DOI:
10.1039/D0MA00681E
(Review Article)
Mater. Adv., 2021,
2, 3806-3850
Recent developments in sustainable corrosion inhibitors: design, performance and industrial scale applications
Received
4th September 2020
, Accepted 23rd April 2021
First published on 28th April 2021
Abstract
Recently, research studies in the fields of science and engineering are directed towards the synthesis, design, development, and consumption of environment-friendly chemical species to replace traditional toxic chemicals. This is because of the escalating demands of conservation understanding and stringent ecological rules. Currently, various environment-friendly alternatives derived from natural resources such as biopolymers, plant extracts, chemical medicines (drugs), etc. are widely used to replace toxic corrosion inhibitors. Moreover, various biopolymers in their pure and modified forms are extensively employed as environment-friendly corrosion inhibitors. Compounds derived through multicomponent reactions (MCRs), and microwave (MW) and ultrasound (US) irradiations are also considered as environment-friendly alternatives. Polyethylene glycol (PEG) and ionic liquids (ILs) possess low vapor pressure and are regarded as designer environment-friendly alternatives. The chemicals synthesized using green solvents such as water, ILs and supercritical CO2 can also be regarded as environment-friendly chemical species. A comprehensive literature survey reveals that these compounds are extensively utilized as metallic corrosion inhibitors in various corrosive electrolytes. Overall, this review provides a summary of several major reports on environment-friendly corrosion inhibitors.
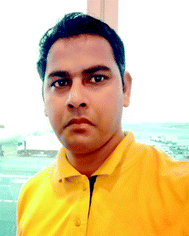
Chandrabhan Verma
| Chandrabhan Verma, PhD is a Postdoctoral Fellow at the Interdisciplinary Center for Research in Advanced Materials, King Fahd University of Petroleum and Minerals (KFUPM), Saudi Arabia. He obtained his PhD in Corrosion Science at the Department of Chemistry, Indian Institute of Technology (Banaras Hindu University) Varanasi, India. He is a member of American Chemical Society (ACS). His research is mainly focused on the synthesis and designing of environmental friendly corrosion inhibitors useful for several industrial applications. Dr Verma is the author of several research and review articles in peer-reviewed international journals of ACS, Elsevier, RSC, Wiley and Springer etc. He has total citation of more than 4600 with H-index of 37 and i-10 index of 83. Dr Verma received several national and international awards for his academic achievements. |
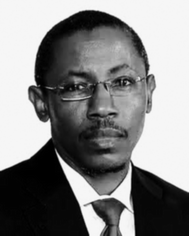
Eno E. Ebenso
| Eno E. Ebenso is a research professor of at the Institute of Nanotechnology and Water Sustainability in the College of Science, Engineering and Technology, University of South Africa. He has published extensively in local and international peer-reviewed journals of wide readership with over three hundred (300) publications (articles in newspapers, plenary/invited lectures and conference proceedings not included). He currently has an H-Index of 67 and over 10 000 total citations from the Scopus Search Engine of Elsevier Science since 1996. According to the Elsevier SciVal Insights Report (2010–2015), he has a citation impact 10% above world average; second most prolific author in the field of corrosion inhibition world wide and fifth most downloads of his publications globally in the field of corrosion inhibition. His Google Scholar Citations since 2013 is over 8000 with an H index of 64 and i10-index of 216. His RESEARCHERID account shows H index of 44 with total citations of 5779 and average citation per article of 24.78. He is also a B3 NRF Rated Scientist in Chemistry (South African National Research Foundation). INTERPRETATION – B3: Most of the reviewers are convinced that he enjoys considerable international recognition for the high quality and impact of his recent research outputs. He is a member of International Society of Electrochemistry, South African Chemical Institute (M.S.A. Chem. I.), South African Council for Natural Scientific Professions (SACNASP) (Pri. Sci. Nat)., Academy of Science of South Africa (ASSAf) and a fellow of the Royal Society of Chemistry, UK (FRSC). |
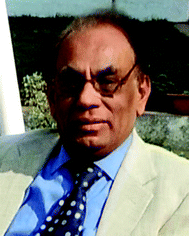
M. A. Quraishi
| Dr Mumtaz A. Quraishi is a Chair Professor at the Interdisciplinary Center for Research in Advanced Materials, King Fahd University of Petroleum and Minerals (KFUPM), Saudi Arabia. He obtained PhD in synthetic organic chemistry in 1986 from Kurukshetra University, and awarded DSc in 2004 from Aligarh Muslim University, Aligarh in the field of Corrosion Inhibition of Industrial Metals and Alloys. Before joining KFUPM he was institute Professor at IIT BHU Varanasi, India. He also served as Head (Chairman), Department of Chemistry, IIT BHU. He has teaching experience more than 35 years. He received several national and international awards. Dr Quraishi is an Associate editor of Current Materials Science, Bentham and a member of Editorial Board of the more than 30 International Journals. Dr Quraishi is a fellow of Royal Society of Chemistry, UK and a member of American Chemical Society. |
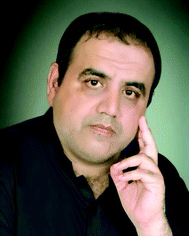
Chaudhery Mustansar Hussain
| Chaudhery Mustansar Hussain, PhD is an Adjunct Professor and Director of Labs in the Department of Chemistry & Environmental Sciences at the New Jersey Institute of Technology (NJIT), Newark, New Jersey, USA. His research is focused on the applications of Nanotechnology & Advanced technologies & Materials, Analytical Chemistry, Environmental Management, and Various Industries. Dr Hussain is the author of numerous papers in peer-reviewed journals as well as prolific author and editor of several (around 200 publications with more than 50 books) scientific monographs and handbooks in his research areas published with Elsevier, Royal Society of Chemistry, John Wiley & sons, CRC, Springer etc. |
1. Introduction
1.1. Corrosion inhibition: current trends and challenges
Metallic materials are widely used for various applications, for example, as constructional materials, especially in the petroleum, oil and gas industries.1,2 However, most of the metals in their pure form are thermodynamically unstable, and therefore they readily undergo corrosive degradation by the reaction of constituents of the surrounding environment. Corrosion causes huge safety concerns and economic damage. According to the recent estimation of the NACE (National Association of Corrosion Engineers), the global cost of corrosion is around US$2.5 trillion, which constitutes about 3.5% of the world's GDP. The cost of corrosion may be of direct or indirect type.3–5 The direct cost of corrosion includes the repair, storage and replacement of the corroded metallic equipment, modifying alloys into metals and vice versa. The cost associated with nickel plating and galvanization can also be regarded as the direct cost of corrosion.6,7 Apart from the above, economic losses associated with the synthesis, characterization and application of compounds as corrosion inhibitors are also integrated as the direct cost of corrosion. The indirect costs of corrosion include the leakage of liquids (petroleum) and gases from transport pipelines that adversely affect the performance of machineries and transport efficiency. Contamination of rusts and scales (corrosion products) can also adversely affect the quantity of the materials (liquids and gases) to be transported. These corrosion products can also reduce transport efficiency and the performance of machineries by choking the valves and joints. Obviously, the leakage of the transported petroleum based liquids and gases is associated with various environment related problems because of their toxicity.
In view of the above, several methods of corrosion mitigation have been developed by corrosion scientists and engineers. One of the oldest (before 1960) methods of corrosion mitigation was the implementation of inorganic compounds, mainly nitrites, chromates, borates, molybdates, silicates and zinc salts.8 These compounds become effective by forming a highly effective passive film over metal surfaces (passivators) through their adsorption. However, they were replaced by more economical alternatives such as phosphonic acid, gluconates, polyacrylates, surface active chelates, polyphosphates, polyphosphonates, phosphonates and carboxylates during 1960–1980.8 Generally, these compounds precipitate at the interface of the metal and environment and therefore they are called precipitating inhibitors (precipitators). Nevertheless, after that, ecological considerations came into play and toxic chemicals were replaced by natural alternatives including natural and bio-polymers, bio-surfactants, vitamins, tannins and natural compounds during 1980–1995. Recently (1995 to present), environment-friendly approaches such as the use of rare earth metals (REM), polyfunctional compounds, the synergism of organic/inorganic compounds using REM and the encapsulation of inhibitors are the main areas of focus.9 Obviously, these alternatives exhibit very low or no toxicity and high protection effectiveness.
Currently, organic compounds are established as one of the most effective and profitable methods of corrosion inhibition because of their association with E4 (efficiency, economy, ecology and environmental friendliness).10–12 Organic corrosion inhibitors are used for different industrial applications (Fig. 1). However, there are numerous challenges of using these compounds. One of the biggest challenges of using organic corrosion inhibitors is their limited solubility, especially in polar electrolytes.13,14 Because of their hydrophobic nature, organic corrosion inhibitors, especially compounds containing aromatic rings and non-polar hydrocarbon chains, show limited solubility that adversely affects their protection efficiency. Therefore, current research studies in corrosion science and engineering are oriented towards the development of corrosion inhibitors that contain hydrophilic polar functional substituents in their molecular structures.
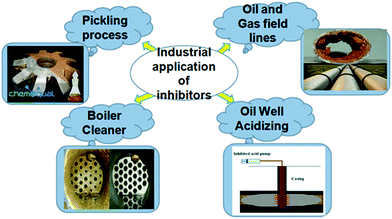 |
| Fig. 1 Applications of organic corrosion inhibitors in different industrial sectors. | |
The electron rich polar functional substituents enhance the bonding efficiency between the metal surface and organic compounds either directly participating in coordination bonding or increasing the electron density over the active sites of inhibitor molecules.15–17 The polar substituents also triggered the protection effectiveness of the organic inhibitors by increasing their hydrophilicity and solubility in polar electrolytes.16,17 The nature of the substituents plays a significant role in designing effective corrosion inhibitors. Electron donating substituents (EDS) with a negative Hammett substituent constant are expected to increase the inhibition effect of organic compounds.15,18–20 On the other hand, electron withdrawing substituents (EWS) with a positive Hammett substituent constant are expected to decrease the protection effectiveness of the inhibitors. Nevertheless, EWS such as –CN, –NO2, –COOH, –SO3H, etc. enhance the corrosion inhibition effectiveness of macromolecules and polymers by increasing their solubility in polar electrolytes.
Thermal decomposition represents another challenge of using organic compounds as inhibitors against metallic corrosion. The inhibition efficiency of most of the organic compounds decreases on increasing the temperature.21,22 This type of decrease in the inhibition efficiency is attributed to the acid or base catalyzed decomposition and/or rearrangement of the inhibitor molecules, especially at high temperatures.23,24 Obviously, the increase in the kinetic energy of the inhibitor molecules on increasing the temperature results in the corresponding decrease in the attractive force between the inhibitor molecules and the metallic surface.23,24 This observation reveals that the bonding between the inhibitor molecules and the metallic surface is mostly electrostatic, i.e. physisorption. However, the increase in protection efficiency on increasing the temperature has also been documented.25–27 The increase in the inhibition efficiency with the rise in temperature suggests that the interaction between the metal surface and the inhibitor molecules involves chemical bonding, i.e. chemisorption. The literature observation suggests that most of the organic corrosion inhibitors adsorb using a mixed mode of adsorption, i.e. physiochemisorption.28–30
1.2. Assessment of sustainable corrosion inhibitors: OSPAR and REACH commissions
Because of the rising environmental consciousness and severe ecological policies, decent advancements in the field of corrosion science and engineering are oriented towards the development, synthesis, design and implementation of environment-friendly alternatives to the conventional toxic corrosion inhibitors.31–33 Obviously, the environmentally benign nature of a chemical species (or corrosion inhibitor) can be determined by assessing its bioaccumulation ability, biodegradability and the impact of its toxicity on the environment.33–35 The Oslo and Paris Commission (OSPAR) and Registration, Evaluation, Authorisation and Restriction of Chemicals (REACH) are the international commissions for the assessment of the above parameters. REACH is a European Union Regulation (EUR) that was dated on 18 December 2006 and came into force on 7 June 2007.36,37 The 849-page EUR addresses the production, design and implementation of chemical substances as well as their impact on the environment and human health. OSPAR, which was formed on 22 September 1992, combines and updates the 1972 Oslo Convention on dumping of wastes at sea and 1974 Paris Convention on marine pollution resulting through land-based sources.38–40 These commissions set up some guidelines and indices, in terms of which the toxicity, biodegradability and bioaccumulation ability and their impact on human health and the environment of chemical compounds can be assessed. The toxicity of a chemical compound to be used as a corrosion inhibitor can be assessed using its lethal and ethical concentrations designated as LC50 and EC50, respectively.41,42 LC50 represents the concentration of the substance that causes the demise of 50% of the living population and EC50 denotes the chemical concentration that adversely affects the growth of the living population.43–45 Obviously, an inferior LC50/EC50 magnitude indicates superior sensitive toxicity and vice versa. A chemical with a LC50/EC50 value greater than 10 mg kg−1 is considered non-toxic.43–45 Most of the chemicals spontaneously undergo degradation by microorganisms called decomposers though the process is quite slow and takes several days, months or even years.46 A chemical can be categorized as environment-friendly if it decomposes 60% or more in 28 days.47,48 Bioaccumulation is the ability of a chemical to gather in a living organism when the source of the chemical is exclusively water.49–51 Generally, it is measured in terms of the partition coefficient, designated as log KOW or DOW. Generally, KOW or DOW represents the ratio of concentrations of a compound in an assortment of two immiscible solvents at equilibrium. Bioaccumulation is a measure in a mixture of water and octanol. For an environment-friendly compound, the KOW or DOW value should be equal to or less than 3.52
1.3. Computational chemistry tools for corrosion inhibition studies
Numerous chemical, electrochemical and surface morphological techniques are widely used for the evaluation of the inhibition performance of corrosion inhibitors.53–55 However, these experimental methods have several shortcomings. Generally, these experimental methods are associated with expensive synthesis and the use of toxic chemicals that adversely affect the surrounding environment.56–58 These chemicals are synthesized using toxic and expensive starting materials and catalyst using multistep reactions that consume huge amounts of solvents for purification and work-up processes. Discharge of excess amounts of organic solvents and toxic catalysts into the environment causes severe environment related issues. Because of the multistep nature, the syntheses of corrosion inhibitors are associated with the formation of several undesirable side products that can also pollute the environment. Instead of their highly toxic and expensive synthesis, most of the compounds do not give fruitful results and fail in experimental trials.28,59,60 In view of this, recently computational chemistry tools have come into play for determining the corrosion inhibition effectiveness of corrosion inhibitors.5,61,62 The environment-friendly nature of these techniques is associated with the fact that the inhibition effectiveness of compounds can be theoretically predicted before their toxic and expensive synthesis.5,62–64 Unlike experimental methods, computational modeling involves the use of computational software for predicting inhibitory efficiency instead of highly expensive instruments.65–67
Recently, several computational methods, especially density functional theory (DFT), molecular dynamics (MD) and Monte Carlo (MC) simulations, have emerged as powerful computational chemistry tools for corrosion monitoring.5,61,62 DFT simulations represent the most powerful computational tool, which give some vital indices including the energy of frontier molecular orbitals (FMOs; EHOMO and ELUMO) and numerous correlation parameters such as energy band gap (ELUMO − EHOMO = ΔE), hardness (η), electronegativity (χ), dipole moment (μ), softness (σ), fraction of electron transfer (ΔN), etc., in terms of which the adsorption ability and corrosion inhibition effectiveness of a compound can be explained.5,61,62,68 DFT studies are mostly used to correlate the relative inhibition effect of a series of compounds having similar molecular structures. In general, higher EHOMO, softness (σ) and dipole moment (μ) and lower ELUMO, ΔE, electronegativity (χ) and hardness (η) values are associated with high inhibition efficiency.61,62 One of the most significant indices is the energy band gap ΔE (ELUMO − EHOMO) and its lower value is associated with a higher inhibition efficiency.69,70 By studying the anticorrosive effect of chitosan (CH) modified with 4-amino-5-methyl-1,2,4-triazole-3-thiol (AMT), the authors reported that both CH and AMT separately have higher ΔE values as compared to the ΔE value of AMT modified CH (CH–AMT). This observation suggests that CH–AMT is relatively more reactive towards its absorption on the metallic surface as compared to CH and AMT separately (Fig. 2).71 An increase in protection efficiency for a series of corrosion inhibitors with a decrease in the ΔE value has been reported extensively.
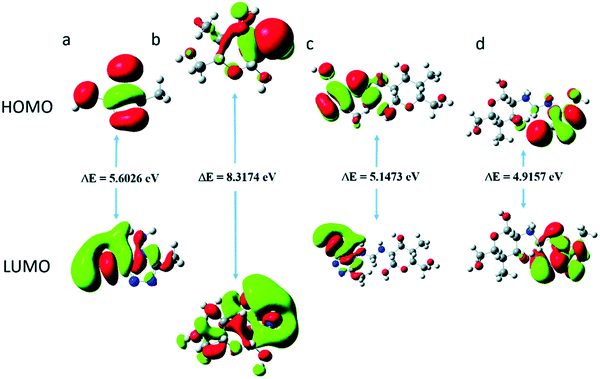 |
| Fig. 2 Presentation of energy band gaps (ΔE: ELUMO − EHOMO) for (a) 4-amino-5-methyl-1,2,4-triazole-3-thiol (AMT), (b) chitosan (CH), (c) neutral AMT modified CH and (d) protonated AMT modified (CH-MAT).71 | |
It is important to mention that, during metal and inhibitor interactions, some parts of the inhibitor molecules are involved in bonding with the metallic surface. These parts are called active or adsorption centers. Among the several experimental and computational techniques, the implementation of DFT is established as one of the most significant methods for identifying the active centers of the inhibitor molecule.61,62 Localized regions in FMOs (HOMO and LUMO) represent the electron rich centers (active centers) that actively contribute to charge sharing with the metallic surface.72–74 Generally, these electron rich centers are aromatic ring(s) and/or polar functional groups. However, the contribution of FMOs in charge sharing with the metallic surface depends greatly on the nature of substituents.15,19 In general, substituents with a negative Hammett (σ) or Taft (σ*) constant (electron donating) show an increased FMO contribution with an increase in electron density.15,19 In contrast, substituents with a positive Hammett (σ) or Taft (σ*) constant (electron withdrawing) show a decreased FMO contribution to charge sharing.15,19
A study on the inhibitive effect of 2-amino-4-arylquinoline-3-carbonitriles (AACs) on mild steel in acidic medium showed that the presence of –OH (AAC-3) increases the inhibition efficiency and the presence of a –NO2 substituent (AAC-1) decreases the inhibition efficiency as compared to the non-substituted compound (AAC-2).75
DFT studies revealed that the –NO2 substituent decreases the HOMO contribution, whereas the –OH substituent increases the HOMO contribution. The FMOs of the AACs are presented in Fig. 3. The negative sign of the Hammett constant for –OH indicates that it is an electron donor in nature and therefore increases the electron donor (HOMO) contribution and the converse is true for the –NO2 substituent. A similar finding was also observed when studying the inhibition effect of 5-arylpyrimido-[4,5-b]quinoline-diones (APQDs) for MS corrosion in acidic medium. DFT analyses showed that the –NO2 substituent with a positive Hammett constant (σ = +0.78) decreases the HOMO and LUMO contributions by decreasing the electron density.76 Very interestingly only nitrophenyl (–Ph–NO2) moieties are involved in the LUMO because of the electron withdrawing nature of –NO2 (APQD-4). In contrast, the presence of one and two –OH substituent(s) in APQD-3 and APQD-4, respectively, increase the contributions of both the HOMO and LUMO because of the electron donating nature of –OH (σ = −0.37).76 The frontier molecular orbital images of APQDs are shown in Fig. 4. A similar finding was also reported in other studies.17,77
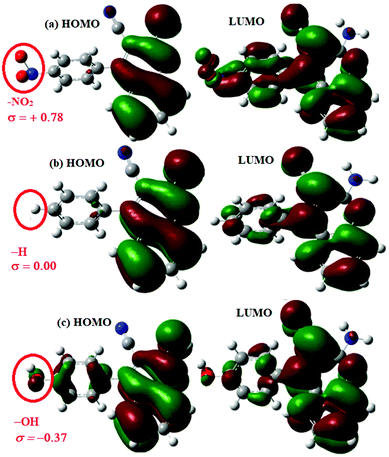 |
| Fig. 3 Frontier molecular orbitals (FMOs) of (a) AAC-1 (–NO2), (b) AAC-2 (–H) and (c) AAC-3 (–OH) derived using the Gaussian 09 software package.75 | |
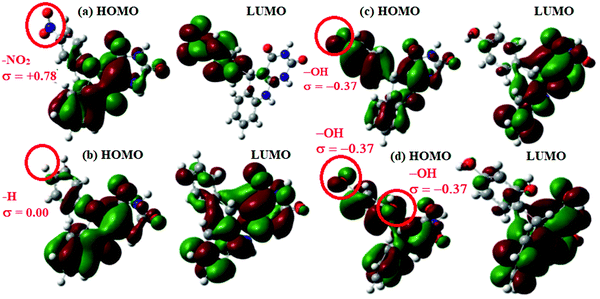 |
| Fig. 4 Frontier molecular orbital images of (a) APQD-1 (–NO2), (b) APQD-2 (–H), (c) APQD-3 (–OH) and (d) APQD-4 (2× –OH) derived using the Gaussian 09 software package.76 | |
Other significant computational techniques are molecular dynamics (MD) and Monte Carlo (MC) simulations.5,78–80 Using these techniques one can determine the orientation of the inhibitor on the metallic surface. Measurement of the orientation of the inhibitor on the metal surface is an important aspect of corrosion monitoring as an inhibitor with planar orientation covers a larger part of the metallic surface and acts as a superior corrosion inhibitor to an inhibitor with vertical orientation. When an inhibitor approaches the metallic surface, due to the effect of different attractive and repulsive forces it gets polarized and acquires a specific orientation depending upon the nature of the electronic structure of the inhibitor molecules. Nevertheless, substituents play a significant role in determining the orientation of the inhibitor molecules over the metal surface. Generally, electron donating substituents force the inhibitor molecules to obtain vertical orientation and vice versa.
The orientations of four 5-arylpyrimido-[4,5-b]quinoline-diones (APQDs) and glucosamine-based, pyrimidine-fused heterocycles (CARBs) are shown in Fig. 5 and 6. It can be clearly observed that in the presence of the electron withdrawing –NO2 substituent the extent of adsorption (yellow circle) decreased with respect to the adsorption extent of inhibitors with electron donating substituents.
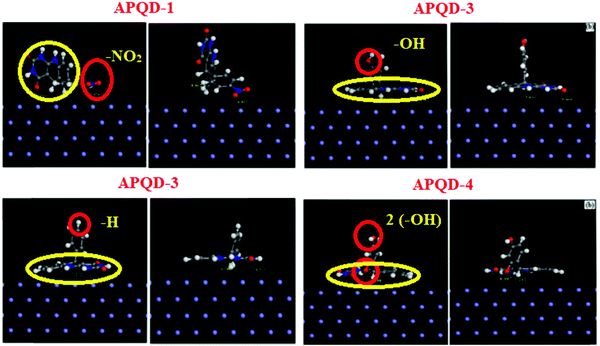 |
| Fig. 5 Top and side views of APQD-1 (–NO2), APQD-2 (–H), APQD-3 (–OH) and APQD-4 (2× –OH) on the Fe(110) surface derived using molecular dynamics simulations.76 | |
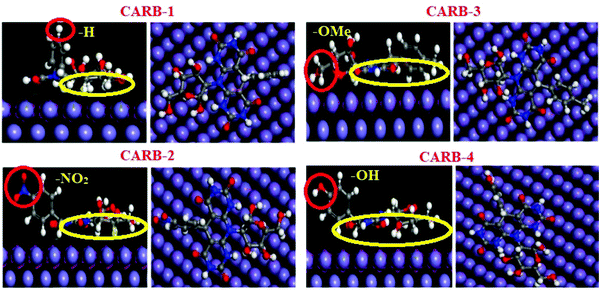 |
| Fig. 6 Top and side views of CARB-1 (–H2), CARB-2 (–NO2), CARB-3 (–OH) and APQD-4 (–OMe) on the Fe(110) surface derived using Monte Carlo simulations.17 | |
1.4. Design of effective corrosion inhibitors based on experimental and computational studies
From the above discussion it is clear that substituents play a significant role in determining the corrosion inhibition effect of organic corrosion inhibitors. Obviously, because of their electron donating or electron withdrawing nature, these substituents affect the overall electron density at the donor site(s) of the inhibitor molecules. It is important to mention that the interaction of organic corrosion inhibitors with the metallic surface involves the donor–acceptor (charge sharing) phenomenon. The effect of substituents on the electron sharing tendency of corrosion inhibitors can be assessed through DFT analysis (Fig. 3 and 4). On the other hand, the effect of substituents on the orientation of corrosion inhibitors over the metal surface can be easily assessed through MD or MC simulations (Fig. 5 and 6). Besides computational chemistry tools, the effect of electron donating as well as electron withdrawing substituents on the inhibition efficiency of the substituted aromatic corrosion inhibitors can also be determined using the Hammett substituent constant (σ) (or Taft substituent constant, σ*-for aliphatic cyclic and linear compounds). The various forms of the Hammett equations are presented below:15 |  | (1) |
| 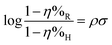 | (2) |
| 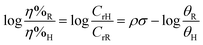 | (3) |
In the above equations, K, η%, θ and Cr represent the equilibrium constant, percentage inhibition efficiency, surface coverage and corrosion rate, respectively. Subscripts “H” and “R” represent the absence and presence of the substituent, “–R”. ρ is the reaction parameter and its value mainly depends upon the nature of reaction. σ is the Hammett substituent constant and its value reflects the total electron density at the donor (adsorption) site(s). Generally, the negative sign of the Hammett constant is consistent with the electron donating ability of the substituents and vice versa.
It is extensively reported that electron donating substituents such as –OH (hydroxyl), –NH2 (amino; 1°-amine), >NH (2°-amine), >N– (3°-amine), –CH3 (methyl), –OCH3 (methoxy), etc. generally increase the corrosion inhibition efficiency of organic compounds, whereas the converse is true for electron withdrawing substituents including –CN (nitrile), –NO2 (nitro) and –COOH (carboxyl).15 Moreover, the addition of a substituent also affects the overall molecular size of the compound to be used as the corrosion inhibitor. An organic compound with a larger molecular size is expected to be a more effective corrosion inhibitor as compared to the molecule that has a relatively smaller molecular size. Therefore, the effects of the molecular size and substituent on the inhibition efficiency for a specified class of organic compounds differ from case to case. Nevertheless, in most of the previous reports, it is observed that the presence of electron donating substituents increases the corrosion inhibition efficiency of organic compounds and the converse is true for electron withdrawing substituents.
M. Abdallah et al.81 studied the inhibition efficiency of five 5-arylazothiazole derivatives for 1018 carbon steel corrosion in 0.5 M H2SO4 using experimental methods. These compounds differed in the nature of substituents and were designated as compound 1 (–NO2), compound 2 (–Br), compound 3 (–H), compound 4 (–CH3) and compound 5 (–OCH3). The results derived through weight loss study showed that, as compared to the non-substituted 5-arylazothiazole derivative (compound 3), compounds 4 and 5 having electron donating substituents showed superior corrosion inhibition efficiency and compounds 1 and 2 having electron withdrawing substituents showed inferior corrosion inhibition efficiency. The weight loss study was further supported by electrochemical studies. The order of inhibition efficiencies derived from electrochemical studies was consistent with the order of weight loss. The analysis showed that the 5-arylazothiazole derivative containing the most electron withdrawing nitro substituent (compound 1) exhibited the lowest inhibition efficiency and the 5-arylazothiazole derivative containing the most electron donating methoxy substituent (compound 5) exhibited the highest inhibition efficiency (compound 1) toward 1018 carbon steel corrosion in 0.5 M H2SO4.
The effect of the substituents on the corrosion inhibition effectiveness of organic corrosion inhibitors is also investigated in various other reports.15,82 Our research group also widely reported the effect of substituent on the corrosion inhibition effectiveness of organic compounds for mild steel in acidic hydrochloric acid solution. Findings from the literature investigation suggest that the inhibition efficiency of organic corrosion inhibitors is greatly dependent upon the nature of substituents. Therefore, it is important to notice that compounds that contain electron donating substituents would be preferred to be used as corrosion inhibitors as compared to compounds that contain electron withdrawing substituents in their molecular structures.
1.5. Corrosion inhibitors for industrial processes
Various industrial processes involve the utilization of highly concentrated acidic solutions.83–85 One of the most significant and widely known acidic processes is acid pickling which involves the removal of rusts and surface impurities of metal plates, wires and pipelines and metal equipment in various industrial sectors including heat exchange, heat transfer, cooling systems and boilers. Acidic solutions of hydrochloric acid, sulfuric acid, phosphoric acid, nitric acid, sulfamic acid, citric acid, and hydrofluoric acid are commonly used in acid pickling and descaling processes to remove surface metal oxide based rusts and scales.86 Because of their highly aggressive nature acidic solutions cause corrosion related failure of metallic structures during these processes. In addition, during these processes hydrogen gas is produced which can penetrate the interior of metal structures and can induce hydrogen embrittlement.87 Therefore, these industrial processes need the addition of some suitable chemical species called corrosion inhibitors. It is important to mention that most of the effective acid pickling based corrosion inhibitors are organic, especially heterocyclic compounds.87 The addition of corrosion inhibitors not only avoids metallic corrosion but can also extend the service duration of metallic equipment and diminish the amount of acid used.
Organic compounds, especially organic amines, quaternary ammonium salt, urea and thiourea derivatives, rosin amine, acetylenic compounds, alkaloids, Mooney alkali and so on, are widely used as corrosion inhibitors for pickling in sulfuric acid solution.88–90 On the other hand, acid pickling of various metallic alloys, especially mild steel and carbon steel, in hydrochloric acid mainly employs heterocyclic organic compounds containing N, O, P and S atoms.91–95 Ammonia and thiourea based organic compounds are extensively used as corrosion inhibitors for pickling in hydrochloric acid based solutions. Metal oxides and boiler scales are extremely soluble in nitric acid. Nitric acid solutions are highly oxidizing in nature and therefore a relatively lower number of corrosion inhibitor formulations have been developed for pickling in nitric acid. Nevertheless, a mixture of hydrazine (C8H7N) and Na2S or NH4SCN and a mixture of thiourea and Na2S are extensively used as corrosion inhibitors for pickling in nitric acid.87 The pickling process in phosphoric acid solutions involves the consumption of various heterocyclic compounds including triazole, benzotriazole and urea derivatives, polyvinylpyrrolidone (PVP), sulfonated imidazoline, polyethyleneimine (PEI), etc.96–99 along with the inorganic and their mixed-formulations. Organic compounds are also used as corrosion inhibitors for pickling in other acidic solutions. These compounds become effective by adsorbing on the metallic surface using their electron rich centers called adsorption sites. It is important to mention that lower concentrations of acidic solutions are used for the descaling process, whereas highly concentrated acidic solutions are used for the acid pickling process.
Another industrial process that requires the use of highly concentrated acidic solution is oil-well acidification in the petroleum industry.100,101 In this process, a highly concentrated acidic solution of hydrochloric acid (mostly 15–28%) is injected into the well through a metallic pipeline to enhance the flow of oil. Tubing of these highly acidic solutions causes extensive corrosion of the metallic surface during the acidization process. Therefore, some external additives known as corrosion inhibitors are added into the acidizing solution. A literature study showed that most of the previously used inhibitors for the acidization process are heterocyclic compounds containing heteroatoms, especially N and O. Obviously, these compounds become effective by adsorbing on the metallic surface using their electron rich centers called adsorption centers. This type of adsorption results in the formation of a protective film which isolates the metal surface from aggressive solutions and protects from corrosive damage.
Most of the previously used industrially useful corrosion inhibitors are not environment-friendly because of their toxic nature and synthesis using toxic chemicals and solvents. However, because of the increasing ecological awareness and strict environmental regulations, the use of environment-friendly compounds as corrosion inhibitors derived through environment-friendly synthetic approaches and natural resources would be preferred. In view of this, compounds derived from amino acids are used as corrosion inhibitors for pickling, descaling and acidization processes.102,103 Organic compounds (especially heterocyclic ones) derived from one-step multicomponent reactions (MCRs) with and without MW and US irradiations can be used as environment-friendly corrosion inhibitors for these industrial processes.104–106
2. Environment-friendly corrosion inhibitors: literature survey
2.1. Biopolymers as environment-friendly corrosion inhibitors
Biopolymers are natural polymers produced by the cells of animals and plants. Because of their natural (animal and plant) origin, biopolymers are considered as environment-friendly alternatives to be used for different biological and industrial applications. Moreover, unlike synthetic polymers, biopolymers are biodegradable and non-bioaccumulative. Some of the common biopolymers are polysaccharides (starch, cellulose, chitosan, etc.), polypeptides, nucleic acids (RNA & DNA), natural rubber, lignin, etc. Because of the increasing ecological awareness and environmental regulations, the use of environment-friendly biopolymers would be preferred. In view of this, various reports have been published describing the anticorrosive effect of biopolymers. It is important to mention that almost all kinds of biopolymers are evaluated as corrosion inhibitors; however, in the present study we described the corrosion inhibition effect of carbohydrate based polymers, especially chitosan and cellulose and their derivatives as they are the most frequently used biopolymer based corrosion inhibitors.
Chitosan is a linear polysaccharide of randomly distributed N-acetyl-D-glucosamine and D-glucosamine connected together by a β-1,4-glycosidic linkage.107–109N-Acetyl-D-glucosamine and D-glucosamine are called acetylated and deacetylated units, respectively.107–109 Generally, the synthesis of chitosan is achieved by deacetylation of chitin as shown in Fig. 7. Chitosan possesses numerous industrial and biological applications and commercially fashioned by the partial deacetylation of chitin which is a structural constituent of the exoskeleton of crustacea (such as shrimps and crabs) and the cell walls of fungi.110–112 Commercially useful chitosan acquires a degree of deacetylation (%DD) of 60–100 and a molecular weight of 3800–20
000 Da.113,114 Polar substituents such as –CH2OH (hydroxymethyl), –NHCOCH3 (acetyl), –OH (hydryl), –NH2 (amine) and –O– (ether) present in the molecular structure of chitosan can effectively form bonding with the metallic surface. The anticorrosive effect of chitosan for metals and alloys is widely reported.115–118 Because of their natural origin, chitosan based corrosion inhibitors are considered as environment-friendly.119–121 Generally, chitosan modified with various organic compounds exhibits a higher corrosion inhibition efficiency than pure chitosan.
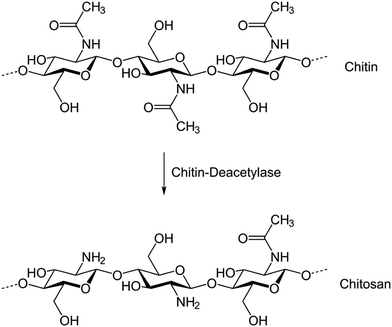 |
| Fig. 7 Schematic illustration of the synthesis of chitosan from chitin. | |
Umoren et al.122 reported the corrosion inhibition effect of chitosan for MS in HCl medium using chemical, surface characterization and electrochemical methods. Studies revealed that CH showed reasonably good efficiencies of 96% and 93% at 60 °C and 70 °C, respectively. Adsorption of CH followed the Langmuir adsorption isotherm model. Electrochemically it was derived that CH behaved as an interface- and mixed-type inhibitor for MS acidic corrosion. The anticorrosive effect of CH for MS in 1 M HCl has also been reported elsewhere.123 Generally, chitosan acts as a mixed-type corrosion inhibitor by retarding the anodic as well as cathodic half-cell reactions. Recently, our research team described the influence of the inhibition of CH for MS corrosion in sulfamic acid medium.124 Numerous experimental methods were employed to determine the inhibition efficiency of CH with and without potassium iodide, KI (5 ppm). The authors observed that at a 200 ppm concentration CH manifests 90% and 73.8% inhibition efficiencies with and without KI, respectively.124 In all the experimental conditions CH behaves as a mixed-type inhibitor. The adsorption mechanism of CH on the MS surface was studied by means of SEM and AFM methods. The outcomes of this study showed that the presence of KI significantly improved the corrosion inhibition effectiveness of CH. An increase in the inhibition efficiency of organic corrosion inhibitors by the addition of other chemical species, especially salts (e.g. KI & ZnCl2), is known as synergism. Synergism or the synergistic effect is widely investigated in the field of corrosion inhibition using organic compounds. CH is also used as a corrosion inhibitor for copper in acidic125 and NaCl126,127 electrolytes. Harmami and coworkers128 demonstrated the inhibition effect of water soluble chitosan (WSC) derived from shrimp and mussel shells for tinplate in 2% NaCl medium. Protection efficiency was determined at different concentrations ranging from 10 to 1500 mg L−1. The weight loss and PDP methods revealed that WSC derived from shrimp shell waste showed the highest efficiencies of 72.73% and 91.41%, respectively, whereas WSC derived from mussel shells showed the highest efficiency of 54.55% at a 1300 mg L−1 concentration. WSC derived from shrimp and mussel shell waste acted as mixed-type inhibitors (PDP analyses).
Although chitosan shows reasonably good anticorrosive properties in salt solutions and acidic electrolytes, its anticorrosive effect and solubility in aqueous electrolytes can be further enhanced by its functionalization using several species.129–137 It is important to mention that this type of functionalization not only increases chitosan's solubility in polar electrolytes but also enhances its corrosion inhibition effectiveness by increasing the number of donor (active) sites responsible for interaction/adsorption with the metallic surface. Generally, this type of functionalization also increases the molecular size which results in a higher protection efficiency of chitosan derivatives as compared to chitosan itself. An inhibitor molecule with a larger molecular size acts as a better corrosion inhibitor as compared to the molecule with a smaller molecular size. Table 1 presents the functionalization of chitosan and its derivatives and their use in corrosion inhibition.
Table 1 Chemical name, abbreviation, nature of adsorption, metal and electrolyte system, method of corrosion measurements, highest inhibition efficiency and optimum concentration of some chitosan derivatives evaluated as corrosion inhibitors
S. no. |
Moiety attached from chitosan & abbreviation |
Nature of adsorption |
Electrolyte and metal |
Method of corrosion monitoring |
IE% and conc. |
Ref. |
1 |
Chitosan–cinnamaldehyde (Cinn-Cht) |
Langmuir isotherm & mixed-type inhibitor |
15% HCl/carbon steel |
WL, EIS, PDP, SEM, DFT and MCS |
87.72% at 600 ppm & 92.67% at 600 ppm + 10 mM KI |
134
|
2 |
Carboxymethyl-chitosan-benzaldehyde (CMChi-B) & carboxymethyl-chitosan–urea-glutaric acid (CMChi-UGLU) |
— |
2% NaCl and 1–3 M HCl/steel |
Fluidization techniques |
CMChi-B (80.82%) > CMChi-UGLU (80.62%) |
133
|
3 |
CH-Benzaldehyde (CSB-1), CH-4 (dimethylamino)benzaldehyde (CSB-2) & 4-hydroxy-3-methoxy benzaldehyde (CSB-3) |
Langmuir isotherm & slight cathodic-type inhibitor |
1 M HCl/mild steel |
WL, EIS, PDP, SEM, EDX, FT-IR, DFT and MDS |
CSB-3 (91.43%) > CSB-2 (89.87%) CSB-1 (88.63%) at 100 ppm |
138
|
4 |
Chitosan–vanillin (Van-Cht) |
Langmuir isotherm & mixed-type inhibitor |
15% HCl/carbon steel |
WL, EIS, EFM, PDP, SEM, FT-IR and DFT |
92.72% at 500 mg L−1 |
139
|
5 |
Chitosan–salicylaldehyde (CHSA) |
Temkin adsorption isotherm/mixed-type inhibitor |
1 M HCl/mild steel |
WL, EIS, PDP, EDX and SEM |
70.08% at 1500 ppm |
140
|
6 |
Chitosan–thiosemicarbazide (TSFCS) & chitosan–thiocarbohydrazide (TCFCS) |
Mixed-type inhibitors |
2% Acetic acid/304 steel |
PDP |
TCFCS (92%) at 60 mg L−1 |
141
|
7 |
Chitosan–poly(vinyl butyral) (PVB-Ch) |
— |
0.3 M salt solution/carbon steel |
EIS, PDP, SEM, EDX and Raman spectroscopy |
— |
142
|
8 |
Chitosan–polyethylene glycol (Cht-PEG) |
Langmuir isotherm & slight cathodic-type inhibitor |
1 M sulfamic acid/mild steel |
WL, EIS, PDP, SEM, FT-IR and DFT |
93.9% at 200 ppm |
143
|
9 |
Chitosan–polyethylene glycol (CS-PEG) |
Langmuir isotherm & slight cathodic-type inhibitor |
1 M HCl/mild steel |
WL, EIS, PDP, AFM and DFT |
93.9% at 200 mg L−1 |
145
|
10 |
Chitosan–polyaniline (PANI/CTS) |
Mixed-type inhibitor |
0.5 M HCl/Q235 steel |
EIS, PDP, SEM and DFT |
79.02% at 200 ppm |
146
|
11 |
Chitosan–methyl acrylate–ethylene diamine (CS-MAA-EN) & chitosan–methyl acrylate–triethylene tetramine (CS-MAA-TN) |
— |
5% HCl/carbon steel |
WL, EIS, PDP and SEM |
CS-MAA-EN (88.06%) > CS (84.22%) > CS-MAA-TN (69.46%) at 0.3% |
135
|
12 |
Chitosan–polyaspartic acid (PASP/CS) |
Anodic-type inhibitor |
3.5% NaCl/carbon steel |
WL, EIS, PDP and SEM |
87.56% at 20 ppm |
136
|
13 |
Carboxymethyl chitosan (CMC) |
Langmuir adsorption isotherm/mixed-type inhibitor |
3.5% NaCl/1020 carbon steel |
PDP, EIS |
85.57% at 80 ppm |
137
|
Recently, various reports dealing with the anticorrosive effect of Schiff bases (SBs) derived from aromatic aldehydes and chitosan have been published.133 SBs show remarkably high solubility and protection efficiency in polar electrolytes as compared to pure chitosan. The increase in the protection effectiveness on adding organic moieties in the molecular structure of chitosan is attributed to the increased number of active centers and enhanced solubility. Haque et al.138 synthesized three chitosan based SBs (CSBs) from benzaldehyde (CSB-1), 4-(dimethylamino)benzaldehyde (CSB-2) and 4-hydroxy-3-methoxybenzaldehyde (CSB-3) and they were evaluated for their ability to prevent metallic corrosion in 1 M HCl. Among the tested CSBs, CSB-3 showed the highest protection efficiency of 90.65% at a 50 ppm concentration. SEM, EDX and FT-IR analyses were conducted to demonstrate the adsorption nature of corrosion protection. Electrochemical studies validated the mixed and interface-type nature of the tested CSBs. DFT and MD simulation studies indicated that the CSBs interact with the metal surface in the donor–acceptor mode and acquire planar orientations on the Fe(100) surface. CSBs are also reported as anticorrosive materials in other studies.139,140 Most of the investigated SBs behave as mixed-type corrosion inhibitors as they adversely affect the anodic as well as cathodic Tafel reactions. Through EIS studies, it can be observed that SBs act as interface-type corrosion inhibitors as they become effective by adsorbing at the interface of metal and electrolyte.
Currently, cross-linking of chitosan with organic compounds and their relevance as corrosion inhibitors is gaining particular attention. Cross-linking of chitosan results in the joining of two or more polymeric chains (of chitosan) through an organic linker. This type of cross-linking increases the solubility as well as protection efficiency of chitosan derivatives.141,142 Recently, Chauhan and coworkers143 cross-linked chitosan with PEG (Cht-PEG) and evaluated its ability to act as a corrosion inhibitor for mild steel in 1 M sulfamic acid. Cht-PEG showed the highest protection of 93.9% at a 200 mg L−1 concentration. The Cht-PEG adsorption obeyed the Langmuir adsorption isotherm model. The polarization study validated the mixed and slightly cathodic dominance behavior of Cht-PEG. Cht-PEG interacts with the MS surface using the donor–acceptor mechanism. Recently, our research team reported the cross-linking of chitosan with thiocarbohydrazide (TC-Cht) and tested it as an anticorrosive material for stainless steel in 3.5% NaCl medium.144 TC-Cht showed more than 94% efficiency at a 500 mg L−1 concentration. Similar to Cht-PEG, TC-Cht adsorption obeyed the Langmuir isotherm model and exhibited slightly cathodic behavior. SEM and EDX studies were performed to demonstrate the adsorption nature of corrosion protection.
The above chitosan based composite materials are also used extensively as corrosion inhibitors for different metals and alloys in various electrolytes. It is important to mention that these formulations exhibit superior anticorrosive properties to pure chitosan. Kong et al. synthesized a chitosan–polyaniline (PANI/CTS) and tested its anticorrosive effect for Q235 steel in acidic medium.146 Electrochemical studies demonstrate that PANI/CTS manifests a mixed-type nature. Interactions of PANI/CTS with the Q235 steel surface take place through the donor–acceptor mode. SEM studies suggested that PANI/CTS adsorbs on the Q235 steel surface and improves the surface morphology of the inhibited metal specimen. Fig. 8 shows the SEM images of the Q235 steel surface after 2 h corrosion in 0.5 M HCl. It can be clearly seen that PANI/CTS remarkably smoothened the surface morphology of Q235 steel. This observation suggests that PANI/CTS forms an inhibitive film through adsorption on the Q235 steel surface. Other chitosan based composites such as chitosan–Zn nanoparticles,147–150 chitosan–Ag nanoparticles,151,152 chitosan–Cu, Ni, Au, and F,153–155 chitosan/TiO2,156–158 chitosan–hydroxyapatite,159–163 chitosan–polyamines,135 chitosan–drug,164 chitosan–polymer,142,146,165,166 chitosan–boron nitrile,167 chitosan–nanocomposites,168–170 carboxymethyl chitosan grafted poly(2-methyl-1-vinylimidazole),171,172 sulfonated chitosan,173,174 chitosan–polymer blends,142,175etc. are also widely used as anticorrosive materials. Generally, the above chitosan based formulations showed a higher protection efficiency as compared to pure chitosan. Therefore, it is recommended that in future studies, instead of using pure chitosan, its derivatives would be preferred. Obviously, these compounds become effective by adsorbing on the metallic surface following the Langmuir adsorption isotherm model. Using potentiodynamic polarization studies, it can be observed that most of the chitosan based formulations act as mixed-type corrosion inhibitors as they become effective by retarding both anodic as well as cathodic Tafel (polarization) reactions.
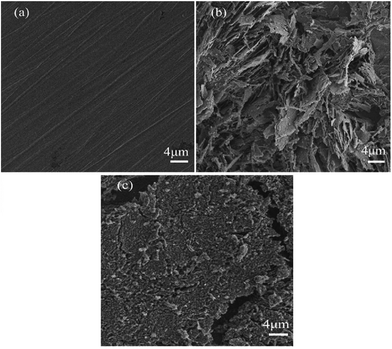 |
| Fig. 8 SEM micrographs of Q235 steel (a) before and (b) after 2 h corrosion in 0.5 M HCl without PANI/CTS and (c) after 2 h corrosion in 0.5 M HCl with PANI/CTS.146 | |
Cellulose is another biologically and industrially useful carbohydrate based polymer. It is a linear chain polysaccharide composed of D-glucose units joined together by a β → 1–4-glycosidic linkage.176,177 Cellulose has the general formula of (C5H10O5)n and it is a structural component of plant cell walls, algae and oomycetes. Cellulose is one of the most plentiful polymers on the earth and around 90%, 40–5% and 57% of cellulose is present in cotton, wood and hemp, respectively.178–181 Cellulose is widely used for various industrial and biological applications. However, its use as an anticorrosive material is scarce because of its limited solubility in most of the polar electrolytes. However, its derivatives are widely used either as corrosion inhibitors in aqueous electrolytes or as coating phase inhibitors.182 Similar to other polysaccharides, cellulose and its derivatives can be regarded as environment-friendly alternatives to be used as corrosion inhibitors. The environment-friendly nature of cellulose and its derivatives is attributed to their biological origin and/or properties of biodegradation and non-bioaccumulation. Derivatization of cellulose has been widely reported; however, carboxymethyl cellulose (CMC) and hydroxymethyl cellulose (HEC) are most frequently utilized as corrosion inhibitors. Table 2 presents a summary of cellulose derivatives used as corrosion inhibitors.183–199 Umoren et al.183 reported the inhibition effect of CMC for MS corrosion in 2 M H2SO4 using weight loss (WL) and hydrogen evolution (HE) methods. The results showed that the adsorption of CMC obeyed the Langmuir adsorption isotherm model. The protection efficiency of CMC was measured at different temperatures in the absence and presence of KCl, KBr and KI (5 mM). Studies showed that significant improvement in the inhibition effectiveness was observed in the presence of halide salts, except in the case of KCl. The inhibition efficiency of CMC with halide salts followed the order: KI > KBr > KCl. CMC (0.5 g L−1), CMC + KCl (5 mM), CMC + KBr (5 mM) and CMC + KI (5 mM) showed protection effectiveness of 56%, 48%, 63% and 85%, respectively.
Table 2 Chemical name, abbreviation, nature of adsorption, metal and electrolyte system, method of corrosion measurements, highest inhibition efficiency and optimum concentration of some cellulose derivatives evaluated as corrosion inhibitors
S. no. |
Moiety attached from chitosan & abbreviation |
Nature of adsorption |
Electrolyte and metal |
Method of corrosion monitoring |
IE% and conc. |
Ref. |
1 |
Carboxymethyl cellulose (CMC) |
Langmuir adsorption isotherm |
2 M H2SO4/mild steel |
WL and hydrogen evolution |
CMC (65% at 0.5 g L−1) |
183
|
2 |
Hydroxyethyl cellulose (HEC) |
Langmuir isotherm & mixed-type inhibitor |
3.5 NaCl/1018 c-steel |
PDP, EIS, EFM, SEM, EDX and DFT |
95.5% at 0.5 mM |
185
|
3 |
Hydroxyethyl cellulose (HEC) |
Freundlich isotherm & mixed-type inhibitor |
0.5 M H2SO4/mild steel |
WL, EIS, PDP, DFT and MDS |
70.35% at 2000 mg L−1 (333 K) |
186
|
4 |
Hydroxyethyl cellulose (HEC) 2017 |
Langmuir isotherm & mixed-type inhibitor |
1 M HCl/A1020 carbon steel |
WL, PDP, EIS, SEM, EDX, AFM and DFT |
91.62% at 500 ppm |
187
|
5 |
Ethyl hydroxyethyl cellulose (EHEC) |
Langmuir isotherm & slightly cathodic-type inhibitor |
1 M H2SO4/mild steel |
WL, EIS, PDP and DFT |
68.19% (EHEC) and 91.05% (EHEC + KI) at 2.5 g L−1 |
188
|
6 |
Hydroxyethyl cellulose (HEC) |
Langmuir isotherm & mixed-type inhibitor |
2% NH4Cl, zinc–carbon battery |
PDP, EIS, SEM and FT-IR |
92.07% at 300 ppm |
189
|
7 |
Hydroxypropyl cellulose (HPC) |
Langmuir isotherm & mixed-type inhibitor |
0.5 M HCl and 2 M H2SO4/aluminum |
WL, PDP and DFT |
92.54% (H2SO4) & 80.33% (HCl) at 5 g L−1 |
190
|
8 |
Hydroxyethyl cellulose (HEC) & hydroxypropyl methylcellulose (HPMC) |
Slightly cathodic-type inhibitors |
1 M HCl/aluminum |
WL, PDP, EIS and DFT |
HEC (83.25%) and HPMC (84.68%) at 2000 mg L−1 (1 day) |
191
|
9 |
Hydroxyethyl cellulose (HEC) |
Langmuir isotherm & mixed-type inhibitor |
0.5 M H2SO4/mild steel & aluminum |
WL, EIS, PDP, DFT and MDS |
93.61% (mild steel) 64.18% (Al) at 2000 mg L−1 |
192
|
10 |
Cellulose acetate |
— |
0.5, 1, 2 & 3 M HCl/aluminum |
WL and SEM |
55.71% |
193
|
11 |
Sodium carboxymethyl cellulose (Na-CMC) 2020 |
Freundlich adsorption isotherm |
1 M HCl/aluminum |
WL |
86.0% at 1 g L−1 (at 35 °C) |
194
|
12 |
Hydroxyethyl cellulose (HEC) |
|
0.5 M HCl/mild steel & aluminum |
WL |
67.94% at 2.5 × 10−3 M |
195
|
13 |
Carboxymethyl cellulose/AgNPs composite (CMC/AgNPs) |
Langmuir isotherm & mixed-type inhibitor |
15% H2SO4/St37 steel |
WL, EIS, PDP, SEM, EDX, AFM and FT-IR |
96.37% at 1000 ppm (at 60 °C) |
184
|
14 |
Chitosan (CH) and carboxymethyl cellulose (CMC) |
Langmuir isotherm & mixed-type inhibitors |
3.5% NaCl + CO2/API 5 L X60 pipeline steel |
EIS, PDP and SEM |
88% (Commercial inh.), 45% (CH) and 39% (CMC) at 100 ppm |
196
|
15 |
Hydroxyethyl cellulose (HEC) |
Mixed-type inhibitor |
1 M HCl and 0.5 M H2SO4/copper |
WL, EIS, PDP, DFT and MDS |
95% at 2000 mg L−1 |
197
|
16 |
Sodium carboxymethyl cellulose (Na-CMC) |
Langmuir isotherm & slightly cathodic-type inhibitor |
Simulated water (NaCl)/copper |
PDP, EIS, AFM, FT-IR, DFT and MDS |
83.34% at 5 mg L−1(at 20 °C) |
198
|
17 |
NEC, NMCC & NCMC |
Mixed-type inhibitors |
3.5% NaCl/copper |
PDP, EIS, SEM and EDX |
94.7% (NEC), 33.2% (NMCC) & 83.4% (NCMC) at 100 ppm |
199
|
The same group of authors reported the synthesis, characterization and corrosion inhibition effectiveness of the CMC and Ag nanoparticle (AgNP) composite (CMC/AgNPs) for St37 steel in 15% H2SO4 using chemical, electrochemical and surface morphological measurements.184 The results showed that CMC/AgNPs inhibited corrosion by adsorption and its adsorption was reinforced by SEM, EDX, AFM and FT-IR methods. The SEM study showed that the surface morphology of the metal is improved significantly in the presence of CMC/AgNPs which validated the adsorption mechanism of corrosion inhibitors. This observation was further supported by the change in elemental composition in the EDX spectra of St37 steel recorded with and without CMC/AgNPs after 25 h immersion. The SEM and EDX spectra of the St37 surface after 25 h immersion in 15% H2SO4 with and without CMC/AgNPs are shown in Fig. 9. The corrosion inhibition properties of cellulose derivatives are also extensively reported in other studies for carbon and mild steel in acidic4,6,13,14 and NaCl3,14 electrolytes. They are also used as corrosion inhibitors for aluminum7,8,11,12,15,18 and copper.5,10,16 The synergistic effect of halide ions on the anticorrosive effect of cellulose derivatives for aluminum is also studied widely.8,11
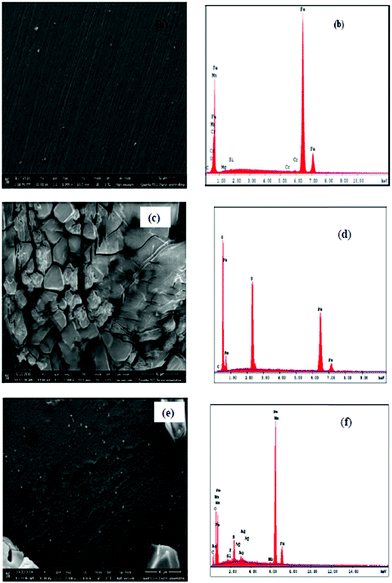 |
| Fig. 9 SEM and EDX spectra of (a and b) abraded St37 surface, (c and d) corroded St37 surface in 15% H2SO4 without CMC/AgNPs for 25 h at 25 °C and (e and f) corroded St37 surface in 15% H2SO4 with 1000 ppm of CMC/AgNPs for 25 h at 25 °C. The morphology of the St37 steel surface is significantly improved in the presence of CMC/AgNPs.184 | |
Starch, consisting of linear amylose and branched-chain amylopectin, is also used as an effective corrosion inhibitor for metals and alloys. However, because of its limited solubility, its application in corrosion inhibition is limited. Therefore, chemically and physically modified starch is widely employed as a corrosion inhibitor for metals. Brindha et al.200 demonstrated the anticorrosive properties of starch modified with 2,6-diphenyl-3-methylpiperidin-4-one (DPMP) for mild steel in acidic medium. Several chemical and electrochemical methods were employed and it was observed that the protection efficiency of modified starch was dependent on immersion time and temperature. The protection effectiveness of starch modified with sodium dodecyl sulfate (DS) and cetyltrimethylammonium bromide (CMAB) was reported using chemical and electrochemical techniques.201 Later on, several other studies dealing with the anticorrosive effect of starch,202–204 pectin,205–210 dextrin & cyclodextrin,13,211–215 pectate,216,217 alginates218,219 and exudate gums220–225 are reported for metals and alloys in different electrolytes. The synergistic effect of halide ions is also reported for different classes of biopolymers.226–229 Similar to chitosan, cellulose and their derivatives, these polymeric carbohydrates also behave as mixed-type corrosion inhibitors. They become effective by retarding the anodic and cathodic Tafel polarization reactions.
2.2. Plant extracts as environment-friendly corrosion inhibitors
Recently, the use of plant based materials is gaining particular attention because of their environmentally benign nature. Because of the natural and biological origin, plant based materials including plant extracts are environment-friendly alternatives to be used as metallic corrosion inhibitors for different biological and industrial applications.230–234 Generally, each plant extract contains several complex phytochemicals containing several electron rich centers that can act as adsorption centers during metal–inhibitor interactions.230,235–237 The electron rich centers include polar functional groups such as hydroxyl (–OH), amino (–NH2), ester (–COOC2H5), amide (–CONH2), acid chloride (–COCl), dimethyl amino (–NMe2), methoxy (–OMe), ether (–O–), etc. and multiple bonds such as >C
C<, >C
N–, >C
O, –N
O, –C
C–, –C
N, –N
N–, etc.
Although most of the phytochemicals are complex molecules, they are readily soluble in polar electrolytes because of their association with high peripheral functionalities in the form of polar functional groups. Plant extracts are extensively used as environment-friendly corrosion inhibitors for different metals and alloys in different electrolytic systems. The protection efficiency of extracts that have a relatively lower protection efficiency against metallic corrosion can be further enhanced by using inorganic salts such as KI that affect inhibition protectiveness through synergism.238–240 Because of their huge availability at the cost-effective and commercial level, the development and consumption of plant extracts are increasing day by day. Extracts of several parts of plants such as leaves, barks, fruits, peels, flowers, etc. are widely used as corrosion inhibitors.241 Generally, extracts of plants are prepared in a biphasic system consisting of an organic and an aqueous phase. A schematic illustration of the preparation of extracts is shown in Fig. 10. Before application, the prepared plant extracts should be stored in a refrigerator at low temperature. Table 1 presents a summary of some major works on extracts as corrosion inhibitors.
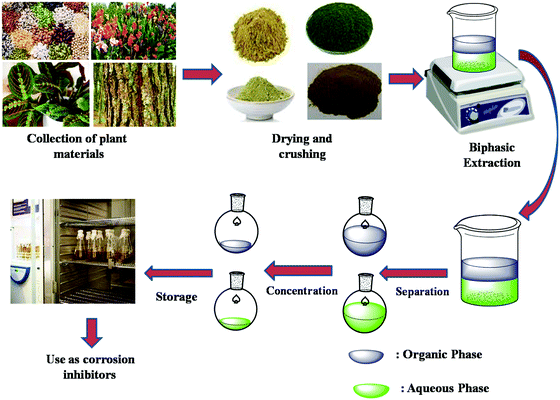 |
| Fig. 10 Schematic illustration of the collection, drying, crushing, separation, extraction, concentration and storage of plant extracts. | |
Among the different parts of plants, the extracts of leaves are most commonly tested as they showed the highest protection efficiency. In most of the plants, the synthesis of phytochemicals mostly takes place in leaves and therefore leaves are the part of plants that are richest in phytochemicals.
Similar to organic corrosion inhibitors, phytochemicals are generally rich in electron donor sites called active or adsorption sites. These electron rich sites of phytochemicals include polar functional groups and multiple bonds. Using these electron rich sites, phytochemicals form strong bonding with the metallic surface, especially through coordination bonding, and behave as strong ligands. Table 3 presents a collection of major leaf extracts evaluated as corrosion inhibitors. Mehdipour et al.242 reported the corrosion inhibition effect of aloe vera leaf extract as an effective corrosion inhibitor for stainless steel in sulfuric acid medium using electrochemical impedance spectroscopy (EIS), potentiodynamic polarization and scanning electron microscopy (SEM). The polarization study revealed that the extract retards both anodic and cathodic reactions and behaves as a mixed-type corrosion inhibitor. However, the authors observed slight anodic predominance. EIS studies showed that the extract becomes effective by controlling the charge transfer process. This finding suggested that the extract acted as an interface-type corrosion inhibitor as it becomes effective by adsorbing at the interface of the metal and electrolyte. The SEM analysis showed that, in the absence of the extract, the metallic surface was extremely damaged because of the unrestricted acidic attack. However, in the presence of the extract, the metallic surface became smooth and was protected significantly. This observation suggested that the phytochemicals present in the extract adsorbed and formed a corrosion protective film over the metallic surface. A similar finding has also been reported using plant extracts as corrosion inhibitors for metals and alloys in various electrolytes (Table 3).
Table 3 Chemical name and metal and electrolyte system of some plant extracts evaluated as corrosion inhibitors
Plant name |
System |
Ref. |
Plant name |
System |
Ref. |
Leaf extracts as corrosion inhibitors in H2SO4 |
Aloe vera
|
Fe/1 M H2SO4 |
242
|
Spondias cytherea
|
Fe/5 M H2SO4 |
243
|
Citrus aurantium
|
Fe/1 M H2SO4 |
244
|
Combretum bracteosum
|
Fe/2& 5 M H2SO4 |
245
|
Sida acuta (leaf and stem)
|
Fe/1 M H2SO4 |
246
|
Hyptis suaveolens
|
Fe/1 M H2SO4 |
247
|
African parquetina
|
Fe/2 M H2SO4 |
248
|
Polyalthia longifolia
|
Fe/1 M H2SO4 |
249
|
Emblica leaves
|
Fe/2 M H2SO4 |
250
|
Buddleia perfoliata
|
Fe/0.5 M H2SO4 |
251
|
Cassia tora
|
Fe/0.5 M H2SO4 |
252
|
Bambusa glaucescens
|
Fe/2 M H2SO4 |
253
|
Nicotiana tabacum
|
Fe/2 M H2SO4 |
254
|
Vernonia amygdalina
|
Fe/1.5 M H2SO4 |
255
|
Mango (Mangifera indica)
|
Fe/1 M H2SO4 |
256
|
Cassava (Manihot esculenta)
|
Fe/0.5 M H2SO4 |
257
|
Achyranthes aspera
|
Fe/0.5 M H2SO4 |
258
|
Oxystelma esculentum
|
Fe/0.5 M H2SO4 |
259
|
African breadfruit
|
Fe/1 M H2SO4 |
260
|
Stachytarpheta indica
|
Fe/1 M H2SO4 |
261
|
Jatropha curcas
|
Fe/0.5 M H2SO4 and 0.5 M NaCl |
262
|
Sida acuta
|
Fe/1 M H2SO4 |
263
|
Wrightiatinctoria, Clerodendrumphlomidis, Ipomoeatriloba
|
Fe/0.5 M H2SO4 |
264
|
Nauclea latifolia (leaf, bark, root)
|
Fe/1 M H2SO4 |
265
|
Leaf extracts as corrosion inhibitors in HCl |
Olea europaea
|
Fe/2 M HCl |
266
|
Azadirachta excelsa
|
Fe/1 M HCl |
267
|
Tabernaemontana divaricata
|
Fe/1 M HCl |
268
|
Boscia senegalensis
|
Fe/1 M HCl |
269
|
Phyllanthus amarus
|
Fe/1 M HCl |
270
|
Acalypha indica
|
Fe/1 M HCl |
271
|
Eleusine aegyptiaca and Croton rottlerin
|
Fe/1 M HCl |
272
|
Phoenix dactylifera
|
Fe/1 M HCl |
273
|
Pimenta dioica
|
Fe/0.5 M and 1.0 M HCl |
274
|
Ruta graveolens
|
Fe/1 M HCl |
275
|
Thyme
|
Fe/2 M HCl |
276
|
Annona muricata L.
|
Fe/1 M HCl |
277
|
(Guatteria ouregou and Simira tinctoria)
|
Fe/1 M HCl |
278
|
Michelia alba
|
Fe/mild steel 1 M HCl |
279
|
Morusalba pendula
|
Fe/1 M HCl |
280
|
Anthocleista djalonensis
|
Fe/1 M HCl |
281, 282
|
Ficus hispida
|
Fe/1 M HCl |
283
|
Morinda tinctoria
|
Fe/1 M HCl |
282
|
Solanam xanthocarpum
|
Fe/1 M HCl |
284
|
Vitex negundo
|
Fe/1 M HCl |
285
|
Gliricidia sepium
|
Fe/1 M HCl |
286
|
Gymnema sylvestre
|
Fe/1 M HCl |
287
|
Rosmarinus officinalis L.
|
Fe/0.1 M HCl |
288
|
Argemone mexicana
|
Fe/1 M HCl |
289
|
Eichhornia crassipes
|
Fe/5 M HCl |
290
|
Acalypha torta
|
Fe/1 M HCl |
291
|
Murraya koenigii (curry leaves)
|
Fe/1 M HCl |
292
|
Podranea ricasoliana
|
Fe/1 M HCl |
293
|
Petroselinum crispum (parsley), Eruca sativa (Arugula), Anethum graveolens
|
Fe/1 M HCl |
99
|
Passiflora foetida
|
Fe/1 M HCl |
294
|
|
Emblica officinalis
|
Fe/1 M HCl |
295
|
Funtumia elastica
|
Fe/1 M HCl |
296
|
Pterocarpus soyauxii
|
Fe/1–2.5 M HCl |
297
|
Tithonia diversifolia
|
Fe/1 M HCl |
298
|
Plumeria alba, Blighia sapida, Secamone afezeli
|
Fe/1 and 2 M HCl |
299
|
Gnetum africana
|
Fe/1 M HCl |
300
|
Emblica officinalis
|
Fe/1 M HCl |
301
|
Lactuca sativa, etc. |
Fe/1 M HCl |
302
|
Sesbania sesban
|
Fe/1 M HCl |
303
|
Bryophyllum pinnatum
|
Fe/0.5 M HCl |
304
|
Sansevieria trifasciata
|
Al/2 M HCl & 2 M KOH |
305
|
Piper longum
|
Al/1 M NaOH |
306
|
Gossypium hirsutum (leaf and seed)
|
Al/2 M NaOH |
307
|
Nicotiana tabacum
|
AA3003Al/0.1 M HCl |
308
|
Phyllanthus amarus
|
Al/2 M NaOH |
309
|
Cannabis sativa (CS)
|
Al/1 M NaOH |
310
|
Jasminum nudiflorum Lindl.
|
Al/1 M HCl |
311
|
Vernonia amygdalina
|
Al/1 M HCl |
312
|
Raphia hookeri
|
Al/0.02–2 M HCl |
223
|
Tender arecanut
|
Al/0.5 M HCl |
313
|
Thymus algeriensis
|
Al/1 M HCl |
314
|
Breadfruit
|
Al/0.5 M H2SO4 |
260
|
Cocos nucifera
|
Al/0.5 M HCl |
315
|
Maesobatrya barteri
|
Al/0.5 M and 2 M HCl |
316
|
Morinda tinctoria
|
Al/0.5 M HCl |
317
|
|
|
|
2.3. Chemical medicines as environment-friendly alternatives
Chemical medicines or drugs represent another class of environment-friendly alternatives to the traditional toxic corrosion inhibitors. Several reports dealing with the inhibition effect of drugs have been published.318–321 Chemical medicines are ideal environment-friendly alternatives as they are derived from biological and natural resources. Further, most of the drugs are bio-tolerable, bio-compatible and non-bioaccumulative in nature and therefore they offer environmentally sustainable alternatives.322,323 Generally, drugs are complex molecules with several electron rich centers in the way of polar functional groups and multiple bonds through which they acquire a high anticorrosion ability. Because of their macromolecular nature, most of the drugs provide a large surface coverage and a high protection efficiency. Possessing numerous polar functional groups along with enhanced protection efficiency, they synergize the solubility of drug molecules in polar electrolytes. In view of their high efficiency, high solubility in polar electrolytes and environmental sustainability, numerous classes of drug molecules are evaluated as effective corrosion inhibitors for numerous metals and alloys in versatile electrolytes. Although the use of chemical medicines is an environmentally sustainable alternative method of corrosion inhibition, most of the drug molecules are highly expensive. Their high cost is associated with their multi-step synthesis and ultra-purification processes. In view of this, corrosion scientists have started to use expired drugs as corrosion inhibitors.324,325 Most of the expired drugs are thrown out as they are useless after their expiry. Although the application of such drugs in the biological system is not possible, they can be effectively used in industries for various applications. One of the greatest uses of expired drugs is in metallic corrosion inhibition. The use of expired drugs as corrosion inhibitors represents an environment-friendly and cost-effective approach.325 Because of this, several articles and research papers have been published on expired drugs as corrosion inhibitors.326,327 Various new and expired drugs are tested as effective corrosion inhibitors for different metals and alloys in various electrolytes. A summary of some major reports on the anticorrosive effect of drug molecules is provided in Table 4. Most of the drug molecules contain highly complex structures that enable them to be effective species to act as good corrosion inhibitors. They become effective by adsorbing on the metallic surface. The adsorption of drug molecules on the metallic surface mostly followed the Langmuir adsorption isotherm model. Using potentiodynamic polarization and electrochemical impedance spectroscopy (EIS), it can be observed that drug molecules mostly behaved as mixed- and interface-type corrosion inhibitors. The adsorption of drug molecules on the metallic surface is mainly supported by SEM, AFM and FT-IR spectroscopy studies.
Table 4 Chemical structures and names of some major drugs that have been tested as corrosion inhibitors for different systems
Drug name and structure |
System |
Ref. |
Drug name and structure |
System |
Ref. |
|
Fe/1 M HCl |
328
|
|
Fe/1 M HCl |
329
|
|
Fe/1 M HCl |
330
|
|
Fe/0.5 M HCl |
331
|
|
Fe/1 M HCl |
332
|
|
Fe/1 M HCl |
333
|
|
Fe/1 M HCl |
334
|
|
Fe/1 M HCl |
335
|
|
Fe/1 M HCl |
336
|
|
Fe/1 M HCl |
324
|
|
Fe/0.1 M H2SO4 |
337
|
|
Fe/1 M HCl |
338
|
|
Fe/1 M HCl |
339
|
|
Fe/2.5 M HCl |
340
|
|
Fe/1 M HCl |
341
|
|
Fe/2.5 M HCl |
340
|
|
Fe/0.1 M H2SO4 |
342
|
|
Fe/1 M HCl |
343
|
|
Fe/1 M HCl |
344
|
|
Fe/1 M HCl |
345
|
|
Fe/1 M HCl |
346
|
|
Fe/1 M HCl |
347
|
|
Fe/1 M HCl |
348
|
|
Fe/1 M HCl |
349
|
|
Fe/1 M HCl |
350
|
|
Fe/1 M HCl |
351
|
|
Fe/1 M HCl |
352
|
|
Fe/1 M HCl |
353
|
|
Fe/1 M HCl |
354
|
|
Fe/1 M HCl |
355
|
|
Fe/1 M HCl |
356
|
|
Fe/15% HCl |
357
|
2.4. Ionic liquids as environment-friendly corrosion inhibitors
Ionic liquids are salts in a liquid (molten) state.358 Ionic liquids are composed of organic cations and inorganic anions and they are liquid at or below 100 °C.359,360 Ionic liquids are also called ionic glasses, ionic melts, liquid fluids, ionic electrolytes or fused salts. Ethylammonium nitrate (MP: 12 °C) was the first reported ionic liquid (in 1914).361,362 After that, several classes of ionic liquids have been developed and used for industrial and biological applications.363–366 Ionic liquids are characterized by their ability to dissolve a wide range of organic and inorganic compounds. Because of their association with several environment-friendly properties including low toxicity, low melting point, high polarity, low vapor pressure and high resistivity for thermal and chemical treatment, ionic liquids are ideal environmentally benign alternatives to the traditional corrosion inhibitors.57,367,368 Unlike traditional organic compounds, ionic liquids are readily soluble in polar electrolytes and manifest reasonably high protection effectiveness. Ionic liquids are rightly called designer corrosion inhibitors as their inhibition effect can be suitably tailored using a proper combination of cations and anions.9,57 The chemical structures of major cations and anions are presented in Fig. 11. Ionic liquids can be classified as neutral, basic, acidic, functionalized or supported type.369–371
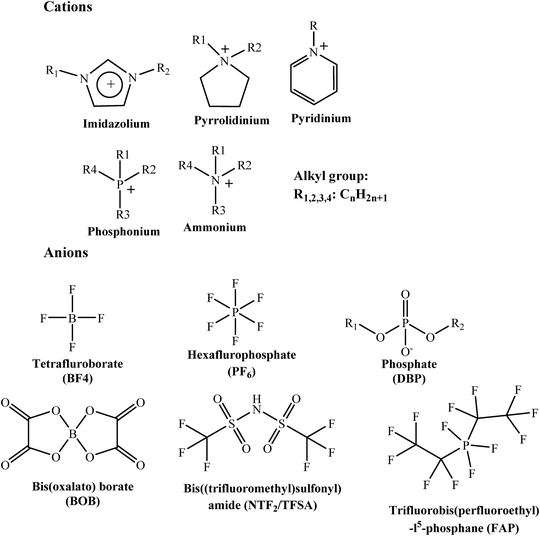 |
| Fig. 11 Chemical structures of some common cations and anions of ionic liquids that can be used for anticorrosive applications. | |
The literature study showed that several classes of ionic liquids are used as effective corrosion inhibitors for different metals and alloys.57,367,372–374 Imidazolium based ionic liquids are most frequently used as corrosion inhibitors.368,375–377 Likhanova et al.378 proposed that the cationic and anionic moieties of ILs differently adsorb on cathodic and anodic active sites and inhibit the corrosion. The cationic part of ionic liquids mainly adsorbs on cathodic sites and anionic moieties mainly adsorb at anodic sites. While studying the corrosion inhibition effect of three imidazolium based liquids for mild steel corrosion in 1 M HCl, the authors observed that the inhibition effect was largely dependent on the nature of anions present in the investigated ionic liquids.379 Imidazolium based ionic liquids interact with the metal surface through donor–acceptor interactions and acquire planar orientations. The polarization study showed that the tested ionic liquids behaved as mixed-type corrosion inhibitors, i.e. the tested ionic liquids adversely affect the rate of cathodic and anodic Tafel reactions. Polarization curves for mild steel corrosion in 1 M HCl with and without the evaluated ionic liquids are shown in Fig. 12. The observation of Fig. 12 shows that the shapes of both anodic and cathodic curves are affected by the presence of ionic liquids which validated the finding that ionic liquids adversely affect the rate of corrosion reactions. The adsorption mechanism was reinforced using SEM and AFM studies. SEM and AFM micrographs of the mild steel surface corroded in 1 M HCl for 3 h are shown in Fig. 13. It can be seen from the SEM and AFM images of the black specimen that the surface of the specimen is highly corroded because of free acid attack. However, in the presence of ionic liquids, the surface morphologies of the metallic specimens are greatly improved because of their anticorrosive effect. The order of metallic surface smoothness is consistent with the order of corrosion inhibition effect of ionic liquids. The average surface roughness of non-protected metallic surface was 372 nm. The average surface roughness in the presence of [bmim][Cl], [bmim][CF3SO3] and [bmim][Ac] 187, 134 and 114 nm, respectively.
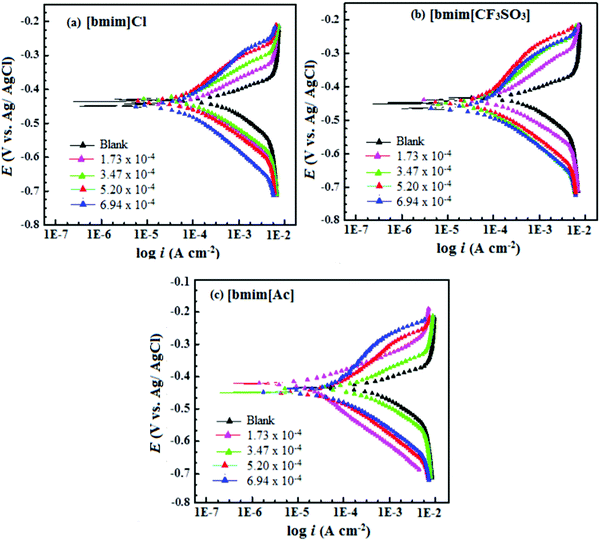 |
| Fig. 12 Potentiodynamic polarization curves for mild steel corrosion in 1 M HCl in the absence and presence of (a) [bmim][Cl], (b) [bmim][CF3SO3] and (b) [bmim][Ac] at different concentrations.379 | |
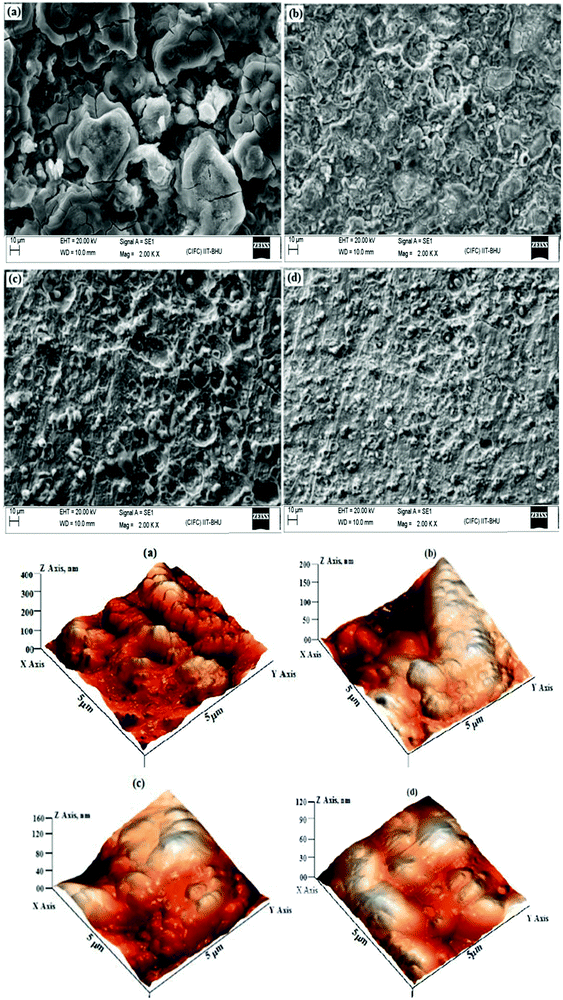 |
| Fig. 13 SEM (above) and AFM (below) micrographs of the mild steel surface in the (a) absence and presence of (b) [bmim][Cl], (c) [bmim][CF3SO3] and (d) [bmim][Ac] at different concentrations.379 | |
Table 5 presents a summary of some major reports published on the anticorrosive effect of imidazolium based ionic liquids.380–401 Several experimental and computational methods are employed to demonstrate the anticorrosive effect of imidazolium ionic liquids. It can be observed that most of the ionic liquids become effective against metallic corrosion by adsorbing on the metallic surface. Adsorption of imidazolium ionic liquids mostly follows the Langmuir adsorption isotherm; however, other adsorption isotherm mechanisms have also been reported. Adsorption of ionic liquids results in an increase in the value of charge transfer resistance that occurs in the form of an effective anticorrosive barrier. Most of the evaluated ionic liquids behave as interface-type corrosion inhibitors. Imidazolium based ionic liquids containing hydrophobic alkyl chain(s) exhibit a reasonably good anticorrosive effect relative to those without hydrophobic chain(s). In this case, it can be expected that the polar and electron rich hydrophilic imidazole ring interacts with the metal surface and forms a protective film, whereas hydrophobic alkyl chains float and prevent the water from coming into contact with the metal surface. Therefore, an increase in the length of the hydrophobic chain is expected to increase the inhibition effect of ionic liquids. Nevertheless, an increase in hydrophobicity can adversely affect the solubility of such ionic liquids in polar electrolytes. Therefore, a proper combination of hydrophilicity and hydrophobicity is highly essential while designing effective corrosion inhibitors.389,402 The anticorrosive effect of pyridinium,378,403–405 pyrrolidinium,406–408 ammonium9,409–412 and phosphonium409,413 based ionic liquids has also been reported in the literature.
Table 5 Chemical structures, abbreviation, nature of adsorption, metal and electrolyte system, method of corrosion measurements, highest inhibition efficiency and optimum concentration of some ionic liquids evaluated as corrosion inhibitors
S. no. |
Chemical structure and abbreviation |
Nature of adsorption |
Electrolyte and metal |
Method of corrosion monitoring |
IE% and conc. |
Ref. |
1 |
|
Langmuir isotherm, mixed type |
1 M HCl/carbon steel |
WL, PDP, EIS, SEM, AFM & CA |
78.7% at 100 mg L−1 |
380
|
2 |
|
— |
Open/A36 mild steel |
WL, EIS, PDP, SEM & DFT |
— |
381
|
3 |
|
Langmuir isotherm, mixed type |
1 M HCl/mild steel |
WL, PDP & EIS |
92% at 20 mM |
382
|
4 |
|
Langmuir isotherm, mixed type |
1 M HCl & 1 M H2SO4/API 5LX52 steel |
PDP, EIS & SEM |
DBImL (30%: H2SO4; 88% HCl) |
383
|
5 |
|
— |
0.01 M NaCl/mild steel |
EIS, PDP, SEM, OM and FT-IR |
86% MeHImn 4-OHC (4 mM) |
385
|
6 |
|
Langmuir adsorption isotherm |
1 M H2SO4/304 S steel |
WL, PDP, EIS & SEM |
[BSMIM][HSO4] (98.48%) & [BSMIM][BF4] (98.29%) at 10−2 M |
386
|
7 |
|
— |
NaCl (3.8 & 6.8 pH)/mild steel |
OCP, EIS, PDP, SEM, EDX and XPS |
98.2% (3.8 pH) & 84.3% (6.8 pH) |
387
|
8 |
|
Flory–Huggins isotherm, mixed type |
0.5 M H2SO4/mild steel |
WL, PDP, EIS, SEM & DFT |
76.9% at 10 mM |
388
|
9 |
|
Langmuir isotherm, mixed type |
1 M H2SO4/carbon steel |
WL, PDP, SEM, EDX & AFM |
90% at 10−4 M (IL4) |
389
|
10 |
|
— |
Ethanol/tinplate |
PDP, SEM and XPS |
I
corr = 2.33 × 10−7 A cm−2 |
390
|
11 |
|
Mixed type inhibitors |
Cast iron/gulf sea water |
WL, OCP, PDP and EIS |
R
p = 1376 ([EMIm]) & 1364 ([Py1,4]) at 5 mM |
391
|
12 |
|
Langmuir isotherm, mixed type |
1 M HCl/carbon steel |
WL, PDP & EIS |
92.3% (PPIB1) & 94.2% (PPIB4) at 10−2 |
392
|
13 |
|
Langmuir isotherm |
1 M HCl/mild steel |
WL and DFT |
94.6% (MA1) & 97.6% (MA2) at 10−2 M |
393
|
14 |
|
Langmuir isotherm, mixed type |
1 M HCl/mild steel |
WL, PDP, EIS, SEM, AFM, EDX & UV-vis |
66.2% [C16M1Im] [Br] & 71.8% [C16M2Im] [Br] at 250 ppm |
394
|
15 |
|
Langmuir isotherm, mixed type |
15% HCl/N80 steel |
STT, WL, EIS, PDP, SEM, AFM, DFT & MDS |
75.3% [DDMIM]C & 98.1% [BDMIM]Cl at 100 ppm |
395
|
16 |
|
Langmuir isotherm, mixed type |
0.5 M H2SO4/mild steel |
PDP, EIS, SEM, AFM, DFT & MDS |
98.9% at 0.01 M (298 K) |
396
|
17 |
|
Langmuir isotherm, mixed type |
0.5 M HCl/carbon steel |
PDP, EIS, CA, SEM, UV-vis, XPS, DFT and MDS |
80.8% (C2-IMIC4-S) & 97.9% (C10-IMIC4-SR = –C10H21) |
397
|
18 |
|
Langmuir isotherm, mixed type |
0.5 MH2SO4/API 5 L X52 |
WL, OCP, EIS, PDP, SEM, E-DX, UV, XPS, AFM, DFT & MDS |
93.1% at 10 ppm (55 °C) |
398
|
19 |
|
Langmuir isotherm, mixed type |
0.5 M HCl & H2SO4/API 5 L X52 |
WL, EIS, PDP, SEM-EDX, AFM, XPS & MDS |
69% (HCl) & 90 (H2SO4) at 75 ppm |
399
|
20 |
|
EI-Awady kinetic-thermodynamic, mixed type |
1 M HCl/Q235 carbon steel |
WL, PDP, EIS, CA, SEM, EDX and MDS |
90.53%: [VAIM]PF6) & 54.01 [VA-IM]PF4) 0.8 mM |
400
|
21 |
|
Langmuir isotherm, mixed type |
0.01 M Na2SO4/copper |
WL, PDP, EIS, CA, SEM, |
91.29% at 100 ppm |
401
|
Because of their high solubility and environmentally benign nature ionic liquids are also tested as anticorrosive agents for other metals and alloys. Zhang and Hua414 demonstrated three imidazolium based ionic liquids designated as BMIC, HMIC and OMIC for aluminum in 1 M HCl using chemical and electrochemical methods. BMIC, HMIC and OMIC inhibit Al corrosion by adsorbing on the metallic surface, which followed the Langmuir adsorption isotherm model. The inhibition effect followed the order: BMIC < HMIC < OMIC. All the tested ionic liquids acted as mixed type corrosion inhibitors. In another report,415 the anticorrosive effect of an ionic liquid, designated as OPEIB, was evaluated for the 6061 Al-15 alloy in 0.5 M H2SO4 using electrochemical and surface analyses. OPEIB acted as a mixed type inhibitor and its adsorption obeyed the Temkin adsorption isotherm model. The anticorrosive effect of ionic liquids for aluminum including the inhibition effect of three poly(ionic liquids) for the 6061 Al-15 alloy in 0.1–1 M HCl416 and quaternary ammonium based surfactants (CmC6Cm(Et)·2Br: m = 10, 12, 14, 16) for the Al/1 M HCl system,417etc. has been widely reported in the literature.418–420 Ionic liquids are also widely used as corrosion inhibitors for zinc421,422 and copper401,422–424 in various electrolytes. In most of the studies, ionic liquids acted as mixed-type corrosion inhibitors and their adsorption followed the Langmuir adsorption isotherm model. Using the EIS technique, it was observed that most of the investigated ionic liquids behaved as interface-type corrosion inhibitors as they become effective by forming a protective film over the metallic surface.
2.5. Polyethylene glycols as environment-friendly corrosion inhibitors
Polyethylene glycols (PEGs) represent a special class of industrially and biologically useful compounds. PEGs are connected with several advantages including low flammability, vapor pressure, cost and environmental toxicity.425 PEGs are available in different molecular weights varying from 200 to tens of thousands.425,426 Low molecular weight PEGs (200–600 D) are in the liquid form at room temperature. PEGs having a molecular weight of 600–800 D are water soluble viscous materials. PEGs having a molecular weight greater than 800 D are in the form of solids. The solubility of PEG polymers decreases with the increase in the molecular weight as PEGs-200–600 D are fully soluble in water, whereas PEG-2000 shows only 60% solubility (at 20 °C) in water. Because of their environment-friendly nature, biocompatibility, high molecular weight and great solubility in polar electrolytes, PEGS are widely used as corrosion inhibitors for metals and alloys. Because of their polymeric nature, PEGs provide massive surface coverage and protection and therefore behave as effective corrosion inhibitors. Although several review articles dealing with the various aspects of PEGs are published, no review on the anticorrosive effect of PEGs has been published yet.425,427–429 The literature survey demonstrates that PEGs possess a strong ability to interact with the metallic surface as they form strong coordination bonding with the metallic substrate.430 Unlike the traditional corrosion inhibitors, PEGs are nonvolatile, nonflammable and biodegradable which are the requirements for being environmentally benign.431–433 PEGs are relatively stable for chemical and thermal treatments and therefore they can be used as effective corrosion inhibitors at high temperature over a wide pH range.434–436 PEGs are almost insusceptible to H2O2 oxidation and reduction via NaBH4. PEGs possess the ability to recover from the medium they use.437,438
PEGs are widely employed as corrosion inhibitors in different electrolytes. Ashassi-Sorkhabi and Ghalebsaz-Jeddi439 reported the anticorrosive properties of PEGs with molecular weights in the range of 200–10
000 g mol−1 for carbon steel in 3 N using chemical and electrochemical methods. It was observed that PEGs exhibited greater than 90% efficiency at a 10−1 M concentration. The interfacial behavior of PEGs was determined using the EIS method. These authors also reported the anticorrosive effect of PEGs-400–10
000 g mol−1 under the same experimental conditions (carbon steel/3 N H2SO4).440 SEM analyses revealed that the presence of PEGs enhances the smoothness of the surface and this enhancement was consistent with the molecular weight of PEGs. Umoren et al. extensively studied the corrosion inhibition effect of PEG in correlation with the anticorrosive effect of polyvinyl alcohol (PVA) and natural polymer (gum Arabic; GA).441–444 They observed that PEG exhibited a better anticorrosive effect. The adsorption of PEG and GA obeyed the Temkin adsorption isotherm model. The authors observed a synergistic effect in the presence of halide ions and their relative order of effectiveness is as follows: KI > KBr > KCl. The corrosion inhibition effect of PEG with halide ions has been reported elsewhere.445 The literature survey showed that PEGs are widely used as inhibitors for metals in H2SO4 medium.446–448
PEGs are also used as metallic corrosion inhibitors in HCl medium. Ashassi-Sorkhabi et al.449 manifested the inhibition properties of PEGs (400–10
000 g mol−1) for carbon steel in 0.5 M HCl. PEGs behave as mixed-type corrosion inhibitors. An analysis in which the comparative inhibition effect of PEG and ciprofloxacin is evaluated for aluminum in acidic medium showed that PEG showed better protection effectiveness.450 The presence of ciprofloxacin synergistically enhanced the protection effect of ciprofloxacin. In another study Awad et al.451 demonstrated the anticorrosive properties of PEGs (300–600) for aluminum in the acidic medium of HCl. The results showed that PEGs behaved as mixed-type inhibitors. Recently, the inhibition effect of chitosan modified PEG was reported for mild steel in 1 M HCl.145 PEGs are also widely used as corrosion inhibitors in NaCl452–454 and MOH (metal oxide)455–457 solutions.
2.6. Environment-friendly alternatives derived from MCRs
The use of organic compounds is one of the most profitable methods of corrosion mitigation. However, most of the synthetic inhibitors are toxic in nature because they are synthesized using toxic chemicals, catalysts and solvents.13,458 Nevertheless, corrosion inhibitors synthesized using one-step multicomponent reactions (MCRs) have recently gained particular advancement towards the development of environmentally benign synthesis methods for chemicals to be used for different industrial applications including corrosion inhibition. MCRs possess several advantages that make them environment-friendly.459–462 Unlike the synthesis of traditional toxic corrosion inhibitors, MCRs are easy to proceed, profitable, and are associated with high yield and selectivity. In fact, in association with ultrasound (US) and microwave (MW) energy heating, MCRs have emerged as one of the greenest synthetic protocols.462 MCRs are characterized by chemical syntheses that employ the reactions between three or more reactants in one step. These types of reactions are generally quick, highly selective and consume less time to complete. Several compounds synthesized using MCRs are evaluated as inhibitors against metallic corrosion inhibitors. The synthetic schemes of MCRs for some classes of corrosion inhibitors are shown in Table 4. A careful observation showed that most of the corrosion inhibitors derived from MCRs are heterocyclic compounds, especially those containing N and O heteroatoms. It can be observed from the table that nitrogen based heterocyclic compounds such as imidazole, indole, pyridine, etc. are derived using MCRs that offer strong bonding with the metallic surface. On this basis, it can be concluded that MCRs act as a potential medium for the synthesis of heterocyclic compounds that can be used as corrosion inhibitors. Along with MCR syntheses, mechanochemical mixing (MCM)463,464 and solid supported syntheses (SSSs)465,466 are also regarded as environment-friendly synthetic approaches because of their easy application, cost-effective nature and easy and lower number of purification and work-up steps (Table 6).
Table 6 Synthetic schemes for some major corrosion inhibitors derived through MCRs
MCR synthetic scheme |
System |
Ref. |
MCR synthetic scheme |
System |
Ref. |
|
Fe/15% HCl, |
467
|
|
Fe/1 M HCl |
468
|
|
Fe/1 M HCl |
469
|
|
Fe/1 M HCl |
470
|
|
Fe/1 M HCl |
471
|
|
Fe/1 M HCl |
472
|
|
Fe/1 M HCl |
23
|
|
Fe/1 M HCl |
16
|
|
Fe/1 M HCl |
17
|
|
Fe/1 M HCl |
473
|
|
Fe/1 M HCl |
75
|
|
Fe/1 M HCl |
474
|
|
Fe/1 M HCl |
77
|
|
Fe/1 M HCl |
475
|
|
Fe/1 M HCl |
53
|
|
Fe/1 M HCl |
476
|
|
Fe/1 M HCl |
60
|
|
Fe/0.5 M HCl |
477
|
|
Fe/1 M HCl |
478
|
|
Fe/0.5 M HCl |
479
|
|
Fe/1 M HCl |
480
|
|
N80/15% HCl |
481
|
|
Fe/1 M HCl |
482
|
|
N80/15% HCl |
483
|
|
Fe/1 M HCl |
484
|
|
Fe/15% HCl |
468
|
|
Fe/1 M HCl |
485
|
|
Fe/1 M HCl |
486
|
|
Fe/1 M HCl |
487
|
|
Fe/1 M HCl |
488
|
|
Fe/1 M HCl |
489
|
|
N80/15% HCl |
73
|
|
Fe/1 M HCl |
490
|
|
Fe/1 M HCl |
491
|
|
Fe/1 M HCl |
492
|
|
Fe/1 M HCl |
493
|
|
Fe/1 M HCl |
494
|
|
Fe/1 M HCl |
495
|
|
N80/15% HCl |
496
|
|
Fe/1 M HCl |
497
|
|
Cu/0.5 M HCl |
498
|
|
Fe/1 M HCl |
499
|
|
N80/15% HCl |
500
|
|
|
91
|
|
N80/15% HCl |
501
|
|
|
502
|
2.7. Environment-friendly alternatives derived from US and MW irradiations
Generally, conventional heating methods offer very slow and non-uniform heating of reaction vessels that allow slow activation of the reactant molecules.503,504 Therefore, completion of the reactions carried out using conventional heating methods requires several minutes, hours or even days to complete. In the last three decades, the use of non-conventional heating sources mainly through ultrasound (US) and microwave (MW) irradiations gained significant attention.505,506 Chemical reactions using these non-conventional heating methods can proceed within a fraction of a second or minute, which require several hours to complete when using conventional heating methods. The literature study showed that MW and US irradiations cause sudden and instantaneous heating that activates most of the reactant molecules together and the reaction proceeds quickly.507–509 The MW and US irradiations are associated with several advantages that are closely related with the principles of green chemistry. Several theories are proposed to describe the activation of reactant molecules using MW and US irradiations. Generally, MW/US irradiation is used to catalyze MCR reactions.510,511 In connection with MCRs, US and MW irradiations offer the greenest synthetic protocol. Along with increasing the rates of chemical reactions, US and MW irradiations can also enhance the selectivity of the reactions.512,513 Moreover, MW and US irradiations also act as catalysts for chemical transformations. Several biologically and industrially useful compounds, especially heterocyclic compounds, are synthesized using MW and US irradiations. Heterocyclic compounds derived from MW and US irradiations have also been evaluated as inhibitors against metallic corrosion.12 Synthetic schemes and chemical structures of some major heterocyclic compounds derived from MW or US irradiation are presented in Table 5. The observation of the table shows that MW/US catalyzed reactions are related with the synthesis of heterocyclic compounds. Although chemical transformations can be catalytically and efficiently catalyzed using MW/US irradiation, their implementation for the synthesis of corrosion inhibitors should be further explored (Table 7).
Table 7 Synthetic schemes and chemical structures of some major heterocyclic compounds synthesized using MW or US irradiation and tested as corrosion inhibitors
Synthetic scheme |
System |
Ref. |
|
Fe/1 M H2SO4 |
514
|
|
Fe/1 M HCl |
515
|
|
Fe/20% H2SO4 |
516
|
|
Fe/1 M HCl |
378
|
|
Fe/H2S medium |
517
|
|
Al-3Mg/0.5 M and 1 M HCl |
518
|
|
Fe/1 M H2SO4 |
60
|
|
Fe/1 M HCl |
495
|
|
Fe/1 M HCl |
493
|
|
Fe/1 M HCl |
519
|
|
Fe/1 M HCl |
520
|
2.8. Environment-friendly alternatives derived using green solvents
Solvents play a significant role in the progress of numerous reactions.521 There are several aspects of green chemistry and green synthesis, one of which is the use of environmentally benign and inexpensive solvents. It is well established that polar protic solvents such as alcohol (ROH) and amines (RNH2, R2NH and R3N) favor SN1 reactions and polar aprotic solvents such as THF (tetrahydrofuran), DMSO (dimethyl sulfoxide), and DMF (dimethyl formamide) favor SN2 reactions.522,523 Nevertheless, most of the traditional solvents used for chemical transformations are toxic and non-environment-friendly in nature.524,525 Generally, after their use, they are discharged into the surrounding environment without adequate treatment, thereby causing pollution.
Although “the best solvent is no solvent”, there are some environmentally benign alternatives that can be used for the synthesis of organic compounds in the place of traditional toxic and volatile solvents.431 One of such solvents is water. Water is regarded as the best alternative to the traditional toxic solvents. Because of its bio-susceptibility, cost-effectivity, unique redox potential, high dielectric constant and huge availability, water represents one of the greenest solvents for chemical transformations.524–526 Several chemical transformations have been carried out using water as a solvent. The literature study showed that water has also been used as a medium for the synthesis of compounds used as corrosion inhibitors.
Toxicity of most of the traditional organic solvents is attributed to their high vapor pressure. Therefore, chemical compounds with a lower vapor pressure that exist in a wide liquid range can also be used as environmentally benign solvents for chemical transformations. One such example is ionic liquids. The high polarity, low vapor pressure and high chemical and thermal stability of ionic liquids make them one of the best alternative environment-friendly solvents.521,527 Several transformations have been carried out in ionic liquids to be used as effective corrosion inhibitors for metals and alloys.57,528 In fact, ionic liquids can themselves be effectively used as corrosion inhibitors for different systems. Because of their ionic nature, most of the ionic liquids are freely soluble in aqueous media and therefore they are mainly used as aqueous phase corrosion inhibitors,529,530 although they have also been tested as corrosion inhibitors in the coating phase. The inhibition effectiveness of ionic liquids can be suitably tailored by proper selection of cations and/or anions. In this sense, ionic liquids can be regarded as designer corrosion inhibitors. Imidazolium and pyridinium based ionic liquids are extensively used as corrosion inhibitors; however, other classes of ionic liquids are also used.57
Another such alternative is supercritical CO2 that has very low vapor pressure; however, it is not a good solvent for high molecular weight compounds.531,532 Therefore, supercritical CO2 is mostly used in combination with other cosolvents (polar and non-polar). CO2 philic (loving) ligands and highly CO2 soluble surfactants can also be used to enhance the solubility of supercritical CO2.533,534 Supercritical CO2 is extensively used for a variety of chemical transformations, as an extraction solvent, and as a solvent in polymer production, purification and processing, powder production and processing and semiconductor processing.
3. Summary and outlook
The present review provides a summary of environmentally sustainable alternatives for traditional toxic corrosion inhibitors. The literature survey shows that several classes of pure and modified natural or bio-polymers have been widely reported as environment-friendly alternatives to traditional toxic corrosion inhibitors. With growing demand for green and sustainable technologies, the implementation of environment-friendly alternatives should be further explored. Among the natural environment-friendly alternatives, plant extracts and chemical medicines (drugs) are frequently used as corrosion inhibitors. Because of their natural and biological origin and property of non-bioaccumulation, they are regarded as environment-friendly alternatives to traditional toxic corrosion inhibitors. Due to their complex molecular structures that contain numerous electron rich centers including polar functional groups and multiple bonds, the drug molecules and phytochemicals present in plant extracts act as effective metallic corrosion inhibitors. Because of their natural and biological origin they are treated as environmentally sustainable chemicals. However, recently the use of drug molecules has been slightly restricted because of their high cost which promoted the idea of consuming expired drugs as environment-friendly corrosion inhibitors. The use of expired drugs as corrosion inhibitors should be further explored. Currently, compounds derived through multicomponent reactions (MCRs), mechanochemical mixing (MCM), and solid supported syntheses (SSSs) and chemicals derived through microwave (MW) and ultrasound (US) irradiations are widely used as environment-friendly alternatives to traditional toxic corrosion inhibitors. These synthetic methods provide several advantages over the traditional multistep reactions (MSRs), such as high yields, low reaction times, high selectivity, easy execution and handling, being cost-effective and a lower number of purification and work-up steps. The literature study shows that numerous environment-friendly alternatives derived from MCRs and MW/US irradiations are tested as corrosion inhibitors; however, the implementation of such types of chemicals should be further enhanced. Apart from the above, there are numerous solvents that are demonstrated as environment-friendly, and chemicals derived using these solvents as reaction media are also regarded as environment-friendly. Examples of such types of solvents include water, ILs, polyethylene glycol (PEG) and supercritical CO2. In the literature, numerous chemicals derived from these solvents are widely used as environment-friendly corrosion inhibitors. Carbohydrates, amino acids (AAs) and their derivatives are other representatives of environment-friendly corrosion inhibitors; however, they are not covered herein as several review articles dealing with their anticorrosion behavior have already been published.
Conflicts of interest
The authors declare that there is no conflict of interest.
References
- J. Güttler and J. Reschke, Metal-carbon layers for industrial application in the automotive industry, Surf. Coat. Technol., 1993, 60, 531–535 CrossRef.
-
J. K. Saha, Corrosion of constructional steels in marine and industrial environment: Frontier work in atmospheric corrosion, Springer Science & Business Media, 2012 Search PubMed.
-
G. Koch, Cost of corrosion, Trends in oil and gas corrosion research and technologies, Elsevier, 2017, pp. 3–30 Search PubMed.
-
S. A. Umoren, M. M. Solomon and V. S. Saji, Corrosion Inhibitors for Sour Oilfield Environment (H2S Corrosion), Corrosion Inhibitors in the Oil and Gas Industry, 2020, pp. 229–254 Search PubMed.
- C. Verma, H. Lgaz, D. Verma, E. E. Ebenso, I. Bahadur and M. Quraishi, Molecular dynamics and Monte Carlo simulations as powerful tools for study of interfacial adsorption behavior of corrosion inhibitors in aqueous phase: a review, J. Mol. Liq., 2018, 260, 99–120 CrossRef CAS.
- N. G. Thompson, M. Yunovich and D. Dunmire, Cost of corrosion and corrosion maintenance strategies, Corros. Rev., 2007, 25, 247–262 CAS.
-
G. H. Koch, M. P. Brongers, N. G. Thompson, Y. P. Virmani and J. H. Payer, Corrosion cost and preventive strategies in the United States, Federal Highway Administration, United States, 2002 Search PubMed.
-
J. De Damborenea, A. Conde and M. Arenas, Corrosion inhibition with rare earth metal compounds in aqueous solutions, Rare Earth-based Corrosion Inhibitors, Elsevier, 2014, pp. 84–116 Search PubMed.
- C. Verma, I. Obot, I. Bahadur, E.-S. M. Sherif and E. E. Ebenso, Choline based ionic liquids as sustainable corrosion inhibitors on mild steel surface in acidic medium: Gravimetric, electrochemical, surface morphology, DFT and Monte Carlo simulation studies, Appl. Surf. Sci., 2018, 457, 134–149 CrossRef CAS.
- M. T. Alhaffar, S. A. Umoren, I. B. Obot and S. A. Ali, Isoxazolidine derivatives as corrosion inhibitors for low carbon steel in HCl solution: experimental, theoretical and effect of KI studies, RSC Adv., 2018, 8, 1764–1777 RSC.
- O. Kaczerewska, R. Leiva-Garcia, R. Akid, B. Brycki, I. Kowalczyk and T. Pospieszny, Effectiveness of O-bridged cationic gemini surfactants as corrosion inhibitors for stainless steel in 3 M HCl: Experimental and theoretical studies, J. Mol. Liq., 2018, 249, 1113–1124 CrossRef CAS.
- C. Verma, M. Quraishi and E. E. Ebenso, Microwave and ultrasound irradiations for the synthesis of environmentally sustainable corrosion inhibitors: An overview, Sustainable Chem. Pharm., 2018, 10, 134–147 CrossRef.
- A. Khramov, N. Voevodin, V. Balbyshev and M. Donley, Hybrid organo-ceramic corrosion protection coatings with encapsulated organic corrosion inhibitors, Thin Solid Films, 2004, 447, 549–557 CrossRef.
-
U. Dahlmann and M. Feustel, Corrosion and gas hydrate inhibitors having improved water solubility and increased biodegradability, US Pat., US7323609B2, 2008 Search PubMed.
- C. Verma, L. Olasunkanmi, E. E. Ebenso and M. Quraishi, Substituents effect on corrosion inhibition performance of organic compounds in aggressive ionic solutions: a review, J. Mol. Liq., 2018, 251, 100–118 CrossRef CAS.
- P. Singh, E. E. Ebenso, L. O. Olasunkanmi, I. Obot and M. Quraishi, Electrochemical, theoretical, and surface morphological studies of corrosion inhibition effect of green naphthyridine derivatives on mild steel in hydrochloric acid, J. Phys. Chem. C, 2016, 120, 3408–3419 CrossRef CAS.
- C. Verma, L. O. Olasunkanmi, E. E. Ebenso, M. A. Quraishi and I. B. Obot, Adsorption behavior of glucosamine-based, pyrimidine-fused heterocycles as green corrosion inhibitors for mild steel: experimental and theoretical studies, J. Phys. Chem. C, 2016, 120, 11598–11611 CrossRef CAS.
- A. Mishra, C. Verma, H. Lgaz, V. Srivastava, M. Quraishi and E. E. Ebenso, Synthesis, characterization and corrosion inhibition studies of N-phenyl-benzamides on the acidic corrosion of mild steel: Experimental and computational studies, J. Mol. Liq., 2018, 251, 317–332 CrossRef CAS.
-
V. S. Sastri, Green corrosion inhibitors: theory and practice, John Wiley & Sons, 2012 Search PubMed.
- E. Ebenso, U. Ekpe, B. Ita, O. Offiong and U. Ibok, Effect of molecular structure on the efficiency of amides and thiosemicarbazones used for corrosion inhibition of mild steel in hydrochloric acid, Mater. Chem. Phys., 1999, 60, 79–90 CrossRef CAS.
- N. Obi-Egbedi and I. Obot, Xanthione: A new and effective corrosion inhibitor for mild steel in sulphuric acid solution, Arabian J. Chem., 2013, 6, 211–223 CrossRef CAS.
- V. R. Saliyan and A. V. Adhikari, Quinolin-5-ylmethylene-3-{[8-(trifluoromethyl)quinolin-4-yl]thio}propanohydrazide as an effective inhibitor of mild steel corrosion in HCl solution, Corros. Sci., 2008, 50, 55–61 CrossRef CAS.
- C. Verma, E. Ebenso, I. Bahadur, I. Obot and M. Quraishi, 5-(Phenylthio)-3H-pyrrole-4-carbonitriles as effective corrosion inhibitors for mild steel in 1 M HCl: experimental and theoretical investigation, J. Mol. Liq., 2015, 212, 209–218 CrossRef CAS.
- M. Goyal, S. Kumar, I. Bahadur, C. Verma and E. E. Ebenso, Organic corrosion inhibitors for industrial cleaning of ferrous and non-ferrous metals in acidic solutions: A review, J. Mol. Liq., 2018, 256, 565–573 CrossRef CAS.
- A. Maayta and N. Al-Rawashdeh, Inhibition of acidic corrosion of pure aluminum by some organic compounds, Corros. Sci., 2004, 46, 1129–1140 CrossRef CAS.
- A. El-Etre, Inhibition of aluminum corrosion using Opuntia extract, Corros. Sci., 2003, 45, 2485–2495 CrossRef CAS.
- S. Refaey, F. Taha and A. Abd El-Malak, Inhibition of stainless steel pitting corrosion in acidic medium by 2-mercaptobenzoxazole, Appl. Surf. Sci., 2004, 236, 175–185 CrossRef CAS.
- C. Verma, J. Haque, M. Quraishi and E. E. Ebenso, Aqueous phase environmental friendly organic corrosion inhibitors derived from one step multicomponent reactions: a review, J. Mol. Liq., 2019, 275, 18–40 CrossRef CAS.
- C. Verma, D. K. Verma, E. E. Ebenso and M. A. Quraishi, Sulfur and phosphorus heteroatom-containing compounds as corrosion inhibitors: An overview, Heteroat. Chem., 2018, 29, e21437 CrossRef.
- C. Verma, E. E. Ebenso and M. Quraishi, Molecular structural aspects of organic corrosion inhibitors: Influence of –CN and –NO2 substituents on designing of potential corrosion: Inhibitors for aqueous media, J. Mol. Liq., 2020, 113874 CrossRef CAS.
- H. Wang and J. Zheng, Progress of Research on Environmental-Friendly Corrosion Inhibitors, Corros. Sci. Prot. Technol., 2002, 14, 275–279 CAS.
- M. Bethencourt, F. Botana, J. Calvino, M. Marcos and M. Rodriguez-Chacon, Lanthanide compounds as environmentally-friendly corrosion inhibitors of aluminium alloys: a review, Corros. Sci., 1998, 40, 1803–1819 CrossRef CAS.
- S. M. Powell, H. McMurray and D. Worsley, Use of the scanning reference electrode technique for the evaluation of environmentally friendly, nonchromate corrosion inhibitors, Corrosion, 1999, 55, 1040–1051 CrossRef CAS.
- M. Sun, H. Lin, W. Guo, F. Zhao and J. Li, Bioaccumulation and biodegradation of sulfamethazine in Chlorella pyrenoidosa, J. Ocean Univ. China, 2017, 16, 1167–1174 CrossRef CAS.
- A. N. Kabra, M.-K. Ji, J. Choi, J. R. Kim, S. P. Govindwar and B.-H. Jeon, Toxicity of atrazine and its bioaccumulation and biodegradation in a green microalga, Chlamydomonas mexicana, Environ. Sci. Pollut. Res., 2014, 21, 12270–12278 CrossRef CAS PubMed.
- J. Greenwood, Organized civil society and democratic legitimacy in the European Union, Brit. J. Polit. Sci., 2007, 333–357 CrossRef.
- R. Dehousse, Delegation of powers in the European Union: The need for a multi-principals model, West Eur. Poli., 2008, 31, 789–805 CrossRef.
-
W. P. Dinter, Biogeography of the OSPAR Maritime Area. A synopsis and synthesis of biogeographical distribution patterns described for the north-east Atlantic, Federal Agency for Nature Conservation, Bonn, Germany, 2001, vol. 167 Search PubMed.
- L. de La Fayette, The OSPAR convention comes into force: continuity and progress, Int. J. Mar. Coast. Law., 1999, 14, 247–297 Search PubMed.
- P. Heslenfeld and E. L. Enserink, OSPAR Ecological Quality Objectives: the utility of health indicators for the North Sea, ICES J. Mar. Sci., 2008, 65, 1392–1397 CrossRef.
- A. Castano, M. Cantarino, P. Castillo and J. Tarazona, Correlations between the RTG-2 cytotoxicity test EC50 and in vivo LC50 rainbow trout bioassay, Chemosphere, 1996, 32, 2141–2157 CrossRef CAS.
- J. Hermens, H. Canton, N. Steyger and R. Wegman, Joint effects of a mixture of 14 chemicals on mortality and inhibition of reproduction of Daphnia magna, Aquat. Toxicol., 1984, 5, 315–322 CrossRef CAS.
- M. Zhang, D. Aguilera, C. Das, H. Vasquez, P. Zage, V. Gopalakrishnan and J. Wolff, Measuring cytotoxicity: a new perspective on LC50, Anticancer Res., 2007, 27, 35–38 CAS.
- A. Zakari and D. Kubmarawa, Acute toxicity (LD50) studies using Swiss albino mice and brine shrimp lethality (LC50 and LC90) determination of the ethanol extract of stem bark of Echinaceae angustifolia DC, Nat. Prod. Chem. Res., 2016, 4, 2 Search PubMed.
- A. L. Parra, R. S. Yhebra, I. G. Sardiñas and L. I. Buela, Comparative study of the assay of Artemia salina L. and the estimate of the medium lethal dose (LD50 value) in mice, to determine oral acute toxicity of plant extracts, Phytomedicine, 2001, 8, 395–400 CrossRef PubMed.
- M. Chomel, C. Fernandez, A. Bousquet-Mélou, C. Gers, Y. Monnier, M. Santonja, T. Gauquelin, R. Gros, C. Lecareux and V. Baldy, Secondary metabolites of P inus halepensis alter decomposer organisms and litter decomposition during afforestation of abandoned agricultural zones, J. Ecol., 2014, 102, 411–424 CrossRef.
-
M. D. Summers, S. L. Blunk and B. M. Jenkins, How straw decomposes: Implications for straw bale construction, Ecological Building Network, 2003 Search PubMed.
- R. Wedmann, I. Ivanovic-Burmazovic and M. R. Filipovic, Nitrosopersulfide (SSNO−) decomposes in the presence of sulfide, cyanide or glutathione to give HSNO/SNO−: consequences for the assumed role in cell signalling, Interface Focus, 2017, 7, 20160139 CrossRef PubMed.
- S. A. van der Heijden and M. T. Jonker, Evaluation of liposome–water partitioning for predicting bioaccumulation potential of hydrophobic organic chemicals, Environ. Sci. Technol., 2009, 43, 8854–8859 CrossRef CAS PubMed.
- J. M. Conder, R. A. Hoke, W.d. Wolf, M. H. Russell and R. C. Buck, Are PFCAs bioaccumulative? A critical review and comparison with regulatory criteria and persistent lipophilic compounds, Environ. Sci. Technol., 2008, 42, 995–1003 CrossRef CAS PubMed.
- R. L. Williamson Jr, D. T. Burton, J. H. Clarke and L. E. Fleming, Gathering danger: the urgent need to regulate toxic substances that can bioaccumulate, Ecology LQ, 1993, 20, 605 Search PubMed.
- W. M. Meylan, P. H. Howard, R. S. Boethling, D. Aronson, H. Printup and S. Gouchie, Improved method for estimating bioconcentration/bioaccumulation factor from octanol/water partition coefficient, Environ. Toxicol. Chem., 1999, 18, 664–672 CrossRef CAS.
- D. K. Yadav and M. A. Quraishi, Application of some condensed uracils as corrosion inhibitors for mild steel: gravimetric, electrochemical, surface morphological, UV–visible, and theoretical investigations, Ind. Eng. Chem. Res., 2012, 51, 14966–14979 CrossRef CAS.
-
F. Kuang, J. Zhang, C. Zou, T. Shi, Y. Wang, S. Zhang and H. Xu, Electrochemical Methods for Corrosion Monitoring: A Survey of Recent Patents, Recent Patents on Corrosion Science, 2010 Search PubMed.
-
C. Page and P. Cunningham, Electro-chemical Methods of Corrosion Monitoring for Marine Concrete Structures, 1988 Search PubMed.
- M. Chigondo and F. Chigondo, Recent natural corrosion inhibitors for mild steel: an overview, J. Chem., 2016, 2016, 1–7 CrossRef.
- C. Verma, E. E. Ebenso and M. Quraishi, Ionic liquids as green and sustainable corrosion inhibitors for metals and alloys: an overview, J. Mol. Liq., 2017, 233, 403–414 CrossRef CAS.
- C. Verma, E. Ebenso and M. Quraishi, Alkaloids as green and environmental benign corrosion inhibitors: An overview, Int. J. Corros. Scale Inhib., 2019, 8, 512–528 CAS.
- P. Dohare, M. Quraishi, C. Verma, H. Lgaz, R. Salghi and E. Ebenso, Ultrasound induced green synthesis of pyrazolo-pyridines as novel corrosion inhibitors useful for industrial pickling process: experimental and theoretical approach, Results Phys., 2019, 13, 102344 CrossRef.
- R. González-Olvera, V. Román-Rodríguez, G. E. Negrón-Silva, A. Espinoza-Vázquez, F. J. Rodríguez-Gómez and R. Santillan, Multicomponent synthesis and evaluation of new 1, 2, 3-triazole derivatives of dihydropyrimidinones as acidic corrosion inhibitors for steel, Molecules, 2016, 21, 250 CrossRef PubMed.
- I. Obot, D. Macdonald and Z. Gasem, Density functional theory (DFT) as a powerful tool for designing new organic corrosion inhibitors. Part 1: an overview, Corros. Sci., 2015, 99, 1–30 CrossRef CAS.
- D. K. Verma, Density Functional Theory (DFT) as a Powerful Tool for Designing Corrosion Inhibitors in Aqueous Phase, Adv. Eng. Testing, 2018, 87 Search PubMed.
- L. H. Madkour, S. Kaya and I. B. Obot, Computational, Monte Carlo simulation and experimental studies of some arylazotriazoles (AATR) and their copper complexes in corrosion inhibition process, J. Mol. Liq., 2018, 260, 351–374 CrossRef CAS.
- R. M. Pidaparti, L. Fang and M. J. Palakal, Computational simulation of multi-pit corrosion process in materials, Comput. Mater. Sci., 2008, 41, 255–265 CrossRef CAS.
- N. O. Eddy, F. E. Awe, C. E. Gimba, N. O. Ibisi and E. E. Ebenso, QSAR, Experimental and computational chemistry simulation studies on the inhibition potentials of some amino acids for the corrosion of mild steel in 0.1 M HCl, Int. J. Electrochem. Sci., 2011, 6, 931–957 CAS.
- I. Obot, K. Haruna and T. Saleh, Atomistic simulation: a unique and powerful computational tool for corrosion inhibition research, Arabian J. Sci. Eng., 2019, 44, 1–32 CrossRef.
- N. Obi-Egbedi, I. Obot, M. El-Khaiary, S. Umoren and E. Ebenso, Computational simulation and statistical analysis on the relationship between corrosion inhibition efficiency and molecular structure of some phenanthroline derivatives on mild steel surface, Int. J. Electrochem. Sci., 2011, 6, e5675 Search PubMed.
- S. Kaya, P. Banerjee, S. K. Saha, B. Tüzün and C. Kaya, Theoretical evaluation of some benzotriazole and phospono derivatives as aluminum corrosion inhibitors: DFT and molecular dynamics simulation approaches, RSC Adv., 2016, 6, 74550–74559 RSC.
- E. E. Annastasia, F. A. Similoluwa, O. Esther, S. Adewale, S. Banjo and O. A. Kolawole, Anti-Corrosion Activity Study of 1,2,3-Triazole Derivatives on Carbon Steel in Acidic Media: Theoretical Approach, Anal. Bioanal. Electrochem., 2019, 11, 1414–1425 CAS.
- M. Belghiti, Y. Karzazi, S. Tighadouini, A. Dafali, C. Jama, I. Warad, B. Hammouti and S. Radi, New hydrazine derivatives as corrosion for mild steel in phosphoric acid medium. Part B: Theoretical investigation, J. Mater. Environ. Sci., 2016, 7, 956–967 CAS.
- D. S. Chauhan, M. Quraishi, A. Sorour, S. K. Saha and P. Banerjee, Triazole-modified chitosan: a biomacromolecule as a new environmentally benign corrosion inhibitor for carbon steel in a hydrochloric acid solution, RSC Adv., 2019, 9, 14990–15003 RSC.
- I. Obot, N. Obi-Egbedi and S. Umoren, Antifungal drugs as corrosion inhibitors for aluminium in 0.1 M HCl, Corros. Sci., 2009, 51, 1868–1875 CrossRef CAS.
-
M. Yadav, P. Yadav and U. Sharma, Substituted imidazoles as corrosion inhibitors for N80 steel in hydrochloric acid, 2013 Search PubMed.
- M. K. Awad, M. R. Mustafa and M. M. A. Elnga, Computational simulation of the molecular structure of some triazoles as inhibitors for the corrosion of metal surface, THEOCHEM, 2010, 959, 66–74 CrossRef CAS.
- C. Verma, M. Quraishi, L. Olasunkanmi and E. E. Ebenso, L-Proline-promoted synthesis of 2-amino-4-arylquinoline-3-carbonitriles as sustainable
corrosion inhibitors for mild steel in 1 M HCl: experimental and computational studies, RSC Adv., 2015, 5, 85417–85430 RSC.
- C. Verma, A. Singh, G. Pallikonda, M. Chakravarty, M. Quraishi, I. Bahadur and E. Ebenso, Aryl sulfonamidomethylphosphonates as new class of green corrosion inhibitors for mild steel in 1 M HCl: electrochemical, surface and quantum chemical investigation, J. Mol. Liq., 2015, 209, 306–319 CrossRef CAS.
- C. Verma, L. O. Olasunkanmi, I. Obot, E. E. Ebenso and M. Quraishi, 2,4-Diamino-5-(phenylthio)-5H-chromeno[2,3-b]pyridine-3-carbonitriles as green and effective corrosion inhibitors: gravimetric, electrochemical, surface morphology and theoretical studies, RSC Adv., 2016, 6, 53933–53948 RSC.
- K. Khaled, Monte Carlo simulations of corrosion inhibition of mild steel in 0.5 M sulphuric acid by some green corrosion inhibitors, J. Solid State Electrochem., 2009, 13, 1743–1756 CrossRef CAS.
- M. Ramezanzadeh, G. Bahlakeh, Z. Sanaei and B. Ramezanzadeh, Corrosion inhibition of mild steel in 1 M HCl solution by ethanolic extract of eco-friendly Mangifera indica (mango) leaves: electrochemical, molecular dynamics, Monte Carlo and ab initio study, Appl. Surf. Sci., 2019, 463, 1058–1077 CrossRef CAS.
- K. Alaoui, M. Ouakki, A. Abousalem, H. Serrar, M. Galai, S. Derbali, K. Nouneh, S. Boukhris, M. E. Touhami and Y. El Kacimi, Molecular dynamics, Monte-Carlo simulations and atomic force microscopy to study the interfacial adsorption behaviour of some triazepine carboxylate compounds as corrosion inhibitors in acid medium, J. Bio-and Tribo-Corros., 2019, 5, 1 CrossRef.
- M. Abdallah, H. Al-Tass, B. A. Jahdaly and A. Fouda, Inhibition properties and adsorption behavior of 5-arylazothiazole derivatives on 1018 carbon steel in 0.5 M H2SO4 solution, J. Mol. Liq., 2016, 216, 590–597 CrossRef CAS.
- E. A. Noor and A. H. Al-Moubaraki, Thermodynamic study of metal corrosion and inhibitor adsorption processes in mild steel/1-methyl-4 [4′(-X)-styryl pyridinium iodides/hydrochloric acid systems, Mater. Chem. Phys., 2008, 110, 145–154 CrossRef CAS.
- M. Bahri, A. Mahdavi, A. Mirzaei, A. Mansouri and F. Haghighat, Integrated oxidation process and biological treatment for highly concentrated petrochemical effluents: a review, Chem. Eng. Process., 2018, 125, 183–196 CrossRef CAS.
- R. Gueccia, A. R. Aguirre, S. Randazzo, A. Cipollina and G. Micale, Diffusion Dialysis for Separation of Hydrochloric Acid, Iron and Zinc Ions from Highly Concentrated Pickling Solutions, Membranes, 2020, 10, 129 CrossRef CAS PubMed.
- A. Agrawal and K. Sahu, An overview of the recovery of acid from spent acidic solutions from steel and electroplating industries, J. Hazard. Mater., 2009, 171, 61–75 CrossRef CAS PubMed.
- M. Finšgar and J. Jackson, Application of corrosion inhibitors for steels in acidic media for the oil and gas industry: a review, Corros. Sci., 2014, 86, 17–41 CrossRef.
-
Y. Chen and W. Yang, Formulation of Corrosion Inhibitors, Water Chemistry, IntechOpen, 2019 Search PubMed.
- A. Singh, K. Ansari, D. S. Chauhan, M. Quraishi, H. Lgaz and I.-M. Chung, Comprehensive investigation of steel corrosion inhibition at macro/micro level by ecofriendly green corrosion inhibitor in 15% HCl medium, J. Colloid Interface Sci., 2020, 560, 225–236 CrossRef CAS PubMed.
- K. Ansari and M. Quraishi, Experimental and quantum chemical evaluation of Schiff bases of isatin as a new and green corrosion inhibitors for mild steel in 20% H2SO4, J. Taiwan Inst. Chem. Eng., 2015, 54, 145–154 CrossRef CAS.
- K. Ansari, M. Quraishi and A. Singh, Isatin derivatives as a non-toxic corrosion inhibitor for mild steel in 20% H2SO4, Corros. Sci., 2015, 95, 62–70 CrossRef CAS.
- S. Kumar, D. Sharma, P. Yadav and M. Yadav, Experimental and quantum chemical studies on corrosion inhibition effect of synthesized organic compounds on N80 steel in hydrochloric acid, Ind. Eng. Chem. Res., 2013, 52, 14019–14029 CrossRef CAS.
- M. Yadav, D. Behera, S. Kumar and R. R. Sinha, Experimental and quantum chemical studies on the corrosion inhibition performance of benzimidazole derivatives for mild steel in HCl, Ind. Eng. Chem. Res., 2013, 52, 6318–6328 CrossRef CAS.
- M. Yadav, R. Sinha, S. Kumar and T. Sarkar, Corrosion inhibition effect of spiropyrimidinethiones on mild steel in 15% HCl solution: insight from electrochemical and quantum studies, RSC Adv., 2015, 5, 70832–70848 RSC.
- S. A. Umoren, M. M. Solomon, I. B. Obot and R. K. Suleiman, Comparative studies on the corrosion inhibition efficacy of ethanolic extracts of date palm leaves and seeds on carbon steel corrosion in 15% HCl solution, J. Adhes. Sci. Technol., 2018, 32, 1934–1951 CrossRef CAS.
- M. Quraishi and D. Jamal, Corrosion inhibition of N-80 steel and mild steel in 15% boiling hydrochloric acid by a triazole compound—SAHMT, Mater. Chem. Phys., 2001, 68, 283–287 CrossRef CAS.
- Y. Jianguo, W. Lin, V. Otieno-Alego and D. Schweinsberg, Polyvinylpyrrolidone and polyethylenimine as inhibitors for the corrosion of a low carbon steel in phosphoric acid, Corros. Sci., 1995, 37, 975–985 CrossRef CAS.
- M. Hegazy, Novel cationic surfactant based on triazole as a corrosion inhibitor for carbon steel in phosphoric acid produced by dihydrate wet process, J. Mol. Liq., 2015, 208, 227–236 CrossRef CAS.
- Y. G. Avdeev, M. Tyurina and Y. I. Kuznetsov, Protection of low-carbon steel in phosphoric acid solutions by mixtures of a substituted triazole with sulfur-containing compounds, Int. J. Corros Scale Inhib., 2014, 3, 246–253 CrossRef.
- S. H. Zaferani, M. Sharifi, D. Zaarei and M. R. Shishesaz, Application of eco-friendly products as corrosion inhibitors for metals in acid pickling processes–A review, J. Environ. Chem. Eng., 2013, 1, 652–657 CrossRef.
- K. Haruna, T. A. Saleh and M. Quraishi, Expired metformin drug as green corrosion inhibitor for simulated oil/gas well acidizing environment, J. Mol. Liq., 2020, 315, 113716 CrossRef CAS.
- A. Singh and M. A. Quraishi, Acidizing corrosion inhibitors: a review, J. Mater. Environ. Sci., 2015, 6, 224–235 CAS.
- E. Ituen, V. Mkpenie and E. Dan, Surface protection of steel in oil well acidizing fluids using L-theanine-based corrosion inhibitor formulations: Experimental and theoretical evaluation, Surf. Interfaces, 2019, 16, 29–42 CrossRef CAS.
- P. Rajeev, A. Surendranathan and C. S. Murthy, Corrosion mitigation of the oil well steels using organic inhibitors–a review, J. Mater. Environ. Sci., 2012, 3, 856–869 CAS.
- D. S. Chauhan, M. J. Mazumder, M. Quraishi, K. Ansari and R. Suleiman, Microwave-assisted synthesis of a new Piperonal-Chitosan Schiff base as a bio-inspired corrosion inhibitor for oil-well acidizing, Int. J. Biol. Macromol., 2020, 158, 231–243 CrossRef CAS PubMed.
- K. Haruna, I. Obot, N. Ankah, A. Sorour and T. Saleh, Gelatin: A green corrosion inhibitor for carbon steel in oil well acidizing environment, J. Mol. Liq., 2018, 264, 515–525 CrossRef CAS.
- M. Quraishi and D. Jamal, CAHMT—a new and eco-friendly acidizing corrosion inhibitor, Corrosion, 2000, 56, 983–985 CrossRef CAS.
- T. Philibert, B. H. Lee and N. Fabien, Current status and new perspectives on chitin and chitosan as functional biopolymers, Appl. Biochem. Biotechnol., 2017, 181, 1314–1337 CrossRef CAS PubMed.
- D. Raafat and H. G. Sahl, Chitosan and its antimicrobial potential–a critical literature survey, Microb. Biotechnol., 2009, 2, 186–201 CrossRef CAS PubMed.
-
P. Suresh, Enzymatic technologies of chitin and chitosan, Enzym. Technol. Mar. Polysaccharides, 2019, p. 449 Search PubMed.
- I. Hamed, F. Özogul and J. M. Regenstein, Industrial applications of crustacean by-products (chitin, chitosan, and chitooligosaccharides): A review, Trends Food Sci. Technol., 2016, 48, 40–50 CrossRef CAS.
-
P. K. Dutta, J. Dutta and V. Tripathi, Chitin and chitosan: Chemistry, properties and applications, 2004 Search PubMed.
- S.-K. Kim and N. Rajapakse, Enzymatic production and biological activities of chitosan oligosaccharides (COS): A review, Carbohydr. Polym., 2005, 62, 357–368 CrossRef CAS.
- T. Imai, S. Shiraishi, H. Saitô and M. Otagiri, Interaction of indomethacin with low molecular weight chitosan, and improvements of some pharmaceutical properties of indomethacin by low molecular weight chitosans, Int. J. Pharm., 1991, 67, 11–20 CrossRef CAS.
- A. Singla and M. Chawla, Chitosan: Some pharmaceutical and biological aspects-an update, J. Pharm. Pharmacol., 2001, 53, 1047–1067 CrossRef CAS PubMed.
- R. R. Mohamed and A. Fekry, Antimicrobial and anticorrosive activity of adsorbents based on chitosan Schiff's base, Int. J. Electrochem. Sci., 2011, 6, 2488–2508 CAS.
- M. M. Solomon, H. Gerengi, T. Kaya and S. A. Umoren, Enhanced corrosion inhibition effect of chitosan for St37 in 15% H2SO4 environment by silver nanoparticles, Int. J. Biol. Macromol., 2017, 104, 638–649 CrossRef CAS PubMed.
- J. Carneiro, J. Tedim and M. Ferreira, Chitosan as a smart coating for corrosion protection of aluminum alloy 2024: A review, Prog. Org. Coat., 2015, 89, 348–356 CrossRef CAS.
- E. Avcu, F. E. Baştan, H. Z. Abdullah, M. A. U. Rehman, Y. Y. Avcu and A. R. Boccaccini, Electrophoretic deposition of chitosan-based composite coatings for biomedical applications: A review, Prog. Mater. Sci., 2019, 103, 69–108 CrossRef CAS.
- Z. Guo, R. Xing, S. Liu, Z. Zhong, X. Ji, L. Wang and P. Li, Antifungal properties of Schiff bases of chitosan, N-substituted chitosan and quaternized chitosan, Carbohydr. Res., 2007, 342, 1329–1332 CrossRef CAS PubMed.
- E. Portes, C. Gardrat, A. Castellan and V. Coma, Environmentally friendly films based on chitosan and tetrahydrocurcuminoid derivatives exhibiting antibacterial and antioxidative properties, Carbohydr. Polym., 2009, 76, 578–584 CrossRef CAS.
-
M. F. Goosen, Applications of Chitan and Chitosan, CRC Press, 1996 Search PubMed.
- S. A. Umoren, M. J. Banera, T. Alonso-Garcia, C. A. Gervasi and M. V. Mirífico, Inhibition of mild steel corrosion in HCl solution using chitosan, Cellulose, 2013, 20, 2529–2545 CrossRef CAS.
- T. Rabizadeh and S. Khameneh, Asl, Chitosan as a green inhibitor for mild steel corrosion: Thermodynamic and electrochemical evaluations, Mater. Corros., 2019, 70, 738–748 CrossRef CAS.
- N. K. Gupta, P. Joshi, V. Srivastava and M. Quraishi, Chitosan: A macromolecule as green corrosion inhibitor for mild steel in sulfamic acid useful for sugar industry, Int. J. Biol. Macromol., 2018, 106, 704–711 CrossRef CAS PubMed.
- A. Jmiai, B. El Ibrahimi, A. Tara, R. Oukhrib, S. El Issami, O. Jbara, L. Bazzi and M. Hilali, Chitosan as an eco-friendly inhibitor for copper corrosion in acidic medium: protocol and characterization, Cellulose, 2017, 24, 3843–3867 CrossRef CAS.
- K. El Mouaden, B. El Ibrahimi, R. Oukhrib, L. Bazzi, B. Hammouti, O. Jbara, A. Tara, D. S. Chauhan and M. A. Quraishi, Chitosan polymer as a green corrosion inhibitor for copper in sulfide-containing synthetic seawater, Int. J. Biol. Macromol., 2018, 119, 1311–1323 CrossRef CAS PubMed.
- Y. Brou, N. Coulibaly, D. N’gys, J. Creus and A. Trokourey, Chitosan biopolymer effect on copper corrosion in 3.5 wt% NaCl solution: Electrochemical and quantum chemical studies, Int. J. Corros. Scale Inhib., 2020, 9, 182–200 CAS.
- H. Harmami, I. Ulfin, A. H. Sakinah and Y. L. Ni’mah, Water-soluble chitosan from shrimp and mussel shells as corrosion inhibitor on tinplate in 2% NaCl, Mal. J. Fund. Appl. Sci., 2019, 15, 212–217 CrossRef.
- Q. Zhao, J. Guo, G. Cui, T. Han and Y. Wu, Chitosan derivatives as green corrosion inhibitors for P110 steel in a carbon dioxide environment, Colloids Surf., B, 2020, 111150 CrossRef CAS.
- G. A. El-Mahdy, A. M. Atta, H. A. Al-Lohedan and A. O. Ezzat, Influence of green corrosion inhibitor based on chitosan ionic liquid on the steel corrodibility in chloride solution, Int. J. Electrochem. Sci., 2015, 10, 5812–5826 CAS.
- A. M. Alsabagh, M. Z. Elsabee, Y. M. Moustafa, A. Elfky and R. E. Morsi, Corrosion inhibition efficiency of some hydrophobically modified chitosan surfactants in relation to their surface active properties, Egypt. J. Pet., 2014, 23, 349–359 CrossRef.
- S. M. El-Sawy, Y. M. Abu-Ayana and F. A. Abdel-Mohdy, Some chitin/chitosan derivatives for corrosion protection and waste water treatments, Anti-Corros. Methods Mater., 2001, 48, 227–235 CrossRef CAS.
- D. Suyanto, H. Darmokoesemo, R. Ruriyanti and L. S. Anggara, Application chitosan derivatives as inhibitor corrosion on steel with fluidization method, J. Chem. Pharm. Res., 2015, 2, 260–267 Search PubMed.
- D. S. Chauhan, M. J. Mazumder, M. Quraishi and K. Ansari, Chitosan-cinnamaldehyde Schiff base: A bioinspired macromolecule as corrosion inhibitor for oil and gas industry, Int. J. Biol. Macromol., 2020, 158, 127–138 CrossRef CAS PubMed.
- H. Li, H. Li, Y. Liu and X. Huang, Synthesis of polyamine grafted chitosan copolymer and evaluation of its corrosion inhibition performance, J. Korean Chem. Soc., 2015, 59, 142–147 CrossRef CAS.
- T. Chen, D. Zeng and S. Zhou, Study of Polyaspartic Acid and Chitosan Complex Corrosion Inhibition and Mechanisms, Pol. J. Environ. Stud., 2018, 27, 1441–1448 CrossRef CAS.
- R. G. M. de Araújo Macedo, N. do Nascimento Marques, J. Tonholo and R. de Carvalho Balaban, Water-soluble carboxymethylchitosan used as corrosion inhibitor for carbon steel in saline medium, Carbohydr. Polym., 2019, 205, 371–376 CrossRef PubMed.
- J. Haque, V. Srivastava, D. S. Chauhan, H. Lgaz and M. A. Quraishi, Microwave-induced synthesis of chitosan Schiff bases and their application as novel and green corrosion inhibitors: experimental and theoretical approach, ACS Omega, 2018, 3, 5654–5668 CrossRef CAS PubMed.
- M. Quraishi, K. Ansari, D. S. Chauhan, S. A. Umoren and M. Mazumder, Vanillin modified chitosan as a new bio-inspired corrosion inhibitor for carbon steel in oil-well acidizing relevant to petroleum industry, Cellulose, 2020, 27, 6425–6443 CrossRef CAS.
- R. Menaka and S. Subhashini, Chitosan Schiff base as eco-friendly inhibitor for mild steel corrosion in 1 M HCl, J. Adhes. Sci. Technol., 2016, 30, 1622–1640 CrossRef CAS.
- M. Li, J. Xu, R. Li, D. Wang, T. Li, M. Yuan and J. Wang, Simple preparation of aminothiourea-modified chitosan as corrosion inhibitor and heavy metal ion adsorbent, J. Colloid Interface Sci., 2014, 417, 131–136 CrossRef CAS PubMed.
- G. E. Luckachan and V. Mittal, Anti-corrosion behavior of layer by layer coatings of cross-linked chitosan and poly (vinyl butyral) on carbon steel, Cellulose, 2015, 22, 3275–3290 CrossRef CAS.
- D. Chauhan, V. Srivastava, P. Joshi and M. Quraishi, PEG cross-linked chitosan: a biomacromolecule as corrosion inhibitor for sugar industry, Int. J. Ind. Chem., 2018, 9, 363–377 CrossRef CAS.
- K. E. Mouaden, D. Chauhan, M. Quraishi and L. Bazzi, Thiocarbohydrazide-crosslinked chitosan as a bioinspired corrosion inhibitor for protection of stainless steel in 3.5% NaCl, Sustainable Chem. Pharm., 2020, 15, 100213 CrossRef.
- V. Srivastava, D. S. Chauhan, P. G. Joshi, V. Maruthapandian, A. A. Sorour and M. A. Quraishi, PEG-Functionalized Chitosan: A Biological Macromolecule as a Novel Corrosion Inhibitor, ChemistrySelect, 2018, 3, 1990–1998 CrossRef CAS.
- P. Kong, H. Feng, N. Chen, Y. Lu, S. Li and P. Wang, Polyaniline/chitosan as a corrosion inhibitor for mild steel in acidic medium, RSC Adv., 2019, 9, 9211–9217 RSC.
- A. Sanmugam, D. Vikraman, K. Karuppasamy, J. Y. Lee and H.-S. Kim, Evaluation of the corrosion resistance properties of electroplated chitosan-Zn1−xCuxO composite thin films, Nanomaterials, 2017, 7, 432 CrossRef PubMed.
- S. John, A. Joseph, A. J. Jose and B. Narayana, Enhancement of corrosion protection of mild steel by chitosan/ZnO nanoparticle composite membranes, Prog. Org. Coat., 2015, 84, 28–34 CrossRef CAS.
- P. A. Rasheed, K. A. Jabbar, K. Rasool, R. P. Pandey, M. H. Sliem, M. Helal, A. Samara, A. M. Abdullah and K. A. Mahmoud, Controlling the biocorrosion of sulfate-reducing bacteria (SRB) on carbon steel using ZnO/chitosan nanocomposite as an eco-friendly biocide, Corros. Sci., 2019, 148, 397–406 CrossRef.
- Y. Liu, C. Zou, X. Yan, R. Xiao, T. Wang and M. Li, β-Cyclodextrin modified natural chitosan as a green inhibitor for carbon steel in acid solutions, Ind. Eng. Chem. Res., 2015, 54, 5664–5672 CrossRef CAS.
- G. Jena, B. Anandkumar, S. Vanithakumari, R. George, J. Philip and G. Amarendra, Graphene oxide-chitosan-silver composite coating on Cu-Ni alloy with enhanced anticorrosive and antibacterial properties suitable for marine applications, Prog. Org. Coat., 2020, 139, 105444 CrossRef CAS.
- M. M. Solomon, H. Gerengi, T. Kaya and S. A. Umoren, Performance evaluation of a chitosan/silver nanoparticles composite on St37 steel corrosion in a 15% HCl solution, ACS Sustainable Chem. Eng., 2017, 5, 809–820 CrossRef CAS.
- E. Tabesh, H. Salimijazi, M. Kharaziha, M. Mahmoudi and M. Hejazi, Development of an in-situ chitosan-copper nanoparticle coating by electrophoretic deposition, Surf. Coat. Technol., 2019, 364, 239–247 CrossRef CAS.
- S. Karimi, E. Salahinejad, E. Sharifi, A. Nourian and L. Tayebi, Bioperformance of chitosan/fluoride-doped diopside nanocomposite coatings deposited on medical stainless steel, Carbohydr. Polym., 2018, 202, 600–610 CrossRef CAS PubMed.
- X. Zhai, Y. Ren, N. Wang, F. Guan, M. Agievich, J. Duan and B. Hou, Microbial Corrosion Resistance and Antibacterial Property of Electrodeposited Zn–Ni–Chitosan Coatings, Molecules, 2019, 24, 1974 CrossRef CAS PubMed.
- S. John, A. Salam, A. M. Baby and A. Joseph, Corrosion inhibition of mild steel using chitosan/TiO2 nanocomposite coatings, Prog. Org. Coat., 2019, 129, 254–259 CrossRef CAS.
- L. Cordero-Arias, S. Cabanas-Polo, H. Gao, J. Gilabert, E. Sanchez, J. Roether, D. Schubert, S. Virtanen and A. R. Boccaccini, Electrophoretic deposition of nanostructured-TiO2/chitosan composite coatings on stainless steel, RSC Adv., 2013, 3, 11247–11254 RSC.
- P. Ledwig, M. Kot, T. Moskalewicz and B. Dubiel, Electrophoretic deposition of nc-TiO2/chitosan composite coatings on X2CrNiMo17-12-2 stainless steel, Arch. Metall. Mater., 2017, 62, 405–410 CAS.
- B.-D. Hahn, D.-S. Park, J.-J. Choi, J. Ryu, W.-H. Yoon, J.-H. Choi, H.-E. Kim and S.-G. Kim, Aerosol deposition of hydroxyapatite–chitosan composite coatings on biodegradable magnesium alloy, Surf. Coat. Technol., 2011, 205, 3112–3118 CrossRef CAS.
- S. Sutha, K. Kavitha, G. Karunakaran and V. Rajendran, In-vitro bioactivity, biocorrosion and antibacterial activity of silicon integrated hydroxyapatite/chitosan composite coating on 316 L stainless steel implants, Mater. Sci. Eng.: C, 2013, 33, 4046–4054 CrossRef CAS PubMed.
- A. Molaei, M. Yari and M. R. Afshar, Investigation of halloysite nanotube content on electrophoretic deposition (EPD) of chitosan-bioglass-hydroxyapatite-halloysite nanotube nanocomposites films in surface engineering, Appl. Clay Sci., 2017, 135, 75–81 CrossRef CAS.
- L. L.d. Sousa, V. P. Ricci, D. G. Prado, R. C. Apolinario, L. C. d. O. Vercik, E. C. d. S. Rigo, M. C. d. S. Fernandes and N. A. Mariano, Titanium coating with hydroxyapatite and chitosan doped with silver nitrate, Mater. Res., 2017, 20, 863–868 CrossRef.
- M. Stevanović, M. Đošić, A. Janković, V. Kojic, M. Vukasinovic-Sekulic, J. Stojanović, J. Odović, M. Crevar Sakač, K. Y. Rhee and V. Mišković-Stanković, Gentamicin-loaded bioactive hydroxyapatite/chitosan composite coating electrodeposited on titanium, ACS Biomater. Sci. Eng., 2018, 4, 3994–4007 CrossRef PubMed.
- F. Ordikhani, E. Tamjid and A. Simchi, Characterization and antibacterial performance of electrodeposited chitosan–vancomycin composite coatings for prevention of implant-associated infections, Mater. Sci. Eng.: C, 2014, 41, 240–248 CrossRef CAS PubMed.
- P. Sambyal, G. Ruhi, S. Dhawan, B. Bisht and S. Gairola, Enhanced anticorrosive properties of tailored poly (aniline-anisidine)/chitosan/SiO2 composite for protection of mild steel in aggressive marine conditions, Prog. Org. Coat., 2018, 119, 203–213 CrossRef CAS.
- D. Junying, L. Weihua, W. Maotao, Z. Xia and H. Baorong, Synthesis and inhibition studies of carboxymethl chitosan doped polianiline for mild steel in hydrochloric acid, Acta Polym. Sin., 2010, 588–593 Search PubMed.
- S. P. Damari, L. Cullari, D. Laredo, R. Nadiv, E. Ruse, R. Sripada and O. Regev, Graphene and boron nitride nanoplatelets for improving vapor barrier properties in epoxy nanocomposites, Prog. Org. Coat., 2019, 136, 105207 CrossRef.
- M. Srivastava, S. Srivastava, G. Ji and R. Prakash, Chitosan based new nanocomposites for corrosion protection of mild steel in aggressive chloride media, Int. J. Biol. Macromol., 2019, 140, 177–187 CrossRef CAS PubMed.
- H. Ashassi-Sorkhabi, R. Bagheri and B. Rezaei-moghadam, Sonoelectrochemical synthesis of ppy-MWCNTs-chitosan nanocomposite coatings: Characterization and corrosion behavior, J. Mater. Eng. Perform., 2015, 24, 385–392 CrossRef CAS.
- M. Barman, S. Mahmood, R. Augustine, A. Hasan, S. Thomas and K. Ghosal, Natural halloysite nanotubes/chitosan based bio-nanocomposite for delivering norfloxacin, an anti-microbial agent in sustained release manner, Int. J. Biol. Macromol., 2020, 162, 1849–1861 CrossRef CAS PubMed.
- U. Eduok, E. Ohaeri and J. Szpunar, Conversion of Imidazole to N-(3-Aminopropyl)imidazole toward Enhanced Corrosion Protection of Steel in Combination with Carboxymethyl Chitosan Grafted Poly(2-methyl-1-vinylimidazole), Ind. Eng. Chem. Res., 2019, 58, 7179–7192 CrossRef CAS.
-
U. Eduok and J. Szpunar, Biocorrosion Reduction of Pipeline Steel in Desulfovibrio ferrophilus Culture in the Presence of Carboxymethyl Chitosan Grafted Poly (2-methyl-1-vinylimidazole)/Cerium Molybdate Nanocomposite, ECS Meeting Abstracts, IOP Publishing, 2019, p. 853 Search PubMed.
- A. Farhadian, M. A. Varfolomeev, A. Shaabani, S. Nasiri, I. Vakhitov, Y. F. Zaripova, V. V. Yarkovoi and A. V. Sukhov, Sulfonated chitosan as green and high cloud point kinetic methane hydrate and corrosion inhibitor: Experimental and theoretical studies, Carbohydr. Polym., 2020, 236, 116035 CrossRef CAS PubMed.
- R. Farahati, A. Ghaffarinejad, H. J. Rezania, S. M. Mousavi-Khoshdel and H. Behzadi, Sulfonated aromatic polyamide as water-soluble polymeric corrosion inhibitor of copper in HCl, Colloids Surf., A, 2019, 578, 123626 CrossRef.
- G. Ruhi, O. Modi and S. Dhawan, Chitosan-polypyrrole-SiO2 composite coatings with advanced anticorrosive properties, Synth. Met., 2015, 200, 24–39 CrossRef CAS.
- M. W. Bauer, L. E. Driskill, W. Callen, M. A. Snead, E. J. Mathur and R. M. Kelly, An endoglucanase, EglA, from the hyperthermophilic ArchaeonPyrococcus furiosus hydrolyzes β-1, 4 bonds in mixed-linkage (1→ 3),(1→ 4)-β-D-glucans and cellulose, J. Bacteriol., 1999, 181, 284–290 CrossRef CAS PubMed.
- A. Akaracharanya, T. Taprig, J. Sitdhipol and S. Tanasupawat, Characterization of cellulase producing Bacillus and Paenibacillus strains from Thai soils, J. Appl. Pharm. Sci., 2014, 4, 6 Search PubMed.
-
S. Kalia, B. Kaith and I. Kaur, Cellulose fibers: bio-and nano-polymer composites:
green chemistry and technology, Springer Science & Business Media, 2011 Search PubMed.
- S. Gawande, Cellulose: A Natural Polymer on the Earth, Int. J. Polym. Sci. Eng., 2017, 3, 32–37 Search PubMed.
- L. Walker and D. Wilson, Enzymatic hydrolysis of cellulose: an overview, Bioresour. Technol., 1991, 36, 3–14 CrossRef CAS.
- A. C. O’Sullivan, Cellulose: the structure slowly unravels, Cellulose, 1997, 4, 173–207 CrossRef.
- S. A. Umoren and U. M. Eduok, Application of carbohydrate polymers as corrosion inhibitors for metal substrates in different media: a review, Carbohydr. Polym., 2016, 140, 314–341 CrossRef CAS PubMed.
- S. Umoren, M. Solomon, I. Udosoro and A. Udoh, Synergistic and antagonistic effects between halide ions and carboxymethyl cellulose for the corrosion inhibition of mild steel in sulphuric acid solution, Cellulose, 2010, 17, 635–648 CrossRef CAS.
- M. M. Solomon, H. Gerengi and S. A. Umoren, Carboxymethyl cellulose/silver nanoparticles composite: synthesis, characterization and application as a benign corrosion inhibitor for St37 steel in 15% H2SO4 medium, ACS Appl. Mater. Interfaces, 2017, 9, 6376–6389 CrossRef CAS PubMed.
- M. N. El-Haddad, Hydroxyethylcellulose used as an eco-friendly inhibitor for 1018 c-steel corrosion in 3.5% NaCl solution, Carbohydr. Polym., 2014, 112, 595–602 CrossRef CAS PubMed.
- I. Arukalam, I. Madufor, O. Ogbobe and E. Oguzie, Inhibition of mild steel corrosion in sulfuric acid medium by hydroxyethyl cellulose, Chem. Eng. Commun., 2015, 202, 112–122 CrossRef CAS.
- M. Mobin and M. Rizvi, Adsorption and corrosion inhibition behavior of hydroxyethyl cellulose and synergistic surfactants additives for carbon steel in 1 M HCl, Carbohydr. Polym., 2017, 156, 202–214 CrossRef CAS PubMed.
- I. Arukalam, I. Madu, N. Ijomah, C. Ewulonu and G. Onyeagoro, Acid corrosion inhibition and adsorption behaviour of ethyl hydroxyethyl cellulose on mild steel corrosion, J. Mater., 2014, 2014, 1–11 CrossRef.
- M. Deyab, Hydroxyethyl cellulose as efficient organic inhibitor of zinc–carbon battery corrosion in ammonium chloride solution: Electrochemical and surface morphology studies, J. Power Sources, 2015, 280, 190–194 CrossRef CAS.
- S. Nwanonenyi, H. Obasi and I. Eze, Hydroxypropyl Cellulose as an Efficient Corrosion Inhibitor for Aluminium in Acidic Environments: Experimental and Theoretical Approach, Chem. Africa, 2019, 2, 471–482 CAS.
- I. Arukalam, I. Madufor, O. Ogbobe and E. Oguzie, Cellulosic polymers for corrosion protection of aluminium, Int. J. Eng. Tech. Res., 2015, 3, 163–169 Search PubMed.
- I. Arukalam, I. Madufor, O. Ogbobe and E. Oguzie, Experimental and theoretical studies of hydroxyethyl cellulose as inhibitor for acid corrosion inhibition of mild steel and aluminium, Open Corros. J., 2014, 6, 1–10 CrossRef.
-
K. Andarany, A. Sagir, A. Ahmad, S. Deni and W. Gunawan, Cellulose acetate layer effect toward aluminium corrosion rate in hydrochloric acid media, IOP Conference Series: Materials Science and Engineering, Institute of Physics Publishing, 2017 Search PubMed.
- O. Egbuhuzor, I. Madufor, S. Nwanonenyi and J. Bokolo, Adsorption behavior and corrosion rate model of sodium carboxymethyl cellulose (NA-CMC) polymer on aluminium in hcl solution, Niger. J. Technol., 2020, 39, 369–378 CrossRef.
- I. O. Arukalam, I. K. Nleme and A. E. Anyanwu, Comparative inhibitive effect of hydroxyethylcellulose on mild steel and aluminium corrosion in 0.5 M HCl solution, Acad. Res. Int., 2011, 1, 492 Search PubMed.
- S. A. Umoren, A. A. AlAhmary, Z. M. Gasem and M. M. Solomon, Evaluation of chitosan and carboxymethyl cellulose as ecofriendly corrosion inhibitors for steel, Int. J. Biol. Macromol., 2018, 117, 1017–1028 CrossRef CAS PubMed.
- I. Arukalam, I. Madufor, O. Ogbobe and E. Oguzie, Acidic corrosion inhibition of copper by hydroxyethyl cellulose, Curr. J. Appl. Sci. Technol., 2014, 1445–1460 CAS.
- M.-M. Li, Q.-J. Xu, J. Han, H. Yun and Y. Min, Inhibition action and adsorption behavior of green inhibitor sodium carboxymethyl cellulose on copper, Int. J. Electrochem. Sci., 2015, 10, 9028–9041 CAS.
- M. S. Hasanin and S. A. Al Kiey, Environmentally benign corrosion inhibitors based on cellulose niacin nano-composite for corrosion of copper in sodium chloride solutions, Int. J. Biol. Macromol., 2020, 161, 345–354 CrossRef CAS PubMed.
- T. Brinda, J. Mallika and V. Sathyanarayana, Moorthy, Synergistic effect between starch and substituted piperidin-4-one on the corrosion inhibition of mild steel in acidic medium, J. Mater. Environ. Sci., 2015, 6, 191–200 Search PubMed.
- M. Mobin, M. Khan and M. Parveen, Inhibition of mild steel corrosion in acidic medium using starch and surfactants additives, J. Appl. Polym. Sci., 2011, 121, 1558–1565 CrossRef CAS.
- M. Bello, N. Ochoa, V. Balsamo, F. López-Carrasquero, S. Coll, A. Monsalve and G. González, Modified cassava starches as corrosion inhibitors of carbon steel: An electrochemical and morphological approach, Carbohydr. Polym., 2010, 82, 561–568 CrossRef CAS.
- R. Rosliza and W. W. Nik, Improvement of corrosion resistance of AA6061 alloy by tapioca starch in seawater, Curr. Appl. Phys., 2010, 10, 221–229 CrossRef.
- X. Li and S. Deng, Cassava starch graft copolymer as an eco-friendly corrosion inhibitor for steel in H2SO4 solution, Korean J. Chem. Eng., 2015, 32, 2347–2354 CrossRef CAS.
- M. V. Fiori-Bimbi, P. E. Alvarez, H. Vaca and C. A. Gervasi, Corrosion inhibition of mild steel in HCL solution by pectin, Corros. Sci., 2015, 92, 192–199 CrossRef CAS.
- S. A. Umoren, I. B. Obot, A. Madhankumar and Z. M. Gasem, Performance evaluation of pectin as ecofriendly corrosion inhibitor for X60 pipeline steel in acid medium: Experimental and theoretical approaches, Carbohydr. Polym., 2015, 124, 280–291 CrossRef CAS PubMed.
- A. N. Grassino, J. Halambek, S. Djaković, S. R. Brnčić, M. Dent and Z. Grabarić, Utilization of tomato peel waste from canning factory as a potential source for pectin production and application as tin corrosion inhibitor, Food Hydrocolloids, 2016, 52, 265–274 CrossRef CAS.
- M. M. Fares, A. Maayta and M. M. Al-Qudah, Pectin as promising green corrosion inhibitor of aluminum in hydrochloric acid solution, Corros. Sci., 2012, 60, 112–117 CrossRef CAS.
- R. Geethanjali, A. Sabirneeza and S. Subhashini, Water-soluble and biodegradable pectin-grafted polyacrylamide and pectin-grafted polyacrylic acid: electrochemical investigation of corrosion-inhibition behaviour on mild steel in 3.5% NaCl media, Indian J. Mater. Sci., 2014, 2014, 1–9 CrossRef.
- B. Charitha and P. Rao, Pectin as a Potential Green Inhibitor for Corrosion Control of 6061Al–15%(V) SiC (P) Composite in Acid Medium: Electrochemical and Surface Studies, J. Fail. Anal. Prev., 2020, 1–13 Search PubMed.
- A. Biswas, D. Das, H. Lgaz, S. Pal and U. G. Nair, Biopolymer dextrin and poly (vinyl acetate) based graft copolymer as an efficient corrosion inhibitor for mild steel in hydrochloric acid: electrochemical, surface morphological and theoretical studies, J. Mol. Liq., 2019, 275, 867–878 CrossRef CAS.
- G. N. Devi, C. B. N. Unnisa, S. M. Roopan, V. Hemapriya, S. Chitra, I.-M. Chung, S.-H. Kim and M. Prabakaran, Floxacins: as Mediators in Enhancing the Corrosion Inhibition Efficiency of Natural Polymer Dextrin, Macromol. Res., 2020, 1–9 Search PubMed.
- A. Altin, M. Rohwerder and A. Erbe, Cyclodextrins as carriers for organic corrosion inhibitors in organic coatings, J. Electrochem. Soc., 2017, 164, C128 CrossRef CAS.
- S. Amiri and A. Rahimi, Anticorrosion behavior of cyclodextrins/inhibitor nanocapsule-based self-healing coatings, J. Coat. Technol. Res., 2016, 13, 1095–1102 CrossRef CAS.
- A. Khramov, N. Voevodin, V. Balbyshev and R. Mantz, Sol–gel-derived corrosion-protective coatings with controllable release of incorporated organic corrosion inhibitors, Thin Solid Films, 2005, 483, 191–196 CrossRef CAS.
- R. M. Hassan and I. A. Zaafarany, Kinetics of corrosion inhibition of aluminum in acidic media by water-soluble natural polymeric pectates as anionic polyelectrolyte inhibitors, Materials, 2013, 6, 2436–2451 CrossRef CAS.
- I. Zaafarany, Corrosion inhibition of aluminum in aqueous alkaline solutions by alginate and pectate water-soluble natural polymer anionic polyelectrolytes, Port. Electrochim. Acta, 2012, 30, 419–426 CrossRef CAS.
- S. M. Shaban, I. Aiad, A. H. Moustafa and O. H. Aljoboury, Some alginates polymeric cationic surfactants; surface study and their evaluation as biocide and corrosion inhibitors, J. Mol. Liq., 2019, 273, 164–176 CrossRef CAS.
- R. Hassan, I. Zaafarany, A. Gobouri and H. Takagi, A revisit to the corrosion inhibition of aluminum in aqueous alkaline solutions by water-soluble alginates and pectates as anionic polyelectrolyte inhibitors, Int. J. Corros., 2013, 2013, 1–8 CrossRef.
-
S. Umoren and E. Ebenso, Studies of the anti-corrosive effect of Raphia hookeri exudate gum-halide mixtures for aluminium corrosion in acidic medium, Pigment & Resin Technology, ( 2008) Search PubMed.
- S. Umoren, I. Obot, E. Ebenso and P. Okafor, Eco-friendly inhibitors from naturally occurring exudate gums for aluminium corrosion inhibition in acidic medium, Port. Electrochim. Acta, 2008, 26, 267–282 CrossRef CAS.
- J. Buchweishaija and G. Mhinzi, Natural products as a source of environmentally friendly corrosion inhibitors: the case of gum exudate from Acacia seyal var. seyal, Port. Electrochim. Acta, 2008, 26, 257–265 CrossRef CAS.
- S. Umoren, I. Obot, E. Ebenso and N. Obi-Egbedi, The Inhibition of aluminium corrosion in hydrochloric acid solution by exudate gum from Raphia hookeri, Desalination, 2009, 247, 561–572 CrossRef CAS.
- S. Umoren, I. Obot and E. Ebenso, Corrosion inhibition of aluminium using exudate gum from Pachylobus edulis in the presence of halide ions in HCl, J. Chem., 2008, 5, 1–10 Search PubMed.
- J. Buchweishaija, Plants as a source of green corrosion inhibitors: the case of gum exudates from Acacia species (A. drepanolobium and A. senegal), Tanz. J. Sci., 2009, 35, 93–106 Search PubMed.
- F. Waanders, S. Vorster and A. Geldenhuys, Biopolymer corrosion inhibition of mild steel: electrochemical/mössbauer results, Hyperfine Interact., 2002, 139, 133–139 CrossRef.
- S. A. Umoren and M. M. Solomon, Synergistic corrosion inhibition effect of metal cations and mixtures of organic compounds: a review, J. Environ. Chem. Eng., 2017, 5, 246–273 CrossRef CAS.
- D. E. Arthur, A. Jonathan, P. O. Ameh and C. Anya, A review on the assessment of polymeric materials used as corrosion inhibitor of metals and alloys, Int. J. Ind. Chem., 2013, 4, 2 CrossRef.
- A. Peter, I. Obot and S. K. Sharma, Use of natural gums as green corrosion inhibitors: an overview, Int. J. Ind. Chem., 2015, 6, 153–164 CrossRef CAS.
- S. Mo, H.-Q. Luo and N.-B. Li, Plant extracts as “green” corrosion inhibitors for steel in sulphuric acid, Chem. Pap., 2016, 70, 1131–1143 Search PubMed.
- A. Miralrio and A. Espinoza Vázquez, Plant Extracts as Green Corrosion Inhibitors for Different Metal Surfaces and Corrosive Media: A Review, Processes, 2020, 8, 942 CrossRef CAS.
- A. Fateh, M. Aliofkhazraei and A. Rezvanian, Review of corrosive environments for copper and its corrosion inhibitors, Arabian J. Chem., 2020, 13, 481–544 CrossRef CAS.
- A. Hamilton-Amachree and N. B. Iroha, Corrosion inhibition of API 5L X80 pipeline steel in acidic environment using aqueous extract of Thevetia peruviana, Chem. Int., 2020, 6, 117–128 Search PubMed.
- V. Izionworu, C. Ukpaka and E. Oguzie, Green and eco-benign corrosion inhibition agents: Alternatives and options to chemical based toxic corrosion inhibitors, Chem. Int., 2020, 6, 232–259 CAS.
- R. Yadav and M. Agarwala, Phytochemical analysis of some medicinal plants, J. Phytol., 2011, 3, 10–14 CAS.
- A. Altemimi, N. Lakhssassi, A. Baharlouei, D. G. Watson and D. A. Lightfoot, Phytochemicals: Extraction, isolation, and identification of bioactive compounds from plant extracts, Plants, 2017, 6, 42 CrossRef PubMed.
- D. O. Kennedy and E. L. Wightman, Herbal extracts and phytochemicals: plant secondary metabolites and the enhancement of human brain function, Adv. Nutr., 2011, 2, 32–50 CrossRef CAS PubMed.
- G. Bahlakeh, M. Ramezanzadeh and B. Ramezanzadeh, Experimental and theoretical studies of the synergistic inhibition effects between the plant leaves extract (PLE) and zinc salt (ZS) in corrosion control of carbon steel in chloride solution, J. Mol. Liq., 2017, 248, 854–870 CrossRef CAS.
- N. O. Eddy, S. A. Odoemelam and A. O. Odiongenyi, Inhibitive, adsorption and synergistic studies on ethanol extract of Gnetum africana as green corrosion inhibitor for mild steel in H2SO4, Green Chem. Lett. Rev., 2009, 2, 111–119 CrossRef CAS.
- J. Tan, L. Guo, H. Yang, F. Zhang and Y. El Bakri, Synergistic effect of potassium iodide and sodium dodecyl sulfonate on the corrosion inhibition of carbon steel in HCl medium: a combined experimental and theoretical investigation, RSC Adv., 2020, 10, 15163–15170 RSC.
- C. Verma, E. E. Ebenso, I. Bahadur and M. Quraishi, An overview on plant extracts as environmental sustainable and green corrosion inhibitors for metals and alloys in aggressive corrosive media, J. Mol. Liq., 2018, 266, 577–590 CrossRef CAS.
- M. Mehdipour, B. Ramezanzadeh and S. Arman, Electrochemical noise investigation of Aloe plant extract as green inhibitor on the corrosion of stainless steel in 1 M H2SO4, J. Ind. Eng. Chem., 2015, 21, 318–327 CrossRef CAS.
- M. N. Jane and T. M. Nakara, Determination of the Corrosion Inhibition Effect of Terminalia Ivorensis Leaves Extract on Galvanized and Mild Steel in Sulphuric Acid Media, American, J. Phys. Chem., 2019, 8, 11 CAS.
- K. H. Hassan, A. A. Khadom and N. H. Kurshed, Citrus aurantium leaves extracts as a sustainable corrosion inhibitor of mild steel in sulfuric acid, S. Afr. J. Chem. Eng., 2016, 22, 1–5 Search PubMed.
- P. Okafor, I. Uwah, O. Ekerenam and U. Ekpe, Combretum bracteosum extracts as eco-friendly corrosion inhibitor for mild steel in acidic medium, Pigm. Resin Technol., 2009, 38, 236–241 CrossRef CAS.
- S. Umoren, U. Eduok, M. Solomon and A. Udoh, Corrosion inhibition by leaves and stem extracts of Sida acuta for mild steel in 1 M H2SO4 solutions investigated by chemical and spectroscopic techniques, Arabian J. Chem., 2016, 9, S209–S224 CrossRef CAS.
- P. Muthukrishnan, B. Jeyaprabha and P. Prakash, Mild steel corrosion inhibition by aqueous extract of Hyptis suaveolens leaves, Int. J. Ind. Chem., 2014, 5, 5 CrossRef.
- A. Abdulrahman, K. Ganiyu and I. Kobe, A. Caroline, The corrosion inhibition of mild steel in sulphuric acid solution by adsorption of African Perquetina leaves extract, Int. J. Innovative Res. Sci. Eng. Technol., 2015, 4, 1809–1821 CrossRef.
- V. Vasudha and K. S. Priya, Corrosion inhibition of mild steel in H2SO4 media using Polyalthia longifolia leaves, Chem. Sci. Rev. Lett., 2014, 2, 435–443 Search PubMed.
- K. Ramya and N. Muralimohan, Study on Corrosion Inhibitor in Mild Steel by Various Habitual Plant Extract–Review, Int. J. Chem. Concepts, 2016, 2, 70–75 CAS.
- R. Lopes-Sesenes, J. Gonzalo Gonzalez-Rodruguez, G. Francisca Dominguez-Patino and A. Marinez-Villafane, Corrosion inhibition of carbon steel by extract of Buddleia perfoliata, J. Electrochem. Sci. Eng., 2012, 2, 77–90 CAS.
- A. Singh, Cassia tora Leaves Extract as Mild Steel Corrosion Inhibitor in Sulphuric Acid Solution, Imp. J. Interdiscip. Res., 2016, 2, 1–3 Search PubMed.
- O. A. Omotosho, O. O. Ajayi, O. S. Fayomi and V. O. Ifepe, Assessing the deterioration behaviour of mild steel in 2 M sulphuric acid using Bambusa glauscescens, Int. J. Appl. Eng. Res., Dindigul, 2011, 2, 406–418 CAS.
- J. Bhawsar, P. Jain and P. Jain, Experimental and computational studies of Nicotiana tabacum leaves extract as green corrosion inhibitor for mild steel in acidic medium, Alex. Eng. J., 2015, 54, 769–775 CrossRef.
- A. Caroline, A. Abdulrahaman, I. Kobe, K. Ganiyu and S. Adams, Inhibitive performance of bitter leaf root extract on mild steel corrosion in sulphuric acid solution, Am. J. Mater. Eng. Technol., 2015, 3, 35–45 Search PubMed.
- O. O. Dominic and O. Monday, Optimization Of The Inhibition Efficiency Of Mango Extract As Corrosion Inhibitor Of Mild Steel In 1.0 MH2SO4 Using Response Surface Methodology, J. Chem. Technol. Metall., 2016, 51, 302–314 CAS.
- M. Al-Ghorbani, B. Begum, M. S. Zabiulla and S. A. Khanum, J. Chem. Pharm. Res., 2015, 7(5), 281–301 CAS.
- F. O. Nwosu, L. A. Nnanna and K. Okeoma, Corrosion inhibition for mild steel in 0.5 M H2SO4 solution using Achyranthes aspera L. leaf extract, Afr. J. Pure Appl. Chem., 2013, 7, 56–60 CAS.
- S. A. Kumar, A. Sankar and S. Rameshkumar, Oxystelma esculentum leaves extracts as corrosion inhibitor for mild steel in acid medium, Int. J. Sci. Technol. Res., 2013, 2, 55–58 CrossRef.
- P. Ejikeme, S. Umana, M. Menkiti and O. Onukwuli, Inhibition of Mild Steel and Aluminium Corrosion in 1M H2SO4 by Leaves Extract of African Breadfruit, Int. J. Mater. Chem., 2015, 5, 14–23 Search PubMed.
- O. U. Abakedi, V. F. Ekpo and E. E. John, Corrosion inhibition of mild steel by Stachytarpheta indica leaf extract in acid medium, Pharm. Chem. J., 2016, 3, 165–171 CAS.
- J. Omotoyinbo, D. Oloruntoba and S. Olusegun, Corrosion Inhibition of Pulverized Jatropha Curcas Leaves on Medium Carbon Steel in 0.5 M H2SO4 and NaCl Environments, Int. J. Sci. Technol., 2013, 2, 510–514 Search PubMed.
- U. Eduok, S. Umoren and A. Udoh, Synergistic inhibition effects between leaves and stem extracts of Sida acuta and iodide ion for mild steel corrosion in 1 M H2SO4 solutions, Arabian J. Chem., 2012, 5, 325–337 CrossRef CAS.
- N. Patel, S. Jauhariand, G. Mehta, S. Al-Deyab, I. Warad and B. Hammouti, Mild steel corrosion inhibition by various plant extracts in 0.5 M sulphuric acid, Int. J. Electrochem. Sci., 2013, 8, 2655 Search PubMed.
- I. Uwah, P. Okafor and V. Ebiekpe, Inhibitive action of ethanol extracts from Nauclea latifolia on the corrosion of mild steel in H2SO4 solutions and their adsorption characteristics, Arabian J. Chem., 2013, 6, 285–293 CrossRef CAS.
- A. El-Etre, Inhibition of acid corrosion of carbon steel using aqueous extract of olive leaves, J. Colloid Interface Sci., 2007, 314, 578–583 CrossRef CAS PubMed.
-
M. Mahat, M. Kamarudin, J. Isa, N. Bonnia and N. Jani, Azadirachta excelsa as green corrosion inhibitor for mild steel in acidic medium, Business, Engineering and Industrial Applications (ISBEIA), 2012 IEEE Symposium on, IEEE, 2012, pp. 538–541.
- K. Rose, B.-S. Kim, K. Rajagopal, S. Arumugam and K. Devarayan, Surface protection of steel in acid medium by Tabernaemontana divaricata extract: Physicochemical evidence for adsorption of inhibitor, J. Mol. Liq., 2016, 214, 111–116 CrossRef CAS.
- F. E. Awe, S. O. Idris, M. Abdulwahab and E. E. Oguzie, Theoretical and experimental inhibitive properties of mild steel in HCl by ethanolic extract of Boscia senegalensis, Cogent Chem., 2015, 1, 1112676 CrossRef.
- K. Anupama, K. Ramya and A. Joseph, Electrochemical and computational aspects of surface interaction and corrosion inhibition of mild steel in hydrochloric acid by Phyllanthus amarus leaf extract (PAE), J. Mol. Liq., 2016, 216, 146–155 CrossRef CAS.
- A. Rajendran and C. Karthikeyan, The inhibitive effect of extract of flowers of Cassia auriculata in 2 M HCl on the corrosion of aluminium and mild steel, Int. J. Plant Res., 2012, 2, 9–14 CrossRef.
- V. Rajeswari, D. Kesavan, M. Gopiraman, P. Viswanathamurthi, K. Poonkuzhali and T. Palvannan, Corrosion inhibition of Eleusine aegyptiaca and Croton rottleri leaf extracts on cast iron surface in 1 M HCl medium, Appl. Surf. Sci., 2014, 314, 537–545 CrossRef CAS.
- S. Umoren, Z. Gasem and I. Obot, Date palm (Phoenix dactylifera) leaf extract as an eco-friendly corrosion inhibitor for carbon steel in 1M hydrochloric acid solution, Anti-Corros. Methods Mater., 2015, 62, 19–28 CrossRef CAS.
- K. Anupama, K. Ramya, K. Shainy and A. Joseph, Adsorption and electrochemical studies of Pimenta dioica leaf extracts as corrosion inhibitor for mild steel in hydrochloric acid, Mater. Chem. Phys., 2015, 167, 28–41 CrossRef CAS.
- K. Anupama, K. Shainy and A. Joseph, Excellent anticorrosion behavior of Ruta Graveolens extract (RGE) for mild
steel in hydrochloric acid: electro analytical studies on the effect of time, temperature, and inhibitor concentration, J. Bio-and Tribo-Corros., 2016, 2, 2 CrossRef.
- T. Ibrahim, H. Alayan and Y. Al Mowaqet, The effect of Thyme leaves extract on corrosion of mild steel in HCl, Prog. Org. Coat., 2012, 75, 456–462 CrossRef CAS.
- J. Rosaline Vimala, A. Leema Rose and S. Raja, A study on the phytochemical analysis and corrosion inhibition on mild steel by Annona Muricata. L leaves extract in 1N Hydrochloric Acid, Der Chem. Sin., 2012, 3, 582–588 Search PubMed.
- A. Lecante, F. Robert, P. Blandinières and C. Roos, Anti-corrosive properties of S. tinctoria and G. ouregou alkaloid extracts on low carbon steel, Curr. Appl. Phys., 2011, 11, 714–724 CrossRef.
- L. Li, W. Xu, J. Lei, J. Wang, J. He, N. Li and F. Pan, Experimental and theoretical investigations of Michelia alba leaves extract as a green highly-effective corrosion inhibitor for different steel materials in acidic solution, RSC Adv., 2015, 5, 93724–93732 RSC.
- M. Jokar, T. S. Farahani and B. Ramezanzadeh, Electrochemical and surface characterizations of morus alba pendula leaves extract (MAPLE) as a green corrosion inhibitor for steel in 1 M HCl, J. Taiwan Inst. Chem. Eng., 2016, 63, 436–452 CrossRef CAS.
- L. Nnanna, W. John, E. Emenike, U. Ihekoronye and I. Dike, Corrosion Inhibition Effect of Anthocleista Djalonensis on Mild Steel in 1.0 M HCL, Int. J. Eng. Res. Rev., 2015, 3, 31–36 Search PubMed.
- K. Krishnaveni, J. Ravichandran and A. Selvaraj, Effect of Morinda tinctoria leaves extract on the corrosion inhibition of mild steel in acid medium, Arabian J. Chem., 2013, 26, 321–327 CAS.
- P. Muthukrishnan, P. Prakash, B. Jeyaprabha and K. Shankar, Stigmasterol extracted from Ficus hispida leaves as a green inhibitor for the mild steel corrosion in 1 M HCl solution, Arab. J. Chem., 2015, 12, 3345–3356 CrossRef.
- K. Hema, A. Sirajunnisa, B. Venkatraman and A. Subramania, The Effect of Solanam Xanthocarpum Leaves Extract on Corrosion Inhibition of Carbon Steel in Acidic Medium, Int. J. Adv. Res. Chem. Sci., 2015, 2, 10–20 Search PubMed.
- A. Sirajunnisa, M. F. Mohamed, A. Subramania and B. Venkatraman, The inhibitive effect of Ziziphus jujuba leaves extract on the alkaline corrosion of aluminium, Eur. J. Appl. Sci. Technol., 2014, 1, 23–31 Search PubMed.
- A. Okoronkwo, S. Olusegun and O. Olaniran, Acid extract of Gliricidia sepium leaves as green corrosion inhibitor for mild steel in HCl solutions, African Corr. J., 2015, 1, 30–35 Search PubMed.
- P. Deivanayagam, I. Malarvizhi and S. Selvaraj, Alcoholic extract of “Gymnema Sylvestre” leaves on mild steel in acid medium Quinquefasciatus say, Int. J. Adv. Sci. Res., 2016, 1, 21–27 Search PubMed.
- M. ur Rahman, S. Gul, M. Umair, A. Anwar and A. Achakzai, Anticorrosive Activity of Rosemarinus officinalis L. Leaves Extract Against Mild Steel in Dilute Hydrochloric Acid, Int. J. Innovative Res. Adv. Eng., 2016, 3, 38–43 Search PubMed.
- G. Ji, S. K. Shukla, P. Dwivedi, S. Sundaram and R. Prakash, Inhibitive effect of Argemone mexicana plant extract on acid corrosion of mild steel, Ind. Eng. Chem. Res., 2011, 50, 11954–11959 CrossRef CAS.
- S. Ulaeto, U. Ekpe, M. Chidiebere and E. Oguzie, Corrosion inhibition of mild steel in hydrochloric acid by acid extracts of Eichhornia crassipes, Int. J. Mater. Chem., 2012, 2, 158–164 CAS.
- P. M. Krishnegowda, V. T. Venkatesha, P. K. M. Krishnegowda and S. B. Shivayogiraju, Acalypha torta leaf extract as green corrosion inhibitor for mild steel in hydrochloric acid solution, Ind. Eng. Chem. Res., 2013, 52, 722–728 CrossRef CAS.
- A. Sharmila, A. A. Prema and P. A. Sahayaraj, Influence of Murraya koenigii (curry leaves) extract on the corrosion inhibition of carbon steel in HCL solution, Rasayan J. Chem., 2010, 3, 74–81 Search PubMed.
- M. N. Fathima, A. Prithiba, S. Leelavathi and R. Rajalakshmi, Investigation of Podranea ricasolianaLeaves Extract as Green Corrosion Inhibitor for Mild Steel in 1M HCl, Int. J. Adv. Sci. Res. Manage., 2016, 1, 123–130 Search PubMed.
- S. Muthumanickam, B. Jeyaprabha, R. Karthik, A. Elangovan and P. Prakash, Adsorption and corrosion inhibiting behavior of Passiflora foetida leaf extract on mild steel corrosion, Int. J. Corros. Scale Inhib., 2015, 4, 365–381 CrossRef CAS.
-
S. Kuppusamy, T. Palanisami, M. Megharaj, K. Venkateswarlu and R. Naidu, In-situ remediation approaches for the management of contaminated sites: a comprehensive overview, Reviews of Environmental Contamination and Toxicology, Springer, 2016, vol. 236, pp. 1–115 Search PubMed.
- M. Ikpi, I. Udoh, P. Okafor, U. Ekpe and E. Ebenso, Corrosion inhibition and adsorption behaviour of extracts from Piper guineensis on mild steel corrosion in acid media, Int. J. Electrochem. Sci., 2012, 7, 12193–12206 CAS.
- I. Iloamaeke, T. Onuegbu, V. Ajiwe and U. Umeobika, Corrosion inhibition of mild steel by pterocarpus soyauxi leaves extract in HCL Medium, Int. J. Plant, Anim. Environ. Sci., 2012, 2, 22–28 CAS.
- S. Firdausi and F. Kurniawan, Corrosion Inhibition by Tithonia diversifolia (Hemsl) A. Gray leaves extract for 304 SS in hydrochloric acid solution, J. Phys.: Conf. Ser., 2016, 012042 CrossRef.
- M. Bodude and O. Sanni, Evaluation of Inhibitive Performance of Some Plants Extracts on Low Carbon Steel Corrosion, Stud. Eng. Technol., 2014, 1, 21–28 CrossRef.
- L. A. Nnanna and I. O. Owate, Electrochemical Study of Corrosion Inhibition of Mild Steel in Acidic Solution Using Gnetum africana Leaves Extracts, Br. J. Appl. Sci. Technol., 2015, 5, 556 CrossRef.
- R. D’Souza and A. Chattree, Emblica Officinalis Leaves Extract as Corrosion Inhibitor, Chem. Sci. Trans., 2015, 4, 865–870 Search PubMed.
- G. M. Al-Senani, S. I. Al-Saeedi and R. Almufarij, Green corrosion inhibitors for carbon steel by green leafy vegetables extracts in 1 M HCl, Orient. J. Chem., 2015, 31, 2077–2086 CrossRef CAS.
- H. H. Al-Sahlanee, A.-W. A. Sultan and M. M. Al-Faize, Corrosion Inhibition of Carbon Steel in 1M HCl Solution Using Sesbania Sesban Extract, Aqua. Sci. Technol., 2013, 1, 135–151 Search PubMed.
- D. K. Verma and F. Khan, Corrosion inhibition of mild steel by extract of Bryophyllum pinnatum leaves in acidic solution, Chem. Mater. Res., 2015, 7, 69–76 Search PubMed.
- E. E. Oguzie, Corrosion inhibition of aluminium in acidic and alkaline media by Sansevieria trifasciata extract, Corros. Sci., 2007, 49, 1527–1539 CrossRef CAS.
- A. Singh, I. Ahamad and M. A. Quraishi, Piper longum extract as green corrosion inhibitor for aluminium in NaOH solution, Arabian J. Chem., 2016, 9, S1584–S1589 CrossRef CAS.
- O. K. Abiola, J. Otaigbe and O. Kio, Gossipium hirsutum L. extracts as green corrosion inhibitor for aluminum in NaOH solution, Corros. Sci., 2009, 51, 1879–1881 CrossRef CAS.
- D. I. Njoku, G. N. Onuoha, E. E. Oguzie, K. L. Oguzie, A. A. Egbedina and A. N. Alshawabkeh, Nicotiana tabacum leaf extract protects aluminium alloy AA3003 from acid attack, Arabian J. Chem., 2016, 12, 4466–4478 CrossRef.
- O. K. Abiola and J. Otaigbe, The effects of Phyllanthus amarus extract on corrosion and kinetics of corrosion process of aluminum in alkaline solution, Corros. Sci., 2009, 51, 2790–2793 CrossRef CAS.
- N. Chaubey, D. K. Yadav, V. K. Singh and M. Quraishi, A comparative study of leaves extracts for corrosion inhibition effect on aluminium alloy in alkaline medium, Ain Shams Eng. J., 2015, 8, 673–682 CrossRef.
- S. Deng and X. Li, Inhibition by Jasminum nudiflorum Lindl. leaves extract of the corrosion of aluminium in HCl solution, Corros. Sci., 2012, 64, 253–262 CrossRef CAS.
- K. Ajanaku, C. Ajanaku, A. Akinsiku, A. Falomo, A. Edobor-Osoh and M. John, Eco-friendly Impact of Vernonia amygdalina as corrosion inhibitor on aluminium in acidic media, Chem. J., 2012, 2, 153–157 Search PubMed.
- N. Raghavendra and J. I. Bhat, Green approach to inhibition of corrosion of aluminum in 0.5 M HCl medium by tender arecanut seed extract: insight from gravimetric and electrochemical studies, Res. Chem. Intermed., 2016, 42, 6351–6372 CrossRef CAS.
- A. Khadraoui, A. Khelifa, K. Hachama and R. Mehdaoui, Thymus algeriensis extract as a new eco-friendly corrosion inhibitor for 2024 aluminium alloy in 1 M HCl medium, J. Mol. Liq., 2016, 214, 293–297 CrossRef CAS.
- O. K. Abiola and Y. Tobun, Cocos nucifera L. water as green corrosion inhibitor for acid corrosion of aluminium in HCl solution, Chin. Chem. Lett., 2010, 21, 1449–1452 CrossRef CAS.
- O. Abakedi and I. Moses, Aluminium corrosion inhibition by Maesobatrya barteri root extract in hydrochloric acid solution, Am. Chem. Sci. J., 2016, 10, 1–10 CrossRef CAS.
- K. Krishnaveni and J. Ravichandran, Effect of aqueous extract of leaves of Morinda tinctoria on corrosion inhibition of aluminium surface in HCl medium, Trans. Nonferrous Met. Soc. China, 2014, 24, 2704–2712 CrossRef CAS.
- G. Gece, Drugs: A review of promising novel corrosion inhibitors, Corros. Sci., 2011, 53, 3873–3898 CrossRef CAS.
- R. Pathak and P. Mishra, Drugs as corrosion inhibitors: a review, Int. J. Sci. Res., 2016, 5, 671–677 Search PubMed.
- Y. Liangtian, M. Zhang, C. Shidong, T. Yunji and W. Haixia, Investigation of Corrosion Inhibition Effect of Enprofylline Drug on Mild Steel Corrosion in Sulphuric Acid Solution, Int. J. Electrochem. Sci., 2020, 15, 5102–5114 CrossRef.
- M. Alfakeer, M. Abdallah and A. Fawzy, Corrosion Inhibition Effect of Expired Ampicillin and Flucloxacillin Drugs for Mild Steel in Aqueous Acidic Medium, Int. J. Electrochem. Sci., 2020, 15, 3283–3297 CrossRef CAS.
- C. Verma, D. Chauhan and M. Quraishi, Drugs as environmentally benign corrosion inhibitors for ferrous and nonferrous materials in acid environment: an overview, J. Mater. Environ. Sci. (JMES), 2017, 8, 4040–4051 CAS.
- M. Abdallah, Antibacterial drugs as corrosion inhibitors for corrosion of aluminium in hydrochloric solution, Corros. Sci., 2004, 46, 1981–1996 CrossRef CAS.
- H. I. Al-Shafey, R. A. Hameed, F. Ali, A.-M. Ae-AS and M. Salah, Effect of expired drugs as corrosion inhibitors for carbon steel in 1M HCL solution, Int. J. Pharm. Sci. Rev. Res., 2014, 27, 146–152 CAS.
- P. Singh, D. S. Chauhan, K. Srivastava, V. Srivastava and M. Quraishi, Expired atorvastatin drug as corrosion inhibitor for mild steel in hydrochloric acid solution, Int. J. Ind. Chem., 2017, 8, 363–372 CrossRef CAS.
- N. Vaszilcsin, D.-A. Duca, A. Flueraş and M.-L. Dana, Expired drugs as inhibitors in electrochemical processes–a mini-review, Stud. Univ. Babes-Bolyai, Chem., 2019, 3, 17–32 Search PubMed.
- P. Singh, D. Chauhan, S. Chauhan, G. Singh and M. Quraishi, Chemically modified expired Dapsone drug as environmentally benign corrosion inhibitor for mild steel in sulphuric acid useful for industrial pickling process, J. Mol. Liq., 2019, 286, 110903 CrossRef CAS.
- G. Golestani, M. Shahidi and D. Ghazanfari, Electrochemical evaluation of antibacterial drugs as environment-friendly inhibitors for corrosion of carbon steel in HCl solution, Appl. Surf. Sci., 2014, 308, 347–362 CrossRef CAS.
- C. Verma, M. Quraishi and N. K. Gupta, 2-(4-{[4-Methyl-6-(1-methyl-1H-1, 3-benzodiazol-2-yl)-2-propyl-1H-1, 3-benzodiazol-1-yl] methyl} phenyl) benzoic acid as green corrosion inhibitor for mild steel in 1 M hydrochloric acid, Ain Shams Eng. J., 2018, 9, 1225–1233 CrossRef.
- L. Yanhong, L. Changcheng, Y. Liangmin, N. Chunhua and Z. Shizheng, Development Progress of Antifouling Agents and Accelerated Test Methods for Antifouling Coatings, Shanghai Coat., 2013, 1 Search PubMed , https://en.cnki.com.cn/Article_en/CJFDTotal-SHTL201301009.htm.
- I. B. Obot, E. E. Ebenso and M. M. Kabanda, Metronidazole as environmentally safe corrosion inhibitor for mild steel in 0.5 M HCl: experimental and theoretical investigation, J. Environ. Chem. Eng., 2013, 1, 431–439 CrossRef CAS.
- G. Karthik and M. Sundaravadivelu, Studies on the inhibition of mild steel corrosion
in hydrochloric acid solution by atenolol drug, Egypt. J. Pet., 2016, 25, 183–191 CrossRef.
- I. Reza, A. Saleemi and S. Naveed, Corrosion inhibition of mild steel in HCl solution by Tinidazole, Pol. J. Chem. Technol., 2011, 13, 67–71 CrossRef.
- J. Aldana-González, A. Espinoza-Vázquez, M. Romero-Romo, J. Uruchurtu-Chavarin and M. Palomar-Pardavé, Electrochemical evaluation of cephalothin as corrosion inhibitor for API 5L X52 steel immersed in an acid medium, Arabian J. Chem., 2019, 12, 3244–3253 CrossRef.
- A. Singh, A. Gupta, A. K. Rawat, K. Ansari, M. Quraishi and E. E. Ebenso, Cimetidine as an effective corrosion inhibitor for mild steel in hydrochloric acid, Int. J. Electrochem. Sci., 2014, 9, 7614–7628 Search PubMed.
- A. Samide, B. Tutunaru, C. Ionescu, P. Rotaru and L. Simoiu, Aminophylline: thermal characterization and its inhibitory properties for the carbon steel corrosion in acidic environment, J. Therm. Anal. Calorim., 2014, 118, 631–639 CrossRef CAS.
- N. O. Eddy, E. E. Ebenso and U. J. Ibok, Adsorption, synergistic inhibitive effect and quantum chemical studies of ampicillin (AMP) and halides for the corrosion of mild steel in H2SO4, J. Appl. Electrochem., 2010, 40, 445–456 CrossRef CAS.
- I. Akpan and N. Offiong, Electrochemical Linear Polarization Studies of Amodiaquine Drug as a Corrosion Inhibitor for Mild Steel in 0.1 M HCL Solution, Chem. Mater. Res., 2015, 7, 17–20 Search PubMed.
- S. K. Shukla and M. Quraishi, The effects of pharmaceutically active compound doxycycline on the corrosion of mild steel in hydrochloric acid solution, Corros. Sci., 2010, 52, 314–321 CrossRef CAS.
- N. Eddy, S. Odoemelam and A. Mbaba, Inhibition of the corrosion of mild steel in HCl by sparfloxacin, Afr. J. Pure Appl. Chem., 2008, 2, 132–138 Search PubMed.
- S. K. Shukla, A. K. Singh, I. Ahamad and M. Quraishi, Streptomycin: A commercially available drug as corrosion inhibitor for mild steel in hydrochloric acid solution, Mater. Lett., 2009, 63, 819–822 CrossRef CAS.
- I. Obot and N. Obi-Egbedi, Adsorption properties and inhibition of mild steel corrosion in sulphuric acid solution by ketoconazole: experimental and theoretical investigation, Corros. Sci., 2010, 52, 198–204 CrossRef CAS.
- I. Reza, E. Ahmad and F. Kareem, Corrosion inhibition mechanism of Piperacillin Sodium for mild steel protection in acidic media, Afinidad, 2012, 69, 47–52 CAS.
- A. K. Singh and M. Quraishi, Effect of Cefazolin on the corrosion of mild steel in HCl solution, Corros. Sci., 2010, 52, 152–160 CrossRef CAS.
- I. A. Akpan and N.-A. O. Offiong, Inhibition of mild steel corrosion in hydrochloric acid solution by Ciprofloxacin drug, Int. J. Corros., 2013, 2013, 1–5 CrossRef.
- I. Ahamad, R. Prasad and M. A. Quraishi, Experimental and theoretical investigations of adsorption of fexofenadine at mild steel/hydrochloric acid interface as corrosion inhibitor, J. Solid State Electrochem., 2010, 14, 2095–2105 CrossRef CAS.
- P. Singh, M. Quraishi and E. E. Ebenso, Investigation of gliclazide drug as novel corrosion inhibitor for mild steel in 1 M HCl solution, Int. J. Electrochem. Sci., 2012, 7, 12270–12282 CAS.
- S. K. Shukla and M. Quraishi, Ceftriaxone: a novel corrosion inhibitor for mild steel in hydrochloric acid, J. Appl. Electrochem., 2009, 39, 1517–1523 CrossRef CAS.
- C. Verma, M. Quraishi and E. Ebenso, Electrochemical Studies of 2-amino-1, 9-dihydro-9-((2-hydroxyethoxy) methyl)-6H-purin-6-one as Green Corrosion Inhibitor for Mild Steel in 1.0 M Hydrochloric Acid Solution, Int. J. Electrochem. Sci., 2013, 8, 7401–7413 CAS.
- A. K. Singh and M. Quraishi, Inhibitive effect of diethylcarbamazine on the corrosion of mild steel in hydrochloric acid, Corros. Sci., 2010, 52, 1529–1535 CrossRef CAS.
- I. Naqvi, A. Saleemi and S. Naveed, Cefixime: A drug as efficient corrosion inhibitor for mild steel in acidic media. Electrochemical and thermodynamic studies, Int. J. Electrochem. Sci., 2011, 6, 146–161 CAS.
- P. Singh, A. Singh, M. Quraishi and E. E. Ebenso, Cetirizine: a new and effective corrosion inhibitor for mild steel in 1 M HCl solution, Int. J. Electrochem. Sci., 2012, 7, 7065–7079 CAS.
- J. I. Bhat and V. D. Alva, Meclizine hydrochloride as a potential non-toxic corrosion inhibitor for mild steel in hydrochloric acid medium, Arch. Appl. Sci. Res., 2011, 3, 343–356 Search PubMed.
- P. B. Matad, P. B. Mokshanatha, N. Hebbar, V. T. Venkatesha and H. C. Tandon, Ketosulfone drug as a green corrosion inhibitor for mild steel in acidic medium, Ind. Eng. Chem. Res., 2014, 53, 8436–8444 CrossRef CAS.
- A. Singh, E. E. Ebenso and M. Quraishi, Theoretical and electrochemical studies of metformin as corrosion inhibitor for mild steel in hydrochloric acid solution, Int. J. Electrochem. Sci., 2012, 7, 4766–4779 CAS.
- R. Farahati, S. M. Mousavi-Khoshdel, A. Ghaffarinejad and H. Behzadi, Experimental and computational study of penicillamine drug and cysteine as water-soluble green corrosion inhibitors of mild steel, Prog. Org. Coat., 2020, 142, 105567 CrossRef CAS.
- K. Haruna, T. A. Saleh and M. Quraishi, Expired metformin drug as green corrosion inhibitor for simulated oil/gas well acidizing environment, J. Mol. Liq., 2020, 113716 CrossRef CAS.
- J. S. Wilkes, A short history of ionic liquids—from molten salts to neoteric solvents, Green Chem., 2002, 4, 73–80 RSC.
- K. N. Marsh, J. A. Boxall and R. Lichtenthaler, Room temperature ionic liquids and their mixtures—a review, Fluid Phase Equilib., 2004, 219, 93–98 CrossRef CAS.
- K. E. Johnson, What's an ionic liquid?, Interface-Electrochem. Soc., 2007, 16, 38–41 CrossRef CAS.
- N. Bicak, A new ionic liquid: 2-hydroxy ethylammonium formate, J. Mol. Liq., 2005, 116, 15–18 CrossRef CAS.
- S. Carda–Broch, A. Berthod and D. Armstrong, Solvent properties of the 1-butyl-3-methylimidazolium hexafluorophosphate ionic liquid, Anal. Bioanal. Chem., 2003, 375, 191–199 CrossRef PubMed.
- N. V. Plechkova and K. R. Seddon, Applications of ionic liquids in the chemical industry, Chem. Soc. Rev., 2008, 37, 123–150 RSC.
- D. R. MacFarlane, N. Tachikawa, M. Forsyth, J. M. Pringle, P. C. Howlett, G. D. Elliott, J. H. Davis, M. Watanabe, P. Simon and C. A. Angell, Energy applications of ionic liquids, Energy Environ. Sci., 2014, 7, 232–250 RSC.
- J. Lu, F. Yan and J. Texter, Advanced applications of ionic liquids in polymer science, Prog. Polym. Sci., 2009, 34, 431–448 CrossRef CAS.
- D. Wei and A. Ivaska, Applications of ionic liquids in electrochemical sensors, Anal. Chim. Acta, 2008, 607, 126–135 CrossRef CAS PubMed.
- M. Deyab, Understanding the anti-corrosion mechanism and performance of ionic liquids in desalination, petroleum, pickling, de-scaling, acid cleaning applications, J. Mol. Liq., 2020, 113107 CrossRef CAS.
- E. K. Ardakani, E. Kowsari and A. Ehsani, Imidazolium-derived polymeric ionic liquid as a green inhibitor for corrosion inhibition of mild steel in 1.0 M HCl: Experimental and computational study, Colloids Surf., A, 2020, 586, 124195 CrossRef.
- R. Ratti, Ionic Liquids: Synthesis and Applications in Catalysis, Adv. Chem, 2014, 2014, 1–16 CrossRef.
- A. N. Masri, A. M. Mi and J.-M. Leveque, A review on dicationic ionic liquids: classification and application, Ind. Eng. Manag., 2016, 5, 197–204 Search PubMed.
- C. A. Angell, Y. Ansari and Z. Zhao, Ionic liquids: past, present and future, Faraday Discuss., 2012, 154, 9–27 RSC.
- S. Amiril, E. Rahim and S. Syahrullail, A review on ionic liquids as sustainable lubricants in manufacturing and engineering: Recent research, performance, and applications, J. Cleaner Prod., 2017, 168, 1571–1589 CrossRef CAS.
- S. Cao, D. Liu, P. Zhang, L. Yang, P. Yang, H. Lu and J. Gui, Green Brönsted acid ionic liquids
as novel corrosion inhibitors for carbon steel in acidic medium, Sci. Rep., 2017, 7, 1–14 CrossRef PubMed.
- P. Hu, Z. Wu, J. Wang, Y. Huang, Q. Liu and S.-F. Zhou, Corrosion inhibiting performance and mechanism of protic ionic liquids as green brass inhibitors in nitric acid, Green Energy Environ., 2020, 5, 214–222 CrossRef.
- T. Zhou, J. Yuan, Z. Zhang, X. Xin and G. Xu, The comparison of imidazolium Gemini surfactant [C14-4-C14im] Br2 and its corresponding monomer as corrosion inhibitors for A3 carbon steel in hydrochloric acid solutions: Experimental and quantum chemical studies, Colloids Surf., A, 2019, 575, 57–65 CrossRef CAS.
- Y. Qiang, S. Zhang, L. Guo, X. Zheng, B. Xiang and S. Chen, Experimental and theoretical studies of four allyl imidazolium-based ionic liquids as green inhibitors for copper corrosion in sulfuric acid, Corros. Sci., 2017, 119, 68–78 CrossRef CAS.
- B. Anandkumar, R. George and J. Philip, Efficacy of imidazolium and piperidinium based ionic liquids on inhibiting biofilm formation on titanium and carbon steel surfaces, Anal. Chim. Acta, 2020, 1126, 38–51 CrossRef CAS PubMed.
- N. V. Likhanova, M. A. Domínguez-Aguilar, O. Olivares-Xometl, N. Nava-Entzana, E. Arce and H. Dorantes, The effect of ionic liquids with imidazolium and pyridinium cations on the corrosion inhibition of mild steel in acidic environment, Corros. Sci., 2010, 52, 2088–2097 CrossRef CAS.
- C. Verma, L. O. Olasunkanmi, I. Bahadur, H. Lgaz, M. Quraishi, J. Haque, E.-S. M. Sherif and E. E. Ebenso, Experimental, density functional theory and molecular dynamics supported adsorption behavior of environmental benign imidazolium based ionic liquids on mild steel surface in acidic medium, J. Mol. Liq., 2019, 273, 1–15 CrossRef CAS.
- E. Kowsari, M. Payami, R. Amini, B. Ramezanzadeh and M. Javanbakht, Task-specific ionic liquid as a new green inhibitor of mild steel corrosion, Appl. Surf. Sci., 2014, 289, 478–486 CrossRef CAS.
- S. Ullah, M. A. Bustam, A. M. Shariff, G. Gonfa and K. Izzat, Experimental and quantum study of corrosion of A36 mild steel towards 1-butyl-3-methylimidazolium tetrachloroferrate ionic liquid, Appl. Surf. Sci., 2016, 365, 76–83 CrossRef CAS.
- H. Ashassi-Sorkhabi and M. Es’Haghi, Corrosion inhibition of mild steel in acidic media by [BMIm] Br Ionic liquid, Mater. Chem. Phys., 2009, 114, 267–271 CrossRef CAS.
- I. Lozano, E. Mazario, C. Olivares-Xometl, N. Likhanova and P. Herrasti, Corrosion behaviour of API 5LX52 steel in HCl and H2SO4 media in the presence of 1, 3-dibencilimidazolio acetate and 1, 3-dibencilimidazolio dodecanoate ionic liquids as inhibitors, Mater. Chem. Phys., 2014, 147, 191–197 CrossRef CAS.
- P. Kannan, J. Karthikeyan, P. Murugan, T. S. Rao and N. Rajendran, Corrosion inhibition effect of novel methyl benzimidazolium ionic liquid for carbon steel in HCl medium, J. Mol. Liq., 2016, 221, 368–380 CrossRef CAS.
- A. L. Chong, J. I. Mardel, D. R. MacFarlane, M. Forsyth and A. E. Somers, Synergistic corrosion inhibition of mild steel in aqueous chloride solutions by an imidazolinium carboxylate salt, ACS Sustainable Chem. Eng., 2016, 4, 1746–1755 CrossRef CAS.
- Y. Ma, F. Han, Z. Li and C. Xia, Acidic-functionalized ionic liquid as corrosion inhibitor for 304 stainless steel in aqueous sulfuric acid, ACS Sustainable Chem. Eng., 2016, 4, 5046–5052 CrossRef CAS.
- D. Yang, M. Zhang, J. Zheng and H. Castaneda, Corrosion inhibition of mild steel by an imidazolium ionic liquid compound: the effect of pH and surface pre-corrosion, RSC Adv., 2015, 5, 95160–95170 RSC.
- X. Zheng, S. Zhang, M. Gong and W. Li, Experimental and theoretical study on the corrosion inhibition of mild steel by 1-octyl-3-methylimidazolium L-prolinate in sulfuric acid solution, Ind. Eng. Chem. Res., 2014, 53, 16349–16358 CrossRef CAS.
- D. Guzmán-Lucero, O. Olivares-Xometl, R. Martínez-Palou, N. V. Likhanova, M. A. Domínguez-Aguilar and V. Garibay-Febles, Synthesis of selected vinylimidazolium ionic liquids and their effectiveness as corrosion inhibitors for carbon steel in aqueous sulfuric acid, Ind. Eng. Chem. Res., 2011, 50, 7129–7140 CrossRef.
-
J. Li, D. Li, F. Zhou, D. Feng, Y. Xia and W. Liu, Tribological and corrosive properties of ionic liquids containing triazole functional groups, Industrial Lubrication and Tribology, 2015 Search PubMed.
- E.-S. M. Sherif, H. S. Abdo and S. Z. E. Abedin, Corrosion inhibition of cast iron in arabian gulf seawater by two different ionic liquids, Materials, 2015, 8, 3883–3895 CrossRef CAS PubMed.
- A. Zarrouk, M. Messali, H. Zarrok, R. Salghi, A. Ali, B. Hammouti, S. Al-Deyab and F. Bentiss, Synthesis, characterization and comparative study of new functionalized imidazolium-based ionic liquids derivatives towards corrosion of C38 steel in molar hydrochloric acid, Int. J. Electrochem. Sci., 2012, 7, 6998–7015 CAS.
- M. Messali and M. Asiri, A green ultrasound-assisted access to some new 1-benzyl-3-(4-phenoxybutyl) imidazolium-based ionic liquids derivatives—Potential corrosion inhibitors of mild steel in acidic environment, J. Mater. Environ. Sci., 2013, 5, 770–785 Search PubMed.
- N. Subasree and J. A. Selvi, Imidazolium based ionic liquid derivatives; synthesis and evaluation of inhibitory effect on mild steel corrosion in hydrochloric acid solution, Heliyon, 2020, 6, e03498 CrossRef CAS PubMed.
- K. Qiao and Y. Zeng, Comparative study on two imidazolium-based ionic liquid surfactants as corrosion inhibitors for N80 steel in 15% hydrochloric acid solution, Mater. Corros., 2020, 71, 1913–1926 CrossRef CAS.
- P. D. Pancharatna, S. Lata and G. Singh, Imidazolium based ionic liquid as an efficient and green corrosion constraint for mild steel at acidic pH levels, J. Mol. Liq., 2019, 278, 467–476 CrossRef.
- S. Cao, D. Liu, H. Ding, J. Wang, H. Lu and J. Gui, Task-specific ionic liquids as corrosion inhibitors on carbon steel in 0.5 M HCl solution: an experimental and theoretical study, Corros. Sci., 2019, 153, 301–313 CrossRef CAS.
- M. C. Luna, T. Le Manh, R. C. Sierra, J. M. Flores, L. L. Rojas and E. A. Estrada, Study of corrosion behavior of API 5L X52 steel in sulfuric acid in the presence of ionic liquid 1-ethyl 3-methylimidazolium thiocyanate as corrosion inhibitor, J. Mol. Liq., 2019, 289, 111106 CrossRef.
- M. Corrales-Luna, T. Le Manh, M. Romero-Romo, M. Palomar-Pardavé and E. M. Arce-Estrada, 1-Ethyl 3-methylimidazolium thiocyanate ionic liquid as corrosion inhibitor of API 5L X52 steel in H2SO4 and HCl media, Corros. Sci., 2019, 153, 85–99 CrossRef CAS.
- Y. Guo, B. Xu, Y. Liu, W. Yang, X. Yin, Y. Chen, J. Le and Z. Chen, Corrosion inhibition properties of two imidazolium ionic liquids with hydrophilic tetrafluoroborate and hydrophobic hexafluorophosphate anions in acid medium, J. Ind. Eng. Chem., 2017, 56, 234–247 CrossRef CAS.
- G. Vastag, A. Shaban, M. Vraneš, A. Tot, S. Belić and S. Gadžurić, Influence of the N-3 alkyl chain length on improving inhibition properties of imidazolium-based ionic liquids on copper corrosion, J. Mol. Liq., 2018, 264, 526–533 CrossRef CAS.
- J. Lei, Y. Gao, X. Zhao, Y. Zhu, C. Zhang, Y. Ma, K. Zhao and F. Du, The dilational rheology and splashing behavior of ionic liquid-type imidazolium Gemini surfactant solutions: Impact of alkyl chain length, J. Mol. Liq., 2019, 283, 725–735 CrossRef CAS.
- S. M. Ali, K. M. Emran and M. Messali, Improved protection performance of modified sol-gel coatings with pyridinium-based ionic liquid for cast iron corrosion in 0.5 M HCL solution, Prog. Org. Coat., 2019, 130, 226–234 CrossRef CAS.
- F. Hajjaji, E. Ech-chihbi, N. Rezki, F. Benhiba, M. Taleb, D. S. Chauhan and M. Quraishi, Electrochemical and theoretical insights on the adsorption and corrosion inhibition of novel pyridinium-derived ionic liquids for mild steel in 1 M HCl, J. Mol. Liq., 2020, 113737 CrossRef.
- K. Shalabi, A. Helmy, A. El-Askalany and M. Shahba, New pyridinium bromide mono-cationic surfactant as corrosion inhibitor for carbon steel during chemical cleaning: Experimental and theoretical studies, J. Mol. Liq., 2019, 293, 111480 CrossRef CAS.
- E. E. El-Katori and A. S. Abousalem, Impact of some pyrrolidinium ionic liquids on copper dissolution behavior in acidic environment: experimental, morphological and theoretical insights, RSC Adv., 2019, 9, 20760–20777 RSC.
- O. A. Al-Rashed and A. A. Nazeer, Ionic liquids with superior protection for mild steel in acidic media: effects of anion, cation, and alkyl chain length, J. Mol. Liq., 2019, 288, 111015 CrossRef CAS.
- I. Otero, E. R. López, M. Reichelt and J. Fernández, Tribo-chemical reactions of anion in pyrrolidinium salts for steel–steel contact, Tribol. Int., 2014, 77, 160–170 CrossRef CAS.
- P. Arellanes-Lozada, O. Olivares-Xometl, N. V. Likhanova, I. V. Lijanova, J. R. Vargas-García and R. E. Hernández-Ramírez, Adsorption and performance of ammonium-based ionic liquids as corrosion inhibitors of steel, J. Mol. Liq., 2018, 265, 151–163 CrossRef CAS.
- C. Gabler, C. Tomastik, J. Brenner, L. Pisarova, N. Doerr and G. Allmaier, Corrosion properties of ammonium based ionic liquids evaluated by SEM-EDX, XPS and ICP-OES, Green Chem., 2011, 13, 2869–2877 RSC.
- O. Olivares-Xometl, E. Álvarez-Álvarez, N. V. Likhanova, I. V. Lijanova, R. E. Hernández-Ramírez, P. Arellanes-Lozada and J. L. Varela-Caselis, Synthesis and corrosion inhibition mechanism of ammonium-based ionic liquids on API 5L X60 steel in sulfuric acid solution, J. Adhes. Sci. Technol., 2018, 32, 1092–1113 CrossRef CAS.
- T. Nesane, S. S. Mnyakeni-Moleele and L. C. Murulana, Exploration of synthesized quaternary ammonium ionic liquids as unharmful anti-corrosives for aluminium utilizing hydrochloric acid medium, Heliyon, 2020, 6, e04113 CrossRef PubMed.
- A. Rafat, M. Atilhan and R. Kahraman, Corrosion behavior of carbon steel in CO2 saturated amine and imidazolium-, ammonium-, and phosphonium-based ionic liquid solutions, Ind. Eng. Chem. Res., 2016, 55, 446–454 CrossRef CAS.
- Q. Zhang and Y. Hua, Corrosion inhibition of aluminum in hydrochloric acid solution by alkylimidazolium ionic liquids, Mater. Chem. Phys., 2010, 119, 57–64 CrossRef CAS.
- S. K. Shetty and A. N. Shetty, Ionic liquid as an effective corrosion inhibitor on 6061 Al-15 Vol. Pct. SiC (p) composite in 0.1 M H2SO4 medium–an ecofriendly approach, Can. Chem. Trans., 2015, 3, 41–64 Search PubMed.
- P. Arellanes-Lozada, O. Olivares-Xometl, D. Guzmán-Lucero, N. V. Likhanova, M. A. Domínguez-Aguilar, I. V. Lijanova and E. Arce-Estrada, The inhibition of aluminum corrosion in sulfuric acid by poly (1-vinyl-3-alkyl-imidazolium hexafluorophosphate), Materials, 2014, 7, 5711–5734 CrossRef PubMed.
- Q. Zhang, Z. Gao, F. Xu and X. Zou, Adsorption and corrosion inhibitive properties of gemini surfactants in the series of hexanediyl-1, 6-bis-(diethyl alkyl ammonium bromide) on aluminium in hydrochloric acid solution, Colloids Surf., A, 2011, 380, 191–200 CrossRef CAS.
- F. Trombetta, R. F. de Souza, M. O. de Souza, C. B. Borges, N. F. Panno and E. M. A. Martini, Stability of aluminium in 1-butyl-3-methylimidazolium tetrafluoroborate ionic liquid and ethylene glycol mixtures, Corros. Sci., 2011, 53, 51–58 CrossRef CAS.
- X. Li, S. Deng and H. Fu, Inhibition by tetradecylpyridinium bromide of the corrosion of aluminium in hydrochloric acid solution, Corros. Sci., 2011, 53, 1529–1536 CrossRef CAS.
- M.-D. Bermúdez, A.-E. Jiménez and G. Martínez-Nicolás, Study of surface interactions of ionic liquids with aluminium alloys in corrosion and erosion–corrosion processes, Appl. Surf. Sci., 2007, 253, 7295–7302 CrossRef.
- K. M. Manamela, L. C. Murulana, M. M. Kabanda and E. E. Ebenso, Adsorptive and DFT studies of some imidazolium based ionic liquids as corrosion inhibitors for zinc in acidic medium, Int. J. Electrochem. Sci., 2014, 9, 3029–3046 Search PubMed.
- S. Fashu, C. Gu, X. Wang and J. Tu, Structure, composition and corrosion resistance of Zn-Ni-P alloys electrodeposited from an ionic liquid based on choline chloride, J. Electrochem. Soc., 2014, 161, D3011 CrossRef CAS.
- M. Scendo and J. Uznanska, The effect of ionic liquids on the corrosion inhibition of copper in acidic chloride solutions, Int. J. Corros., 2011, 2011, 1–13 Search PubMed.
- T. Espinosa, J. Sanes, A.-E. Jiménez and M.-D. Bermúdez, Surface interactions, corrosion processes and lubricating performance of protic and aprotic ionic liquids with OFHC copper, Appl. Surf. Sci., 2013, 273, 578–597 CrossRef CAS.
- J. Chen, S. K. Spear, J. G. Huddleston and R. D. Rogers, Polyethylene glycol and solutions of polyethylene glycol as green reaction media, Green Chem., 2005, 7, 64–82 RSC.
-
F. Bailey Jr and J. Koleske, Poly (ethylene oxide), Academic Press, New York, NY, USA, 1976 Search PubMed.
- D. Candy and J. Belsey, Macrogol (polyethylene glycol) laxatives in children with functional constipation and faecal impaction: a systematic review, Arch. Dis. Child., 2009, 94, 156–160 CrossRef CAS PubMed.
- A. A. D'souza and R. Shegokar, Polyethylene glycol (PEG): a versatile polymer for pharmaceutical applications, Expert Opin. Drug Delivery, 2016, 13, 1257–1275 CrossRef.
- M. Roberts, M. Bentley and J. Harris, Chemistry for peptide and protein PEGylation, Adv. Drug Delivery Rev., 2002, 54, 459–476 CrossRef CAS PubMed.
-
G. Totten and N. Clinton, Poly (ethylene glycol) and derivatives as phase transfer catalysts, 1998 Search PubMed.
-
F. M. Kerton and R. Marriott, Alternative solvents for green chemistry, Royal Society of chemistry, 2013 Search PubMed.
-
N. F. Leininger, R. Clontz, J. L. Gainer and D. J. Kirwan, Aqueous polyglycol solutions as alternative solvents, ACS Publications, 2002 Search PubMed.
- D. J. Heldebrant and P. G. Jessop, Liquid poly (ethylene glycol) and supercritical carbon dioxide: a benign biphasic solvent system for use and recycling of homogeneous catalysts, J. Am. Chem. Soc., 2003, 125, 5600–5601 CrossRef CAS PubMed.
- K. Kocheva, T. Kartseva, S. Landjeva and G. Georgiev, Parameters of cell membrane stability and levels of oxidative stress in leaves of wheat seedlings treated with PEG 6000, Gen. Appl. Plant Physiol., 2009, 35, 127–133 CAS.
- Y. Li, H. Zhang, Y. Yao, T. Gong, R. Dong, D. Li, Y. Liu and B. Lei, Promoted off-on recognition of H2O2 based on the fluorescence of silicon quantum dots assembled two-dimensional PEG-MnO2 nanosheets hybrid nanoprobe, Microchim. Acta, 2020, 187, 347 CrossRef CAS PubMed.
-
A. R. Kiasat, M. Zayadi, T. F. Mohammad and M. M. Fallah, Simple, Practical and Eco-friendly Reduction of Nitroarenes with Zinc in the Presence of Polyethylene Glycol Immobilized on Silica Gel as a New Solid–liquid Phase Transfer Catalyst in Water, 2011 Search PubMed.
- E. Rytting, K. A. Lentz, X.-Q. Chen, F. Qian and S. Venkatesh, A quantitative structure-property relationship for predicting drug solubility in PEG 400/water cosolvent systems, Pharm. Res., 2004, 21, 237–244 CrossRef CAS PubMed.
- H. Yang, J. J. Morris and S. T. Lopina, Polyethylene glycol–polyamidoamine dendritic micelle as solubility enhancer and the effect of the length of polyethylene glycol arms on the solubility of pyrene in water, J. Colloid Interface Sci., 2004, 273, 148–154 CrossRef CAS PubMed.
- H. Ashassi-Sorkhabi and N. Ghalebsaz-Jeddi, Inhibition effect of polyethylene glycol on the corrosion of carbon steel in sulphuric acid, Mater. Chem. Phys., 2005, 92, 480–486 CrossRef CAS.
- H. Ashassi-Sorkhabi and N. Ghalebsaz-Jeddi, Effect of ultrasonically induced cavitation on inhibition behavior of polyethylene glycol on carbon steel corrosion, Ultrason. Sonochem., 2006, 13, 180–188 CrossRef CAS PubMed.
- S. Umoren, O. Ogbobe, P. Okafor and E. Ebenso, Polyethylene glycol and polyvinyl alcohol as corrosion inhibitors for aluminium in acidic medium, J. Appl. Polym. Sci., 2007, 105, 3363–3370 CrossRef CAS.
- S. Umoren, O. Ogbobe, I. Igwe and E. Ebenso, Inhibition of mild steel corrosion in acidic medium using synthetic and naturally occurring polymers and synergistic halide additives, Corros. Sci., 2008, 50, 1998–2006 CrossRef CAS.
- S. A. Umoren, Synergistic inhibition effect of polyethylene glycol–polyvinyl pyrrolidone blends for mild steel corrosion in sulphuric acid medium, J. Appl. Polym. Sci., 2011, 119, 2072–2084 CrossRef CAS.
- S. John, M. Kuruvilla and A. Joseph, Surface morphological and impedance spectroscopic studies on the interaction of polyethylene glycol (PEG) and polyvinyl pyrrolidone (PVP) with mild steel in acid solutions, Res. Chem. Intermed., 2013, 39, 1169–1182 CrossRef CAS.
- S. Umoren, O. Ogbobe and E. Ebenso, The adsorption characteristics and synergistic inhibition between polyethylene glycol and halide ions for the corrosion of mild steel in acidic medium, Bull. Electrochem., 2006, 22, 155–168 CAS.
- M. Mobin and M. A. Khan, Adsorption and corrosion inhibition behavior of polyethylene glycol and surfactants additives on mild steel in H2SO4, J. Mater. Eng. Perform., 2014, 23, 222–229 CrossRef CAS.
- A. Algaber, E. M. El-Nemma and M. M. Saleh, Effect of octylphenol polyethylene oxide on the corrosion inhibition of steel in 0.5 M H2SO4, Mater. Chem. Phys., 2004, 86, 26–32 CrossRef CAS.
- S. Deng, X. Li and J. Sun, Adsorption and inhibition effect of polyethylene glycol 20
000 on steel in H2SO4 solution, Clean. World, 2011, 4 Search PubMed , https://en.cnki.com.cn/Article_en/CJFDTotal-HXQX201104004.htm.
- H. Ashassi-Sorkhabi, N. Ghalebsaz-Jeddi, F. Hashemzadeh and H. Jahani, Corrosion inhibition of carbon steel in hydrochloric acid by some polyethylene glycols, Electrochim. Acta, 2006, 51, 3848–3854 CrossRef CAS.
- M. M. Fares, A. Maayta and J. A. Al-Mustafa, Synergistic corrosion inhibition of aluminum by polyethylene glycol and ciprofloxacin in acidic media, J. Adhes. Sci. Technol., 2013, 27, 2495–2506 CrossRef CAS.
- M. Awad, M. Metwally, S. Soliman and A. El-Zomrawy, Experimental and quantum chemical studies of the effect of poly ethylene glycol as corrosion inhibitors of aluminum surface, J. Ind. Eng. Chem., 2014, 20, 796–808 CrossRef CAS.
- H. Boudellioua, Y. Hamlaoui, L. Tifouti and F. Pedraza, Comparison between the inhibition efficiencies of two modification processes with PEG–ceria based layers against corrosion of mild steel in chloride and sulfate media, J. Mater. Eng. Perform., 2017, 26, 4402–4414 CrossRef CAS.
- H. Boudellioua, Y. Hamlaoui, L. Tifouti and F. Pedraza, Effects of polyethylene glycol (PEG) on the corrosion inhibition of mild steel by cerium nitrate in chloride solution, Appl. Surf. Sci., 2019, 473, 449–460 CrossRef CAS.
- S. Božović, S. Martinez and V. Grudić, A novel environmentally friendly synergistic mixture for steel corrosion inhibition in 0.51 M NaCl, Acta Chim. Slov., 2019, 66, 112 CrossRef.
- S. A. El Wanees, A. A. El Aal and E. A. El Aal, Effect of polyethylene glycol on pitting corrosion of cadmium in alkaline solution, Br. Corros. J., 1993, 28, 222–226 CrossRef.
- Y. Ein-Eli, M. Auinat and D. Starosvetsky, Electrochemical and surface studies of zinc in alkaline solutions containing organic corrosion inhibitors, J. Power Sources, 2003, 114, 330–337 CrossRef CAS.
- D. Gelman, I. Lasman, S. Elfimchev, D. Starosvetsky and Y. Ein-Eli, Aluminum corrosion mitigation in alkaline electrolytes containing hybrid inorganic/organic inhibitor system for power sources applications, J. Power Sources, 2015, 285, 100–108 CrossRef CAS.
- M. Quraishi and D. Jamal, Development and testing of all organic volatile corrosion inhibitors, Corrosion, 2002, 58, 387–391 CrossRef CAS.
- R. C. Cioc, E. Ruijter and R. V. Orru, Multicomponent reactions: advanced tools for sustainable organic synthesis, Green Chem., 2014, 16, 2958–2975 RSC.
- C. G. Lima, N. M. Moreira, M. W. Paixao and A. G. Corrêa, Heterogenous green catalysis: Application of zeolites on multicomponent reactions, Curr. Opin. Green Sustainable Chem., 2019, 15, 7–12 CrossRef.
- Y. Gu, Multicomponent reactions in unconventional solvents: state of the art, Green Chem., 2012, 14, 2091–2128 RSC.
-
J. B. Bariwal, J. C. Trivedi and E. V. Van der Eycken, Microwave irradiation and multicomponent reactions, Synthesis of Heterocycles via Multicomponent Reactions II, Springer, 2010, pp. 169–230 Search PubMed.
- R. S. Varma, “Greener” chemical syntheses using mechanochemical mixing or microwave and ultrasound irradiation, Green Chem. Lett. Rev., 2007, 1, 37–45 CrossRef CAS.
- W. Liu, K. Zhu, S. J. Teat, B. J. Deibert, W. Yuan and J. Li, A mechanochemical route toward the rational, systematic, and cost-effective green synthesis of strongly luminescent copper iodide based hybrid phosphors, J. Mater. Chem. C, 2017, 5, 5962–5969 RSC.
- M. Kidwai, R. Mohan and S. Saxena, Solid-supported Hantzsch—Biginelli reaction for syntheses of pyrimidine derivatives, Russ. Chem. Bull., 2003, 52, 2457–2460 CrossRef CAS.
- R. S. Varma, Solvent-free organic syntheses. using supported reagents and microwave irradiation, Green Chem., 1999, 1, 43–55 RSC.
- J. Haque, K. Ansari, V. Srivastava, M. Quraishi and I. Obot, Pyrimidine derivatives as novel acidizing corrosion inhibitors for N80 steel useful for petroleum industry: a combined experimental and theoretical approach, J. Ind. Eng. Chem., 2017, 49, 176–188 CrossRef CAS.
- M. Yadav, L. Gope, N. Kumari and P. Yadav, Corrosion inhibition performance of pyranopyrazole derivatives for mild steel in HCl solution: Gravimetric, electrochemical and DFT studies, J. Mol. Liq., 2016, 216, 78–86 CrossRef CAS.
- C. Verma, M. Quraishi and A. Singh, 5-Substituted 1H-tetrazoles as effective corrosion inhibitors for mild steel in 1 M hydrochloric acid, J. Taibah Univ. Sci., 2016, 10, 718–733 CrossRef.
- C. Verma, M. Quraishi, E. Ebenso, I. Obot and A. El Assyry, 3-Amino alkylated indoles as corrosion inhibitors for mild steel in 1M HCl: Experimental and theoretical studies, J. Mol. Liq., 2016, 219, 647–660 CrossRef CAS.
- P. Singh, V. Srivastava and M. Quraishi, Novel quinoline derivatives as green corrosion inhibitors for mild steel in acidic medium: electrochemical, SEM, AFM, and XPS studies, J. Mol. Liq., 2016, 216, 164–173 CrossRef CAS.
- M. Jeeva, M. Susai Boobalan and G. V. Prabhu, Adsorption and anticorrosion behavior of 1-((pyridin-2-ylamino)(pyridin-4-yl) methyl) pyrrolidine-2, 5-dione on mild steel surface in hydrochloric acid solution, Res. Chem. Intermed., 2018, 44, 425–454 CrossRef CAS.
- C. Verma, M. A. Quraishi, K. Kluza, M. Makowska-Janusik, L. O. Olasunkanmi and E. E. Ebenso, Corrosion inhibition of mild steel in 1M HCl by D-glucose derivatives of dihydropyrido [2, 3-d: 6, 5-d′] dipyrimidine-2, 4, 6, 8 (1H, 3H, 5H, 7H)-tetraone, Sci. Rep., 2017, 7, 44432 CrossRef CAS PubMed.
- C. Verma, L. Olasunkanmi, I. Obot, E. E. Ebenso and M. Quraishi, 5-Arylpyrimido-[4, 5-b] quinoline-diones as new and sustainable corrosion inhibitors for mild steel in 1 M HCl: a combined experimental and theoretical approach, RSC Adv., 2016, 6, 15639–15654 RSC.
- N. K. Gupta, C. Verma, R. Salghi, H. Lgaz, A. Mukherjee and M. Quraishi, New phosphonate based corrosion inhibitors for mild steel in hydrochloric acid useful for industrial pickling processes: experimental and theoretical approach, New J. Chem., 2017, 41, 13114–13129 RSC.
- M. A. Quraishi, 2-Amino-3, 5-dicarbonitrile-6-thio-pyridines: new and effective corrosion inhibitors for mild steel in 1 M HCl, Ind. Eng. Chem. Res., 2014, 53, 2851–2859 CrossRef.
- M. Bahrami, S. Hosseini and P. Pilvar, Experimental and theoretical investigation of organic compounds as inhibitors for mild steel corrosion in sulfuric acid medium, Corros. Sci., 2010, 52, 2793–2803 CrossRef CAS.
- C. Verma, M. Quraishi and A. Singh, 2-Amino-5-nitro-4, 6-diarylcyclohex-1-ene-1, 3, 3-tricarbonitriles as new and effective corrosion inhibitors for mild steel in 1 M HCl: Experimental and theoretical studies, J. Mol. Liq., 2015, 212, 804–812 CrossRef CAS.
- A. K. Singh and M. Quraishi, Inhibiting effects of 5-substituted isatin-based Mannich bases on the corrosion of mild steel in hydrochloric acid solution, J. Appl. Electrochem., 2010, 40, 1293–1306 CrossRef CAS.
- M. Jeeva, G. V. Prabhu, M. S. Boobalan and C. M. Rajesh, Interactions and inhibition effect of urea-derived Mannich bases on a mild steel surface in HCl, J. Phys. Chem. C, 2015, 119, 22025–22043 CrossRef CAS.
- K. Ansari and M. Quraishi, Experimental and computational studies of naphthyridine derivatives as corrosion inhibitor for N80 steel in 15% hydrochloric acid, Phys. E, 2015, 69, 322–331 CrossRef CAS.
- P. Singh, M. Makowska-Janusik, P. Slovensky and M. Quraishi, Nicotinonitriles as green corrosion inhibitors for mild steel in hydrochloric acid: Electrochemical, computational and surface morphological studies, J. Mol. Liq., 2016, 220, 71–81 CrossRef CAS.
- K. Ansari, M. Quraishi and A. Singh, Pyridine derivatives as corrosion inhibitors for N80 steel in 15% HCl: Electrochemical, surface and quantum chemical studies, Measurement, 2015, 76, 136–147 CrossRef.
- K. Ansari, M. Quraishi and A. Singh, Corrosion inhibition of mild steel in hydrochloric acid by some pyridine derivatives: an experimental and quantum chemical study, J. Ind. Eng. Chem., 2015, 25, 89–98 CrossRef CAS.
- V. Srivastava, J. Haque, C. Verma, P. Singh, H. Lgaz, R. Salghi and M. Quraishi, Amino acid based imidazolium zwitterions as novel and green corrosion inhibitors for mild steel: Experimental, DFT and MD studies, J. Mol. Liq., 2017, 244, 340–352 CrossRef CAS.
- P. Singh, M. Quraishi, S. Gupta and A. Dandia, Investigation of the corrosion inhibition effect of 3-methyl-6-oxo-4-(thiophen-2-yl)-4, 5, 6, 7-tetrahydro-2H-pyrazolo [3, 4-b] pyridine-5-carbonitrile (TPP) on mild steel in hydrochloric acid, J. Taibah Univ. Sci., 2016, 10, 139–147 CrossRef.
- C. Verma and M. Quraishi, Adsorption behavior of 8, 9-bis (4 (dimethyl amino) phenyl) benzo [4, 5] imidazo [1, 2-a] pyridine-6, 7-dicarbonitrile on mild steel surface in 1 M HCl, J. Assoc. Arab Univ. Basic Appl. Sci., 2017, 22, 55–61 Search PubMed.
- P. Singh, A. Singh and M. Quraishi, Thiopyrimidine derivatives as new and effective corrosion inhibitors for mild steel in hydrochloric acid: Electrochemical and quantum chemical studies, J. Taiwan Inst. Chem. Eng., 2016, 60, 588–601 CrossRef CAS.
- C. Verma and M. Quraishi, 2-Amino-4-(2, 4-dihydroxyphenyl) quinoline-3-carbonitrile as sustainable corrosion inhibitor for SAE 1006 steel in 1 M HCl: Electrochemical and surface investigation, J. Assoc. Arab Univ. Basic Appl. Sci., 2017, 23, 29–36 Search PubMed.
- D. K. Yadav, B. Maiti and M. Quraishi, Electrochemical and quantum chemical studies of 3, 4-dihydropyrimidin-2 (1H)-ones as corrosion inhibitors for mild steel in hydrochloric acid solution, Corros. Sci., 2010, 52, 3586–3598 CrossRef CAS.
-
C. B. Verma, E. E. Ebenso and M. Quraishi, Electrochemical investigation of substituted aniline, melamine and formaldehydebBased terpolymers as effective corrosion inhibitors for mild steel in 1M hydrochloric acid solution, 2014 Search PubMed.
- S. Gupta, A. Dandia, P. Singh and M. Qureishi, Green synthesis of pyrazolo [3, 4-b] pyridine derivatives by ultrasonic technique and their application as corrosion inhibitor for mild steel in acid medium, J. Mater. Einviron. Sci., 2015, 6, 168–177 CAS.
- C. Verma, M. Quraishi and E. Ebenso, Green ultrasound assisted synthesis of N 2, N 4, N 6-tris ((pyridin-2-ylamino) methyl)-1, 3, 5-triazine-2, 4, 6-triamine as effective corrosion inhibitor for mild steel in 1 M hydrochloric acid medium, Int. J. Electrochem. Sci., 2013, 8, 10864–10877 CAS.
- C. Verma, M. Quraishi and E. Ebenso, Mannich bases derived from melamine, formaldehyde alkanoleamines as novel corrosion inhibitors for mild steel in hydrochloric acid medium, Int. J. Electrochem. Sci., 2013, 8, 10851–10863 CAS.
- A. Dandia, S. Gupta, P. Singh and M. Quraishi, Ultrasound-assisted synthesis of pyrazolo [3, 4-b] pyridines as potential corrosion inhibitors for mild steel in 1.0 M HCl, ACS Sustainable Chem. Eng., 2013, 1, 1303–1310 CrossRef CAS.
- M. Yadav, D. Sharma, S. Kumar, I. Bahadur and E. Ebenso, Electrochemical and theoretical studies on amino phosphonates as efficient corrosion inhibitor for N80 steel in hydrochloric acid solution, Int. J. Electrochem. Sci., 2014, 9, 6580–6593 Search PubMed.
- K. Ansari, Sudheer, A. Singh and M. Quraishi, Some pyrimidine derivatives as corrosion inhibitor for mild steel in hydrochloric
acid, J. Dispersion Sci. Technol., 2015, 36, 908–917 CrossRef CAS.
- M. Quraishi, The corrosion inhibition effect of aryl pyrazolo pyridines on copper in hydrochloric acid system: computational and electrochemical studies, RSC Adv., 2015, 5, 41923–41933 RSC.
- C. B. Verma, M. Quraishi and E. Ebenso, Electrochemical and Thermodynamic Investigation of Some Soluble Terpolymers as effective corrosion inhibitors for Mild Steel in 1M hydrochloric acid solution, Int. J. Electrochem. Sci., 2013, 8, 12894–12906 CAS.
- K. Ansari, M. Quraishi, A. Singh, S. Ramkumar and I. B. Obote, Corrosion inhibition of N80 steel in 15% HCl by pyrazolone derivatives: electrochemical, surface and quantum chemical studies, RSC Adv., 2016, 6, 24130–24141 RSC.
- K. Ansari, M. Quraishi and A. Singh, Chromenopyridin derivatives as environmentally benign corrosion inhibitors for N80 steel in 15% HCl, J. Assoc. Arab Univ. Basic Appl. Sci., 2017, 22, 45–54 Search PubMed.
- R. Korde, C. B. Verma, E. Ebenso and M. Quraishi, Electrochemical and Thermo Dynamical Investigation of 5-ethyl 4-(4-methoxyphenyl)-6-methyl-2-thioxo-1, 2, 3, 4 tetrahydropyrimidine-5-carboxylate on Corrosion Inhibition Behavior of Aluminium in 1M Hydrochloric Acid Medium, Int. J. Electrochem. Sci., 2015, 10, 1081–1093 Search PubMed.
- C. O. Kappe, Controlled microwave heating in modern organic synthesis, Angew. Chem., Int. Ed., 2004, 43, 6250–6284 CrossRef CAS PubMed.
- W. Ao, J. Fu, X. Mao, Q. Kang, C. Ran, Y. Liu, H. Zhang, Z. Gao, J. Li and G. Liu, Microwave assisted preparation of activated carbon from biomass: A review, Renewable Sustainable Energy Rev., 2018, 92, 958–979 CrossRef CAS.
- B. B. Goldberg, J.-B. Liu and F. Forsberg, Ultrasound contrast agents: a review, Ultrasound Med. Biol., 1994, 20, 319–333 CrossRef CAS.
- S. Nain, R. Singh and S. Ravichandran, Importance of Microwave Heating in Organic Synthesis, Adv. J. Chem., Sect. A, 2019, 2, 94–104 CrossRef CAS.
-
M. E. Brown, Introduction to thermal analysis: techniques and applications, Springer Science & Business Media, 2001 Search PubMed.
- C. Marcos and I. Rodríguez, Expansibility of vermiculites irradiated with microwaves, Appl. Clay Sci., 2011, 51, 33–37 CrossRef CAS.
- V. K. Tyagi and S.-L. Lo, Microwave irradiation: A sustainable way for sludge treatment and resource recovery, Renewable Sustainable Energy Rev., 2013, 18, 288–305 CrossRef CAS.
- D. N. K. Reddy, K. B. Chandrasekhar, Y. S. S. Ganesh, G. R. Reddy, J. P. Kumar, R. K. Kapavarapu and M. Pal, FeF 3-catalyzed MCR in PEG-400: ultrasound assisted synthesis of N-substituted 2-aminopyridines, RSC Adv., 2016, 6, 67212–67217 RSC.
- M. Dell’Acqua, G. Abbiati and E. Rossi, Palladium-catalyzed, microwave-enhanced three-component synthesis of isoquinolines with aqueous ammonia, Synlett, 2010, 2672–2676 Search PubMed.
- N. A. Khan and S. H. Jhung, Synthesis of metal-organic frameworks (MOFs) with microwave or ultrasound: Rapid reaction, phase-selectivity, and size reduction, Coord. Chem. Rev., 2015, 285, 11–23 CrossRef CAS.
- A. Lew, P. O. Krutzik, M. E. Hart and A. R. Chamberlin, Increasing rates of reaction: microwave-assisted organic synthesis for combinatorial chemistry, J. Comb. Chem., 2002, 4, 95–105 CrossRef CAS PubMed.
- M. Palomar-Pardavé, M. Romero-Romo, H. Herrera-Hernández, M. Abreu-Quijano, N. V. Likhanova, J. Uruchurtu and J. Juárez-García, Influence of the alkyl chain length of 2 amino 5 alkyl 1, 3, 4 thiadiazole compounds on the corrosion inhibition of steel immersed in sulfuric acid solutions, Corros. Sci., 2012, 54, 231–243 CrossRef.
- Q. Deng, H.-W. Shi, N.-N. Ding, B.-Q. Chen, X.-P. He, G. Liu, Y. Tang, Y.-T. Long and G.-R. Chen, Novel triazolyl bis-amino acid derivatives readily synthesized via click chemistry as potential corrosion inhibitors for mild steel in HCl, Corros. Sci., 2012, 57, 220–227 CrossRef CAS.
- A. Dutta, S. K. Saha, P. Banerjee and D. Sukul, Correlating electronic structure with corrosion inhibition potentiality of some bis-benzimidazole derivatives for mild steel in hydrochloric acid: combined experimental and theoretical studies, Corros. Sci., 2015, 98, 541–550 CrossRef CAS.
- H. I. Beltrán, R. Esquivel, A. Sosa-Sánchez, J. L. Sosa-Sánchez, H. Höpfl, V. Barba, N. Farfán, M. Galicia García, O. Olivares-Xometl and L. S. Zamudio-Rivera, Microwave assisted stereoselective synthesis of cis-substituted tinIV phthalocyanine dicarboxylates. Application as corrosion inhibitors, Inorg. Chem., 2004, 43, 3555–3557 CrossRef.
- J. Halambek, M. Jukić, K. Berković and J. Vorkapić-Furač, Investigation of novel heterocyclic compounds as inhibitors of Al-3Mg alloy corrosion in hydrochloric acid solutions, Int. J. Electrochem. Sci., 2012, 7, 1580–1601 CAS.
- P. Singh, M. Quraishi, E. Ebenso and C. B. Verma, Ultrasound assisted synthesis of chalcones as green corrosion inhibitors for mild steel in 1M hydrochloric solution, Int. J. Electrochem. Sci., 2014, 9, 7446–7459 CAS.
- A. Ehsani, R. Moshrefi and M. Ahmadi, Electrochemical investigation of inhibitory of new synthesized 3-(4-iodophenyl)-2-imino-2, 3-dihydrobenzo [d] oxazol-5-yl 4-methylbenzenesulfonate on corrosion of stainless steel in acidic medium, J. Electrochem. Sci. Technol., 2015, 6, 7–15 CrossRef.
-
S. Mallakpour and M. Dinari, Ionic liquids as green solvents: progress and prospects, Green Solvents II, Springer, 2012, pp. 1–32 Search PubMed.
- T. W. Bentley and G. E. Carter, The SN2-SN1 spectrum. 4. The SN2 (intermediate) mechanism for solvolyses of tert-butyl chloride: a revised Y scale of solvent ionizing power based on solvolyses of 1-adamantyl chloride, J. Am. Chem. Soc., 1982, 104, 5741–5747 CrossRef CAS.
-
N. M. Aljamali, Review: General Principles in Chemistry, 2018, ch. 5, pp. 60–91, ISBN: 978-93-87905-41-2 Search PubMed.
- P. G. Jessop, Searching for green solvents, Green Chem., 2011, 13, 1391–1398 RSC.
- J. M. DeSimone, Practical approaches to green solvents, Science, 2002, 297, 799–803 CrossRef CAS PubMed.
- M. Reslow, P. Adlercreutz and B. Mattiasson, On the importance of the support material for bioorganic synthesis: Influence of water partition between solvent, enzyme and solid support in water-poor reaction media, Eur. J. Biochem., 1988, 172, 573–578 CrossRef CAS PubMed.
- H. Zhao, S. Xia and P. Ma, Use of ionic liquids as ‘ green'solvents for extractions, J. Chem. Technol. Biotechnol.: Int. Res. Process, Environ. Clean Technol., 2005, 80, 1089–1096 CrossRef CAS.
- S. M. Tawfik, Ionic liquids based gemini cationic surfactants as corrosion inhibitors for carbon steel in hydrochloric acid solution, J. Mol. Liq., 2016, 216, 624–635 CrossRef CAS.
- A. Simoes, D. Tallman and G. Bierwagen, Use of ionic liquids for the electrochemical characterization of water transport in organic coatings, Electrochem. Solid-State Lett., 2005, 8, B60 CrossRef CAS.
- Z. Li, X. Wang, Y. Zhang and C. Jing, Enhancing the Corrosion Resistance of Epoxy Coatings by Impregnation with a Reduced Graphene Oxide-Hydrophobic Ionic Liquid Composite, ChemElectroChem, 2018, 5, 3300–3306 CrossRef CAS.
- S. P. Nalawade, F. Picchioni and L. Janssen, Supercritical carbon dioxide as a green solvent for processing polymer melts: Processing aspects and applications, Prog. Polym. Sci., 2006, 31, 19–43 CrossRef CAS.
- Á. L. Santana, D. T. Santos and M. A. A. Meireles, Perspectives on small-scale integrated biorefineries using supercritical CO2 as a green solvent, Curr. Opin. Green Sustainable Chem., 2019, 18, 1–12 CrossRef.
- W. Wu, W. Li, B. Han, T. Jiang, D. Shen, Z. Zhang, D. Sun and B. Wang, Effect of organic cosolvents on the solubility of ionic liquids in supercritical CO2, J. Chem. Eng. Data, 2004, 49, 1597–1601 CrossRef CAS.
- P. G. Jessop, R. R. Stanley, R. A. Brown, C. A. Eckert, C. L. Liotta, T. T. Ngo and P. Pollet, Neoteric solvents for asymmetric hydrogenation: supercritical fluids, ionic liquids, and expanded ionic liquids, Green Chem., 2003, 5, 123–128 RSC.
|
This journal is © The Royal Society of Chemistry 2021 |
Click here to see how this site uses Cookies. View our privacy policy here.