DOI:
10.1039/D0GC03723K
(Communication)
Green Chem., 2021,
23, 274-279
Transition-metal-free synthesis of thiazolidin-2-ones and 1,3-thiazinan-2-ones from arylamines, elemental sulfur and CO2†
Received
3rd November 2020
, Accepted 3rd December 2020
First published on 8th December 2020
Abstract
Transfering waste to treasure is highly important in green chemistry. However, it is difficult to realize it efficiently due to the low reactivity, especially the simultaneous utilization of two unreactive feedstocks in one reaction. Herein, we report the first utilization of both elemental sulfur and CO2 in a multi-component reaction to generate valuable thiazolidin-2-ones and 1,3-thiazinan-2-ones. Under transition-metal-free reaction conditions, a variety of easily available arylamines react with elemental sulfur and CO2 (1 atm) to give functional thiazolidin-2-ones and 1,3-thiazinan-2-ones in moderate to good yields via C–H bond functionalization. This strategy is highlighted by high step economy with generation of three bonds in one reaction and good functional groups tolerance.
Tremendous efforts have been devoted to the development of green and sustainable chemistry, given the significance of its environmental impact and sustainability both in academia and industry. Among diverse investigation, the formation of valuable chemicals using CO2 as the C1 source is an attractive strategy due to the abundance, availability, sustainability, and nontoxicity of CO2.1 Although the thermodynamic stability and kinetic inertness of CO2 set challenges for its utilization in organic synthesis, many kinds of CO2 transformations have been developed to construct important carbonyl-containing heterocycles.2 Recently, carbonylation of C–H bonds with CO2 has emerged as an efficient and highly promising strategy to generate a variety of valuable carbonyl-containing heterocycles base on the concept of “CO2 = CO + [O]”.3,4 It is still highly desirable to develop other sustainable strategies for the construction of carbonyl-containing heterocycles via C–H functionalization.
Thiazolidin-2-ones are important structural motifs in a large number of pharmaceuticals, agrochemicals, and natural products (Scheme 1A).5 Therefore, the development of efficient protocols for selective synthesis of thiazolidin-2-one derivatives is of much interest and importance. Recently, many groups have made great contributions to this field by developing various strategies to generate thiazolidin-2-ones with a series of carbonyl sources (e.g., CO2, CO, COS, or DMF).6 In most cases, however, the substrates are limited to bifunctional arenes bearing two functional groups (e.g., halogen, sulfur-containing groups) (Scheme 1B).7 To the best of our knowledge, the mono-functionalized substrates, such as arylamines, have not been used to construct thiazolidin-2-ones with CO2 and one sulfur source. Considering that elemental sulfur (S8), which is non-toxic, stable, inexpensive and easy to handle, is well known as industrial waste8 and has been applied in C–S bond formation,9 we hypothesized whether we could construct thiazolidin-2-one derivatives by using three simple and easily available substrates, including aryl amines, S8 and CO2. However, there are several challenges. First of all, the formation of C–S bond via C–H bond sulfuration is inefficient due to the low activity of C–H bonds and S8. Moreover, the inert property of CO2 might also hamper the carbonylation. Thus, the low reactivities of such three components might call for harsh reaction conditions, which would cause problems to control the chemo- and regio-selectivities. For example, di- or multi-sulfuration instead of mono-sulfuration might happen. The generation of other carbonylative products, such as ureas and carbamates, is also possible. Therefore, it is highly challenging to develop an efficient and selective three-component reaction to directly synthesize thiazolidin-2-ones by using two unreactive feedstocks. With our continuous interest in the utilization of CO2 to generate carbonyl-containing heterocycles, herein we report a practical three-component reaction to generate valuable thiazolidin-2-ones from arylamines, S8, and CO2 (1 atm) under transition-metal-free and redox-neutral conditions (Scheme 1C).
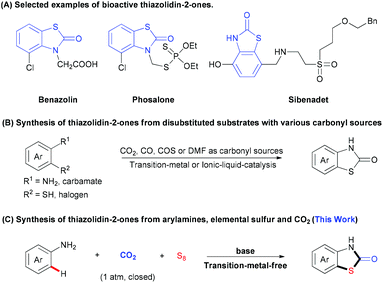 |
| Scheme 1 Importance and synthesis of thiazolidin-2-ones. | |
We began to investigate the reaction using naphthalen-2-amine 1a as the standard substrate with S8 as the sulfur source under one atmosphere of CO2 (Table 1). To our delight, the desired product 2a was obtained in 78% yield with NaOtBu as base and 1,4-dioxane as solvent at 140 °C for 24 h (Table 1, entry 3). This reaction is highly regioselective, as the sulfuration at the C3-position was not detected. A variety of bases, such as LiOtBu, KOtBu, and Cs2CO3, were tested to give lower yields than NaOtBu (Table 1, entries 1–4). The screening of the amount of NaOtBu demonstrated that 6.0 equivalent was the best choice (Table 1, entries 5–7). Moreover, lowering reaction temperature also provided worse results, which might arise from the unfavorable carbonylation and sulfuration with unreactive CO2 and S8 (Table 1, entries 8 and 9). Furthermore, we also examined the amount of S8 and found that 4.0 equivalent of S8 was the best choice (Table 1, entries 10 and 11). Other solvents, such as diglyme, DMF, DMA, and DMSO, were also tested and gave poor results (Table 1, entries 12–15), indicating a unique role of 1,4-dioxane in this reaction. Further change in concentration did not improve the reaction (Table 1, entry 16). Control experiments showed that both CO2 and S8 were vital to this transformation (Table 1, entries 17 and 18).
| 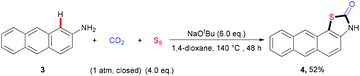 | (1) |
Table 1 Optimization of reaction conditionsa
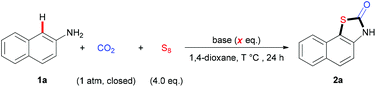
|
Entry |
Base |
X
|
T/°C |
Yieldb (%) |
Entry |
Base |
X
|
T/°C |
Yieldb (%) |
Reaction conditions: 1a (0.2 mmol), S8 (32 g mol−1, 0.8 mmol, 25.6 mg), CO2 (1 atm, closed), 1,4-dioxane (1 mL), 24 h.
Isolated yields.
S8 (32 g mol−1, 0.4 mmol, 12.8 mg).
S8 (32 g mol−1, 0.6 mmol, 19.2 mg).
diglyme (1.0 mL).
N,N-Dimethylformamide (DMF, 1.0 mL).
N,N-Dimethylacetamide (DMA, 1.0 mL).
Dimethyl sulfoxide (DMSO, 1.0 mL).
1,4-Dioxane (2.0 mL).
Without S8.
Under the atmospheric of N2 instead of CO2. n.d. = not detected.
|
1 |
LiOtBu |
5 |
140 |
44 |
10c |
NaOtBu |
6 |
140 |
35 |
2 |
KOtBu |
5 |
140 |
62 |
11d |
NaOtBu |
6 |
140 |
74 |
3 |
NaOtBu |
5 |
140 |
78 |
12e |
NaOtBu |
6 |
140 |
22 |
4 |
Cs2CO3 |
5 |
140 |
8 |
13f |
NaOtBu |
6 |
140 |
35 |
5 |
NaOtBu |
3 |
140 |
24 |
14g |
NaOtBu |
6 |
140 |
12 |
6 |
NaOtBu |
4 |
140 |
40 |
15h |
NaOtBu |
6 |
140 |
36 |
7
|
NaO
t
Bu
|
6
|
140
|
87
|
16i |
NaOtBu |
6 |
140 |
68 |
8 |
NaOtBu |
6 |
120 |
n.d. |
17j |
NaOtBu |
6 |
140 |
n.d. |
9 |
NaOtBu |
6 |
130 |
34 |
18k |
NaOtBu |
6 |
140 |
n.d. |
With the optimal reaction conditions in hand, we explored the substrate scope and generality of the three-component reaction (Table 2). We found that diverse naphthalen-2-amines 1a–k underwent this transformation smoothly to afford the expected products in 56%–87% yields. Notably, a gram-scale reaction of 1a (8 mmol), CO2, and S8 was successfully performed to give 2a in 76% yield. Different substituents, such as electron-neutral groups, electron-donating groups (EDGs) and electron-withdrawing groups (EWGs), did not affect the reaction. Various functional groups, such as methoxyl, trifluoromethyl, cyano groups, C–Br and C–F bonds, were tolerated well. Importantly, the quinolin-6-amine 1l also showed good reactivity in this transformation. Moreover, a related fused aryl amine, anthracen-2-amine 3, could also take part in this transformation to generate the desired product 4 in moderate yield (eqn (1)).
Table 2 Substrate scope of naphthalen-2-amines and quinolin-6-aminea
Reaction conditions: 1 (0.2 mmol), S8 (32 g mol−1, 0.8 mmol, 25.6 mg), NaOtBu (6.0 eq.), CO2 (1 atm, closed), 1,4-dioxane (1 mL), 24 h, 140 °C. Isolated yields.
Gram scale.
48 h.
72 h.
|
|
In addition to naphthalen-2-amines, the reactions of naphthalen-1-amines 5 could also proceed well, furnishing the target products with moderate to good yields (Table 3). Interestingly, the sulfuration of 5 happened selectively at the C8-position to construct 1,3-thiazinan-2-ones with the 6-member ring. A variety of naphthalen-1-amines 5 bearing different substituents, such as methoxyl, thiophene, thiol, and trifluoromethyl group, could undergo this transformation to provide the desired products in good yields. Similarly, the quinolin-5-amine 5h also behaved well in this transformation.
Table 3 Substrate scope of naphthalen-1-amines and quinolin-5-aminea
Reaction conditions: 5 (0.2 mmol), S8 (32 g mol−1, 0.8 mmol, 25.6 mg), NaOtBu (6.0 eq.), CO2 (1 atm, closed), 1,4-dioxane (1 mL), 24 h, 140 °C. Isolated yields.
72 h.
|
|
To demonstrate the power of the strategy, we further challenged us by testing less reactive anilines in this reaction, which would be even more difficult arising from a higher energy barrier for the possible dearomization process in the sulfuration step. To our delight, after slightly modifying the reaction conditions with additional NaI, which might make the isocyanate intermediate more active10 and/or promote the S–S bond cleavage, we could apply simple anilines 7 in such a three-component reaction to give the desired products 8a–8d in moderate yields (Table 4). When we used a meta-substituted aniline 7d as the substrate, we observed a highly regioselective sulfuration at the position with less steric hindrance. As the ortho-substituted anilines are less reactive due to the steric hindrance, the corresponding products could only be obtained with low yields (please see ESI† for details). Successful implementation of these reactions suggests that this strategy would be useful for the formation of valuable thiazolidin-2-one derivatives.
Table 4 Substrate scope of anilinesa
Reaction conditions: 7 (0.2 mmol), S8 (32 g mmol−1, 0.5 mmol, 16 mg), LiOtBu (6.5 eq.), NaI (1.0 eq.), CO2 (1 atm, closed), DMF (0.5 mL), 36 h, 140 °C. Isolated yields.
60 h.
|
|
To shed light on the mechanism of this reaction, a variety of control experiments were performed (Scheme 2). In the presence of TEMPO or PhSeSePh as the radical scavenger (Scheme 2A), the reaction of 1a underwent smoothly to give the desired product 2a in 80% and 82% yield respectively, which implied that a single electron transfer pathway might not be involved in this reaction. As we could detect the carbamate and urea as by-products in the reaction mixture, we applied tert-butyl naphthalen-2-ylcarbamate 9, 1,3-di(naphthalen-1-yl)urea 10 and 1-isocyanatonaphthalene 11 as starting materials in such a transformation respectively (Scheme 2B–D). We found all of these three compounds could be transformed into the final products under CO2 or N2 atmosphere. However, the efficiency of the reactions of 9 and 10 under N2 was much lower than those under CO2, indicating that neither carbamate nor urea is the key intermediate to undergo following sulfuration. Moreover, N-phenylnaphthalen-2-amine 12 could not be converted into 13 under the standard conditions (Scheme 2E). All of these results indicated that the isocyanate might be the most active intermediate to generate the desired product. When we applied 2-aminonaphthalene-1-thiol 14 in the reaction in the presence or absence of S8, we could obtain the desired product 2a in good yields in both cases (Scheme 2F). However, we were not able to detect the sulfuration of arylamines under standard reaction conditions, which indicated that the sequence of sulfuration and then carbonylation might be less favored. Therefore, we speculate that the formation of isocyanate and following cyclization reaction would be more favored to provide the desired product.
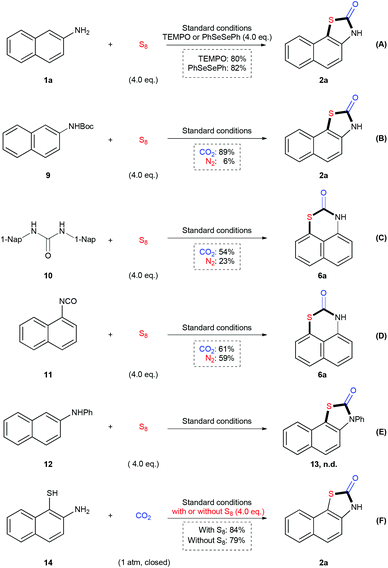 |
| Scheme 2 Control experiments. 1-Nap = 1-naphthyl. | |
Based on the results above and previous reports,4e,p,10 we proposed a possible mechanism (Scheme 3). Arylamine 1 might react with two molecules of CO2 in the presence of NaOtBu to generate the isocyanate C, along with the formation of A and B as intermediates as well as NaHCO3 and HOtBu as by-products.4p Both of D and E, which might be formed from 1 or C in the presence of CO2 and/or NaOtBu, could also decompose to generate 1 and C. Meanwhile, nucleophilic attack to elemental sulfur might lead to ring-opening generation of F.9l Then, nucleophilic attack of F to the isocyanate C would generate G (path A), which could further undergo intramolecular sulfuration to give H. Final deprotonation and re-aromatization would afford the desired product. The intermolecular sulfuration of arylamine derivatives, such as 1 and intermediates A–E, with S8 to generate the aryl thiolate J (path B) and following intramolecular cyclization with isocyanates might also be possible (please see ESI† for details).
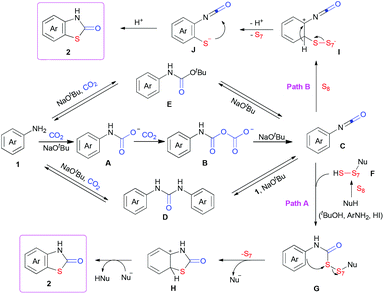 |
| Scheme 3 Proposed mechanism. | |
Conclusions
In summary, we have developed the first three-component reaction to synthesize valuable thiazolidin-2-ones from arylamines, S8, and CO2via C(sp2)–H bond functionalization. This strategy features easy availability of starting materials, broad substrate scope, and good functional group tolerance, allowing access to a series of functionalized thiazolidin-2-one derivatives in the absence of transition metals and external oxidants. The preliminary mechanistic studies indicate that isocyanate might be the key intermediate.
Conflicts of interest
There are no conflicts to declare.
Acknowledgements
We thank the National Natural Science Foundation of China (21822108, 21801025), Sichuan Science and Technology Program (2019YJ0669, 20CXTD0112), and the Fundamental Research Funds for the Central Universities for financial support. We also thank the comprehensive training platform of the Specialized Laboratory in the College of Chemistry at Sichuan University for compound testing.
Notes and references
-
(a)
Carbon Dioxide as Chemical Feedstock, ed. M. Aresta, Wiley-VCH, Weinheim, Germany, 2010 Search PubMed;
(b)
Carbon Dioxide Chemistry, ed. L.-N. He, Science Press, Beijing, 2013 Search PubMed;
(c) L. Ackermann, Angew. Chem., Int. Ed., 2011, 50, 3842–3844 CrossRef CAS PubMed;
(d) K. Huang, C.-L. Sun and Z.-J. Shi, Chem. Soc. Rev., 2011, 40, 2435–2452 RSC;
(e) M. Cokoja, C. Bruckmeier, B. Rieger, W. A. Herrmann and F. E. Kühn, Angew. Chem., Int. Ed., 2011, 50, 8510–8537 CrossRef CAS PubMed;
(f) R. Martín and A. W. Kleij, ChemSusChem, 2011, 4, 1259–1263 CrossRef PubMed;
(g) T. Tsuji and T. Fujihara, Chem. Commun., 2012, 48, 9956–9964 RSC;
(h) W. Zhang and X.-B. Lu, Chin. J. Catal., 2012, 33, 745–756 CrossRef CAS;
(i) M. He, Y. Sun and B. Han, Angew. Chem., Int. Ed., 2013, 52, 9620–9633 CrossRef CAS PubMed;
(j) L. Zhang and Z. Hou, Chem. Sci., 2013, 4, 3395–3403 RSC;
(k) C. Maeda, Y. Miyazaki and T. Ema, Catal. Sci. Technol., 2014, 4, 1482–1497 RSC;
(l) M. Aresta, A. Dibenedetto and A. Angelini, Chem. Rev., 2014, 114, 1709–1742 CrossRef CAS PubMed;
(m) Q. Liu, L. Wu, R. Jackstell and M. Beller, Nat. Commun., 2015, 6, 5933 CrossRef PubMed;
(n) X.-F. Wu and F. Zheng, Top. Curr. Chem., 2017, 375, 4–63 CrossRef PubMed;
(o) J. Luo and I. Larrosa, ChemSusChem, 2017, 10, 3317–3332 CrossRef CAS PubMed;
(p) A. Tortajada, F. Juliá-Hernández, M. Börjesson, T. Moragas and R. Martin, Angew. Chem., Int. Ed., 2018, 57, 15948–15982 CrossRef CAS PubMed;
(q) Y.-G. Chen, X.-T. Xu, K. Zhang, Y.-Q. Li, L.-P. Zhang, P. Fang and T.-S. Mei, Synthesis, 2018, 50, 35–48 CrossRef CAS;
(r) J. Hou, J.-S. Li and J. Wu, Asian J. Org. Chem., 2018, 7, 1439–1447 CrossRef CAS;
(s) S.-S. Yan, Q. Fu, L.-L. Liao, G.-Q. Sun, J.-H. Ye, L. Gong, Y.-Z. Bo-Xue and D.-G. Yu, Coord. Chem. Rev., 2018, 374, 439–463 CrossRef CAS;
(t) C. S. Yeung, Angew. Chem., Int. Ed., 2019, 58, 5492–5502 CrossRef CAS PubMed;
(u) Z. Zhang, L. Gong, X.-Y. Zhou, S.-S. Yan, J. Li and D.-G. Yu, Acta Chim. Sin., 2019, 77, 783–793 CrossRef CAS;
(v) M. D. Burkart, N. Hazari, C. L. Tway and E. L. Zeitler, ACS Catal., 2019, 9, 7937–7956 CrossRef CAS;
(w) Z. Zhang, J.-H. Ye, T. Ju, L.-L. Liao, H. Huang, Y.-Y. Gui, W.-J. Zhou and D.-G. Yu, ACS Catal., 2020, 10, 10871–10885 CrossRef CAS;
(x) C.-K. Ran, X.-W. Chen, Y.-Y. Gui, J. Liu, L. Song, K. Ren and D.-G. Yu, Sci. China: Chem., 2020, 63, 1336–1351 CrossRef CAS;
(y) X. He, L.-Q. Qiu, W.-J. Wang, K.-H. Chen and L.-N. He, Green Chem., 2020, 20, 7301–7320 RSC;
(z) K.-H. Chen, H.-R. Li and L.-N. He, Chin. J. Org. Chem., 2020, 40, 2195–2207 CrossRef;
(a
a) X. Guo, Y. Wang, J. Chen, G. Li and J.-B. Xia, Chin. J. Org. Chem., 2020, 40, 2208–2220 CrossRef;
(a
b) C. Zhou, M. Li, J. Yu, S. Sun and J. Cheng, Chin. J. Org. Chem., 2020, 40, 2221–2231 CrossRef;
(a
c) B. Limburg, À. Cristòfol, F. D. Monica and A. W. Kleij, ChemSusChem, 2020, 13, 1056–1065 Search PubMed;
(a
d) Z. Fan, Z. Zhang and C. Xi, ChemSusChem, 2020, 13, 1056–1065 Search PubMed;
(a
e) Z. Chen, S. Du, J. Zhang and X.-F. Wu, Green Chem., 2020, 22, 8169–8182 RSC.
- For reviews, see:
(a) Q.-W. Song, Z.-H. Zhou and L.-N. He, Green Chem., 2017, 19, 3707–3728 RSC;
(b) Z. Zhang, J.-H. Ye, D.-S. Wu, Y.-Q. Zhou and D.-G. Yu, Chem. – Asian J., 2018, 13, 2292–2306 CrossRef CAS PubMed;
(c) S. Wang and C. Xi, Chem. Soc. Rev., 2019, 48, 382–404 RSC. For selected examples, see:
(d) T. Toda and Y. Kitagawa, Angew. Chem., Int. Ed. Engl., 1987, 26, 334–335 CrossRef;
(e) E. García-Egido, I. Fernández and L. Muñoz, Synth. Commun., 2006, 36, 3029–3042 CrossRef;
(f) Y. Takeda, S. Okumura, S. Tone, I. Sasaki and S. Minakata, Org. Lett., 2012, 14, 4874–4877 CrossRef CAS PubMed;
(g) L. Soldi, C. Massera, M. Costa and N. Della Ca’, Tetrahedron Lett., 2014, 55, 1379 CrossRef CAS;
(h) W. J. Yoo, T. V. Nguyen and S. Kobayashi, Angew. Chem., Int. Ed., 2014, 53, 10213–10217 CrossRef CAS PubMed;
(i) B. A. Vara, T. J. Struble, W. Wang, M. C. Dobish and J. N. Johnston, J. Am. Chem. Soc., 2015, 137, 7302–7305 CrossRef CAS PubMed;
(j) J.-H. Ye, L. Song, W.-J. Zhou, T. Ju, Z.-B. Yin, S.-S. Yan, Z. Zhang, J. Li and D.-G. Yu, Angew. Chem., Int. Ed., 2016, 55, 10022–10026 CrossRef CAS PubMed;
(k) J. Rintjema, R. Epping, G. Fiorani, E. Martin, E. C. Escudero-Adán and A. W. Kleij, Angew. Chem., Int. Ed., 2016, 55, 3972–3976 CrossRef CAS PubMed;
(l) M.-Y. Wang, Y. Cao, X. Liu, N. Wang, L.-N. He and S.-H. Li, Green Chem., 2017, 19, 1240–1244 RSC;
(m) J.-H. Ye, L. Zhu, S.-S. Yan, M. Miao, X.-C. Zhang, W.-J. Zhou, J. Li, Y. Lan and D.-G. Yu, ACS Catal., 2017, 7, 8324–8330 CrossRef CAS;
(n) Z.-B. Yin, J.-H. Ye, W.-J. Zhou, Y.-H. Zhang, L. Ding, Y.-Y. Gui, S.-S. Yan, J. Li and D.-G. Yu, Org. Lett., 2018, 20, 190–193 CrossRef CAS PubMed;
(o) L. Sun, J.-H. Ye, W.-J. Zhou, X. Zeng and D.-G. Yu, Org. Lett., 2018, 20, 3049–3052 CrossRef CAS PubMed;
(p) L. Zhu, J.-H. Ye, M. Duan, X. Qi, D.-G. Yu, R. Bai and Y. Lan, Org. Chem. Front., 2018, 5, 633–639 RSC;
(q) R. Yousefi, T. J. Struble, J. L. Payne, M. Vishe, N. D. Schley and J. N. Johnston, J. Am. Chem. Soc., 2019, 141, 618–625 CrossRef CAS PubMed;
(r) C.-K. Ran, H. He, X.-H. Li, W. Wang, J.-H. Ye, S.-Y. Yan, B.-Q. Wang, C. Feng and D.-G. Yu, Chin. J. Chem., 2020, 38, 69–76 CrossRef CAS.
-
(a) Z. Zhang, T. Ju, J.-H. Ye and D.-G. Yu, Synlett, 2017, 28, 741 CrossRef CAS;
(b) L. Song, Y.-X. Jiang, Z. Zhang, Y.-Y. Gui, X.-Y. Zhou and D.-G. Yu, Chem. Commun., 2020, 56, 8355–8367 RSC.
- For selected C–H carbonylations with CO2:
(a) K. Sasano, J. Takaya and N. Iwasawa, J. Am. Chem. Soc., 2013, 135, 10954–10957 CrossRef CAS PubMed;
(b) W.-Z. Zhang, S. Liu and X.-B. Lu, Beilstein J. Org. Chem., 2015, 11, 906–912 CrossRef CAS PubMed;
(c) Z. Zhang, L.-L. Liao, S.-S. Yan, L. Wang, Y.-Q. He, J.-H. Ye, J. Li, Y.-G. Zhi and D.-G. Yu, Angew. Chem., Int. Ed., 2016, 55, 7068–7072 CrossRef CAS PubMed;
(d) W.-Z. Zhang, M.-W. Yang and X.-B. Lu, Green Chem., 2016, 18, 4181–4184 RSC;
(e) S. Wang, P. Shao, G. Du and C. Xi, J. Org. Chem., 2016, 81, 6672–6676 CrossRef CAS PubMed;
(f) W.-Z. Zhang, M.-W. Yang and X.-B. Lu, Green Chem., 2016, 18, 4181–4184 RSC;
(g) Z. Zhang, T. Ju, M. Miao, J.-L. Han, Y.-H. Zhang, X.-Y. Zhu, J.-H. Ye, D.-G. Yu and Y.-G. Zhi, Org. Lett., 2017, 19, 396–399 CrossRef CAS PubMed;
(h) L. Song, L. Zhu, Z. Zhang, J.-H. Ye, S.-S. Yan, J. Han, Z.-B. Yin, Y. Lan and D.-G. Yu, Org. Lett., 2018, 20, 3776–3799 CrossRef CAS PubMed;
(i) L. Fu, S. Li, Z. Cai, Y. Ding, X. Guo, L. Zhou, D. Yuan, Q. Sun and G. Li, Nat. Catal., 2018, 1, 469–478 CrossRef CAS;
(j) R. Huang, S. Li, L. Fu and G. Li, Asian J. Org. Chem., 2018, 7, 1376–1379 CrossRef CAS;
(k) Z. Zhang, C.-J. Zhu, M. Miao, J.-L. Han, T. Ju, L. Song, J.-H. Ye, J. Li and D.-G. Yu, Chin. J. Chem., 2018, 36, 430–436 CrossRef CAS;
(l) Z. Cai, S. Li, Y. Gao and G. Li, Adv. Synth. Catal., 2018, 360, 4005–4011 CrossRef CAS;
(m) W. Li, C. Li and Y. Lyu, Org. Chem. Front., 2018, 5, 2189–2201 RSC;
(n) L. Song, G.-M. Cao, W.-J. Zhou, J.-H. Ye, Z. Zhang, X.-Y. Tian, J. Li and D.-G. Yu, Org. Chem. Front., 2018, 5, 2086–2090 RSC;
(o) Y. Gao, Z. Cai, S. Li and G. Li, Org. Lett., 2019, 21, 3663–3699 CrossRef CAS PubMed;
(p) Z. Zhang, X.-Y. Zhou, J.-G. Wu, L. Song and D.-G. Yu, Green Chem., 2020, 22, 28–32 RSC.
-
(a) T. Onkol, D. S. Dogruer, S. Ito and M. F. Sahin, Arch. Pharm. Med. Chem., 2000, 333, 337–340 CrossRef CAS;
(b) R. V. Bonnert, R. C. Brown, D. Chapman, D. R. Cheshire, J. Dixon, F. Ince, E. C. Kinchin, A. J. Lyons, A. M. Davis and C. Hallam, J. Med. Chem., 1998, 41, 4915–4917 CrossRef CAS PubMed;
(c) M. J. Stocks, L. Alcaraz, A. Bailey, R. Bonnert, E. Cadogan, J. Christie, S. Connolly, A. Cook, A. Fisher, A. Flaherty, S. Hill, A. Humphries, A. Ingall, S. Jordan, M. Lawson, A. Mullen, D. Nicholls, S. Paine, G. Pairaudeau, S. St-Gallay and A. Young, Bioorg. Med. Chem. Lett., 2011, 21, 4027–4031 CrossRef CAS PubMed;
(d) R. P. Austin, P. Barton, R. V. Bonnert, R. C. Brown, P. A. Cage, D. R. Cheshire, A. M. Davis, I. G. Dougall, F. Ince and G. Pairaudeau, J. Med. Chem., 2003, 46, 3210–3020 CrossRef CAS PubMed;
(e) S. Inoue and T. Kato, J. Pestic. Sci., 1983, 8, 333–338 CrossRef CAS;
(f) S. Inoue, T. Uematsu and T. Kato, J. Pestic. Sci., 1984, 9, 689–695 CrossRef CAS.
-
(a) Y. Wan, M. Alterman, M. Larhed and A. Hallberg, J. Org. Chem., 2002, 67, 6232–6235 CrossRef CAS PubMed;
(b) X. Wu, A. K. Mahalingam, Y. Wan and M. Alterman, Tetrahedron Lett., 2004, 45, 4635–4638 CrossRef CAS;
(c) L. Troisi, C. Granito, S. Perrone and F. Rosato, Tetrahedron Lett., 2011, 52, 4330–4332 CrossRef CAS;
(d) S. Ding and N. Jiao, Angew. Chem., Int. Ed., 2012, 51, 9226–9237 CrossRef CAS PubMed;
(e) B. Yu, H. Zhang, Y. Zhao, S. Chen, J. Xu, L. Hao and Z. Liu, ACS Catal., 2013, 3, 2076–2089 CrossRef CAS;
(f) Y. Jing, R. Liu, Y. Lin and X. Zhou, Sci. China: Chem., 2014, 57, 1117–1125 CrossRef CAS;
(g) B. Zhou, H. Hong, H. Wang, T. Zhang, L. Han and N. Zhu, Eur. J. Org. Chem., 2018, 6983–6900 CrossRef CAS.
-
(a) J. Li, Y. Zhang, Y. Jiang and D. Ma, Tetrahedron Lett., 2012, 53, 2511–2513 CrossRef CAS;
(b) K. E. Ryu, B. R. Kim, G. H. Sueng, H. J. Yoon and Y.-J. Yoon, Synlett, 2015, 26, 1985–1990 CrossRef CAS;
(c) H.-X. Weng, Z.-Q. Ji, Y. Chu, C. Cheng and J.-J. Zhang, Environ. Earth Sci., 2012, 65, 561–569 CrossRef CAS;
(d) T. Itoh and T. Mase, Org. Lett., 2007, 9, 3687–3689 CrossRef CAS PubMed.
- For recent reviews, see:
(a) H. Liu and X. Jiang, Chem. – Asian J., 2013, 8, 2546–2563 CrossRef CAS PubMed;
(b) T. B. Nguyen, Adv. Synth. Catal., 2017, 359, 1066–1130 CrossRef CAS;
(c) T. B. Nguyen, Asian J. Org. Chem., 2017, 6, 477 CrossRef CAS.
- For selected examples, see:
(a) L.-L. Sun, C.-L. Deng, R.-Y. Tang and X.-G. Zhang, J. Org. Chem., 2011, 76, 7546–7550 CrossRef CAS PubMed;
(b) T. B. Nguyen, L. Ermolenko, P. Retailleau and A. Al-Mourabit, Angew. Chem., Int. Ed., 2014, 53, 13808–13812 CrossRef CAS PubMed;
(c) Y. Yang, X. Zhang, W. Zeng, H. Huang and Y. Liang, RSC Adv., 2014, 4, 6090–6093 RSC;
(d) Y. Liao, Y. Peng, H. Qi, G.-J. Deng, H. Gong and C.-J. Li, Chem. Commun., 2015, 51, 1031–1034 RSC;
(e) F. Chen, G. Liao, X. Li, J. Wu and B.-F. Shi, Org. Lett., 2014, 16, 5644–5647 CrossRef CAS PubMed;
(f) L. Meng, T. Fujikawa, M. Kuwayama, Y. Segawa and K. Itami, J. Am. Chem. Soc., 2016, 138, 10351–10355 CrossRef CAS PubMed;
(g) H. Xie, J. Cai, Z. Wang, H. Huang and G.-J. Deng, Org. Lett., 2016, 18, 2196–2199 CrossRef CAS PubMed;
(h) X. Zhu, Y. Yang, G. Xiao, J. Song, Y. Liang and G. Deng, Chem. Commun., 2017, 53, 11917–11920 RSC;
(i) M. Wu, Y. Jiang, Z. An, Z. Qi and R. Yan, Adv. Synth. Catal., 2018, 360, 4236–4240 CrossRef CAS;
(j) S. S. E. Ghodsinia and B. Akhlaghinia, Green Chem., 2019, 21, 3029–3049 RSC;
(k) A. Dey and A. Hajra, Org. Lett., 2019, 21, 1686–1689 CrossRef CAS PubMed;
(l) M. Saito, S. Murakami, T. Nanjo, Y. Kobayashi and Y. Takemoto, J. Am. Chem. Soc., 2020, 142, 8130–8135 CrossRef CAS PubMed;
(m) X. Ma, X. Yu, H. Huang, Y. Zhou and Q. Song, Org. Lett., 2020, 22, 5284–5288 CrossRef CAS PubMed;
(n) Y. Yue, H. Shao, Z. Wang, K. Wang, L. Wang, K. Zhuo and J. Liu, J. Org. Chem., 2020, 85, 11265–11279 CrossRef CAS PubMed.
-
(a) H. Xu, H. Deng, Z. Li, H. Xiang and X. Zhou, Eur. J. Org. Chem., 2013, 7054–7057 CrossRef CAS;
(b) X. Che, J. Jiang, F. Xiao, H. Huang and G.-J. Deng, Org. Lett., 2017, 19, 4576–4579 CrossRef CAS PubMed.
Footnote |
† Electronic supplementary information (ESI) available. See DOI: 10.1039/d0gc03723k |
|
This journal is © The Royal Society of Chemistry 2021 |