Commun., 1999, 783 RSC.
- K. R. Vignesh, S. K. Langley, B. Moubaraki, K. S. Murray and G. Rajaraman, Large hexadecametallic {MnIII–LnIII} wheels: Synthesis, structural, magnetic, and theoretical characterization, Chem. – Eur. J., 2015, 21, 16364 CrossRef CAS PubMed; A. J. Tasiopoulos and S. P. Perlepes, Diol-type ligands as central ‘players’ in the chemistry of high-spin molecules and single-molecule magnets, Dalton Trans., 2008, 5537 RSC.
- A. Baniodeh, Y. Lan, G. Novitchi, V. Mereacre, A. Sukhanov, M. Ferbinteanu, V. Voronkova, C. E. Anson and A. K. Powell, Magnetic anisotropy and exchange coupling in a family of isostructural FeIII2LnIII2 complexes, Dalton Trans., 2013, 42, 8926 RSC.
- T. N. Hooper, R. Inglis, G. Lorusso, J. Ujma, P. E. Barran, D. Uhrin, J. Schnack, S. Piligkos, M. Evangelisti and E. K. Brechin, Structurally flexible and solution stable [Ln4TM8(OH)8(L)8(O2CR)8(MeOH)y](ClO4)4: A playground for magnetic refrigeration, Inorg. Chem., 2016, 55, 10535 CrossRef CAS PubMed.
- M. V. Baker, D. H. Brown, B. W. Skelton and A. H. White, Chromium complexes of hydroxyl-functionalised 1,3,5-triazacyclohexanes, J. Chem. Soc., Dalton Trans., 1999, 1483 RSC.
- M. Riaz, R. K. Gupta, H.-F. Su, Z. Jagličić, M. Kurmoo, C.-H. Tung, D. Sun and L.-S. Zheng, Hexadecanuclear MnII2MnIII14 molecular torus built from in situ tandem ligand transformations, Inorg. Chem., 2019, 58, 14331 CrossRef CAS PubMed.
- O. V. Dolomanov, L. J. Bourhis, R. J. Gildea, J. A. K. Howard and H. Puschmann, OLEX2: a complete structure solution, refinement and analysis program, J. Appl. Crystallogr., 2009, 42, 339 CrossRef CAS.
- G. M. Sheldrick, SHELXT – Integrated space-group and crystal-structure determination, Acta Crystallogr., Sect. A: Found. Adv., 2015, A71, 3 CrossRef PubMed.
- G. M. Sheldrick, Crystal structure refinement with SHELXL, Acta Crystallogr., Sect. C: Struct. Chem., 2015, C71, 3 Search PubMed.
- P. Comar, T. Rajeshkumar, G. S. Nichol, M. B. Pitak, S. J. Coles, G. Rajaraman and E. K. Brechin, Switching the orientation of Jahn–Teller axes in oxime-based MnIII dimers and its effect upon magnetic exchange: a combined experimental and theoretical study, Dalton Trans., 2015, 44, 19805 RSC; W. P. Barros, R. Inglis, G. S. Nichol, T. Rajeshkumar, G. Rajaraman, S. Piligkos, H. O. Stumpf and E. K. Brechin, From antiferromagnetic to ferromagnetic exchange in a family of oxime-based MnIII dimers: a magneto-structural study, Dalton Trans., 2013, 42, 16510 RSC; N. Berg, T. Rajeshkumar, S. M. Taylor, E. K. Brechin, G. Rajaraman and L. F. Jones, What Controls the magnetic interaction in bis–μ–alkoxo MnIII dimers? A combined experimental and theoretical exploration, Chem. – Eur. J., 2012, 18, 5906 CrossRef CAS PubMed.
Footnote |
† Electronic supplementary information (ESI) available: FTIR-ATR spectra (Fig. S1), PXRD patterns (Fig. S2), coordination modes of the ligand (Fig. S3), BVS calculations (Tables S1 and S2). See DOI: 10.1039/d0qi01495h |
|
This journal is © the Partner Organisations 2021 |
Click here to see how this site uses Cookies. View our privacy policy here.