C4-aldehyde of guaiazulene: synthesis and derivatisation†
Received
23rd December 2020
, Accepted 25th February 2021
First published on 4th March 2021
Abstract
Guaiazulene is an alkyl-substituted azulene available from natural sources and is a much lower cost starting material for the synthesis of azulene derivatives than azulene itself. Here we report an approach for the selective functionalisation of guaiazulene which takes advantage of the acidity of the protons on the guaiazulene C4 methyl group. The aldehyde produced by this approach constitutes a building block for the construction of azulenes substituted on the seven-membered ring. Derivatives of this aldehyde synthesised by alkenylation, reduction and condensation are reported, and the halochromic properties of a subset of these derivatives have been studied.
Introduction
Azulene (1) is a non-alternant bicyclic aromatic hydrocarbon, that has significantly different properties to those of its isomer naphthalene (2).1 Whereas the HOMO–LUMO gap in naphthalene leads to absorption in the UV region, the corresponding molecular orbitals are closer in energy in azulene, hence leading to an absorption in the visible region. Thus, naphthalene is colourless but azulene is blue. Azulene also displays anomalous fluorescence characteristics, being an exception to Kasha's rule, with the S2 → S0 transition being the dominant mode of emission.2 Additional interesting optical properties such as halochromism,3 solvatochromism4 and barochromism5 have also been reported for certain azulenes. Azulene also has a dipole moment of 1.08 D, unusually high for a simple hydrocarbon.6 This arises from the contribution of resonance structure 1′, in which both individual rings are 6π aromatic systems (Fig. 1). These notable properties have led to azulenes being used extensively as both colorimetric7 and fluorescent8 probes for various analytes. Azulenes have also been employed in solar cells9 and organic electronics,10 as well as in medicinal chemistry.11
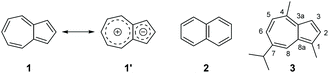 |
| Fig. 1 Structures of azulene, naphthalene and guaiazulene. | |
These various applications have been enabled by the development of synthetic routes to substituted azulenes.12 One approach is to synthesise the azulene ring from a precursor which already incorporates the desired substituents, for example by aromatisation of 1,1-dicyanodihydroazulenes13 or by a carbene insertion/ring expansion approach.14 Another strategy is to employ synthetic methodology that introduces the desired substituents onto a pre-existing azulene ring. Approaches for functionalisation of the 5-membered ring of azulene include SEAr reactions,15 cross-coupling16 and C–H activation.17 Approaches for functionalisation of the 7-membered ring of azulene are less numerous, but include vicarious nucleophilic substitution18 and nucleophilic addition/rearomatisation.16g,19 However, regardless of the diversity of approaches for azulene derivatisation, work in this area is hindered by the high cost of azulene itself – preparation of the parent hydrocarbon requires multistep procedures and/or reagents that are themselves costly.15a,20 There is therefore a motivation to use an alternative azulene starting material which is abundantly available from natural sources and hence has a much lower cost, namely guaiazulene (3).
Guaiazulene and its derivatives are sesquiterpene natural products produced by various organisms, in particular gorgonian octocorals and the fungal genus Lactarius.21 Moreover, guaiazulene may be produced from the essential oils isolated from genera such as Eucalyptus,22Matricaria (chamomile)23 and others.24 These processes proceed via the dehydration/dehydrogenation of other sesquiterpenes such as guaiol and occur in high yields, permitting the large scale production of guaiazulene at low cost. The main uses of guaiazulene and its derivative sodium guaiazulene-3-sulfonate are as anti-inflammatory agents and in cosmetics.25
The alkyl groups on guaiazulene do not influence the azulene chromophore significantly, but they impart a much lower melting point (30–33 °C)26 and guaiazulene can undergo very slow decomposition on exposure to air.27 However, derivatives bearing electron withdrawing groups are generally stable. Methods for derivatisation of guaiazulene are not as extensively developed as for azulene itself and in some instances may be hindered by the alkyl substituents. On the other hand, the alkyl substituents of guaiazulene can be seen as handles for further derivatisation themselves.28 One strategy to achieve this exploits the known acidity of the protons on the guaiazulene C4 methyl group.3h,17n,29 The low pKa of these protons arises from the resonance stabilisation of the corresponding conjugate base, [3–H]− that may be attributed to resonance structure [3′–H]− (Scheme 1) that comprises an aromatic cyclopentadienide substructure.
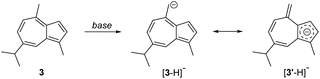 |
| Scheme 1 Guaiazulene deprotonates preferentially at the C4 methyl group. | |
Amide acetals such as dimethylformamide dimethyl acetal (DMFDMA) are versatile reagents able to effect a variety of synthetic transformations.30 Reaction of an amide acetal with aryl methyl groups to give the corresponding enamines was first reported by Meerwein31 and was further developed by Bredereck.32 Oxidative cleavage of such enamines is possible using sodium periodate (with no requirement for osmium tetroxide).33 Taken together, these two procedures allow formation of an aryl aldehyde in two steps from the corresponding aryl methyl group. The reaction mechanism requires that the arene is able to stabilise a benzylic anion, hence electron-poor arene substrates are generally required (Scheme 2a). We reasoned that this synthetic sequence could be applicable to guaiazulene, resulting in a regioselective transformation of the C4 methyl group to an aldehyde, although whether the C4 methyl protons were sufficiently acidic was unclear at the outset. To our knowledge there are three prior reports of azulenyl aldehydes being formed from the corresponding azulene methyl groups in this way. In 2006, Kim, Osuka and co-workers reported the reaction of a 4-methylazulene 4, bearing an electron-withdrawing ester at the 1-position, which would lower the pKa of the C4 methyl protons (Scheme 2b).34 In 2015, Leino and co-workers reported the reaction of 6-methylazulene 6, which lacks any other substituents (Scheme 2c).35 Most recently, in 2018 Shoji and co-workers reported the reaction of various 2-methylazulenes of type 8, finding that the reaction did not proceed without an electron-withdrawing group at the 1-position (Scheme 2d).36
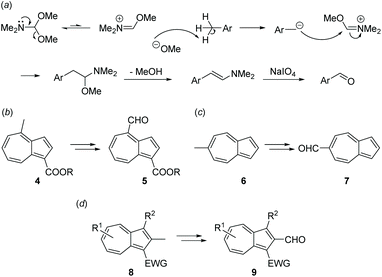 |
| Scheme 2 (a) Mechanism for transformation of aryl methyl group to aryl aldehyde. (b) Work of Kim, Osuka and co-workers. (c) Work of Leino and co-workers. (d) Work of Shoji and co-workers. | |
In this paper we describe the first synthesis of guaiazulene-4-carbaldehyde, as well as some further transformations of this compound.
Results and discussion
Treatment of guaiazulene 3 with DMFDMA in DMF gave crude enamine 10 as a green solid after aqueous workup.‡ This proved to be somewhat unstable and could not be purified by chromatography on silica. Thus, 10 was used crude, and was directly subjected to oxidative cleavage with NaIO4 to give novel aldehyde 11 in 77% yield over two steps (Scheme 3). In contrast to 10, aldehyde 11 could be purified by chromatography on silica, and is stable in pure form. Crystals of 11 were subjected to X-ray diffraction analysis and the resultant structure is shown in Fig. 2.
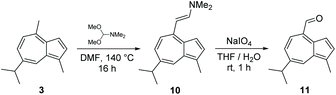 |
| Scheme 3 Synthesis of guaiazulene-4-carbaldehyde 11. | |
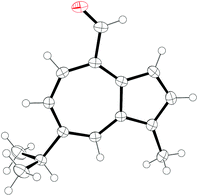 |
| Fig. 2 ORTEP representation of the X-ray structure of 11. Ellipsoids are shown at 50% probability. Hydrogens are shown as spheres of arbitrary radius. CCDC 2047319.† | |
We anticipate that aldehyde 11 may find various uses in synthesis, since it is accessible in a concise fashion from a cheap azulenic starting material. Reduction of 11 with NaBH4 furnished alcohol 12 in sufficient purity that chromatography was not required (Scheme 4a). Alkenylation of 11 by Horner–Wadsworth–Emmons reaction was carried out using a variety of phosphonates (Scheme 4b, Table 1).37 Alkenes 20 and 21 were synthesised from phosphonate reagents containing additional Lewis basic groups, and as such the modified Masamune–Rousch reaction conditions were employed in these instances.38
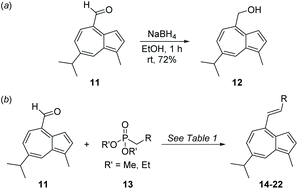 |
| Scheme 4 (a) Reduction of 11. (b) Horner–Wadsworth–Emmons reaction of 11. | |
Table 1 Horner–Wadsworth–Emmons reactions of 11
Entry |
R |
Product |
R′ |
Methoda,b |
Yield (%) |
Method A: 60% NaH (2 eq.), THF, rt, 2 h.
Method B: LiCl (3 eq.), DBU (3 eq.), THF, 0 °C, 3 h.
|
1 |
|
14
|
Et |
A |
38 |
2 |
|
15
|
Et |
A |
46 |
3 |
|
16
|
Et |
A |
18 |
4 |
|
17
|
Et |
A |
56 |
5 |
|
18
|
Et |
A |
42 |
6 |
|
19
|
Et |
A |
44 |
7 |
|
20
|
Me |
B |
41 |
8 |
|
21
|
Et |
B |
33 |
9 |
|
22
|
Et |
A |
50 |
C4-alkenylated guaiazulene derivatives such as 14–22 have been reported previously,3h,11j,29c,j,29l–n,29r although in these cases the C4 methyl carbon reacted as a nucleophile, as opposed to as an electrophile as is the case here. A notable property of certain C4-alkenylated guaiazulenes described previously is their halochromism.3h,29c Accordingly, selected alkenylated derivatives of 11 and their response to Brønsted acid were studied. 4-Fluorostyrylazulene 19 was titrated against increasing quantities of trifluoroacetic acid in CH2Cl2 and UV/vis absorption spectra were acquired (Fig. 3).
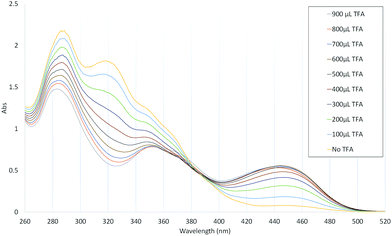 |
| Fig. 3 Titration of 19 (0.08 mM) with TFA (1 M in CH2Cl2) in CH2Cl2. | |
The absorption maxima at λ = 289 nm and λ = 319 nm decreased in intensity with increasing acid concentration, whereas a new absorption maximum at λ = 447 nm (in the blue region) was seen to increase in intensity; an isosbestic point was observed at λ ≈ 388 nm. 1H-NMR spectra of 19 in CDCl3 and in CDCl3/TFA were acquired (Fig. 4), and show the site of protonation to be the guaiazulene C3 position. Specifically, for the spectrum in CDCl3, the H-3 resonance is observed as a 1H doublet at 7.46 ppm, whereas in TFA/CDCl3 it is observed as a 2H broad singlet at 4.08 ppm, this chemical shift being indicative of an sp3-hybridised carbon. Further support for the guaiazulene C3 position being the site of protonation comes from comparison with spectra of similar azulenium cations generated by hydride abstraction from dihydroazulenes.13d,39
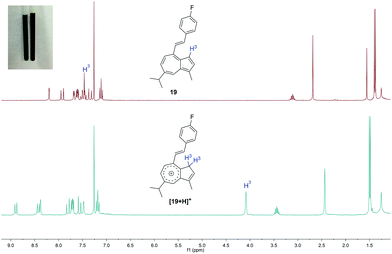 |
| Fig. 4 Top: 1H-NMR spectrum of 19 in CDCl3. Bottom: 1H-NMR spectrum of 19 in TFA/CDCl3 1 : 9. Inset: NMR tubes containing 19 in CDCl3 (left) and 19 in TFA/CDCl3 (right). | |
Cyano-substituted 15, chloro-substituted 18 and α,β-unsaturated ester 20 were investigated in a similar fashion, and all were observed by NMR to undergo protonation at the same position (Fig. S1–S3†). Whereas 15 and 18 exhibited similar maxima and isosbestic points to 19 (Fig. S4 and S5†), the isosbestic point for 20 was blue shifted (Fig. S6†). As a further demonstration of the scope for functionalisation of 11, formation of a nitrone was attempted. Azulenyl nitrones are of interest as free radical spin traps with neuroprotective activity and potential clinical utility.40 In the present case, condensation of 11 with N-tert-butylhydroxylamine gave nitrone 23 (Scheme 5).
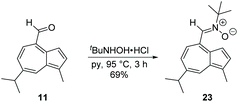 |
| Scheme 5 Synthesis of nitrone 23. | |
Conclusions
We have described the concise synthesis of an azulene bearing an aldehyde at the 4-position (11). The use of a low-cost, commercially available azulene starting material and the requirement for only one purification step render 11 an attractive building block for the synthesis of more complex azulenes bearing substituents at the 4-position. We have demonstrated the utility of 11 through its use in reduction, alkenylation and condensation reactions.
Experimental
General details
Reactions which required the use of anhydrous, inert atmosphere techniques were carried out under an atmosphere of argon. Reaction solvents were obtained by passing though anhydrous alumina columns using an Innovative Technology Inc. PS-400-7 solvent purification system. In most cases, solvents were purchased as “anhydrous” grade from Fisher Scientific. “Petrol” refers to petroleum spirit b.pt. 40–60 °C. TLC was performed using aluminium backed plates pre-coated with Alugram®SIL G/UV 254 nm. Where needed, visualisation was accomplished by UV light and/or KMnO4 or using phosphomolybdic acid (PMA) dip followed by gentle warming. Following work up, the organic layers were routinely dried using anhydrous MgSO4 and evaporated using a Büchi rotary evaporator. When necessary, further drying was facilitated by high vacuum. During purification, flash column chromatography was carried out using 60 angstroms (Å) silica gel (40–75 micron) purchased from Sigma Aldrich. 1H and 13C NMR spectra were acquired on Agilent ProPulse 500 MHz or Bruker Advance 250, 300, 400 or 500 megahertz (MHz) instruments at 298 K. All spectra were referenced to residual solvent peaks; chemical shifts are reported in parts per million (ppm) relative to residual chloroform (δ = 7.26 ppm, 1H; 77.16 ppm, 13C). All 13C{1H} resonances are singlets, unless stated otherwise. Coupling constants, J, reported in Hz, were calculated using MestreNova 9.0 to the nearest 0.1 Hz. The following abbreviations are used to label the multiplicities: s, singlet; d, doublet; t, triplet; q, quartet; quin, quintet; sex, sextet; sep, septet; dd, doublet of doublets; dq, doublet of quartets; td, triplet of doublets; m, multiplet and br, broad. 1H and 13C{1H} assignments for novel compounds are corroborated though 2D (COSY, HSQC, HMBC). Infrared (IR) spectra were recorded on a PerkinElmer Spectrum 100 ATR-FTIR spectrometer with only selected absorbances quoted as ν in cm−1. For mass spectrometry a microTOF electrospray time-of-flight (ESI-TOF) mass spectrometer (Bruker Daltonik GmbH, Bremen, Germany) was used. Data are reported in the form of m/z. The observed mass and isotope pattern matched the corresponding theoretical values as calculated from the expected elemental formula. Melting points (mp) were determined on an Stanford Research Systems OptiMelt automated capillary melting point apparatus in open capillary tubes and are uncorrected. X-Ray intensity data for 11 were collected at 150(2) K on a Rigaku SuperNova Dual EosS2 single crystal diffractometer using monochromated Cu-Kα radiation (λ = 1.54184 Å). Unit cell determination, data collection data reduction and absorption correction were performed using the CrysAlisPro software (version 1.171.40.43a, Rigaku Oxford Diffraction, 2019). The structure was solved with SHELXT and refined by a full-matrix least-squares procedure based on F2 (SHELXL-2018/3). All non-hydrogen atoms were refined anisotropically. Hydrogen atoms were placed onto calculated positions and refined using a riding model.
7-Isopropyl-1-methylazulene-4-carbaldehyde (11).
Under an atmosphere of nitrogen, guaiazulene (0.32 g, 1.6 mmol, 1.0 eq.) was dissolved in degassed anhydrous DMF (5.0 mL), to which N,N-dimethylformamide dimethyl acetal (0.43 mL, 3.2 mmol, 2.0 eq.) was added. The reaction was heated to 140 °C and stirred for 9 h, during which the solution turned from blue to green. The solution was cooled to room temperature and diluted with ethyl acetate (30 mL). The organic mixture was washed with water (30 mL) and 5% LiCl(aq) solution (2 × 30 mL). The organic phase was dried over MgSO4 and filtered, and the filtrate was concentrated under reduced pressure to give crude 10, used immediately. Under aerobic conditions, the crude material was re-dissolved in THF/H2O (30 mL, 1
:
1, v/v), to which NaIO4 (1.0 g, 4.8 mmol, 3.0 eq.) was added. The solution was stirred room temperature for 2.5 hours, turning from green to blue, then diluted with ethyl acetate (30 mL). The organic phase was washed with water (30 mL) and sat. NaHCO3(aq) solution (2 × 30 mL), for which the initial aqueous extract was orange and the latter extract was colourless. The organic phase was dried over MgSO4 and filtered, and the filtrate was concentrated under reduced pressure. The crude material was purified by silica column chromatography, eluting with EtOAc/petrol (5
:
95, Rf = 0.50) to give 7-isopropyl-1-methylazulene-4-carbaldehyde 11 (0.21 g, 62%) as a blue-brown solid. 1H NMR (500 MHz, CDCl3) δ 1.40 (d, J = 6.9 Hz, 6H, –CH(C
3)2), 2.71 (s, 3H, C1–CH3), 3.15 (hept, J = 6.9 Hz, 1H, –C
(CH3)2), 7.56 (d, J = 10.4 Hz, 1H, C5–H), 7.63 (dd, J = 10.5, 1.9 Hz, 1H, C6–H), 7.92 (d, J = 3.6 Hz, 1H, C3–H), 8.08 (d, J = 3.7 Hz, 1H, C2–H), 8.25 (d, J = 1.3 Hz, 1H, C8–H), 10.77 (s, 1H, –CHO) ppm. 13C NMR (500 MHz, (CDCl3) δ 13.0, 24.6, 39.0, 112.4, 123.8, 126.2, 133.9, 134.1, 134.7, 136.2, 141.4, 141.6, 145.1, 195.1 ppm. IR νmax 701, 761, 1682, 2861, 2924, 2955 cm−1. Melting point 81–84 °C. HRMS (ESI+): calculated for C15H16O [M + H]+ 213.1274, found 213.1278.
(7-Isopropyl-1-methylazulen-4-yl)methanol (12).
To a solution of 11 (75 mg, 0.3 mmol, 1.0 eq.) in EtOH was added NaBH4 (27 mg, 0.7 mmol, 2.0 eq.). The solution was stirred for 1 h at rt and was then quenched with water (10 mL) and extracted with CH2Cl2 (3 × 10 mL), dried over MgSO4 and filtered, then the filtrate was evaporated under reduced pressure to give (7-isopropyl-1-methylazulen-4-yl)methanol as a turquoise gum (54 mg, 72%). 1H NMR (500 MHz, (CD3)2CO) δ 1.37 (d, J = 6.9 Hz, 6H, –CH(C
3)2), 2.65 (s, 3H, C1–CH3), 3.14 (hept, J = 6.9 Hz, 1H, –C
(CH3)2), 4.57 (t, J = 5.8 Hz, 1H, –OH), 5.18 (d, J = 5.3 Hz, 2H, –C
2OH), 7.25 (d, J = 3.7 Hz, 1H, C3–H), 7.52 (d, J = 10.7 Hz, 1H, C5–H), 7.62–7.59 (m, 2H, C2–H, C6–H), 8.27 (d, J = 2.0 Hz, 1H, C8–H) ppm. 13C NMR (500 MHz, (CD3)2CO) δ 12.9, 25.0, 39.0, 64.5, 111.3, 121.3, 125.4, 133.9, 135.7, 135.7, 137.2, 138.6, 141.4, 147.4 ppm. IR νmax 1008, 1386, 2924, 2956, 3316 cm−1. HRMS (ESI+): calculated for C15H18O [M + H]+ 215.1430, found 215.1438.
General procedure A for Horner–Wadsworth–Emmons reactions
To a stirred solution of 60% NaH (11 mg, 0.3 mmol, 2.0 eq.) in anhyd. THF (3 mL) was added dropwise a solution of the required phosphonate (0.3 mmol, 2.0 eq.) in anhyd. THF (4 mL) under nitrogen. The mixture was stirred for 10 min after which a solution of 11 (30 mg, 0.1 mmol, 1.0 eq.) in anhyd. THF (3 mL) was added dropwise. The mixture was stirred at rt until the starting material was consumed as shown by TLC (approx. 2 h). The reaction mixture was diluted with water (10 mL) and extracted with CH2Cl2 (3 × 30 mL). The combined organic fractions were dried over MgSO4 and filtered, then the filtrate was evaporated under reduced pressure. Purification was achieved by column chromatography to give the desired alkenylazulene.
(E)-4-(4-Iodostyryl)-7-isopropyl-1-methylazulene (14).
General procedure A was used with diethyl (4-iodobenzyl)phosphonate (100 mg) to give the product as a green waxy gum (22 mg, 38%) following purification via column chromatography using Petrol (100%, Rf 0.50). 1H NMR (500 MHz, (CD3)2CO) δ 1.38 (d, J = 6.9 Hz, 6H, –CH(C
3)2), 2.66 (s, 3H, C1–CH3), 3.15 (hept, J = 6.9 Hz, 1H, –C
(CH3)2), 7.48 (d, J = 16.2 Hz, 1H, C6H4–C![[H with combining low line]](https://www.rsc.org/images/entities/char_0048_0332.gif)
CH–), 7.58–7.61 (m, 5H, C3–H, C5–H, C6–H, m–I–Ar–H), 7.67 (d, J = 3.9 Hz, 1H, C2–H), 7.80 (d, J = 8.4 Hz, 2H, o–I–Ar–H), 8.20 (d, J = 16.2 Hz, 1H, C6H4–CH
C
–), 8.25 (s, 1H, C8–H) ppm. 13C NMR (500 MHz, (CD3)2CO) δ 13.1, 24.9, 38.9, 94.2, 113.1, 120.8, 126.7, 130.0, 130.9, 133.7, 133.7, 135.6, 137.5, 137.7, 137.8, 138.0, 138.8, 141.0, 142.1 ppm. IR νmax 763, 950, 2958 cm−1. HMS (ESI+): calc. for C22H21I [M + H]+ 413.0761, found 413.0771.
(E)-4-(2-(7-Isopropyl-1-methylazulen-4-yl)vinyl)benzonitrile (15).
General procedure A was used with diethyl (4-cyanobenzyl)phosphonate (72 mg) to give the product as a green gum (20 mg, 46%) following purification via column chromatography using Petrol (100%, Rf 0.40). 1H NMR (500 MHz, (CD3)2CO) δ 1.38 (d, J = 6.9 Hz, 6H, –CH(C
3)2), 2.66 (s, 3H, C1–CH3), 3.16 (hept, J = 6.9 Hz, 1H, –C
(CH3)2), 7.59 (d, J = 15.9 Hz, 1H, C6H4–C![[H with combining low line]](https://www.rsc.org/images/entities/char_0048_0332.gif)
CH–), 7.59–7.60 (m, 2H, C5–H, C6–H), 7.63 (d, J = 3.9 Hz, 1H, C3–H), 7.70 (d, J = 3.9 Hz, 1H, C2–H), 7.81 (d, J = 8.4 Hz, 2H, m–CN–Ar–H), 7.98 (d, J = 8.3 Hz, 2H, o–CN–Ar–H), 8.28 (s, 1H, C8–H), 8.33 (d, J = 16.3 Hz, 1H, C6H4–CH
C
–) ppm. 13C NMR (500 MHz, (CD3)2CO) δ 13.0, 24.9, 38.9, 112.1, 113.2, 119.4, 120.8, 126.8, 128.7, 133.0, 133.4, 133.7, 133.9, 135.7, 137.7, 138.1, 138.2, 141.4, 141.6, 142.7 ppm. IR νmax 738, 787, 1262, 2222, 2849, 2917, 2959 cm−1. HRMS (ESI+): calc. for C23H21N [M + H]+ 312.1747, found 312.1753.
(E)-7-Isopropyl-1-methyl-4-(4-nitrostyryl)azulene (16).
General procedure A was used with diethyl (4-nitrobenzyl)phosphonate (77 mg) to give the product as a dark green waxy gum (8.6 mg, 18%) following purification via column chromatography using Petrol (100%, Rf 0.40). 1H NMR (500 MHz, CDCl3) δ 1.40 (d, J = 6.9 Hz, 6H, –CH(C
3)2), 2.70 (s, 3H, C1–CH3), 3.13 (hept, J = 6.9 Hz, 1H, –C
(CH3)2), 7.40 (d, J = 16.2 Hz, 1H, C6H4–C![[H with combining low line]](https://www.rsc.org/images/entities/char_0048_0332.gif)
CH–), 7.43 (d, J = 10.9 Hz, 1H, C5–H), 7.45 (br s, 1H, C3–H), 7.54 (dd, J = 10.9, 1.9 Hz, C6–H), 7.72 (s, 1H, C2–H), 7.74–7.77 (m, 2H, m–NO2–Ar–H), 8.15 (d, J = 16.2 Hz, 1H, C6H4–CH
C
–), 8.23 (d, J = 1.9 Hz, 1H, C8–H), 8.26–8.30 (m, 2H, o–NO2–Ar–H). 13C NMR (500 MHz, (CD3)2CO) δ 13.0, 24.9, 39.0, 113.2, 120.9, 124.8, 126.9, 128.9, 132.6, 133.9, 134.6, 135.7, 137.7, 138.2, 138.3, 141.4, 141.6, 144.8, 148.2 ppm. IR νmax 744, 1335, 1511, 1592, 2924, 2957 cm−1. HRMS (ESI+): calc. for C22H21NO2 [M + H]+ 332.1645, found 332.1653.
(E)-7-Isopropyl-1-methyl-4-(4-(trifluoromethyl)styryl)azulene (17).
General procedure A was used with (4-(trifluoromethyl)benzyl)phosphonate (74 mg) to give the product as a green waxy gum (25 mg, 56%) following purification via column chromatography using Petrol (100%, Rf 0.60). 1H NMR (500 MHz, (CD3)2CO) δ 1.38 (d, J = 6.9 Hz, 6H, –CH(C
3)2), 2.66 (s, 3H, C1–CH3), 3.15 (hept, J = 7.0 Hz, 1H, –C
(CH3)2), 7.59 (d, J = 16.2 Hz, 1H, C6H4–C![[H with combining low line]](https://www.rsc.org/images/entities/char_0048_0332.gif)
CH–), 7.61–7.60 (m, 2H, C5–H, C6–H), 7.62 (d, J = 3.9 Hz, C3–H), 7.69 (d, J = 3.8 Hz, 1H, C2–H), 7.76 (d, J = 8.1 Hz, 2H, m–CF3–Ar–H), 7.99 (d, J = 7.8 Hz, 2H, o–CF3–Ar–H), 8.27 (s, 1H, C8–H), 8.30 (d, J = 16.2 Hz, 1H, C6H4–CH
C
–). 13C NMR (500 MHz, (CD3)2CO) δ 142.2 (q, 4JCF = 1.5 Hz), 141.8, 141.3, 138.1, 138.1, 137.6, 135.7, 133.8, 133.2, 132.9, 130.1 (q, 2JCF = 32.0 Hz), 128.5, 126.8, 126.5 (q, 3JCF = 3.9 Hz), 125.4 (q, 1JCF = 270.9 Hz), 120.9, 113.2, 38.9, 24.9, 13.0 ppm. IR νmax 825, 1065, 1106, 1320, 1413, 2960 cm−1. HRMS (ESI+): calc. for C23H21F3 [M + H]+ 355.1668, found 355.1655.
(E)-4-(4-Chlorostyryl)-7-isopropyl-1-methylazulene (18).
General procedure A was used with diethyl (4-chlorobenzyl)phosphonate (74 mg) to yield a green waxy gum (19 mg, 42%) following purification via column chromatography using Petrol (100%, Rf 0.40). 1H NMR (500 MHz, (CD3)2CO) δ 1.38 (d, J = 7.0 Hz, 6H, –CH(C
3)2), 2.66 (s, 3H, C1–CH3), 3.15 (hept, J = 6.8 Hz, 1H, –C
(CH3)2), 7.44–7.48 (m, 2H, m–Cl–Ar–H), 7.54 (d, J = 16.2 Hz, 1H, C6H4–C![[H with combining low line]](https://www.rsc.org/images/entities/char_0048_0332.gif)
CH–), 7.59–7.60 (m, 2H, C5–H, C6–H), 7.60–7.62 (m, 1H, C3–H), 7.67–7.68 (m, 1H, C2–H), 7.79–7.84 (m, 2H, o–Cl–Ar–H), 8.19 (d, J = 16.2 Hz, 1H, C6H4–CH
C
–), 8.26 (s, 1H, C8–H) ppm. 13C NMR (500 MHz, (CD3)2CO) δ 13.1, 24.9, 38.9, 113.1, 120.7, 126.6, 129.6, 129.7, 130.8, 133.5, 133.7, 134.3, 135.6, 137.1, 137.4, 137.7, 137.8, 141.0, 142.1 ppm. IR νmax 772, 817, 1011, 1091, 1490, 2923 cm−1. HRMS (ESI+): calc. for C22H21Cl [M + H]+ 321.1405, found 321.1412.
(E)-4-(4-Fluorostyryl)-7-isopropyl-1-methylazulene (19).
General procedure A was used with diethyl (3-fluorobenzyl) phosphonate (70 mg) to give the product as a green waxy gum (19 mg, 44%) following purification via column chromatography using Petrol (100%, Rf 0.30). 1H NMR (500 MHz, (CD3)2CO) δ 1.38 (d, J = 6.9 Hz, 6H, –CH(C
3)2), 2.66 (s, 3H, C1–CH3), 3.15 (hept, J = 6.9 Hz, 1H, –C
(CH3)2), 7.21 (dd, 3JHH = 8.8 Hz, 3JHF = 8.8 Hz, 2H, o–F–Ar–H), 7.54 (d, J = 16.2 Hz, 1H, C6H4–C![[H with combining low line]](https://www.rsc.org/images/entities/char_0048_0332.gif)
CH–), 7.59 (br s, 2H, C5–H, C6–H), 7.60 (d, J = 4.0 Hz, 1H, C3–H), 7.66 (d, J = 4.0 Hz, 1H, C2–H), 7.85 (dd, 3JHH = 8.6 Hz, 4JHF = 5.5 Hz, 2H, m–F–Ar–H), 8.12 (d, J = 16.2 Hz, 1H, C6H4–CH
C
–), 8.26 (s, 1H, C8–H) ppm. 13C NMR (500 MHz, (CD3)2CO) δ 13.1, 25.0, 38.9, 113.1, 116.5 (d, 2JCF = 21.8 Hz), 120.8, 126.6, 129.9 (d, 5JCF = 2.4 Hz), 130.0 (d, 3JCF = 8.1 Hz), 133.6, 133.7, 134.8 (d, 4JCF = 3.3 Hz), 135.7, 137.4, 137.7, 138.0, 140.9, 142.4, 163.7 (d, 1JCF = 246.5 Hz) ppm. IR νmax 771, 1215, 1505, 2955 cm−1. HRMS (ESI+): calc. for C22H21F [M + H]+ 305.1700, found 305.1706.
General procedure B for Horner–Wadsworth–Emmons reactions
To the required phosphonate (0.1 mmol, 1.0 eq.) in anhyd. THF (5 mL) was added 1,8-diazabicyclo[5.4.0]undec-7-ene (60 μL, 0.4 mmol, 3.0 eq.) and lithium chloride (18 mg, 0.4 mmol, 3.0 eq.) under nitrogen. The mixture was stirred for 5 min after which a solution of 11 (30 mg, 0.1 mmol, 1.0 eq.) in anhyd. THF (5 mL) was added dropwise. The mixture was stirred at rt until the starting material was consumed as shown by TLC (approx. 3 h). The mixture was then quenched with water (2 mL) and sat. NH4Cl. The mixture was then extracted with EtOAC (3 × 20 mL) and the combined organic fractions were washed with water (30 mL) and brine (30 mL), dried over MgSO4 and filtered, then the filtrate was evaporated under reduced pressure. Purification was achieved by column chromatography to give the desired alkenylazulene.
Methyl (E)-3-(7-isopropyl-1-methylazulen-4-yl)acrylate (20).
General procedure B was used with methyl 2-(diethoxyphosphoryl)acetate (26 mg) to give the product as a green waxy gum (15 mg, 41%) following purification via column chromatography using Petrol/EtOAc (95
:
5; Rf 0.5). 1H NMR (400 MHz, CDCl3) δ 1.39 (d, J = 6.9 Hz, 6H, –CH(C
3)2), 2.69 (s, 3H, C1–CH3), 3.11 (hept, J = 6.8 Hz, 1H, –C
(CH3)2), 3.87 (s, 3H, –OCH3), 6.65 (d, J = 15.9 Hz, 1H, –CH
C
–COOCH3), 7.29 (d, J = 10.8 Hz, 1H, C5–H), 7.46 (d, J = 3.9 Hz, 1H, C3–H), 7.50 (dd, J = 10.8, 2.0 Hz, 1H, C6–H), 7.75 (d, J = 3.3 Hz, 1H, C2–H), 8.21 (d, J = 1.9 Hz, 1H, C8–H), 8.53 (d, J = 15.9 Hz, 1H, –C![[H with combining low line]](https://www.rsc.org/images/entities/char_0048_0332.gif)
CH–COOCH3) ppm. 13C NMR (500 MHz, CDCl3) δ 13.1, 24.8, 38.6, 52.1, 112.6, 120.4, 122.8, 126.6, 133.6, 134.9, 137.3, 137.4, 138.4, 138.5, 141.8, 145.9, 167.3 ppm. IR νmax 1018, 1169, 1273, 1718, 2958 cm−1. HRMS (ESI+): calc. for C18H20O2 [M + H]+ 269.1536, found 269.1535.
(E)-3-(7-Isopropyl-1-methylazulen-4-yl)acrylonitrile (21).
General procedure B was used with diethyl (cyanomethyl)phosphonate (25 mg) to give the product as a dark green waxy gum (10 mg, 33%) following purification via column chromatography using Petrol/EtOAc (80
:
20; Rf 0.6). 1H NMR (500 MHz, CDCl3) δ 1.38 (d, J = 6.9 Hz, 6H, –CH(C
3)2), 2.69 (s, 3H, C1–CH3), 3.12 (hept, J = 6.8 Hz, 1H, –C
(CH3)2), 6.13 (d, J = 16.6 Hz, 1H, –CH
C
–CN), 7.15 (d, J = 10.7 Hz, 1H, C5–H), 7.34 (d, J = 3.8 Hz, 1H, C3–H), 7.50 (dd, J = 10.7, 2.0 Hz, 1H, C6–H), 7.78 (d, J = 3.7 Hz, 1H, C2–H), 8.22 (d, J = 1.9 Hz, 1H, C8–H), 8.28 (d, J = 16.7 Hz, 1H, –C![[H with combining low line]](https://www.rsc.org/images/entities/char_0048_0332.gif)
CH–CN) ppm. 13C NMR (500 MHz, CDCl3) δ 13.1, 24.7, 38.6, 101.3, 112.4, 118.0, 119.4, 127.2, 134.0, 134.8, 136.4, 136.9, 137.9, 139.1, 142.5, 151.9 ppm. IR νmax 721, 787, 918, 976, 1422, 2215, 2959 cm−1. HMS (ESI−): calc. for C17H17N [M − H]− 234.1288, found 234.1290.
(E)-3-(7-Isopropyl-1-methylazulen-4-yl)-N-methoxy-N-methylacrylamide (22).
General procedure A was used with diethyl (2-(methoxy(methyl)amino)-2-oxoethyl)phosphonate (68 mg) to give the product as a light blue waxy gum (28 mg, 50%) following purification via column chromatography using Petrol (100%, Rf 0.3). 1H NMR (500 MHz, (CD3)2CO) δ 1.38 (d, J = 6.9 Hz, 6H, –CH(C
3)2), 2.67 (s, 3H, C1–CH3), 3.16 (hept, J = 6.9 Hz, 1H, –C
(CH3)2), 3.30 (s, 3H, CH3), 3.84 (s, 3H, CH3), 7.36 (d, J = 15.7 Hz, 1H, –CH
C
–COO–), 7.46 (d, J = 3.9 Hz, 1H, C3–H), 7.50 (d, J = 10.9 Hz, 1H, C5–H), 7.61 (dd, J = 10.9, 1.9 Hz, 1H, C6–H), 7.74 (d, J = 3.9 Hz, 1H, C2–H), 8.30 (d, J = 1.9 Hz, 1H, C8–H), 8.51 (d, J = 15.7 Hz, 1H, –C![[H with combining low line]](https://www.rsc.org/images/entities/char_0048_0332.gif)
CH–COO–) ppm. 13C NMR (500 MHz, (CD3)2CO) δ 13.0, 24.9, 32.6 (br), 39.0, 62.4, 113.0, 121.2, 122.5, 127.1, 134.2, 135.7, 138.0, 138.4, 138.8, 140.1, 142.2, 143.7, 166.7 (br) ppm. IR νmax 977, 1001, 1378, 1414, 1652, 2958 cm−1. HRMS (ESI+): calc. for C19H23NO2 [M + H]+ 298.1810, found 298.1812.
(Z)-N-tert-Butyl-1-(7-isopropyl-1-methylazulen-4-yl)methanimine oxide (23).
To aldehyde 11 (70 mg, 0.3 mmol, 1.0 eq.) was added N-tert-butylhydroxylamine hydrochloride (62 mg, 0.5 mmol, 1.5 eq.) and pyridine (5 mL) under nitrogen. The mixture was stirred at 95 °C until all the starting material was consumed by TLC (3 h). After cooling to rt, the pyridine was evaporated under reduced pressure. The mixture was diluted with water (10 mL) and extracted with EtOAc (3 × 30 mL) and the combined organic fractions were washed with brine and dried over MgSO4 and filtered. The filtrate was evaporated under reduced pressure to yield a turquoise/green waxy gum (64 mg, 69%). 1H NMR (500 MHz, (CDCl3) δ 1.38 (d, J = 6.9 Hz, 6H, –CH(C
3)2), 1.71 (s, 9H, C(CH3)3), 2.68 (s, 3H, C1–CH3), 3.12 (hept, J = 6.9 Hz, 1H, –C
(CH3)2), 7.18 (d, J = 3.7 Hz, 1H, C3–H), 7.57 (dd, J = 11.0, 2.0 Hz, 1H, C6–H), 7.67 (d, J = 3.7 Hz, 1H, C2–H), 8.21 (d, J = 1.8 Hz, 1H, C8–H), 8.37 (s, 1H, –CH
N), 8.85 (d, J = 11.0 Hz, C5–H) ppm. 13C NMR (500 MHz, (CDCl3) δ 13.1, 24.7, 28.6, 38.7, 72.6, 109.7, 121.5, 125.4, 129.5, 133.5, 134.0, 135.3, 137.1, 137.4, 138.4, 143.3 ppm. IR νmax 766, 1131, 1361, 1537, 2958 cm−1. HRMS (ESI+): calc. for C19H25NO [M + H]+ 284.2009, found 284.2019.
Conflicts of interest
There are no conflicts to declare.
Acknowledgements
We thank the University of Bath for funding. TDJ wishes to thank the Royal Society for a Wolfson Research Merit Award and the Open Research Fund of School of Chemistry and Chemical Engineering, Henan Normal University for support (2020ZD01).
Notes and references
- R. S. H. Liu, J. Chem. Educ., 2002, 79, 183 CrossRef CAS
.
-
(a) M. Beer and H. C. Longuet-Higgins, J. Chem. Phys., 1955, 23, 1390 CrossRef CAS
;
(b) B. D. Wagner, D. Tittelbach-Helmrich and R. P. Steer, J. Phys. Chem., 1992, 96, 7904 CrossRef CAS
;
(c) K. Veys and D. Escudero, J. Phys. Chem. A, 2020, 124, 7228 CrossRef CAS PubMed
.
-
(a) T. Shoji, S. Sugiyama, Y. Kobayashi, A. Yamazaki, Y. Ariga, R. Katoh, H. Wakui, M. Yasunami and S. Ito, Chem. Commun., 2020, 56, 1485 RSC
;
(b) P. Dunås, L. C. Murfin, O. J. Nilsson, N. Jame, S. E. Lewis and N. Kann, J. Org. Chem., 2020, 85, 13453 CrossRef PubMed
;
(c) K. Ohtsu, R. Hayami, T. Sagawa, S. Tsukada, K. Yamamoto and T. Gunji, Tetrahedron, 2019, 75, 130658 CrossRef CAS
;
(d) S. J. Webster, C. M. López-Alled, X. Liang, C. L. McMullin, G. Kociok-Köhn, C. L. Lyall, T. D. James, J. Wenk, P. J. Cameron and S. E. Lewis, New J. Chem., 2019, 43, 992 RSC
;
(e) H. L. Gao, X. D. Yang, H. S. Xin, T. Z. Gao, H. G. Gong and X. K. Gao, Chin. J. Org. Chem., 2018, 38, 2680 CrossRef CAS
;
(f) T. Tang, T. T. Lin, F. Erden, F. Wang and C. B. He, J. Mater. Chem. C, 2018, 6, 5153 RSC
;
(g) L. Z. Gai, J. Z. Chen, Y. Zhao, J. Mack, H. Lu and Z. Shen, RSC Adv., 2016, 6, 32124 RSC
;
(h) E. H. Ghazvini Zadeh, S. Tang, A. W. Woodward, T. Liu, M. V. Bondar and K. D. Belfield, J. Mater. Chem. C, 2015, 3, 8495 RSC
;
(i) M. Murai, K. Takami, H. Takeshima and K. Takai, Org. Lett., 2015, 17, 1798 CrossRef CAS
;
(j) E. Amir, M. Murai, R. J. Amir, J. S. Cowart, M. L. Chabinyc and C. J. Hawker, Chem. Sci., 2014, 5, 4483 RSC
;
(k) M. Murai, S. Y. Ku, N. D. Treat, M. J. Robb, M. L. Chabinyc and C. J. Hawker, Chem. Sci., 2014, 5, 3753 RSC
;
(l) M. Koch, O. Blacque and K. Venkatesan, J. Mater. Chem. C, 2013, 1, 7400 RSC
;
(m) M. Koch, O. Blacque and K. Venkatesan, Org. Lett., 2012, 14, 1580 CrossRef CAS PubMed
;
(n) E. Amir, R. J. Amir, L. M. Campos and C. J. Hawker, J. Am. Chem. Soc., 2011, 133, 10046 CrossRef CAS PubMed
.
-
(a) T. Shoji and S. Ito, Chem. – Eur. J., 2017, 23, 16696 CrossRef CAS PubMed
;
(b) T. Shoji, M. Maruyama, A. Maruyama, S. Ito, T. Okujima and K. Toyota, Chem. – Eur. J., 2014, 20, 11903 CrossRef CAS PubMed
;
(c) T. Shoji, A. Yamamoto, Y. Inoue, E. Shimomura, S. Ito, J. Higashi and N. Morita, Chem. Lett., 2012, 41, 1644 CrossRef CAS
;
(d) A. C. Razus, L. Birzan, N. M. Surugiu, A. C. Corbu and F. Chiraleu, Dyes Pigm., 2007, 74, 26 CrossRef CAS
;
(e) A. Blice-Baum, A. Van Dyke, I. Sigmon, E. A. Salter, A. Wierzbicki, Y. Pocker and G. T. Spyridis, Int. J. Quantum Chem., 2006, 106, 2331 CrossRef CAS
;
(f) L. Cristian, I. Sasaki, P. G. Lacroix, B. Donnadieu, I. Asselberghs, K. Clays and A. C. Razus, Chem. Mater., 2004, 16, 3543 CrossRef CAS
;
(g) A. C. Razus, L. Birzan, S. Nae, L. Cristian, F. Chiraleu and V. Cimpeanu, Dyes Pigm., 2003, 57, 223 CrossRef CAS
;
(h) S. Ito, S. Kikuchi, H. Kobayashi, N. Morita and T. Asao, J. Org. Chem., 1997, 62, 2423 CrossRef CAS PubMed
.
-
(a) M. Okamoto, S. Hirayama and R. P. Steer, Can. J. Chem., 2007, 85, 432 CrossRef CAS
;
(b) S. Hirayama and R. P. Steer, J. Chem. Educ., 2008, 85, 317 CrossRef CAS
.
- A. G. Anderson Jr. and B. M. Steckler, J. Am. Chem. Soc., 1959, 81, 4941 CrossRef
.
-
(a) H. Xin, J. Li, X. Yang and X. Gao, J. Org. Chem., 2020, 85, 70 CrossRef CAS PubMed
;
(b) L. C. Murfin, C. M. López-Alled, A. C. Segwick, J. Wenk, T. D. James and S. E. Lewis, Front. Chem. Sci. Eng., 2020, 14, 90 CrossRef CAS
;
(c) C. M. López-Alled, L. C. Murfin, G. Kociok-Köhn, T. D. James, J. Wenk and S. E. Lewis, Analyst, 2020, 145, 6262 RSC
;
(d) L. C. Murfin, K. Chiang, G. T. Williams, C. L. Lyall, A. T. A. Jenkins, J. Wenk, T. D. James and S. E. Lewis, Front. Chem., 2020, 8, 10 CrossRef CAS PubMed
;
(e) H. Gao, C. Ge, B. Hou, H. Xin and X. Gao, ACS Macro Lett., 2019, 8, 1360 CrossRef CAS
;
(f) H. Xin, J. Li, X. Yang and X. Gao, J. Org. Chem., 2019, 85, 70 CrossRef PubMed
;
(g) D. Lichosyt, S. Wasiłek, P. Dydio and J. Jurczak, Chem. Eur. J., 2018, 24, 11683 CrossRef CAS PubMed
;
(h) G.-O. Buica, I.-G. Lazar, L. Birzan, C. Lete, M. Prodana, M. Enachescu, V. Tecuceanu, A. B. Stoian and E.-M. Ungureanu, Electrochim. Acta, 2018, 263, 382 CrossRef CAS
;
(i) C. M. López-Alled, A. Sanchez-Fernandez, K. J. Edler, A. C. Sedgwick, S. D. Bull, C. L. McMullin, G. Kociok-Köhn, T. D. James, J. Wenk and S. E. Lewis, Chem. Commun., 2017, 53, 12580 RSC
;
(j) L. Birzan, M. Cristea, C. C. Draghici, V. Tecuceanu, M. Maganu, A. Hanganu, G.-L. Arnold, E.-M. Ungureanu and A. C. Razus, Tetrahedron, 2016, 72, 2316 CrossRef CAS
;
(k) S. Wakabayashi, M. Uchida, R. Tanaka, Y. Habata and M. Shimizu, Asian J. Org. Chem., 2013, 2, 786 CrossRef CAS
.
-
(a) L. C. Murfin and S. E. Lewis, Molecules, 2021, 26, 353 CrossRef CAS PubMed
;
(b) L. C. Murfin, M. Weber, S. J. Park, W. T. Kim, C. M. Lopez-Alled, C. L. McMullin, F. Pradaux-Caggiano, C. L. Lyall, G. Kociok-Köhn, J. Wenk, S. D. Bull, J. Yoon, H. M. Kim, T. D. James and S. E. Lewis, J. Am. Chem. Soc., 2019, 141, 19389 CrossRef CAS PubMed
;
(c) Y. Zhou, G. Baryshnikov, X. Li, M. Zhu, H. Ågren and L. Zhu, Chem. Mater., 2018, 30, 8008 CrossRef CAS
;
(d) P. M. Gosavi, Y. S. Moroz and I. V. Korendovych, Chem. Commun., 2015, 51, 5347 RSC
;
(e) Y. S. Moroz, W. Binder, P. Nygren, G. A. Caputo and I. V. Korendovych, Chem. Commun., 2013, 49, 490 RSC
;
(f) H. Salman, Y. Abraham, S. Tal, S. Meltzman, M. Kapon, N. Tessler, S. Speiser and Y. Eichen, Eur. J. Org. Chem., 2005, 2207 CrossRef CAS
;
(g) C. Mazzuca, L. Stella, M. Venanzi, F. Formaggio, C. Toniolo and B. Pispisa, Biophys. J., 2005, 88, 3411 CrossRef CAS PubMed
;
(h) G. Loidl, H.-J. Musiol, N. Budisa, R. Huber, S. Poirot, D. Fourmy and L. Moroder, J. Pept. Sci., 2000, 6, 139 CrossRef CAS PubMed
.
-
(a) L. Yang, Y. Zhu, J. Liu, Y. Chen, J. Wu, Z. Pang, Z. Lu, S. Zhao and Y. Huang, Dyes Pigm., 2021, 187, 109079 CrossRef CAS
;
(b) P. Cowper, A. Pockett, G. Kociok-Köhn, P. J. Cameron and S. E. Lewis, Tetrahedron, 2018, 74, 2775 CrossRef CAS
;
(c) H. Xin, C. Ge, X. Jiao, X. Yang, K. Rundel, C. R. McNeill and X. Gao, Angew. Chem., Int. Ed., 2018, 57, 1322 CrossRef CAS PubMed
;
(d) E. Puodziukynaite, H.-W. Wang, J. Lawrence, A. J. Wise, T. P. Russell, M. D. Barnes and T. Emrick, J. Am. Chem. Soc., 2014, 136, 11043 CrossRef CAS PubMed
;
(e) K. Kakiage, E. Fujimura, Y. Nakada, T. Ogino, T. Kyomen and M. Hanaya, Key Eng. Mater., 2014, 596, 35 CAS
;
(f) X.-H. Zhang, C. Li, W.-B. Wang, X.-X. Cheng, X.-S. Wang and B.-W. Zhang, J. Mater. Chem., 2007, 17, 642 RSC
.
-
(a) B. Hou, J. Li, H. Xin, X. Yang, H. Gao, P. Peng and X. Gao, Acta Chim. Sin., 2020, 78, 788 CrossRef
;
(b) Y. Shibuya, K. Aonuma, T. Kimura, T. Kaneko, W. Fujiwara, Y. Yamaguchi, D. Kumaki, S. Tokito and H. Katagiri, J. Phys. Chem. C, 2020, 124, 4738 CrossRef CAS
;
(c) H. Xin, J. Li, R.-Q. Lu, X. Gao and T. M. Swager, J. Am. Chem. Soc., 2020, 142, 13598 CrossRef CAS PubMed
;
(d) H. Gao, C. Ge, B. Hou, H. Xin and X. Gao, ACS Macro Lett., 2019, 8, 1360 CrossRef CAS
;
(e) H. Xin, J. Li, C. Ge, X. Yang, T. Xue and X. Gao, Mater. Chem. Front., 2018, 2, 975 RSC
;
(f) H. Xin, C. Ge, L. Fu, X. Yang and X. Gao, Chin. J. Org. Chem., 2017, 37, 711 CrossRef CAS
;
(g) H. Xin and X. Gao, ChemPlusChem, 2017, 82, 945 CrossRef CAS PubMed
;
(h) Y. Yamaguchi, M. Takubo, K. Ogawa, K.-i. Nakayama, T. Koganezawa and H. Katagiri, J. Am. Chem. Soc., 2016, 138, 11335 CrossRef CAS PubMed
;
(i) H. Xin, C. Ge, X. Yang, H. Gao, X. Yang and X. Gao, Chem. Sci., 2016, 7, 6701 RSC
;
(j) H. Nishimura, N. Ishida, A. Shimazaki, A. Wakamiya, A. Saeki, L. T. Scott and Y. Murata, J. Am. Chem. Soc., 2015, 137, 15656 CrossRef CAS PubMed
;
(k) J. Yao, Z. Cai, Z. Liu, C. Yu, H. Luo, Y. Yang, S. Yang, G. Zhang and D. Zhang, Macromolecules, 2015, 48, 2039 CrossRef CAS
;
(l) J. Xia, B. Capozzi, S. Wei, M. Strange, A. Batra, J. R. Moreno, R. J. Amir, E. Amir, G. C. Solomon, L. Venkataraman and L. M. Campos, Nano Lett., 2014, 14, 2941 CrossRef CAS PubMed
;
(m) Y. Yamaguchi, K. Ogawa, K. Nakayama, Y. Ohba and H. Katagiri, J. Am. Chem. Soc., 2013, 135, 19095 CrossRef CAS PubMed
;
(n) Y. Yamaguchi, Y. Maruya, H. Katagiri, K.-i. Nakayama and Y. Ohba, Org. Lett., 2012, 14, 2316 CrossRef CAS PubMed
.
-
(a) A. Turku, T. O. Leino, L. Karhu, J. Yli-Kauhaluoma, J. P. Kukkonen, E. A. A. Wallén and H. Xhaard, ChemMedChem, 2019, 14, 965 CrossRef CAS PubMed
;
(b) T. Wada, R. Maruyama, Y. Irie, M. Hashimoto, H. Wakabayashi, N. Okudaira, Y. Uesawa, H. Kagaya and H. Sakagami, In Vivo, 2018, 32, 479 CAS
;
(c) T. O. Leino, A. Turku, J. Yli-Kauhaluoma, J. P. Kukkonen, H. Xhaard and E. A. A. Wallén, Eur. J. Med. Chem., 2018, 157, 88 CrossRef CAS PubMed
;
(d) E. Stempel, R. F.-X. Kaml, N. Budisa and M. Kalesse, Bioorg. Med. Chem., 2018, 26, 5259 CrossRef CAS PubMed
;
(e) J. Peet, A. Selyutina and A. Bredihhin, Bioorg. Med. Chem., 2016, 24, 1653 CrossRef CAS PubMed
;
(f) K. Ikegai, M. Imamura, T. Suzuki, K. Nakanishi, T. Murakami, E. Kurosaki, A. Noda, Y. Kobayashi, M. Yokota, T. Koide, K. Kosakai, Y. Okhura, M. Takeuchi, H. Tomiyama and M. Ohta, Bioorg. Med. Chem., 2013, 21, 3934 CrossRef CAS PubMed
;
(g) S. Löber, H. Hübner, A. Buschauer, F. Sanna, A. Argiolas, M. R. Melis and P. Gmeiner, Bioorg. Med. Chem. Lett., 2012, 22, 7151 CrossRef PubMed
;
(h) C. Chen, O. Lee, C. Yao, M. Chuang, Y. Chang, M. Chang, Y. Wen, W. Yang, C. Ko, N. Chou, M. Lin, C. Lai, C. Sun, L. Wang, Y. Chen, T. Hseu, C. Chang, H. Hsu, H. Lin, Y. Chang, Y. Shih, S. Chou, Y. Hsu, H. Tseng, C. Liu, C. Tu, T. Hu, Y. Tsai, T. Chen, C. Lin, S. Chiou, C. Liu and C. Hwang, Bioorg. Med. Chem. Lett., 2010, 20, 6129 CrossRef CAS PubMed
;
(i) Y. Tanaka and K. Shigenobu, Cardiovasc. Drug Rev., 2001, 19, 297 CrossRef CAS PubMed
;
(j) A. E. Asato, A. Peng, M. Z. Hossain, T. Mirzadegan and J. S. Bertram, J. Med. Chem., 1993, 36, 3137 CrossRef CAS PubMed
;
(k) T. Tomiyama, M. Yokota, S. Wakabayashi, K. Kosakai and T. Yanagisawa, J. Med. Chem., 1993, 36, 791 CrossRef CAS PubMed
;
(l) T. Yanagisawa, S. Wakabayashi, T. Tomiyama, M. Yasunami and K. Takase, Chem. Pharm. Bull., 1988, 36, 641 CrossRef CAS PubMed
.
- For recent reviews, see:
(a) A. Konishi and M. Yasuda, Chem. Lett., 2021, 50, 195 CrossRef CAS
;
(b) T. Shoji, T. Okujima and S. Ito, Int. J. Mol. Sci., 2020, 21, 7087 CrossRef CAS PubMed
;
(c) H. N. Zeng, Z. M. Png and J. Xu, Chem. – Eur. J., 2020, 15, 1904 CAS
;
(d) A. C. Razus and L. Birzan, Monatsh. Chem., 2019, 150, 139 CrossRef CAS
;
(e) T. Shoji and S. Ito, Adv. Heterocycl. Chem., 2018, 126, 1 CrossRef CAS
.
-
(a) J. Mogensen, O. Christensen, M. D. Kilde, M. Abildgaard, L. Metz, A. Kadziola, M. Jevric, K. V. Mikkelsen and M. B. Nielsen, Eur. J. Org. Chem., 2019, 1986 CrossRef CAS
;
(b) M. D. Kilde, M. H. Hansen, S. L. Broman, K. V. Mikkelsen and M. B. Nielsen, Eur. J. Org. Chem., 2017, 1052 CrossRef CAS
;
(c) A. U. Petersen, M. Jevric, R. J. Mandle, M. T. Sims, J. N. Moore, S. J. Cowling, J. W. Goodby and M. B. Nielsen, Chem. – Eur. J., 2017, 23, 5090 CrossRef CAS PubMed
;
(d) A. U. Petersen, M. Jevric, J. Elm, S. T. Olsen, C. G. Tortzen, A. Kadziola, K. V. Mikkelsen and M. B. Nielsen, Org. Biomol. Chem., 2016, 14, 2403 RSC
;
(e) A. Vlasceanu, C. L. Andersen, C. R. Parker, O. Hammerich, T. J. Morsing, M. Jevric, S. L. Broman, A. Kadziola and M. B. Nielsen, Chem. – Eur. J., 2016, 22, 7514 CrossRef CAS PubMed
;
(f) A. B. Skov, S. L. Broman, A. S. Gertsen, J. Elm, M. Jevric, M. Cacciarini, A. Kadziola, K. V. Mikkelsen and M. B. Nielsen, Chem. – Eur. J., 2016, 22, 14567 CrossRef CAS PubMed
;
(g) M. D. Kilde, S. L. Broman, A. Kadziola and M. B. Nielsen, Synlett, 2016, 450 CAS
;
(h) S. L. Broman, O. Kushnir, M. Rosenberg, A. Kadziola, J. Daub and M. B. Nielsen, Eur. J. Org.
Chem., 2015, 4119 CrossRef
;
(i) S. L. Broman, M. Jevric, A. D. Bond and M. B. Nielsen, J. Org. Chem., 2014, 79, 41 CrossRef CAS PubMed
;
(j) M. Jevric, S. L. Broman and M. B. Nielsen, J. Org. Chem., 2013, 78, 4348 CrossRef CAS PubMed
;
(k) S. L. Broman, S. Lara-Avila, C. L. Thisted, A. D. Bond, S. Kubatkin, A. Danilov and M. B. Nielsen, Adv. Funct. Mater., 2012, 22, 4249 CrossRef CAS
;
(l) V. Mazzanti, M. Cacciarini, S. L. Broman, C. R. Parker, M. Schau-Magnussen, A. D. Bond and M. B. Nielsen, Beilstein J. Org. Chem., 2012, 8, 958 CrossRef CAS PubMed
;
(m) M. Å. Petersen, A. S. Andersson, K. Kilså and M. B. Nielsen, Eur. J. Org. Chem., 2009, 1855 CrossRef CAS
.
-
(a) A. L. Crombie, J. L. Kane, K. M. Shea and R. L. Danheiser, J. Org. Chem., 2004, 69, 8652 CrossRef CAS PubMed
;
(b) J. L. Kane, K. M. Shea, A. L. Crombie and R. L. Danheiser, Org. Lett., 2001, 3, 1081 CrossRef CAS PubMed
.
-
(a) A. G. Anderson Jr., J. A. Nelson and J. J. Tazuma, J. Am. Chem. Soc., 1953, 75, 4980 CrossRef
;
(b) A. G. Anderson Jr., R. Scotoni Jr., E. J. Cowles and C. G. Fritz, J. Org. Chem., 1957, 22, 1193 CrossRef
;
(c) A. G. Anderson Jr., R. G. Anderson and L. L. Replogle, Proc. Chem. Soc., 1960, 72 Search PubMed
;
(d) A. G. Anderson Jr. and R. G. Anderson, J. Org. Chem., 1962, 27, 3578 CrossRef
;
(e) L. J. Mathias and C. G. Overberger, J. Org. Chem., 1980, 45, 1701 CrossRef CAS
.
-
(a) P. Cowper, Y. Jin, M. D. Turton, G. Kociok-Köhn and S. E. Lewis, Angew. Chem., Int. Ed., 2016, 55, 2564 CrossRef CAS PubMed
;
(b) J. Dubovik and A. Bredihhin, Synthesis, 2015, 538 CAS
;
(c) J. Dubovik and A. Bredihhin, Synthesis, 2015, 2663 CAS
;
(d) S. Ito, T. Shoji and N. Morita, Synlett, 2011, 2279 CrossRef CAS
;
(e) T. Shoji, S. Ito, K. Toyota, T. Iwamoto, M. Yasunami and N. Morita, Eur. J. Org. Chem., 2009, 4307 CrossRef CAS
;
(f) M. Fujinaga, K. Suetake, K. Gyoji, T. Murafuji, K. Kurotobi and Y. Sugihara, Synthesis, 2008, 3745 CAS
;
(g) T. Shibasaki, T. Ooishi, N. Yamanouchi, T. Murafuji, K. Kurotobi and Y. Sugihara, J. Org. Chem., 2008, 73, 7971 CrossRef CAS PubMed
;
(h) S. Ito, T. Terazono, T. Kubo, T. Okujima, N. Morita, T. Murafuji, Y. Sugihara, K. Fujimori, J. Kawakami and A. Tajiri, Tetrahedron, 2004, 60, 5357 CrossRef CAS
.
-
(a) S. C. Lee, J.-Y. Son, J. Y. Kim, H. Eom, S. B. Jang and P. H. Lee, Adv. Synth. Catal., 2021, 363, 512 CrossRef
;
(b) Z. M. Png, T. L. D. Tam and J. Xu, Org. Lett., 2020, 22, 5009 CrossRef CAS PubMed
;
(c) Z. M. Png and J. Xu, Chem. – Asian J., 2020, 15, 2505 CrossRef PubMed
;
(d) B. Pigulski, K. Shoyama and F. Würthner, Angew. Chem., 2020, 59, 15908 CrossRef CAS PubMed
;
(e) C. Maeng, H. J. Seo, H. Jeong, K. Lee, H. C. Noh and P. H. Lee, Org. Lett., 2020, 22, 7267 CrossRef CAS PubMed
;
(f) C. Maeng, J.-Y. Son, S. C. Lee, Y. Baek, K. Um, S. H. Han, G. H. Ko, G. U. Han, K. Lee, K. Lee and P. H. Lee, J. Org. Chem., 2020, 85, 3824 CrossRef CAS PubMed
;
(g) G. U. Han, J.-Y. Son, D. Park, H. Eom, K. Lee, H. C. Noh, K. Lee and P. H. Lee, Adv. Synth. Catal., 2020, 362, 4749 CrossRef CAS
;
(h) X. Shi, A. Sasmal, J.-F. Soulé and H. Doucet, Chem. – Asian J., 2018, 13, 143 CrossRef CAS PubMed
;
(i) J. Carreras, Y. Popowski, A. Caballero, E. Amir and P. J. Perez, J. Org. Chem., 2018, 83, 11125 CrossRef CAS PubMed
;
(j) A. Székely, A. Péter, K. Aradi, G. L. Tolnai and Z. Novák, Org. Lett., 2017, 19, 954 CrossRef PubMed
;
(k) M. Murai, S. Iba, H. Ota and K. Takai, Org. Lett., 2017, 19, 5585 CrossRef CAS PubMed
;
(l) M. Murai, M. Yanagawa, M. Nakamura and K. Takai, Asian J. Org. Chem., 2016, 5, 629 CrossRef CAS
;
(m) M. Murai, K. Takami, H. Takeshima and K. Takai, Org. Lett., 2015, 17, 1798 CrossRef CAS PubMed
;
(n) L. Zhao, C. Bruneau and H. Doucet, Chem. Commun., 2013, 49, 5598 RSC
;
(o) M. Fujinaga, T. Murafuji, K. Kurotobi and Y. Sugihara, Tetrahedron, 2009, 65, 7115 CrossRef CAS
;
(p) J. Liu, E. Muth, U. Floerke, G. Henkel, K. Merz, J. Sauvageau, E. Schwake and G. Dyker, Adv. Synth. Catal., 2006, 348, 456 CrossRef CAS
;
(q) K. Kurotobi, M. Miyauchi, K. Takakura, T. Murafuji and Y. Sugihara, Eur. J. Org. Chem., 2003, 3663 CrossRef CAS
;
(r) G. Dyker, S. Borowski, J. Heiermann, J. Körning, K. Opwis, G. Henkel and M. Köckerling, J. Organomet. Chem., 2000, 606, 108 CrossRef CAS
.
-
(a) M. Makosza, P. W. Osinski and S. Ostrowski, Pol. J. Chem., 2001, 75, 275 CAS
;
(b) M. Makosza and R. Podraza, Eur. J. Org. Chem., 2000, 193 CrossRef CAS
.
-
(a) T. Morita, N. Abe and K. Takase, J. Chem. Soc., Perkin Trans. 1, 2000, 3063 RSC
;
(b) R. N. McDonald, H. E. Petty, N. L. Wolfe and J. V. Paukstelis, J. Org. Chem., 1974, 39, 1877 CrossRef CAS
;
(c) K. Hafner and H. Weldes, Liebigs Ann., 1957, 606, 90 CrossRef CAS
.
-
(a) D. Copland, D. Leaver and W. B. Menzies, Tetrahedron Lett., 1977, 18, 639 CrossRef
;
(b) S. E. Reiter, L. C. Dunn and K. N. Houk, J. Am. Chem. Soc., 1977, 99, 4199 CrossRef CAS
;
(c) L. T. Scott, M. A. Minton and M. A. Kirms, J. Am. Chem. Soc., 1980, 102, 6311 CrossRef CAS
;
(d) H. Langhals and M. Eberspächer, Synthesis, 2018, 1862 CrossRef CAS
.
- See: D. Chen, S. Yu, L. van Ofwegen, P. Proksch and W. Lin, J. Agric. Food Chem., 2012, 60, 112 CrossRef CAS
and references therein.
-
G. Li, Chin. Pat, CN109096038A, 2018 Search PubMed
.
-
Y. Zhou, Chin. Pat, CN105753623A, 2016 Search PubMed
.
-
F. Xu, Chin. Pat, CN108863707A, 2018 Search PubMed
.
- See: T. Yanagisawa, K. Kosakai, T. Tomiyama, M. Yasunami and K. Takase, Chem. Pharm. Bull., 1990, 38, 3355 CrossRef CAS PubMed
and references therein.
- Y. Inoue, I. Shinohara, I. Murata and I. Kanamoto, J. Mol. Struct., 2019, 1186, 50 CrossRef CAS
.
- Y. Matsubara, H. Yamamoto and T. Nozoe, Stud. Nat. Prod. Chem., 1994, 14, 313 CAS
.
-
(a) T. Nozoe, S. Ishikawa and K. Shindo, Chem. Lett., 1989, 18, 353 CrossRef
;
(b) Z. Zhao, Y. Matsubara and T. Nozoe, Nippon Kagaku Kaishi, 1994, 49, DOI:10.1246/nikkashi.1994.49
.
-
(a) J. Richter, P. Liebing and F. T. Edelmann, Inorg. Chim. Acta, 2018, 475, 18 CrossRef CAS
;
(b) A. Kiriazis, I. B. Aumüller, R. Arnaudova, V. Brito, T. Rüffer, H. Lang, S. M. Silvestre, P. J. Koskinen and J. Yli-Kauhaluoma, Org. Lett., 2017, 19, 2030 CrossRef CAS PubMed
;
(c) A. W. Woodward, E. H. Ghazvini Zadeh, M. V. Bondar and K. D. Belfield, R. Soc. Open Sci., 2016, 3, 160373 CrossRef PubMed
;
(d) O. Sato, T. Saito, M. Iwase and A. Sakai, Heterocycles, 2016, 93, 714 CrossRef CAS
;
(e) L. Birzan, M. Cristea, C. C. Draghici, V. Tecuceanu, M. Maganu, A. Hanganu, G.-L. Arnold, E.-M. Ungureanu and A. C. Razus, Tetrahedron, 2016, 72, 2316 CrossRef CAS
;
(f) I. B. Aumüller and J. Yli-Kauhaluoma, Org. Lett., 2011, 13, 1670 CrossRef
;
(g) A. Kiriazis, I. B. Aumüller and J. Yli-Kauhaluoma, Tetrahedron Lett., 2011, 52, 1151 CrossRef CAS
;
(h) R. Sigrist and H.-J. Hansen, Helv. Chim. Acta, 2010, 93, 1545 CrossRef CAS
;
(i) I. B. Aumüller and T. K. Lindhorst, J. Carbohydr. Chem., 2009, 28, 330 CrossRef
;
(j) A. C. Razus, L. Birzan, V. Tecuceanu, M. Cristea and C. Enache, ARKIVOC, 2008, 210 Search PubMed
;
(k) I. B. Aumüller and T. K. Lindhorst, Eur. J. Org. Chem., 2006, 1103 CrossRef
;
(l) S. Balduzzi, H. Muller-Bunz and M. A. McGlinchey, Chem. – Eur. J., 2004, 10, 5398 CrossRef CAS PubMed
;
(m) J. Song and H.-J. Hansen, Helv. Chim. Acta, 1999, 82, 309 CrossRef CAS
;
(n) A. A. S. Briquet and H.-J. Hansen, Helv. Chim. Acta, 1994, 77, 1921 CrossRef CAS
;
(o) S. Kurokawa, Nippon Kagaku Kaishi, 1997, 365, DOI:10.1246/nikkashi.1997.365
;
(p) S. Kurokawa, Nippon Kagaku Kaishi, 1995, 39, DOI:10.1246/nikkashi.1995.39
;
(q) S. Kurokawa, Bull. Chem. Soc. Jpn., 1983, 56, 2311 CrossRef CAS
;
(r) M. Scholz, L. N. Vien, G. Fischer, B. Tschapke and M. Mühlstädt, Chem. Ber., 1967, 100, 375 CrossRef CAS
.
-
(a) R. F. Abdulla and R. S. Brinkmeyer, Tetrahedron, 1979, 35, 1675 CrossRef CAS
;
(b) F. A. Abu-Shanab, S. M. Sherif and S. A. S. Mousa, J. Heterocycl. Chem., 2009, 46, 801 CrossRef CAS
;
(c) S. M. Riyadh, I. A. Abdelhamid, H. M. Al-Matar, N. M. Hilmy and M. H. Elnagdi, Heterocycles, 2008, 75, 1849 CrossRef CAS
.
- H. Meerwein, W. Florian, N. Schön and G. Stopp, Liebigs Ann., 1961, 641, 1 CrossRef CAS
.
-
(a) H. Bredereck and G. Simchen, Angew. Chem., Int. Ed. Engl., 1963, 2, 738 CrossRef
;
(b) H. Bredereck, G. Simchen and R. Wahl, Chem. Ber., 1968, 101, 4048 CrossRef CAS
.
- M. G. Vetelino and J. W. Coe, Tetrahedron Lett., 1994, 35, 219 CrossRef
.
- K. Kurotobi, K. S. Kim, S. B. Noh, D. Kim and A. Osuka, Angew. Chem., Int. Ed., 2006, 45, 3944 CrossRef CAS PubMed
.
- T. O. Leino, M. Baumann, J. Yli-Kauhaluoma, I. R. Baxendale and E. A. A. Wallén, J. Org. Chem., 2015, 80, 11513 CrossRef CAS PubMed
.
- T. Shoji, T. Araki, N. Iida, Y. Kobayashi, A. Ohta, R. Sekiguchi, S. Ito, S. Mori, T. Okujima and M. Yasunami, Eur. J. Org. Chem., 2018, 1145 CrossRef CAS
.
-
(a) L. Horner, H. Hoffmann and H. G. Wippel, Chem. Ber., 1958, 91, 61 CrossRef CAS
;
(b) W. S. Wadsworth Jr. and W. D. Emmons, J. Am. Chem. Soc., 1961, 83, 1733 CrossRef
.
- M. A. Blanchette, W. Choy, J. T. Davis, A. P. Essenfeld, S. Masamune, W. R. Roush and T. Sakai, Tetrahedron Lett., 1984, 25, 2183 CrossRef CAS
.
-
(a) S. Itoh, N. Morita, R. Miyatake and S. Kuroda, Chem. Lett., 1997, 1011 Search PubMed
;
(b) M. Oda, A. Sakamoto, T. Uchiyama, T. Kajioka, R. Miyatake and S. Kuroda, Tetrahedron Lett., 1999, 40, 3595 CrossRef CAS
;
(c) M. Oda, H. Kainuma, T. Uchiyama, R. Miyatake and S. Kuroda, Tetrahedron, 2003, 59, 2831 CrossRef CAS
;
(d) M. Oda, N. Nakajima, N. C. Thanh, T. Kajioka and S. Kuroda, Tetrahedron, 2006, 62, 8177 CrossRef CAS
;
(e) M. Oda, N. Nakajima, N. C. Thanh, K. Kitahara, R. Miyatake and S. Kuroda, Eur. J. Org. Chem., 2008, 5301 CrossRef CAS
.
-
(a) M. Rosselin, B. Poeggeler and G. Durand, Curr. Top. Med. Chem., 2017, 17, 2006 CrossRef CAS PubMed
;
(b) D. A. Becker, J. J. Ley, L. Echegoyen and R. Alvarado, J. Am. Chem. Soc., 2002, 124, 4678 CrossRef CAS PubMed
;
(c) D. A. Becker, J. Am. Chem. Soc., 1996, 118, 905 CrossRef CAS
.
-
(a) K. Satoh, M. Yamaguchi and I. Ogura, Bunseki Kagaku, 1983, 32, 61, DOI:10.2116/bunsekikagaku.32.61
;
(b) K. Satoh, M. Yamaguchi and I. Ogura, Nippon Kagaku Kaishi, 1982, 1982, 1199, DOI:10.1246/nikkashi.1982.1199
.
Footnotes |
† Electronic supplementary information (ESI) available. CCDC 2047319. For ESI and crystallographic data in CIF or other electronic format see DOI: 10.1039/d0ob02567d |
‡ Although 10 has not been reported previously, various substituted analogues have been described, having been formed under Vilsmeier conditions – see ref. 41. |
|
This journal is © The Royal Society of Chemistry 2021 |