DOI:
10.1039/D1FO01935J
(Review Article)
Food Funct., 2021,
12, 12194-12220
Beneficial effects of cinnamon and its extracts in the management of cardiovascular diseases and diabetes†
Received
19th June 2021
, Accepted 2nd November 2021
First published on 3rd November 2021
Abstract
Cardiovascular diseases (CVDs) and diabetes are the leading causes of death worldwide, which underlines the urgent necessity to develop new pharmacotherapies. Cinnamon has been an eminent component of spice and traditional Chinese medicine for thousands of years. Numerous lines of findings have elucidated that cinnamon has beneficial effects against CVDs in various ways, including endothelium protection, regulation of immune response, lowering blood lipids, antioxidative properties, anti-inflammatory properties, suppression of vascular smooth muscle cell (VSMC) growth and mobilization, repression of platelet activity and thrombosis and inhibition of angiogenesis. Furthermore, emerging evidence has established that cinnamon improves diabetes, a crucial risk factor for CVDs, by enhancing insulin sensitivity and insulin secretion; regulating the enzyme activity involved in glucose; regulating glucose metabolism in the liver, adipose tissue and muscle; ameliorating oxidative stress and inflammation to protect islet cells; and improving diabetes complications. In this review, we summarized the mechanisms by which cinnamon regulates CVDs and diabetes in order to provide a theoretical basis for the further clinical application of cinnamon.
1. Introduction
Cardiovascular diseases (CVDs) and diabetes are the leading causes of death worldwide.1 According to the statistics of the American Heart Association, the prevalence of heart disease in 2017 was 10.6%, and it is reported that 17.8 million people died from CVDs in this year.2,3 Diabetes may result in many types of complications, and cardiovascular complications are the main cause of morbidity and mortality in diabetic patients.1 Currently, various types of Western medicine, such as hypoglycaemic drugs, antihypertensive drugs, statins, and anticoagulants, have been developed. However, the side effects and medication adherence remain concerns.4,5 In recent years, increasingly more attention has been given to the treatment of CVDs and diabetes with traditional Chinese medicine. Herbal medicine could improve the surrogate endpoints of CVD, including blood pressure, electrocardiogram changes or left ventricular function and/or drug-related adverse reactions; and Herbal medicine is considered to be a substitute and supplementary method for the primary and secondary prevention of CVD.6 Traditional Chinese medicine has also been reported to play a more important role in fighting diabetes.7
Cinnamon, a common spice, is an herbal medicine and an anti-inflammatory dietary supplement recommended for the primary and secondary prevention of coronary artery disease.8 Furthermore, cinnamon has been found to decrease fasting blood glucose (FBG) and homeostatic model assessment for insulin resistance (HOMA-IR) level, thus bringing benefits to diabetic patients.9,10 The genus Cinnamomum, also known as cinnamon (Fig. 1), is a small tropical tree of the lauraceae family. There are approximately 250 species of Cinnamomum; and the plant was originally grown in Sri Lanka, East and Middle Asia.11,12 Essential oils made from cinnamon are also widely used in the food and cosmetic industries.13 A plethora of studies have deeply explored the active ingredients and therapeutic effects of cinnamon. Numerous studies have revealed that cinnamon has neuroprotective, lipid-lowering, antioxidant, anti-inflammatory, and hypoglycaemic effects, some of which are closely related to the development of diabetes and CVDs.13–18 In this review, we provide a comprehensive overview of the therapeutic potential and possible mechanisms of cinnamon in treating diabetes and CVDs.
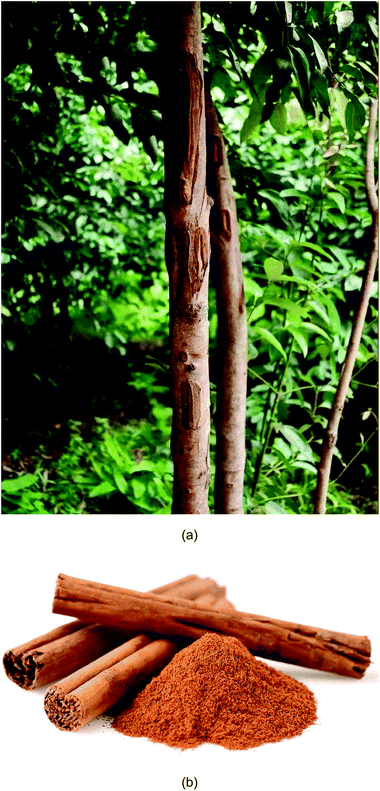 |
| Fig. 1 The whole plant of Cinnanmomi Cortex (a). Traditional Chinese herb Rougui and cinnamon powder (b). | |
2. Chemical constituents and pharmacological features of cinnamon
The German Commission E and the European Scientific Cooperative on Phytotherapy (ESCOP) approved two types of cinnamon for herbal use: Cinnamomum (C.): C. zeylanicum and C. cassia.19 The bark of cinnamon can be peeled and dried in shade to produce Chinese medicine, which can treat chest pain, abdominal pain and dysmenorrhea. Pharmaceutical manufacturers often use Ceylon cinnamon and Chinese cinnamon to produce cinnamon oil, which is absorbed into the body through the skin or olfactory system to play a therapeutic role, for aromatherapy.13,20,21 Different types of cinnamon extracts, such as the methanolic crude extracts of C. verum, the C. zeylanicum Blume essential oil, the ethanol extracts of cinnamon bark, and cinnamon bark aqueous extract, all exhibit antioxidant activity, which indicates the potential of cinnamon to treat oxidative stress-related diseases.22–25
More than 80 compounds were separated and characterized from different parts of cinnamon. The major compounds of cinnamon include eugenol, cinnamaldehyde, camphor, cinnamyl acetate, and copane, as well as other minor constituents (Fig. 2). Eugenol has been reported to be a major component of leaf oil; cinnamaldehyde and camphor were found to be the major compounds of stem bark and root bark volatile oils, respectively; and trans-cinnamyl acetate was identified as a major compound in fruits, flowers, and fruit stalks.12
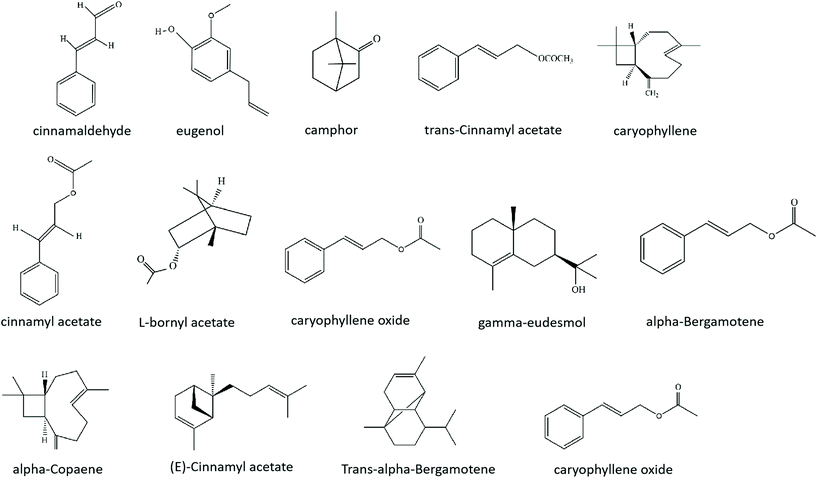 |
| Fig. 2 Chemical structures of relevant isolated components of cinnamon. | |
C. zeylanicum bark essential oil can scavenge free radicals, inhibit the oxidation of β-carotene to play an antioxidant role, and has a dose-dependent antiproliferative effect on adipose-derived mesenchymal stem cells, which makes C. zeylanicum bark essential oils have the potential to develop medical treatments.21 Thirteen compounds were extracted from the essential oil of C. zeylanicum Blume bark, of which (E)-cinnamaldehyde accounted for 97.7%.26 Cinnamaldehyde has been revealed to exert antioxidation, anti-inflammatory, blood vessel protection and hypoglycaemic and antibacterial effects.27–30 The main component of cinnamon leaf essential oil is eugenol.19 Nineteen ingredients were extracted from the volatile oil of C. zeylanicum Blume leaves, and eugenol accounted for 87.3%.26 Eugenol showed very powerful activities of inhibiting peroxynitrite-induced lipid peroxidation.31Trans-Cinnamaldehyde and D-(+)-camphor are the most important compounds in the essential oils extracted from the leaves of two C. osmophloeum clones and could be applied as nutraceuticals or antioxidant remedies with their antioxidant effects.32Trans-Cinnamaldehyde has also been found to possess substantial antimicrobial, anticancer and anti-inflammatory properties.33,34Trans-Cinnamaldehyde, T-cadinol and α-cadinol obtained from the essential oils of C. osmophloeum leaves could strongly inhibit nitric oxide (NO) production and have excellent anti-inflammatory activities, thus having great potential as a source for natural health products.35 The phenolic constituents extracted from the fruits of C. zeylanicum also show antioxidant and radical scavenging activities.36 Gas chromatographic-mass spectrometry studies showed that the main components of the twig essential oil of C. osmophloeum Kaneh are L-bornyl acetate (15.89%), caryophyllene oxide (12.98%), gamma-eudesmol (8.03%), beta-caryophyllene (6.60%), T-cadinol (5.49%), delta-cadinene (4.79%), trans-beta-elemenone (4.25%), cadalene (4.19%), and trans-cinnamaldehyde (4.07%); and trans-cinnamaldehyde, caryophyllene oxide, L-borneol, L-bornyl acetate, eugenol, β-caryophyllene, E-nerolidol, cinnamyl acetate, α-terpineol and p-allylanisole could inhibit the production of NO by lipopolysaccharide (LPS)-stimulated macrophages, thus exhibiting anti-inflammatory properties.37 (E)-Cinnamyl acetate (36.59%) and (E)-caryophyllene (22.36%), which possess good antioxidant activity were found to be major compounds in the volatile oil of C. zeylanicum fruit stalks that possess good antioxidant capacity.38 Several flavonoids obtained from cinnamon also show DPPH radical scavenging activity.39 The major compounds of the hydrodistilled volatile oil of the C. zeylanicum buds are terpene hydrocarbons (78%), alpha-bergamotene (27.38%), alpha-copaene (23.05%) and oxygenated terpenoids (9%).40 The chemical composition of the flower oil of C. zeylanicum was analysed by GC and GC-MS, and it has been reported that (E)-cinnamyl acetate (41.98%), trans-alpha-bergamotene (7.97%), and caryophyllene oxide (7.2%) are major compounds.41 The contents and activities of the chemical constituents of different parts of cinnamon are summarized in Table 1.42–44
Table 1 The concentration and activities of chemical constituents in different parts of cinnamon
Parts of cinnamon |
Dominant ingredients |
Activity |
Ref. |
Bark |
Cinnamaldehyde: 65.00 to 80.00% |
Antioxidant, anti-inflammatory, blood vessel protection and hypoglycemic and anti-bacterial effects |
27–30 and 42–44 |
Eugenol: 5.00 to 10.00% |
Antioxidant activity |
42–44
|
Leaves |
Eugenol: 70.00 to 95.00% |
Antioxidant activity |
31 and 42–44 |
Cinnamaldehyde: 1.00 to 5.00% |
Antioxidant, anti-inflammatory, blood vessel protection and hypoglycemic and anti-bacterial effects |
43 and 44 |
Fruit |
Trans-Cinnamyl acetate: 42.00 to 54.00% |
— |
36 and 42 |
Caryophyllene: 9.00 to 14.00% |
— |
36 and 42 |
Twigs |
L-Bornyl acetate: 15.89% |
Anti-inflammatory activity |
37
|
Caryophyllene oxide: 12.98% |
Anti-inflammatory activity |
37
|
Gamma-eudesmol: 8.03% |
— |
37
|
Fruit stalks |
Cinnamyl acetate: 36.59% |
Antioxidant activity |
38
|
Caryophyllene: 22.36% |
— |
38
|
Buds |
Alpha-bergamotene: 27.38% |
— |
40
|
Alpha-copaene: 23.05% |
— |
40
|
Flowers |
(E)-Cinnamyl acetate: 41.98% |
— |
41
|
Trans-Alpha-bergamotene: 7.97% |
— |
41
|
Caryophyllene oxide: 7.20% |
— |
41
|
3. Side effects and toxicity of cinnamon
Cinnamon is safe to use as a spice and/or flavouring agent. It has been reported that cinnamon has minimal toxic and adverse effects.45 Thirty healthy adults in a clinical trial were instructed to take water extracts of C. zeylanicum with the dose increased at monthly intervals (85 mg d−1, 250 mg d−1 and 500 mg d−1).46 It has been reported that no serious adverse effects (including hypersensitivity) occurred during the 3-month treatment period or 3-month follow-up period after taking C. zeylanicum extract.46 An in vitro study found that indigenous cinnamon (C. osmophloeum) twig extracts had no toxicity on 3T3-L1 preadipocytes at 100 mg mL−1.47 Moreover, a plethora of animal and cell experiments have demonstrated that cinnamon and its constituents alleviate the natural toxin-induced and chemical-induced toxicities.48–56 In MPTP mouse model of Parkinson's disease, daily treatment with cinnamon powder (100 mg per kg body weight, 7 days) protected striatal tyrosine hydroxylase fibers from the toxicity of MPTP and restored the neurotransmitters level.48 In 3 month old Spraque-Dawley male rats, intragastrical pre-treatment with 2.5 ml per kg body weight Oleum cinnamomi 1 h before the ethanol administration protected the stomach, liver and kidney from ethanol-induced damage.49 In healthy Wistar albino rats, oral administration with aqueous bark extract of C. zeylanicum ((2.0 g kg−1, 14 days) showed protective effect against doxorubicin induced cardiotoxicity.50 It was reported that cinnamon diet (25 mg mL−1, 10 days) had a marginal protective effect against paraquat in female flies and cinnamon diet (2.5, 25, and 75 mg mL−1, 10 days) could extend lifespan in the fly.51 In male adult Sprague–Dawley rats, both cinnamic acid (50 mg kg−1, p.o., 7 days) and cinnamaldehyde (40 mg kg−1, p.o., 7 days) revealed the ameliorative effects on cisplatin-induced splenotoxicity.52 In male albino rats, pre-treatment with cinnamon aqueous extract (200 mg per kg per day body weight, 3 times weekly for 50 days) was revealed to ameliorate the Bisphenol A- and octylphenol-induced pathological changes in kidney, brain and testis.53 Another study showed that erythrocytes suspensions incubated with cinnamaldehyde (40 μM) could reduce the hazardous effects of cyadox, maintain the normal function of the body cells and protect the cells against oxidative injury.54 In male Wister rats, cinnamon extract (200 mg per kg body weight orally, 8 weeks) was revealed to improve the liver and kidney functions and ameliorate the toxic effect of cadmium.55 In cultured human lymphocytes, cinnamic acid (0.74, 3.70, 7.40, 14.8, 74.0, 148 mg ml−1) was shown to possess antigenotoxic effects against H2O2 induced genotoxicity.56 Despite this, several studies have identified some side effects that cinnamon may cause in clinical practice. A 13-week repeat-dose oral toxicity study on rats revealed that the use of cinnamon extract in excess of the daily recommended dose, 2000 mg kg−1, may increase the weight of the kidney/liver and the total cholesterol (TC) content.57In vitro mammalian cell micronucleus assays indicated that cinnamon extract (312.5, 625, 1250 μg ml−1) was not aneugenic or clastogenic, and in vivo bone marrow micronucleus assays demonstrated that cinnamon extract (500, 1000, 2000 mg per kg body weight) was not cytotoxic.57 A systematic review of cinnamon's adverse effects identified that cinnamon could cause allergic reactions and gastrointestinal disease, which are self-limiting in most cases.58 A clinical trial involving 15 patients showed that oral administration of 80 mg ethanol extract of cinnamon twice daily for 4 weeks caused stomach aches, nausea, and constipation.59 In a randomized, controlled trial, one of 54 diabetic patients in the cinnamon treatment group developed a rash after administration with usual care with management changes plus 1 g cinnamon capsules daily for 90 days.60 We should also be careful when there is repeated exposure to high levels of cinnamaldehyde (100 μM, 20 min) as an in vitro study on human induced pluripotent stem cell-derived cardiac myocytes (hiPSC-CMs) revealed that cinnamon may cause progressive electrical remodelling and cardiac dysfunction.61 In addition, a randomized controlled trial involving 23 females in the cinnamon group found that some females with polycystic ovarian syndrome developed headaches (4), heartburn symptoms (2), menstrual cramps (2), and nausea with diarrhoea (1) after taking 1.5 g of cinnamon powder daily for 6 months.62 Moreover, seven of the 20 seasonal allergic rhinitis patients who received 200 μg/200 μL cinnamon nasal spray twice a day for 4 weeks developed headaches, coughs, fevers, body aches, and throat irritation.63 Generally, adverse reactions should be monitored when high doses or long durations of cinnamon are for medical purposes, and further study pertaining to cinnamon's toxicity mechanism is necessary.
4 Cinnamon in the treatment of CVDs
4.1 Atherosclerosis (AS)
AS, which leads to lumen stenosis or obstruction, is a crucial cause of various CVDs.64 The occurrence of AS is associated with endothelial dysfunction, elevated blood lipids, imbalanced oxidative stress, dysregulation of the immune response, inflammatory response, growth and mobilization of VSMCs, platelet activity and thrombosis, and angiogenesis.65 Cinnamon exhibits protective effects against AS by modulating various proatherogenic cellular events (Fig. 3).
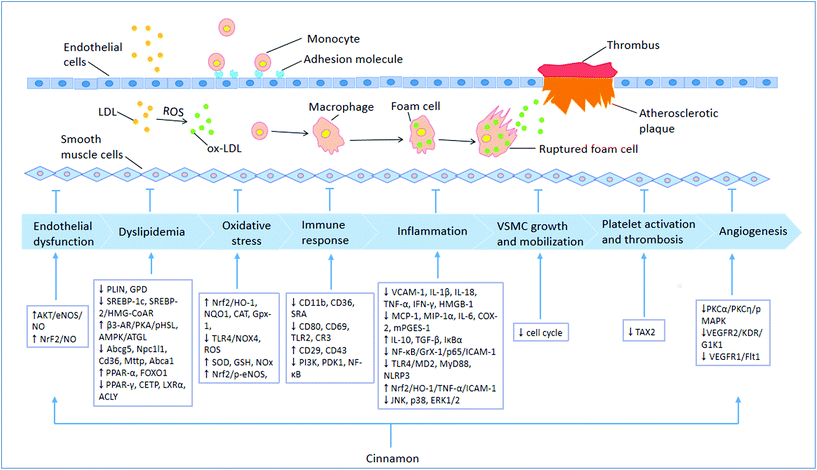 |
| Fig. 3 The anti-atherosclerotic effects by which cinnamon alleviates the development of AS. | |
4.1.1. Endothelium protection.
AS is a complex disease characterized by arterial intima damage. Endothelial dysfunction, the primary stage of AS, is accompanied by a decrease in endothelial permeability and the expression of cytokines and adhesion molecules; later, lipids and macrophages in the blood tend to deposit in the subendothelial space, which further leads to the formation of foam cells.66 Endothelium-dependent diastolic dysfunction is also an important cause of aggravation of atherosclerotic disease. Endothelial cells (ECs) attach to the lining of blood vessels and play a significant role in regulating vascular tension and maintaining the vascular smooth surface whose dysfunction is due to decreased secretion of NO and increased levels of endothelin.67 NO is generated by three isozymes of nitric oxide synthase: endothelial nitric oxide synthase (eNOS), the most important transmitter for EC function; neuronal nitric oxide synthase (nNOS); and inducible nitric oxide synthase (iNOS), which is assumed to be a harmful enzyme and a major contributor to AS in pathological conditions.68,69 Endogenous NO can adjust vascular tension and inhibit the proliferation of vascular smooth muscle, the aggregation of platelets and the development of inflammation.
In isolated rat aortic rings pre-treated with KCl (60 mM), an aqueous extract of C. zeylanicum Blume stem bark exhibited cumulative vasodilating effects partially via enhancement of endothelial NO production and activation of KATP channels in VSMCs.70 A study in isolated rat thoracic aortae showed that cinnamaldehyde obviously alleviated the exaggerated contraction, improved endothelial NO production and attenuated detrimental advanced glycation end products (AGEs)-inflicted vascular damage.71 Cinnamaldehyde also exerts endothelium-dependent vasorelaxant effects, which are affected by NO; and endothelium-independent vasorelaxant 156effects by blocking Ca2+ channels in isolated rat aortae.72 Eugenol enhances the expression of eNOS and the accumulation of intracellular calcium, thus protecting human umbilical vein endothelial cells (HUVECs) from oxidized low-density lipoprotein (ox-LDL)-induced dysfunction.73 Furthermore, cinnamaldehyde was demonstrated to preserve NO levels via endogenous nuclear factor (erythroid-derived 2)-like 2 protein (Nrf2) activation, thus preserving endothelium-dependent relaxation in the endothelium of mouse aortas treated with high glucose.74 According to the experimental results, cinnamon has a beneficial effect on protecting endothelial function through various pathways.
4.1.2. Hypolipidemic effect.
Dyslipidaemia is a crucial factor for the occurrence of atherosclerotic CVDs.75 Low-density lipoprotein (LDL) and other apolipoprotein (apo) B-containing lipoproteins involving very low-density lipoprotein (VLDL), intermediate-density lipoprotein (IDL) and lipoprotein (a) [Lp(a)] are retained and accumulate in the intima of the arterial wall, which together with inflammation and the immune response contribute to the formation of atherosclerotic plaques.76,77 Ox-LDL is closely related to the formation of foam cells whose apoptosis and necrosis result in the release of lipids and the development of AS.78 It has been reported that plasma high-density lipoprotein (HDL) promotes the outflow of cholesterol, which is inversely associated with the risk of atherosclerotic CVD.79 Epidemiological and genetic evidence indicates that elevated triglycerides (TGs) are implicated in the development of CVD.80 TG-rich lipoprotein degradation and uptake into macrophage foam cells in the intima of arteries can lead to inflammation in atherosclerotic CVD.81
A clinical study involving 60 diabetic patients demonstrated that daily consumption of 1, 3, or 6 g of cinnamon reduced low-density lipoprotein cholesterol (LDL-C), mean TG, and TC levels.15 Multiple clinical trials on cinnamon and its constituents have yielded similar results.46,72–74 Ranasinghe et al. investigated the efficacy and safety of cinnamon in healthy adults.46 Subjects were instructed to take the water extract of C. zeylanicum with the dose increased at monthly intervals (85 mg d−1, 250 mg d−1 and 500 mg d−1).46 No significant alteration was observed in HDL-L, VLDL-d and TG levels; and a significant decrease in TC and LDL-C was noted after 3 months of treatment.46 Azimi et al. reported that the consumption of cinnamon (3 g d−1) for 8 weeks reduced TC, LDL, and HDL levels in T2D patients.82 A randomized double-blind control trial evaluated the effect of oral cinnamon consumption (2.5 g d−1) on body composition and metabolic parameters in individuals with metabolic syndrome.83 Serum TC, LDL-C, TG, and HDL-L showed significantly greater improvement in the intervention group.83 Askari et al. conducted a randomized double-blind placebo-controlled trial on fifty patients with non-alcoholic fatty liver disease (NAFLD).84 After 12 weeks of treatment with cinnamon (1.5 g d−1), obvious reductions were observed in TC, TG and LDL-C level.84 Paradoxically, a single-blind randomized crossover study demonstrated that there was no difference in the TC, HDL, or LDL levels of 9 healthy adults treated with 3 g cinnamon or wheat flour (placebo).85 A clinical trial by Blevins was designed to observe the effects of cinnamon on patients with type 2 diabetes.86 The results showed that the lipid levels had no significant change after daily treatment with 1 g cinnamon for 3 months.86
Cinnamon and its extract exhibit lipid-lowering effects in animal experiments. Treatment with cinnamic acid reduced TC and LDL-C levels and increased high-density lipoprotein cholesterol (HDL-C) levels in rats.87 Moreover, the aqueous extract of C. zelanicum significantly prevented dyslipidaemia and protected aortae from the atherosclerotic changes induced by dexamethasone in Wistar rats.88S-(+)-Linalool, a major constituent of the leaf essential oil of C. osmophloeum ct. Linalool, and leaf essential oil resulted in significantly decreased blood TG levels and suppressed lipid accumulation by downregulating 3T3-L1 adipocyte differentiation in mice.89
The molecular mechanism by which cinnamon and its extracts improve lipid profiles is mainly involved in regulating enzymes or genes related to the generation, accumulation, transport and degradation of lipids. First, cinnamon reduces lipogenesis. A large number of studies have revealed that cinnamon attenuates lipogenic processes in the liver and adipose tissue. The chronic inclusion of aqueous cinnamon extract improves the lipid profile in the liver and adipose tissue by downregulating the mRNA expression of sterol regulatory element-binding protein-1c (SREBP-1c) and the mRNA expression of sterol regulatory element-binding protein-2 (SREBP-2) and its target genes 3-hydroxy-3-methylglutaryl-CoA reductase (HMG-CoAR), cholesterol acyltransferase-1 (ACAT-1) and diacylglycerol O-acyltransferase 2 (DGAT2), which are directly involved in lipogenesis.90Trans-Cinnamic acid plays a positive role in regulating lipid metabolism in a series of adipocyte models. Trans-Cinnamic acid was found to reduce the expression of the transcription factors CCAAT/enhancer-binding protein-alpha (C/EBP-α) and peroxisome proliferator-activated receptor γ (PPARγ) in white adipocytes, which tightly modulate the conversion of preadipocytes to mature adipocytes; and increase their expression in brown adipocytes.91 Furthermore, trans-cinnamic acid stimulated the adiponectin (ADIPOQ) secretion and AMP-activated protein kinase (AMPK) activation involved in Gi/Go-protein-coupled receptors (GPRs) in differentiated 3T3-L1 cells.92Trans-Cinnamic acid can activate β3-adrenergic receptors (β3-ARs) and consequently activate protein kinase A (PKA), thus phosphorylating hormoneesensitive lipase (pHSL); and activate AMPK to increase the levels of pHSL and adipocyte triglyceride lipase (ATGL) to upregulate lipid catabolism in 3T3-L1 adipocytes.91 In C57BL/Ks db/db mice, lower TG and TC contents and higher HDL-C and HTR levels (HDL-C/TC × 100) were observed after treatment with Cinnamomi cassiae (Cinnamon bark) extract due to the enhanced expression of PPARα in the liver and PPARγ in adipose tissue.93 Cinnamaldehyde upregulates the expression of HSL and downregulates the expression of glycerol-3-phosphate dehydrogenase (GPD) and adipocyte marker genes, including PPAR-γ and C/EBP-α, to increase adipose tissue lipolysis in mature 3T3-L1 adipocytes in vitro.94 In the adipose tissue of fructose-fed rats, cinnamon extract enhanced the mRNA levels of ADIPOQ, a protein with anti-atherosclerotic potential; and inhibited the level of CD36, an ox-LDL scavenger receptor associated with an increased risk of AS in patients with type 2 diabetes and insulin resistance.95,96
Cinnamon could also improve the intestinal absorption of fat in food. Microsomal TG transfer protein (MTP) is a microsomal protein that facilitates the conversion of VLDL by catalysing the shift of lipids to nascent apoB-containing lipoprotein particles.97 A cinnamon extract (cinnulin PF), high in type A polyphenols, may repress postprandial hyper-triglycerides and the overproduction of apoB48 by inhibiting the overexpression of MTP and SREBP-1c mRNA levels in enterocytes isolated from fructose-fed hamsters.98 Another study on the primary enterocytes of chow-fed rats showed that aqueous cinnamon extract suppresses genes associated with elevated levels of cholesterol, triacylglycerols, and apolipoprotein-B48, including adenosine triphosphate-binding cassette subfamily G member 5 (Abcg5), Niemann-Pick c1-like 1 (Npc1l1), CD36, microsomal triacylglycerol transfer protein (Mttp), and SREBP-1c; and facilitates adenosine triphosphate-binding cassette transporter-1 (Abca1) expression.99
Second, cinnamon could improve the accumulation of lipids. previous research showed that cinnamon reduces lipid accumulation via the upregulation of SREBP-1c, SREBP2, forkhead box O1 (FOXO1) mRNA, and LDL receptors (LDLRs) in adult male zebrafish.100 Another possible mechanism by which cinnamon alleviates lipid accumulation is regulating the expression of cholesteryl ester transfer protein (CETP), a plasma protein that accelerates the migration of cholesteryl ester from HDL to LDL particles.101 In high-cholesterol diet (HCD)-fed rabbits, cinnamaldehyde prevents lipid accumulation and decreases the levels of TC, TGs, and LDL-C, which might be related to the lower mRNA expression of CETP.29 In mouse RAW264.7 macrophage cells, two cinnamic acid derivatives, 4-hydroxycinnamic acid (L-phenylalanine methyl ester) amide and 3,4-dihydroxyhydrocinammic acid (L-aspartic acid dibenzyl ester) amide, suppress cellular cholesterol storage and transport by inhibiting the allosteric enzymes acyl-CoA: ACAT-1 and ACAT-2 to demonstrate their anti-atherosclerotic potential.102 Intake of cinnamon polyphenol significantly decreases the lipid profile specifically by suppressing the expression of hepatic SREBP-1c, liver X receptor-α (LXR-α), ATP-citrate lyase (ACLY), and fatty acid synthase (FAS) and enhancing PPAR-α expression in the livers of rats fed a high-fat diet (HFD).103 Studies have demonstrated that the modulatory mechanism of the effect of cinnamon on lipid metabolism has multiple pathways, which are expected to offer new ideas and pharmacological bases for the treatment of AS.
4.1.3. Antioxidative effect.
Oxidative stress is a state of imbalance between oxidation and antioxidation. During oxidate stress, the production of reactive oxygen species (ROS) exceeds the scavenging capacity of the antioxidant system, leading to the accumulation of ROS and the main pathological state of oxidative stress.104 Excessive production of ROS promotes lipid peroxidation and the formation of ox-LDL, which then facilitates the secretion of monocyte chemotactic protein 1 (MCP-1), a protein that can draw monocytes through the EC space to a lesion from ECs. Furthermore, overproduction of ROS can induce EC apoptosis and smooth muscle cell proliferation and migration to promote the development of AS.105
A double-blind placebo-controlled trial involving twenty-two subjects revealed that administration of 250 mg of an aqueous extract of cinnamon (cinnulin PF) for 12 weeks shows increased ferric reducing antioxidant power and decreased plasma malondialdehyde (MDA) levels.16 Conversely, a single-blind randomized crossover study of nine healthy young adults showed that the use of 3 g cinnamon supplementation following a high-fat meal had no effect on arterial stiffness and oxidative stress.85
Positive results were obtained in in vivo and in vitro experiments. An in vitro study in HUVECs demonstrated that eugenol treatment inhibits reactive ROS generation.73 Experimental data also revealed that new cinnamic acid derivatives possess useful biological activity as anti-atherosclerotic agents with the inhibition of LDL oxidation in mouse RAW264.7 macrophage cells.102 Furthermore, superoxide dismutase (SOD), glutathione S-transferase (GST), catalase (CAT), glutathione peroxidase (Gpx), haemeoxygenase-1 (HO-1), HMOX1, NAD(P)H dehydrogenase, and quinone 1 (NQO1) are antioxidant enzymes that are conducive to inhibiting the oxidation of LDL. Dietary cinnamate, a phenolic compound found in cinnamon bark, enhances CAT and Gpx activities and suppresses lipid peroxidation in rats fed a high cholesterol diet.106 In HFD and streptozotocin (STZ)-induced diabetic rats, eugenol was demonstrated to reduce the level of MDA and increase the serum level of glutathione (GSH). Previous evidence has uncovered that cinnamaldehyde enhances SOD and CAT activities, restores the expression levels of HO-1, and increases aortic NO metabolite (NOx) content in HCD-fed rabbits.29
Molecular mechanism studies showed that cinnamaldehyde reversed intracellular ROS production through the Toll-like receptor 4-NADPH oxidase 4 (TLR4-NOX4) pathway in male Sprague–Dawley rats stimulated by LPS.107 An experiment using db/db mice showed that cinnamaldehyde attenuated oxidative stress status and increased phosphorylated endothelial nitric oxide synthase (p-eNOS) through activating the Nrf2 signalling pathway.108 Nrf2 can induce the transcription of antioxidant enzymes such as HO-1, HMOX1 and NQO1 by transferring them from the cytoplasm to the nucleus to regulate the adaptive response of cells to oxidative stress.109 Cinnamaldehyde elevated the cellular protein level of HO-1 and facilitated the translocation of Nrf2 to the nucleus in HUVECs, which indicated that Nrf2/HO-1 may be the pathway by which cinnamaldehyde protects against oxidative stress.17 In mouse aortas under high glucose conditions, cinnamaldehyde exerts antioxidant effects mediated through Nrf2 activation and the upregulation of downstream target proteins, including HO-1, NQO1, CAT, and glutathione peroxidase 1 (Gpx-1).74 In short, the anti-atherosclerotic effects of cinnamon can also be explained by its antioxidative properties.
4.1.4. Regulation of immune response.
Macrophages differentiate from monocytes that migrate under the endothelium engulf ox-LDL to generate foam cells.110 If the foam cells exceed the clearing ability of HDL, a large number of them will die and contribute to the formation of lipid pools and promote the migration of smooth muscle cells in the middle layer of the artery to the intima.111 Macrophage scavenger receptors (SRAs) are the main scavenger receptors by which macrophages phagocytose modified LDL. Receptors including CD36 may also be involved in the removal of oxidized LDL particles and the formation of foam cells, and CD11b facilitates monocyte recruitment in atherogenic lesions.112 In phorbol-12-myristate-13-acetate (PMA)-stimulated THP-1 cells, cinnamon water extract interfered with monocyte differentiation and macrophage scavenger activity by decreasing the expression of costimulatory molecules (CD11b and CD36), the uptake of acetyl LDL, and the expression of SRA associated with mitigated activity of extracellular signal-related kinase 1/2 (ERK 1/2).113 In addition, Kim's research results showed that cinnamaldehyde has also been demonstrated to regulate monocyte/macrophage-mediated immune responses by attenuating the upregulation of surface levels of CD80 and CD69, Toll-like receptor 2 (TLR2) and complement receptor 3 (CR3) while enhancing the levels of adhesion molecules such as CD29 and CD43 to block cell–cell adhesion.114 In addition, cinnamaldehyde could be developed as an immunoregulatory drug to prevent AS by exhibiting the functional activation of monocytes and macrophages due to the inhibition of phosphoinositide-3-kinase (PI3K) and phosphoinositide-dependent kinase 1 (PDK1) and nuclear factor-κB (NF-κB) activation.114 These research data reveal that regulation of the immune response is also a pivotal part of the mechanism of cinnamon in the treatment of AS.
4.1.5. Anti-inflammatory activity.
Atherogenesis is a chronic inflammatory reaction.115 Leukocyte recruitment and proinflammatory cytokines play crucial roles in the early stages of atherogenesis. Activation of the inflammatory cascades in vascular ECs stimulates the biosynthesis of adhesion molecules (vascular cell adhesion molecule-1 (VCAM-1), intercellular adhesion molecule-1 (ICAM-1), and E-selection) and chemokines (MCP-1) and promotes the recruitment and retention of circulating monocytes in the intima membrane, which then differentiate into macrophages and aggravate atherosclerotic lesions by transforming into foam cells and secreting plentiful proinflammatory factors.116,117
A clinical trial has demonstrated the anti-inflammatory effects of cinnamon. In a randomized double-blind clinical trial involving 36 women with rheumatoid arthritis, the levels of serum inflammatory factors in the cinnamon group and the control group treated with placebo for 8 weeks were compared. Serum CRP and tumour necrosis factor-α (TNF-α) levels in the cinnamon group were significantly lower than those in the placebo group.118 However, a clinical trial studied 44 adult patients with type 2 diabetes who received either three grams cinnamon supplementation or placebo. The results elucidated that ingestion of cinnamon did not reduce the plasma levels of NF-kB, sirtuin-1 (SIRT1), hs-CRP, interleukin (IL)-6 and TNF-α in type 2 diabetes patients.119 Furthermore, there was no significant difference in the serum levels of ICAM-1 and VCAM-1 in patients between the groups.120
In addition, many in vitro experiments have identified the anti-inflammatory effects of cinnamon. The production of adhesion molecules and subsequent attachment of leukocytes to ECs are the key early events in the pathological process of atherogenesis. Liao's research showed that CA and C. cassia extracts inhibited the expression of VCAM-1 and ICAM-1 and the TNF-induced adhesion of monocytes to ECs at the transcriptional level in human ECs.121 A study using endotoxin-injected mice showed that cinnamaldehyde and linalool mitigated the endotoxin-induced levels of interleukin-1β (IL-1β), IL-18, TNF-α, interferon (IFN)-γ, and inflammatory mediator high-mobility group box 1 protein (HMGB-1) in the spleen and mesenteric lymph nodes.122 In THP-1 monocytes, trans-cinnamaldehyde and p-cymene were found to decrease the secretion of IL-8 and inhibit the phosphorylation of Akt and inhibitor of NF-κB (IκBα).123 Furthermore, Pannee et al. reported that the essential oil of C. cassia leaves and cinnamaldehyde reduced the levels of MCP-1, macrophage inflammatory protein-1α (MIP-1α), TNF-α, IL-1β and IL-6 and inhibited the expression of cyclooxygenase-2 (COX-2) and microsomal prostaglandin-E synthase-1 (mPGES-1) in LPS-activated J774A.1 cells.124 Cassia leaf oil and cinnamaldehyde enhanced mRNA expression and the generation of the anti-inflammatory mediators IL-10 and transforming growth factor-β (TGF-β) in LPS-activated J774A.1 cells.124
In addition, extensive experimental work has clarified the molecular and cellular pathways of inflammation through which cinnamon promotes AS. Tristetraprolin (TTP) family proteins have anti-inflammatory effects, and cinnamon polyphenol extract was found to enhance TTP and TNF mRNA levels in mouse RAW264.7 macrophages.125 A plethora of molecular mechanism studies have demonstrated that cinnamon extract can reduce inflammation by modulating NF-κB, which has been regarded as the central mediator of the inflammatory process.126 NO produced by iNOS plays a crucial role in the pathogenesis of various inflammatory diseases. Experimental data revealed that 2′-hydroxycinnamaldehyde isolated from C. cassia Blume bark inhibits NF-κB activation, thus hindering NO production in RAW264.7 cells.127 Moreover, cinnamaldehyde significantly lowered the levels of TNF-α, and prostaglandin E2 (PGE2) inhibited the expression of iNOS, COX-2, NF-κB, and IκBα in LPS-stimulated mouse macrophages (RAW264.7).128 Cinnamaldehyde was elucidated to significantly reduce the overproduction of inflammatory cytokines, decrease the expression of matrix metalloproteinase-2 (MMP-2) and achieve an anti-atherosclerotic effect through the IκBα/NF-κB signalling pathway in an HFD-fed ApoE−/− atherosclerotic mouse model.129 In TNFα-treated ECs, cinnamaldehyde inhibits p65 nuclear translocation and ICAM-1 expression by mediating the activity of NF-Kb and glutaredoxin-1 (Grx-1).130 In a study using endotoxin-induced mice, cinnamaldehyde and linalool, two major active components in the essential oil of C. osmophloeum leaves, inhibited the activation of NF-κB and caspase-1 activity by decreasing the expression of toll-like receptor 4/myeloid differentiation protein 2 (TLR4/MD2), myeloid differentiation primary response gene 88 (MyD88), nod-like receptor family, pyrin domain containing 3 (NLRP3), apoptosis-associated speck-like protein containing a caspase-recruitment domain (ASC), and caspase-1 in the spleen and mesenteric lymph nodes to exhibit an anti-inflammatory effect.122 Cinnamaldehyde elevates the expression of the Nrf2-related gene HO-1, which is associated with the suppressed expression of TNFα-induced ICAM-1, over long-term pre-treatment to elicit anti-inflammatory activity in TNFα-treated ECs.121 Hong's study showed that treatment with cinnamon water extract reduced serum LPS-induced TNF-α by blocking the degradation of IκBα and the activation of c-Jun NH2-terminal kinase (JNK), p38, and ERK 1/2 in vivo.131 In conclusion, these studies demonstrated that cinnamon extracts may be promising drugs for the treatment and prevention of the inflammatory response in atherogenesis.
4.1.6. Suppression of VSMC growth and mobilization.
Pharmacological effects with antiproliferative and antimigratory properties on VSMCs are conducive to treating AS, given that unlimited proliferation and movement of VSMCs within the arterial wall contribute to plaque expansion and vascular narrowing.132 Cinnamaldehyde was shown to repress the proliferation and migration of VSMCs and decrease the proliferative index of injured arteries in Zucker diabetic fatty rats.133 Another in vitro study also indicated that cinnamaldehyde inhibited ox-LDL-induced VSMC proliferation and migration in VSMCs.134 Platelet-derived growth factor (PDGF) is a potent mitogen and chemoattractant that has been found to promote the proliferation and migration of VSMCs.135 Previous research reported that cinnamon extracts, including cinnamyl alcohol, cinnamic acid, and eugenol, inhibit PDGF-BB-triggered VSMC proliferation by arresting the cell cycle at G0/G1 phase; downregulating the expression of cell cycle-positive regulatory proteins by upregulating p21 and p27 expression; and inhibiting the phosphorylation of anti-phospho-phospholipase C-γ1 (PLCγ1), anti-phospho-phosphatidylinositol 3-kinase-linked protein kinase B (AKT), p38, and JNK, which regulate the activation of VSMC proliferation.136–138 Previous research reported that 2-methoxycinnamaldehyde, a natural compound of C. cassia, suppresses PDGF-induced migration of human aortic smooth muscle cells (HASMCs) and inhibits TNF-α-induced proliferation of HASMCs by lowering the cyclin D1, cyclin D3, cyclin-dependent kinase 4 (CDK 4) and CDK6 levels and enhancing the levels of cyclin-dependent kinase inhibitors p21 and p27.139 Thus, the therapeutic utility of cinnamon in AS has been partly attributed to suppression of VSMC growth and mobilization.
4.1.7. Repression of platelet activity and thrombosis.
Because of circulating haemorheologic abnormalities or procoagulant material upregulation in atherosclerotic lesion areas, platelets are extensively activated and recruited to the damaged endothelium, which initiates the coagulation cascade, thereby inducing a life-threatening acute coronary event, artery thrombosis and vascular occlusion.140 An experiment using a rat model revealed that administration of cinnamaldehyde significantly prolonged haemorrhage and coagulation times by mitigating platelet aggregation and thrombosis.141In vitro and in endotoxin-treated normal rats, methanolic extract from Cinnamomi Cortex and cinnamic aldehyde, major essential oily components of methanolic extract, repressed the platelet aggregation triggered by collagen, arachidonic acid and adenosine diphosphate.142 Eugenol, amygdalactone, 2-methoxycinnamaldehyde, and coniferaldehyde, 4 compounds obtained from C. cassia, elicited inhibitory potencies on arachidonic acid-, U46619 (a thromboxane A2 mimic)-, and epinephrine-induced platelet aggregation in an in vitro study.143 Thromboxane A2 (TAX2) is the main cyclo-oxygenase derivative of aracidonic acid in platelets and an effective platelet aggregation agent. Cinnamophilin in cinnamon was found to be a TAX2 receptor antagonist that inhibited platelet aggregation in human platelet-rich plasma and rat aortae.144 According to the findings, it is evident that repression of platelet activity and thrombosis is a crucial constituent of the atheroprotective effects of cinnamon.
4.1.8. Inhibition of angiogenesis.
Angiogenesis can aggravate inflammatory responses, induce haemorrhages in the plaque, affect the stability of the plaque, and consequently lead to the occurrence of acute cardiovascular events.145,146 A water extract of C. cassia represses vascular endothelial growth factor (VEGF)-induced angiogenesis in HUVECs by suppressing the tyrosine phosphorylation of VEGFR2 and induces a decrease in VEGF-stimulated microvessel outgrowth in an ex vivo angiogenesis model of a rat aortic ring.147 In HUVECs treated with phorbol ester, cinnamon extract was observed to repress protein kinase C (PKCα and PKCη) mRNA and PKC-dependent phosphorylation of MAPK (pMAPK) in a dose-dependent manner and suppress the mRNA expression of vascular endothelial growth factor receptor 1 (VEGFR1/Flt1) and vascular endothelial growth factor receptor 2 (VEGFR2/KDR/Flk1) to exert inhibitory effects on angiogenesis.148 Experimental data indicate the potential of cinnamon extracts as a good therapeutic drug for inhibiting angiogenesis to improve AS.149
4.2. Hypertension
Hypertension is a common CVD whose continuous progression may lead to a variety of heart, brain, kidney and other complications. Previous research identified that short-term administration of cinnamon shows promising BP lowering potential.150 In a randomized, placebo-controlled clinical trial, Ziegenfuss et al. showed that the cinnamon group (0.5 g d−1, 12 weeks) had significant decreases in FBG and systolic blood pressure (SBP), small but statistically significant decreases in body fat and an increase in lean mass, suggesting that cinnamon was effective in lowering BP.151 Another clinical study yielded similar results.152 Akilen et al. reported that cinnamon (2 g d−1) for 12 weeks lowered glycated haemoglobin (HbA1C), SBP and diastolic blood pressure (DBP) in poorly controlled type 2 diabetes patients.152 A randomized, placebo-controlled clinical trial was designed to evaluate the hypotensive effect of cinnamon. Subjects with type 2 diabetes were instructed to take cinnamon (2.4 g d−1) at the first treatment period visit.153 At the second treatment visit, volunteers were instructed to reduce their intake of cinnamon to 1.2 g d−1 as a maintenance dose.153 After a 12-week treatment period, Wainstein et al. observed lower blood pressures than the baseline values. However, the by-treatment difference in SBP was a function of a regression to the mean rather than a change related to treatment.153 Consistent with this, consumption of a water extract of C. zeylanicum was reported to significantly reduce SBP and DBP in healthy adults.46 A single supplement with cinnamon (3 g d−1, 16 weeks) was revealed to significantly improve the BP of individuals with metabolic syndrome in a randomized double-blind control trial.83 In a randomized double-blind clinical trial, DBP was significantly lower after the administration of cinnamon powder (500 mg d−1, 8 weeks) in women with rheumatoid arthritis.118 A meta-analysis showed that cinnamon may be play a hypotensive role in adults.154
The intake of cinnamon lowered the SBP of spontaneously hypertensive rats consuming sucrose-containing diets. Several studies have shown that cinnamon exerts hypotensive effects through various mechanisms, such as improving endothelial dysfunction, regulating ion channels, and suppressing oxidative stress.155 The production of NO has a protective effect on the endothelium.66 Cinnamaldehyde was demonstrated to improve the relaxation of methylglyoxal (MG)-injured aortas, enhance NO production from isolated aortae, and repress AGE prompted by MG to ameliorate exaggerated vasoconstriction.71 The methanol extract of C. zeylanicum stem bark possesses antihypertensive effects due to the ability to elevate the production of endogenous NO in L-arginine analogues such as Nω nitro-L-arginine methyl-ester (L-NAME)-induced hypertensive rats.156 Another animal experiment revealed that the acute intravenous injection of C. zeylanicum Blume stem bark aqueous extract could decrease the mean arterial BP in a rat model partially by increasing endothelial NO.70 Micelles containing cinnamaldehyde could also induce endothelium-dependent and NOS-dependent relaxations of coronary vascular smooth muscle mediated by NO and H2O2.157 Phosphorylated eNOS at serine 1177 (eNOS-Ser1177) plays a key role in producing NO from ECs. Upregulation of eNOS upstream kinases, such as AMPK, AKT, or PKA, promotes eNOS phosphorylation and may improve endothelial dysfunction. Trans-Cinnamaldehyde stimulates HUVECs to promote the release of NO by significantly increasing eNOS and AKT phosphorylation and its upstream target PI3K, insulin receptor substrate 1 (IRS1).158 Angiotensin converting enzyme (ACE), a nonspecific enzyme in vascular ECs, promotes the conversion of angiotensin I to angiotensin II, which then contracts arteries and veins to increase BP. Cinnamic acid is endowed with a strong effect on the regulation of BP by suppressing ACE activity in serum and defending animals against vasoconstriction in HFD-induced obese rats.159 The function of KATP channels is reduced in hypertension models.160C. zeylanicum Blume stem bark aqueous extract could activate KATP channels in vascular smooth muscle and reduce BP.156 An increased intracellular concentration of Ca2+ would lead to higher vascular wall tension and consequently elevated BP. Cinnamaldehyde has peripheral vasodilatation properties by blocking Ca2+ channels in mouse ventricular cardiomyocytes and in mesenteric artery smooth muscle cells.161 In isolated rings of porcine coronary arteries, cinnamaldehyde could relax the coronary arteries by inhibiting the sensitivity of Ca2+ and influx of Ca2+ endothelium-independently.157
Activation of the transient receptor potential ankyrin 1 (TRPA1) channel can cause peripheral vasodilation and a biphasic blood pressure response.162 Intravenously injected TRPA1 agonist cinnamaldehyde contributed to a transient hypotensive response and decreased heart rate (HR) dose-dependently, followed by a more sustained pressor response depending on dose.163 The BP response was lower in TRPA1 KO mice treated with cinnamaldehyde, which showed the involvement of TRPA1 in the modulation of BP.163 Oxidative stress has been implicated in vascular stiffness and remodelling, which is a crucial process of hypertension.164 Cinnamaldehyde was demonstrated to regulate oxidative stress. In rats with fructose-induced hypertension, cinnamaldehyde decreased the elevated SBP and DBP; restored the levels of antioxidants such as Gpx, CAT, SOD, and GSH; and lowered MDA levels.165 These experiments suggested the great potential of cinnamon in treating hypertension.
4.3. Myocardial ischaemia-reperfusion injury (MIRI)
Acute myocardial infarction (AMI) is a leading cause of morbidity and mortality worldwide.166 After treatment for acute myocardial infarction, the coronary artery will be reperfused. However, the process of reperfusion can result in cardiomyocyte death, which is known as myocardial ischaemia reperfusion injury.167 Cinnamon and its extracts have been found to reduce infarct size and bring benefits to the treatment of myocardial infarction through various mechanisms. Administration of C. zeylanicum bark extract significantly improved ischaemia/reperfusion (I/R)-induced myocardial injury by reducing the infarct size and exhibited a significant decrease in serum cardiac troponin I (cTnI), lactate dehydrogenase (LDH), and MDA levels in a rat model of regional heart ischaemia.168 2-Methoxycinnamaldehyde (2-MCA), an active ingredient of C. cassia, could obviously improve I/R-injury myocardial dysfunction and reduce infarct size in adult male rats.169 The underlying mechanism is that 2-MCA exhibits its antioxidant and anti-inflammatory action through decreasing the expression of HMGB1 and VCAM-1 in I/R myocardium along with increasing HO-1 induction and enhancing SOD activity in ischaemic tissues.169 Cinnamaldehyde is a TRPA1 agonist, and TRPA1 was found to drive myofibroblast trans-differentiation initiated after MI injury, which is important to maintain ventricular wall integrity and to reduce dilation after infarction remodelling.170 Thus, TRPA1 may be a possible target for cinnamon to treat MIRI.
4.4. Viral myocarditis
Viral myocarditis is a disease of inflammatory destruction of the myocardium after myocardial virus infection and is a common cause of sudden cardiac death and dilated cardiomyopathy.171 Research has shown that cinnamon may have the potential to treat viral myocarditis. Previous research demonstrated that α-bromo-4-chlorocinnamaldehyde, synthesized by cinnamaldehyde, dose-dependently decreased the viral titers and alleviated cardiac pathological changes in a coxsackie virus B3-induced viral myocarditis model.173 The underlying mechanism might be inhibiting inflammatory signalling as BCC inhibits the secretion of inflammatory cytokines such as TNF-α, IL-β and IL-6 in cardiomyocytes; suppresses the activation of NF-κB and the degradation and phosphorylation of IκBα; and reduces the protein level of TLR4 in hearts.172
4.5. Heart failure
Myocardial fibrosis accelerates the development of heart dysfunction and eventually leads to heart failure.173 Cinnamaldehyde was identified to improve cardiac fibrosis, which exhibited possible benefits for the treatment of heart failure.174 Cinnamaldehyde was revealed to inhibit NLRP3 inflammasome activation by blocking CD36-mediated TLR4/6-IL-1R-associated kinase 4/1 (IRAK4/1) signalling to protect rats from fructose-induced cardiac inflammation and fibrosis.174
4.6. Arrhythmias
There are few studies on the effect of cinnamon on arrhythmias, which needs further study. The only study we retrieved demonstrated that myocardial ischaemia rats after oral administration of C. zeylanicum bark extract showed reduced ventricular tachycardia and ventricular ectopic beat episodes, smoother ST segment changes, shorter QTc, lower R-wave amplitude, and increased heart rate during ischaemia compared to the control group.168
5. Cinnamon in the treatment of diabetes
Diabetes mellitus (DM) is closely related to CVD, which is the most common cause of morbidity and mortality in diabetic patients.175 DM has been shown to independently exacerbate the initiation and progression of AS and blunt the regression of lesions following lipid lowering.176 DM is characterized by chronic hyperglycaemia due to insufficient insulin secretion and/or insulin dysfunction.177 Long-term metabolic disorder of carbohydrates, fat and protein will result in damage to the eyes, kidneys, nerves, heart, blood vessels and other tissues and organs. Furthermore, it was estimated that diabetes prevalence, deaths and health care expenditures attributable to diabetes would cause a large burden on society, finance and the health system worldwide.178 Cinnamon and its extracts have been demonstrated to have therapeutic effects on diabetes mellitus and its cardiovascular complications. The effects of cinnamon on improving diabetes may be achieved in a variety of ways.179–185
5.1. Enhancement of insulin sensitivity and insulin secretion
Multiple studies have reported that cinnamon and its extracts possess the capacity to enhance insulin sensitivity and insulin secretion.186 Administration of C. cassia and zeylanicum extracts was found to increase plasma insulin in rats.187 Polyphenols from cinnamon restored pancreatic weight and alleviated mesenteric white fat accumulation related to improved insulin sensitivity in high fat/high fructose (HF/HF)-fed rats.188 Polyphenols from cinnamon have been shown to improve insulin sensitivity in HF/HF-fed rats.188 The influence of cinnamon on insulin sensitivity and its secretion is achieved by a variety of signalling pathways. Insulin-like growth factor 1 (IGF-1), produced by the liver, could promote glucose uptake in peripheral tissues, increase insulin sensitivity, and inhibit the release of glucose in the liver.189,190 Previous research reported that encapsulated cinnamon oil emulsion (COE) improved the concentrations of glucose, insulin and amylase and enhanced IGF-1 mRNA expression in diabetic rats.191 Insulin receptor-β (IR-β) is auto-phosphorylated and plays a role in phosphorylating insulin receptor substrates. HPLC-purified cinnamon polyphenols increased the levels of IRβ in 3T3-L1 adipocytes.192 NO was found to promote the uptake of insulin-mediated glucose in muscle and play an important role in peripheral tissue uptake of glucose.193,194 The pathway involving IRβ, IRS1, PI3K and eNOS has been reported to account for important physiological actions of insulin in facilitating the production of NO.195,196 In HFD-fed rats, cinnamon extract prevented insulin resistance, enhanced the tyrosine phosphorylation levels of IR-β and IRS-1, and strengthened the association between IRS1 and PI3K by improving NO production.197 Additionally, the decreased AKT signalling is closely related to hepatic insulin resistance.198 Previous research reported that cinnamaldehyde upregulates the IRS1/PI3K/AKT2 signalling pathway to induce its hypoglycaemic effect in a STZ-induced T2D rat model.199 Cinnamaldehyde was revealed to induce the activation of PPARδ, PPARγ and retinoid X receptor (RXR) to enhance insulin sensitivity.200In vivo and in vitro studies have identified that cinnamic acid dose-dependently improved glucose tolerance and obviously enhanced insulin secretion in isolated islets.201 Cinnamon extracts suppressed the expression of glycogen synthase kinase 3 beta (GSK3B), insulin-like growth factor receptor (IGF1R), IGF2R, and phosphatidylinositol 3-kinase regulatory subunit 1 (PIK3R1), which encodes insulin signalling pathway proteins in adipocytes that exhibit antidiabetic activity.202 It is well known that protein tyrosine phosphatase 1B (PTP1B) alleviates the signalling pathways of insulin and leptin receptors. Indigenous cinnamon (C. osmophloeum) twig extracts were found to inhibit PTP1B activity and exhibit great potential as natural health products.47 ADIPOQ is known to improve insulin resistance. In 3T3-L1 adipocytes, treatment with trans-cinnamic acid increased the level of ADIPOQ, which is involved in stimulated GPRs.203 In 3T3-L1 adipocytes, cinnamaldehyde was revealed to improve insulin sensitivity, possibly by activating the TRPA1 pathway.204
5.2. Regulation of the enzyme activity involved in glucose
The regulation of enzyme activities related to glucose metabolism by natural products has attracted much attention.205 In db/db mice, cinnamon extract was revealed to decrease the activity of small intestinal α-glycosidase, which converts carbohydrates into glucose and slows the absorption of carbohydrates in the small intestine.206 Cumulative findings have produced similar results.207 Cinnamon bark extracts were found to inhibit intestinal α-glucosidase and pancreatic α-amylase and exhibited potential in reducing postprandial glucose.208 Another in vitro study revealed that cinnamon may inhibit lipase, α-amylase and α-glucosidase digestive enzymes.209 In rat hepatoma cells, aqueous cinnamon extract was also found to decrease the gene expression of phosphoenolpyruvate carboxykinase (PEPCK) and glucose-6-phosphatase (G6Pase), which regulate hepatic gluconeogenesis, to reduce FBG.210
5.3. Regulation of glucose metabolism in liver, adipose tissue and muscle
The liver plays an important role in glucose metabolism by converting glucose into liver glycogen for storage. Cinnamon extract could increase liver glycogen content, improve insulin action in liver tissues, reduce liver fat and improve glucose homeostasis.211 The upregulation of glucose transporter 2 (GLUT2) expression may increase the hepatic glucose output to the circulatory system.212 SREBP-1c increases the level of GLUT2 mRNA in a primary hepatocyte model.213 Increased FAS mRNA expression is associated with restricted conversion of glucose into fatty acids. PEPCK mRNA is a limiting gene in gluconeogenesis.214 COE dose-dependently decreased hepatic GLUT2, FAS, SREBP-1c and PEPCK gene expression in diabetic rats.191 GLUT-1 could facilitate the transport of glucose into cells.215 Cinnamon extract was reported to upregulate the expression of GLUT1 mRNA levels in adipocytes.202 Reduced activity of pyruvate kinase (PK) in the liver tissue of diabetic rats is associated with reduced glycolysis and amplified gluconeogenesis. Cinnamaldehyde was found to enhance PK levels and alleviate PEPCK levels in diabetic rats.216
Glucose can be converted into fat stored in adipose tissue or converted into muscle glycogen in muscle. Cinnamon was revealed to facilitate the uptake of glucose in adipose tissue and muscle. GLUT4, the main transporter for glucose removal from the circulatory system, works after insulin stimulation. Uncoupling protein-1 (UCP-1) promotes the uptake of glucose by brown adipose tissue. An aqueous cinnamon extract upregulated UCP-1 and GLUT4 in brown adipose tissues and muscles of STZ-induced diabetic rats.217 Serum levels of retinol binding protein 4 (RBP4) and the expression of RBP4 were increased in IR mice, and serum RBP4 levels were negatively correlated with the expression of adipose tissue glucose transporter 4 (GLUT4).218,219 Previous research reported that cinnamaldehyde displays outstanding antidiabetic efficacy by decreasing serum RBP4 levels and enhancing the expression of tissue GLUT4 protein.220 In 3T3 adipocytes, treatment with a cinnamon extract increased glucose uptake but inhibited the secretion of the antidiabetic hormone ADIPOQ to levels that were nondetectable.221 In C57BLKS db/db mice, cinnamaldehyde upregulated the mRNA expression of GLUT-4 and the protein expression of p-Akt in skeletal muscle, which suggested that cinnamaldehyde could regulate the stimulation and translocation of GLUT4 to exert antidiabetic effects.222 In 3T3-L1 adipocytes and C2C12 myotubes, cinnamon extract stimulated the LKB1-AMPK signalling cascade, consequently leading to increased glucose uptake.223
5.4. Protection of islet cells
Cinnamon polyphenols have been shown to ameliorate the pathological damage of pancreatic beta cells in STZ-diabetic mice.175 In addition, cinnamon methanol extract ameliorated pancreatic functions and restored them to a normal state due to the antioxidant activity acquired by their phenolic phytochemicals in diabetic rats.224 Oxidative stress and the inflammatory response may lead to islet β cell injury.225,226 Attenuating oxidative stress and anti-inflammation are crucial ways for cinnamon to treat diabetes. Previous research demonstrated that camphor could enhanced the activity of Gpx, CAT, SOD, and the GSH content in the pancreatic tissues of alloxan-induced diabetic rats.227 Consistent with this is that STZ-induced diabetic rats treated with C. tamala leaf oil experienced reduced blood glucose levels and increased plasma insulin levels, which may be attributed to the antioxidant effect of C. tamala leaf oil.228 COE improved the histological picture of the liver and pancreas by enhancing antioxidant capacity.191 Trimer procyanidin oligomers in cinnamon extracts protected pancreatic β-cells by increasing cell viability and reducing ROS accumulation in H2O2-treated β-cells.229 Previous research demonstrated that premating treatment with cinnamaldehyde alleviated the levels of leptin, TNF-α, MDA and NO while enhancing the levels of GSH and CAT activity at term pregnancy in fatty-sucrosed diet/STZ (FSD/STZ)-induced gestational diabetic rats.230 Leaf essential oil from indigenous cinnamon was reported to enhance the activities of SOD and GSH and ameliorate pancreatic levels of IL-1β, TNF-α and NO, thus protecting pancreatic β cells.231 Human islet amyloid polypeptide (hIAPP), a 37 residue peptide hormone, aggregates and causes dysfunction of pancreatic β-cells.232,233 Proanthocyanidins in cinnamon water extract repressed the amyloid formation of hIAPP and obviously improved the membrane damaging and cytotoxic effects due to hIAPP aggregation.234 Moreover, cinnamon oil was demonstrated to promote the immunoreactivity of pancreatic islet β-cells in type 2 diabetic mice.235
5.5. Modulating gut microbiota
Several animal experiments have identified that PTPBs could reduce blood glucose by altering the composition of the gut microbiota. Previous research reported that treatment with polyphenol-containing extracts from cinnamon bark significantly improved the insulin resistance index in mice fed a HFD, which was achieved by modulating the microbiota composition and reducing inflammation.236 Cinnamaldehyde intervention was demonstrated to significantly increase Lactobacillus johnsonii in T1DM mice, suggesting that cinnamaldehyde could protect against STZ-induced T1DM by interfering with gut microbiota.237
5.6. Improvement of diabetes complications
Cinnamon also shows prospects for improving diabetic complications. AGEs are a stable and heterogeneous group of compounds that are involved in the pathogenesis of diabetic complications.238C. burmanii, Vietnamese; C. loureirii and Ceylon; C. zeylanicum showed great potential to suppress the formation of AGEs.239 In diabetic rats, cinnamon and its active component procyanidin-B2 (PCB2) inhibited AGE accumulation and ameliorated AGE-mediated pathogenesis of diabetic nephropathy.240 It has also been reported that the procyanidin-B2 fraction has the physiological significance of delaying diabetic cataracts by repressing AGEs in diabetic rats.241 Cinnamic aldehyde was reported to ameliorate common metabolic disorder symptoms related to diabetes, improve renal function and minimize pathological alterations by activating Nrf2.242 Moreover, multiple substances, including nonpolar sesquiterpenoids, in the bark of C. cassia were reported to exhibit renoprotective effects by suppressing the expression of fibronectin, MCP-1 and IL-6 in high glucose-induced mesangial cells, which provided evidence for clinical applications of cinnamon in diabetic nephropathy prevention and treatment.243 Consistent with this, administration of cinnamon oil was revealed to blunt oxidant stress-induced renal injury in alloxan-induced diabetic rats due to its antioxidant and insulin interaction properties.244 Furthermore, cinnamaldehyde treatment prevents the elevation of BP in insulin deficiency and insulin resistance by normalizing vascular contractility in rats.245 Cinnamaldehyde was also revealed to improve behavioural deficits in diabetic rats by enhancing brain ChE activity and neurotransmitter levels and reducing IL-6 and TNF-α levels.246 Administration of cinnamic acid was revealed to significantly improve dose-dependent memory in STZ-induced diabetic mice by alleviating oxidative stress and cholinergic dysfunction.247 In obese/T2D female mice, cinnamaldehyde has been shown to possess potential therapeutic value for the management of diabetic gastroparesis, which may be related to modulating key regulating detoxifying enzymes by Nrf2-mediated gastric estrogen receptors (ERs) and nNOS function.248 In addition, the topical application of cinnamon increased fibroblast proliferation and glucose uptake in keratinocytes and consequently accelerated wound healing during diabetes by enhancing the antioxidant capacity and the expression of cyclin D1, GLUT-1 and IGF-1.249
6. Clinical trials of cinnamon and its extracts in DM
Multiple clinical trials revealed that cinnamon improved glycaemic indicators [fasting plasma glucose (FPG), two-hour postprandial glucose (2hpp), HbA1C, fasting insulin, and insulin resistance] and clarified the hypoglycaemic properties of cinnamon. Khan et al. conducted a randomized, placebo-controlled study on 60 subjects with T2DM.15 After 40 days of treatment with cinnamon (1, 3, or 6 g d−1), an improvement was observed in the mean fasting serum glucose levels (18–29%).15 A triple-blind placebo-controlled randomized clinical trial was conducted in patients with T2DM to investigate the efficacy of cinnamon.250 After consuming 500 mg capsules of cinnamon bark twice daily for 3 months, subjects exhibited improved glycaemic indices including FPG, 2hpp, HbA1c, fasting insulin, and insulin resistance.250 Crawford compared the effects of cinnamon capsules (1 g d−1) plus usual care with management changes or usual care with management changes for 90 days in type 2 diabetes patients.60 After the treatment period, the cinnamon group showed lower HbA1c than the usual care alone group.60 Similarly, Mang et al. showed that aqueous cinnamon extract (3 g d−1) reduced fasting plasma glucose levels.251 The effects were more obvious in subjects with a higher initial plasma glucose level, which showed the potential of cinnamon for treating diabetic patients with poor glycaemic control.251 Lu et al. reported that cinnamon extract (120 and 360 mg d−1) decreased HbA1c and FBG levels in a randomized, double-blinded clinical study.252 A randomized, placebo-controlled, triple-blind clinical trial was designed to evaluate the effects of cinnamon (3 g d−1) for 90 days in people with type 2 diabetes.253 The cinnamon group exhibited statistically significant reductions in HbA1c and fasting venous glucose compared with the placebo group.253 Consistent with this, supplementation with cinnamon decreased the HOMA index and FBG in patients with NAFLD.84 Despite this, studies have demonstrated that cinnamon does not contribute to glycaemic control and has no preventive or ameliorating effect on metabolic diseases.254 Altschuler et al. conducted a prospective, double-blind, placebo-controlled study on 72 adolescent type 1 diabetic subjects.255 After 90 days of treatment with cinnamon (1 g d−1), no significant differences were observed in the final A1C, change in A1C, total daily insulin intake, or number of hypoglycaemic episodes.255 A double-blind, randomized, placebo-controlled clinical trial evaluated the hypoglycaemic effects of cinnamon (3 g d−1, 8 weeks) in patients with T2DM.256 No significant changes were observed in the levels of FBG, insulin, haemoglobin bA1c, or HOMA-IR between the cinnamon supplementation group and the placebo group.256
7. Conclusion and future perspectives
In conclusion, a large amount of literature has shown that cinnamon and its components possess great potential in treating diabetes and CVDs, including AS, hypertension, MIRI, viral myocarditis, heart failure, and arrhythmias (Fig. 4 and 5). The potential of cinnamon has been tested in a number of clinical trials, animal and cell experiments (ESI Tables 1 and 2†). Generally, cinnamon's therapeutic capacity for CVDs is attributed to its multifactorial functions. Cinnamon and its extracts, especially cinnamaldehyde, increase the production of endothelial NO by activating the Nrf2 signalling pathway and enhancing pAKT-Ser473 and eNOS-Ser1177, thereby protecting ECs. Cinnamon and its extracts, in particular cinnamaldehyde and trans-cinnamic acid, might attenuate lipogenic processes, improve the intestinal absorption of fat in food and lower the accumulation of lipids to regulate lipid metabolism, thus reducing the risk of CVD. In addition, cinnamon and its extracts could blunt the production of ROS and ameliorate the level of antioxidant enzymes to mitigate the formation of ox-LDL, thereby preventing the occurrence of AS. Cinnamaldehyde can regulate oxidative stress in a TLR4-NOX4- and Nrf2-dependent manner. Furthermore, cinnamon and its extracts have strong roles in modulating immune responses and inflammatory responses. Moreover, cinnamon extracts, such as cinnamaldehyde, cinnamyl alcohol, cinnamic acid, and eugenol, can suppress the growth and mobilization of VSMCs by regulating the cell cycle. Cinnamon and its extracts also repress platelet activity and thrombosis and inhibit angiogenesis, which are helpful in protecting against CVDs.
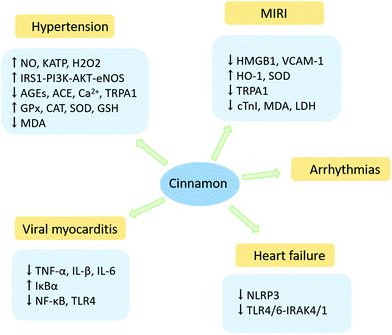 |
| Fig. 4 Molecular mechanism underlying cinnamon protects against hypertension, MIRI, viral myocarditis, heart failure and arrhythmias. | |
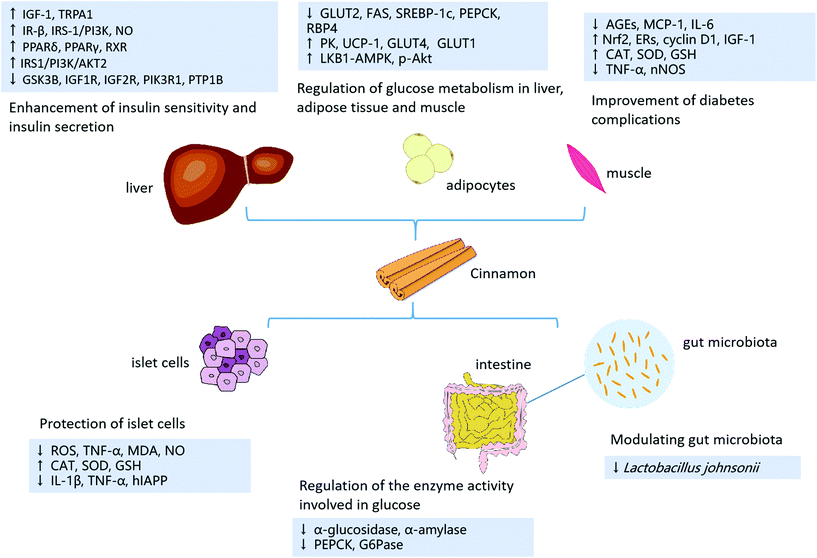 |
| Fig. 5 Molecular mechanim of cinnamon on diabetes. | |
The impact of cinnamon on diabetes is ambiguous. Some clinical studies have yielded conflicting results on whether cinnamon improves glucose metabolism. Several mechanisms could be responsible for the supposed positive effects of cinnamon on diabetes, including enhancing insulin sensitivity and insulin secretion; regulating the enzyme activity involved in glucose; regulating glucose metabolism in the liver, adipose tissue and muscle; ameliorating oxidative stress and inflammation to protect islet cells; and improving diabetes complications.
Evidence for cinnamon and its extracts as a natural product to prevent and counteract CVDs and diabetes is increasing.257 Nevertheless, most experiments were conducted in animals and in vitro, and the clinical effect and metabolic impact in humans need to be further determined. Concluding that intake of cinnamon or its extracts is a useful way to ameliorate the pathological process of CVDs and diabetes is probably premature. Long-term, large-sample, randomized and controlled trials are required to clarify the role of cinnamon in the control of CVDs and diabetes.
Abbreviations
2-MCA | 2-Methoxycinnamaldehyde |
HMG-CoAR | 3-Hydroxy-3-methylglutaryl-CoA reductase |
AMI | Acute myocardial infarction |
Abcg5 | Adenosine triphosphate-binding cassette subfamily G member |
Abca1 | Adenosine triphosphate-binding cassette transporter-1 |
ATGL | Adipocyte triglyceride lipase |
ADIPOQ | Adiponectin |
AGEs | Advanced glycation end products |
AMPK | AMP-activated protein kinase |
ACE | Angiotensin converting enzyme |
AKT | Anti-phospho-phosphatidylinositol 3-kinase-linked protein kinase B |
PLCγ1 | Anti-phospho-phospholipase C-γ1 |
ASC | Apoptosis-associated speck-like protein containing a caspase-recruitment domain |
AS | Atherosclerosis |
ACLY | ATP-citrate lyase |
cTnI | Cardiac troponin I |
CVDs | Cardiovascular diseases |
CAT | Catalase |
C/EBP-α | CCAAT/enhancer-binding protein-alpha |
ACAT-1 | Cholesterol acyltransferase-1 |
CETP | Cholesteryl ester transfer protein |
C. | Cinnamomum |
JNK | c-Jun NH2-terminal kinase |
CR3 | Complement receptor 3 |
CDK 4 | Cyclin-dependent kinase 4 |
COX-2 | Cyclooxygenase-2 |
DM | Diabetes mellitus |
DGAT2 | Diacylglycerol O-acyltransferase 2 |
DBP | Diastolic blood pressure |
COE | Encapsulated cinnamon oil emulsion |
ECs | Endothelial cells |
eNOS | Endothelial nitric oxide synthase |
ERs | Estrogen receptors |
ERK 1/2 | Extracellular signal-related kinase 1/2 |
FBG | Fasting blood glucose |
FPG | Fasting plasma glucose |
FAS | Fatty acid synthase |
FSD/STZ | Fatty-sucrosed diet/STZ |
FOXO1 | Forkhead box O1 |
GPRs | Gi/Go-protein-coupled receptors |
GLUT2 | Glucose transporter 2 |
GLUT4 | Glucose transporter 4 |
G6Pase | Glucose-6-phosphatase |
Grx-1 | Glutaredoxin-1 |
Gpx | Glutathione peroxidase |
Gpx-1 | Glutathione peroxidase 1 |
GST | Glutathione S-transferase |
HbA1C | Glycated haemoglobin |
GPD | Glycerol-3-phosphate dehydrogenase |
GSK3B | Glycogen synthase kinase 3 beta |
HO-1 | Haemeoxygenase-1 |
HR | Heart rate |
HF/HF | High fat/high fructose |
HDL | High-density lipoprotein |
HDL-C | High-density lipoprotein cholesterol |
HFD | High-fat diet |
HMGB-1 | High-mobility group box 1 protein |
HOMA-IR | Homeostatic model assessment for insulin resistance |
HASMCs | Human aortic smooth muscle cells |
hiPSC-CMs | Human induced pluripotent stem cell-derived cardiac myocytes |
hIAPP | human islet amyloid polypeptide |
HUVECs | Human umbilical vein endothelial cells |
IRAK4/1 | IL-1R-associated kinase 4/1 |
iNOS | Inducible nitric oxide synthase |
IκBα | Inhibitor of NF-κB |
IRS1 | Insulin receptor substrate 1 |
IR-β | Insulin receptor-β |
IGF-1 | Insulin-like growth factor 1 |
IGF1R | Insulin-like growth factor 1 receptor |
ICAM-1 | Intercellular adhesion molecule-1 |
IFN-γ | Interferon-γ |
IL-1β | Interleukin-1β |
IDL | Intermediate-density lipoprotein |
I/R | Ischaemia/reperfusion |
LDH | Lactate dehydrogenase |
LDLRs | LDL receptors |
LPS | Lipopolysaccharide |
[Lp(a)] | Lipoprotein (a) |
LXR-α | Liver X receptor-α |
LDL | Low-density lipoprotein |
LDL-C | Low-density lipoprotein cholesterol |
MIP-1α | Macrophage inflammatory protein-1α |
MMP-2 | Matrix metalloproteinase-2 |
MG | Methylglyoxal |
mPGES-1 | Microsomal prostaglandin-E synthase-1 |
MTP | Microsomal TG transfer protein |
Mttp | Microsomal triacylglycerol transfer protein |
MCP-1 | Monocyte chemotactic protein 1 |
MyD88 | Myeloid differentiation primary response gene 88 |
MIRI | Myocardial ischaemia-reperfusion Injury |
NQO1 | NAD(P)H dehydrogenase, and quinone 1 |
nNOS | Neuronal nitric oxide synthase |
Npc1l1 | Niemann-Pick c1-like 1 |
NO | Nitric oxide |
NOx | NO metabolite |
NLRP3 | Nod-like receptor family, pyrin domain containing 3 |
Nrf2 | Nuclear factor (erythroid-derived 2)-like 2 protein |
NF-κB | Nuclear factor-κB |
L-NAME | Nω nitro-L-arginine methyl-ester |
ox-LDL | Oxidized low-density lipoprotein |
PPARγ | Peroxisome proliferator-activated receptor γ |
PMA | Phorbol-12-myristate-13-acetate |
PIK3R1 | Phosphatidylinositol 3-kinase regulatory subunit 1 |
PEPCK | Phosphoenolpyruvate carboxykinase |
PI3K | Phosphoinositide-3-kinase |
PDK1 | Phosphoinositide-dependent kinase 1 |
p-eNOS | Phosphorylated endothelial nitric oxide synthase |
pHSL | Phosphorylating hormonesensitive lipase |
pMAPK | Phosphorylation of MAPK |
PDGF | Platelet-derived growth factor |
PCB2 | Procyanidin-B2 |
PGE2 | Prostaglandin E2 |
PKA | Protein kinase A |
PKC | Protein kinase C |
PTP1B | Protein tyrosine phosphatase 1B |
PK | Pyruvate kinase |
ROS | Reactive oxygen species |
RXR | Retinoid X receptor |
RBP4 | Retinol binding protein 4 |
SRAs | Scavenger receptors |
SIRT1 | Sirtuin-1 |
SREBP 1c | Sterol regulatory element-binding protein 1c |
SREBP 2 | Sterol regulatory element-binding protein 2 |
STZ | Streptozotocin |
SOD | Superoxide dismutase |
SBP | Systolic blood pressure |
ESCOP | The german commission E and the european scientific cooperative on phytotherapy |
TAX2 | Thromboxane A2 |
TLR2 | Toll-like receptor 2 |
TLR4/MD2 | Toll-like receptor 4/myeloid differentiation protein 2 |
TLR4-NOX4 | Toll-like receptor 4-NADPH oxidase 4 |
TC | Total cholesterol |
TGF-β | Transforming growth factor-β |
TRPA1 | Transient receptor potential ankyrin 1 |
TGs | Triglycerides |
TTP | Tristetraprolin |
TNF-α | Tumour necrosis factor-α |
2hpp | Two-hour postprandial glucose |
UCP-1 | Uncoupling protein-1 |
VCAM-1 | Vascular cell adhesion molecule-1 |
VEGF | Vascular endothelial growth factor |
VEGFR | Vascular endothelial growth factor receptor |
VSMC | Vascular smooth muscle cell |
VLDL | Very low-density lipoprotein |
β3-ARs | β3-adrenergic receptors |
Author contributions
CS, HC-L and XQ-F wrote the main text. HC-L and XQ-F contributed equally to this work. CS, HC-L, XQ-F, YL-W, ZL-J, YQ, MX, ZH-S and LY-X reviewed the literature available; JL-G, YD-L and XN-C revised the manuscript.
Conflicts of interest
The authors declare that they do not have anything to disclose regarding conflict of interest with respect to this manuscript.
Acknowledgements
This work was funded by grants from the National Natural Science Foundation of China (No. 81973842). We thank J. L. Gao, Y. D. Lu, and X. N. Cui for the guidance and revision of this manuscript.
References
- Y. Zheng, S. H. Ley and F. B. Hu, Global aetiology and epidemiology of type 2 diabetes mellitus and its complications, Nat. Rev. Endocrinol., 2018, 14, 88–98 CrossRef PubMed.
- G. A. Mensah, G. A. Roth and V. Fuster, The Global Burden of Cardiovascular Diseases and Risk Factors: 2020 and Beyond, J. Am. Coll. Cardiol., 2019, 74, 2529–2532 CrossRef PubMed.
- G. A. Roth, C. Johnson, A. Abajobir, F. Abd-Allah, S. F. Abera, G. Abyu, M. Ahmed, B. Aksut, T. Alam, K. Alam, F. Alla, N. Alvis-Guzman, S. Amrock, H. Ansari, J. Arnlov, H. Asayesh, T. M. Atey, L. Avila-Burgos, A. Awasthi, A. Banerjee, A. Barac, T. Barnighausen, L. Barregard, N. Bedi, E. Belay Ketema, D. Bennett, G. Berhe, Z. Bhutta, S. Bitew, J. Carapetis, J. J. Carrero, D. C. Malta, C. A. Castaneda-Orjuela, J. Castillo-Rivas, F. Catala-Lopez, J. Y. Choi, H. Christensen, M. Cirillo, L. Cooper Jr., M. Criqui, D. Cundiff, A. Damasceno, L. Dandona, R. Dandona, K. Davletov, S. Dharmaratne, P. Dorairaj, M. Dubey, R. Ehrenkranz, M. El Sayed Zaki, E. J. A. Faraon, A. Esteghamati, T. Farid, M. Farvid, V. Feigin, E. L. Ding, G. Fowkes, T. Gebrehiwot, R. Gillum, A. Gold, P. Gona, R. Gupta, T. D. Habtewold, N. Hafezi-Nejad, T. Hailu, G. B. Hailu, G. Hankey, H. Y. Hassen, K. H. Abate, R. Havmoeller, S. I. Hay, M. Horino, P. J. Hotez, K. Jacobsen, S. James, M. Javanbakht, P. Jeemon, D. John, J. Jonas, Y. Kalkonde, C. Karimkhani, A. Kasaeian, Y. Khader, A. Khan, Y. H. Khang, S. Khera, A. T. Khoja, J. Khubchandani, D. Kim, D. Kolte, S. Kosen, K. J. Krohn, G. A. Kumar, G. F. Kwan, D. K. Lal, A. Larsson, S. Linn, A. Lopez, P. A. Lotufo, H. M. A. El Razek, R. Malekzadeh, M. Mazidi, T. Meier, K. G. Meles, G. Mensah, A. Meretoja, H. Mezgebe, T. Miller, E. Mirrakhimov, S. Mohammed, A. E. Moran, K. I. Musa, J. Narula, B. Neal, F. Ngalesoni, G. Nguyen, C. M. Obermeyer, M. Owolabi, G. Patton, J. Pedro, D. Qato, M. Qorbani, K. Rahimi, R. K. Rai, S. Rawaf, A. Ribeiro, S. Safiri, J. A. Salomon, I. Santos, M. Santric Milicevic, B. Sartorius, A. Schutte, S. Sepanlou, M. A. Shaikh, M. J. Shin, M. Shishehbor, H. Shore, D. A. S. Silva, E. Sobngwi, S. Stranges, S. Swaminathan, R. Tabares-Seisdedos, N. Tadele Atnafu, F. Tesfay, J. S. Thakur, A. Thrift, R. Topor-Madry, T. Truelsen, S. Tyrovolas, K. N. Ukwaja, O. Uthman, T. Vasankari, V. Vlassov, S. E. Vollset, T. Wakayo, D. Watkins, R. Weintraub, A. Werdecker, R. Westerman, C. S. Wiysonge, C. Wolfe, A. Workicho, G. Xu, Y. Yano, P. Yip, N. Yonemoto, M. Younis, C. Yu, T. Vos, M. Naghavi and C. Murray, Global, Regional, and National Burden of Cardiovascular Diseases for 10 Causes, 1990 to 2015, J. Am. Coll. Cardiol., 2017, 70, 1–25 CrossRef PubMed.
- A. T. Larkin, C. Hoffman, A. Stevens, A. Douglas and Z. Bloomgarden, Determinants of adherence to diabetes treatment, J. Diabetes, 2015, 7, 864–871 CrossRef PubMed.
- F. Rodriguez, D. J. Maron, J. W. Knowles, S. S. Virani, S. Lin and P. A. Heidenreich, Association of Statin Adherence With Mortality in Patients With Atherosclerotic Cardiovascular Disease, JAMA Cardiol., 2019, 4, 206–213 CrossRef PubMed.
- P. P. Hao, F. Jiang, Y. G. Chen, J. Yang, K. Zhang, M. X. Zhang, C. Zhang, Y. X. Zhao and Y. Zhang, Traditional Chinese medication for cardiovascular disease, Nat. Rev. Cardiol., 2015, 12, 318 CrossRef PubMed.
- X. L. Tong, L. Dong, L. Chen and Z. Zhen, Treatment of diabetes using traditional Chinese medicine: past, present and future, Am. J. Chin. Med., 2012, 40, 877–886 CrossRef PubMed.
- J. H. O'Keefe, N. M. Gheewala and J. O. O'Keefe, Dietary strategies for improving post-prandial glucose, lipids, inflammation, and cardiovascular health, J. Am. Coll. Cardiol., 2008, 51, 249–255 CrossRef PubMed.
- S. Deyno, K. Eneyew, S. Seyfe, N. Tuyiringire, E. L. Peter, R. A. Muluye, C. U. Tolo and P. E. Ogwang, Efficacy and safety of cinnamon in type 2 diabetes mellitus and pre-diabetes patients: A meta-analysis and meta-regression, Diabetes Res. Clin. Pract., 2019, 156, 107815 CrossRef CAS PubMed.
- P. Ranasinghe, R. Jayawardana, P. Galappaththy, G. R. Constantine, N. de Vas Gunawardana and P. Katulanda, Efficacy and safety of ‘true’ cinnamon (Cinnamomum zeylanicum) as a pharmaceutical agent in diabetes: a systematic review and meta-analysis, Diabetic Med., 2012, 29, 1480–1492 CrossRef CAS PubMed.
- H. Mollazadeh and H. Hosseinzadeh, Cinnamon effects on metabolic syndrome: a review based on its mechanisms, Iran. J. Basic Med. Sci., 2016, 19, 1258–1270 Search PubMed.
- G. K. Jayaprakasha and L. J. Rao, Chemistry, biogenesis, and biological activities of Cinnamomum zeylanicum, Crit. Rev. Food Sci. Nutr., 2011, 51, 547–562 CrossRef CAS PubMed.
- S. F. Nabavi, A. Di Lorenzo, M. Izadi, E. Sobarzo-Sanchez, M. Daglia and S. M. Nabavi, Antibacterial Effects of Cinnamon: From Farm to Food, Cosmetic and Pharmaceutical Industries, Nutrients, 2015, 7, 7729–7748 CrossRef CAS PubMed.
- S. C. Ho, K. S. Chang and P. W. Chang, Inhibition of neuroinflammation by cinnamon and its main components, Food Chem., 2013, 138, 2275–2282 CrossRef CAS PubMed.
- A. Khan, M. Safdar, M. M. Ali Khan, K. N. Khattak and R. A. Anderson, Cinnamon improves glucose and lipids of people with type 2 diabetes, Diabetes Care, 2003, 26, 3215–3218 CrossRef PubMed.
- A. M. Roussel, I. Hininger, R. Benaraba, T. N. Ziegenfuss and R. A. Anderson, Antioxidant effects of a cinnamon extract in people with impaired fasting glucose that are overweight or obese, J. Am. Coll. Nutr., 2009, 28, 16–21 CrossRef CAS PubMed.
- N. Y. Kim, N. T. Trinh, S. G. Ahn and S. A. Kim, Cinnamaldehyde protects against oxidative stress and inhibits the TNFalphainduced inflammatory response in human umbilical vein endothelial cells, Int. J. Mol. Med., 2020, 46, 449–457 CAS.
- H. K. Kwon, W. K. Jeon, J. S. Hwang, C. G. Lee, J. S. So, J. A. Park, B. S. Ko and S. H. Im, Cinnamon extract suppresses tumor progression by modulating angiogenesis and the effector function of CD8+T cells, Cancer Lett., 2009, 278, 174–182 CrossRef CAS PubMed.
- J. Gruenwald, J. Freder and N. Armbruester, Cinnamon and health, Crit. Rev. Food Sci. Nutr., 2010, 50, 822–834 CrossRef PubMed.
- D. G. Barceloux, Cinnamon (Cinnamomum species), Dis. Mon., 2009, 55, 327–335 CrossRef PubMed.
- B. A. Behbahani, F. Falah, F. L. Arab, M. Vasiee and F. T. Yazdi, Chemical Composition and Antioxidant, Antimicrobial, and Antiproliferative Activities of Cinnamomum zeylanicum Bark Essential Oil, Evidence-Based Complementary Altern. Med., 2020, 2020, 5190603 Search PubMed.
- S. Sultana, F. A. Ripa and K. Hamid, Comparative antioxidant activity study of some commonly used spices in Bangladesh, Pak. J. Biol. Sci., 2010, 13, 340–343 CrossRef CAS PubMed.
- I. Kallel, B. Hadrich, B. Gargouri, A. Chaabane, S. Lassoued, R. Gdoura, A. Bayoudh and E. B. Messaoud, Optimization of Cinnamon (Cinnamomum zeylanicum Blume) Essential Oil Extraction: Evaluation of Antioxidant and Antiproliferative Effects, Evidence-Based Complementary Altern. Med., 2019, 2019, 6498347 Search PubMed.
- C. H. Yang, R. X. Li and L. Y. Chuang, Antioxidant activity of various parts of Cinnamomum cassia extracted with different extraction methods, Molecules, 2012, 17, 7294–7304 CrossRef CAS PubMed.
- Z. Hussain, J. A. Khan, A. Arshad, P. Asif, H. Rashid and M. I. Arshad, Protective effects of Cinnamomum zeylanicum L. (Darchini) in acetaminophen-induced oxidative stress, hepatotoxicity and nephrotoxicity in mouse model, Biomed. Pharmacother., 2019, 109, 2285–2292 CrossRef CAS PubMed.
- G. Singh, S. Maurya, M. P. DeLampasona and C. A. Catalan, A comparison of chemical, antioxidant and antimicrobial studies of cinnamon leaf and bark volatile oils, oleoresins and their constituents, Food Chem. Toxicol., 2007, 45, 1650–1661 CrossRef CAS PubMed.
- S. Mateen, M. T. Rehman, S. Shahzad, S. S. Naeem, A. F. Faizy, A. Q. Khan, M. S. Khan, F. M. Husain and S. Moin, Anti-oxidant and anti-inflammatory effects of cinnamaldehyde and eugenol on mononuclear cells of rheumatoid arthritis patients, Eur. J. Pharmacol., 2019, 852, 14–24 CrossRef CAS PubMed.
- M. Friedman, Chemistry, Antimicrobial Mechanisms, and Antibiotic Activities of Cinnamaldehyde against Pathogenic Bacteria in Animal Feeds and Human Foods, J. Agric. Food Chem., 2017, 65, 10406–10423 CrossRef CAS PubMed.
- O. A. A. Nour, G. S. G. Shehatou, M. A. Rahim, M. S. El-Awady and G. M. Suddek, Cinnamaldehyde exerts vasculoprotective effects in hypercholestrolemic rabbits, Naunyn-Schmiedeberg's Arch. Pharmacol., 2018, 391, 1203–1219 CrossRef CAS PubMed.
- R. Zhu, H. Liu, C. Liu, L. Wang, R. Ma, B. Chen, L. Li, J. Niu, M. Fu, D. Zhang and S. Gao, Cinnamaldehyde in diabetes: A review of pharmacology, pharmacokinetics and safety, Pharmacol. Res., 2017, 122, 78–89 CrossRef CAS PubMed.
- S. Chericoni, J. M. Prieto, P. Iacopini, P. Cioni and I. Morelli, In vitro activity of the essential oil of Cinnamomum zeylanicum and eugenol in peroxynitrite-induced oxidative processes, J. Agric. Food Chem., 2005, 53, 4762–4765 CrossRef CAS PubMed.
- F. L. Hsu, W. H. Li, C. W. Yu, Y. C. Hsieh, Y. F. Yang, J. T. Liu, J. Shih, Y. J. Chu, P. L. Yen, S. T. Chang and V. H. Liao, In vivo antioxidant activities of essential oils and their constituents from leaves of the Taiwanese Cinnamomum osmophloeum, J. Agric. Food Chem., 2012, 60, 3092–3097 CrossRef CAS PubMed.
- A. A. Doyle and J. C. Stephens, A review of cinnamaldehyde and its derivatives as antibacterial agents, Fitoterapia, 2019, 139, 104405 CrossRef CAS PubMed.
- B. J. Chen, C. S. Fu, G. H. Li, X. N. Wang, H. X. Lou, D. M. Ren and T. Shen, Cinnamaldehyde Analogues as Potential Therapeutic Agents, Mini-Rev. Med. Chem., 2017, 17, 33–43 CrossRef CAS PubMed.
- Y. T. Tung, P. L. Yen, C. Y. Lin and S. T. Chang, Anti-inflammatory activities of essential oils and their constituents from different provenances of indigenous cinnamon (Cinnamomum osmophloeum) leaves, Pharm. Biol., 2010, 48, 1130–1136 CrossRef CAS PubMed.
- G. K. Jayaprakasha, M. Ohnishi-Kameyama, H. Ono, M. Yoshida and L. J. Rao, Phenolic constituents in the fruits of Cinnamomum zeylanicum and their antioxidant activity, J. Agric. Food Chem., 2006, 54, 1672–1679 CrossRef CAS PubMed.
- Y. T. Tung, M. T. Chua, S. Y. Wang and S. T. Chang, Anti-inflammation activities of essential oil and its constituents from indigenous cinnamon (Cinnamomum osmophloeum) twigs, Bioresour. Technol., 2008, 99, 3908–3913 CrossRef CAS PubMed.
- G. K. Jayaprakasha, L. J. M. Rao and K. K. Sakariah, Volatile constituents from Cinnamomum zeylanicum fruit stalks and their antioxidant activities, J. Agric. Food Chem., 2003, 51, 4344–4348 CrossRef CAS PubMed.
- M. Okawa, J. Kinjo, T. Nohara and M. Ono, DPPH (1,1-diphenyl-2-picrylhydrazyl) radical scavenging activity of flavonoids obtained from some medicinal plants, Biol. Pharm. Bull., 2001, 24, 1202–1205 CrossRef CAS PubMed.
- G. K. Jayaprakasha, L. J. Rao and K. K. Sakariah, Chemical composition of volatile oil from Cinnamomum zeylanicum buds, Z. Naturforsch., C: J. Biosci., 2002, 57, 990–993 CrossRef CAS PubMed.
- G. K. Jayaprakasha, L. J. M. Rao and K. K. Sakariah, Chemical composition of the flower oil of Cinnamomum zeylanicum blume, J. Agric. Food Chem., 2000, 48, 4294–4295 CrossRef CAS PubMed.
- P. V. Rao and S. H. Gan, Cinnamon: a multifaceted medicinal plant, Evidence-Based Complementary Altern. Med., 2014, 2014, 642942 Search PubMed.
- N. Singh, A. S. Rao, A. Nandal, S. Kumar, S. S. Yadav, S. A. Ganaie and B. Narasimhan, Phytochemical and pharmacological review of Cinnamomum verum J. Presl-a versatile spice used in food and nutrition, Food Chem., 2021, 338, 127773 CrossRef CAS PubMed.
- Z. Hussain, J. A. Khan and H. Rashid, Cinnamomum zeylanicum (Darchini): A Boon to Medical Science and a Possible Therapy for Stress-Induced Ailments, Crit. Rev. Eukaryot. Gene Expr., 2019, 29, 263–276 CrossRef PubMed.
- P. Ranasinghe, S. Pigera, G. A. Premakumara, P. Galappaththy, G. R. Constantine and P. Katulanda, Medicinal properties of ‘true’ cinnamon (Cinnamomum zeylanicum): a systematic review, BMC Complementary Altern. Med., 2013, 13, 275 CrossRef PubMed.
- P. Ranasinghe, R. Jayawardena, S. Pigera, W. S. Wathurapatha, H. D. Weeratunga, G. A. S. Premakumara, P. Katulanda, G. R. Constantine and P. Galappaththy, Evaluation of pharmacodynamic properties and safety of Cinnamomum zeylanicum (Ceylon cinnamon) in healthy adults: a phase I clinical trial, BMC Complementary Altern. Med., 2017, 17, 550 CrossRef PubMed.
- G. M. Lin, Y. H. Chen, P. L. Yen and S. T. Chang, Antihyperglycemic and antioxidant activities of twig extract from Cinnamomum osmophloeum, J. Tradit. Complementary Med., 2016, 6, 281–288 CrossRef PubMed.
- S. Khasnavis and K. Pahan, Cinnamon treatment upregulates neuroprotective proteins Parkin and DJ-1 and protects dopaminergic neurons in a mouse model of Parkinson's disease, J. Neuroimmune Pharmacol., 2014, 9, 569–581 CrossRef PubMed.
- C. Ozbayer, H. Kurt, Z. Ozdemir, T. Tuncel, S. M. Saadat, D. Burukoglu, H. Senturk, I. Degirmenci and H. V. Gunes, Gastroprotective, cytoprotective and antioxidant effects of Oleum cinnamomi on ethanol induced damage, Cytotechnology, 2014, 66, 431–441 CrossRef CAS PubMed.
- J. A. N. Sandamali, R. P. Hewawasam, K. Jayatilaka and L. K. B. Mudduwa, Cinnamomum zeylanicum Blume (Ceylon cinnamon) bark extract attenuates doxorubicin induced cardiotoxicity in Wistar rats, Saudi Pharm. J., 2021, 29, 820–832 CrossRef CAS PubMed.
- S. E. Schriner, S. Kuramada, T. E. Lopez, S. Truong, A. Pham and M. Jafari, Extension of Drosophila lifespan by cinnamon through a sex-specific dependence on the insulin receptor substrate chico, Exp. Gerontol., 2014, 60, 220–230 CrossRef CAS PubMed.
- O. M. Abd El-Raouf, E. M. El-Sayed and M. F. Manie, Cinnamic Acid and Cinnamaldehyde Ameliorate Cisplatin-Induced Splenotoxicity in Rats, J. Biochem. Mol. Toxicol., 2015, 29, 426–431 CrossRef CAS PubMed.
- A. M. Morgan, S. S. El-Ballal, B. E. El-Bialy and N. B. El-Borai, Studies on the potential protective effect of cinnamon against bisphenol A- and octylphenol-induced oxidative stress in male albino rats, Toxicol. Rep., 2014, 1, 92–101 CrossRef CAS PubMed.
- M. R. Farag, M. Alagawany and V. Tufarelli, In vitro antioxidant activities of resveratrol, cinnamaldehyde and their synergistic effect against cyadox-induced cytotoxicity in rabbit erythrocytes, Drug Chem. Toxicol., 2017, 40, 196–205 CrossRef CAS PubMed.
- A. Ghonim, A. Abdeen, R. El-Shawarby, N. Abdel-Aleem, E. El-Shewy, M. Abdo and E. Abdelhiee, Protective effect of cinnamon against cadmium-induced hepatorenal oxidative damage in rats, Int. J. Pharmacol. Toxicol., 2017, 5, 17–22 CrossRef.
- G. Taner, D. O. Vardar, S. Aydin, Z. Aytac, A. Basaran and N. Basaran, Use of in vitro assays to assess the potential cytotoxic, genotoxic and antigenotoxic effects of vanillic and cinnamic acid, Drug Chem. Toxicol., 2017, 40, 183–190 CrossRef CAS PubMed.
- J. W. Yun, J. R. You, Y. S. Kim, S. H. Kim, E. Y. Cho, J. H. Yoon, E. Kwon, J. J. Jang, J. S. Park, H. C. Kim, J. H. Che and B. C. Kang, In vitro and in vivo safety studies of cinnamon extract (Cinnamomum cassia) on general and genetic toxicology, Regul. Toxicol. Pharmacol, 2018, 95, 115–123 CrossRef CAS PubMed.
- M. Hajimonfarednejad, M. Ostovar, M. J. Raee, M. H. Hashempur, J. G. Mayer and M. Heydari, Cinnamon: A systematic review of adverse events, Clin. Nutr., 2019, 38, 594–602 CrossRef CAS PubMed.
- Y. Nir, I. Potasman, E. Stermer, M. Tabak and I. Neeman, Controlled trial of the effect of cinnamon extract on Helicobacter pylori, Helicobacter, 2000, 5, 94–97 CrossRef CAS PubMed.
- P. Crawford, Effectiveness of cinnamon for lowering hemoglobin A1C in patients with type 2 diabetes: a randomized, controlled trial, J. Am. Board Fam. Med., 2009, 22, 507–512 CrossRef PubMed.
- M. A. Nystoriak, P. J. Kilfoil, P. K. Lorkiewicz, B. Ramesh, P. J. Kuehl, J. McDonald, A. Bhatnagar and D. J. Conklin, Comparative effects of parent and heated cinnamaldehyde on the function of human iPSC-derived cardiac myocytes, Toxicol. in Vitro, 2019, 61, 104648 CrossRef CAS PubMed.
- D. H. Kort and R. A. Lobo, Preliminary evidence that cinnamon improves menstrual cyclicity in women with polycystic ovary syndrome: a randomized controlled trial, Am. J. Obstet. Gynecol., 2014, 211, 487 CrossRef PubMed.
- S. Walanj, A. Walanj, V. Mohan and P. A. Thakurdesai, Efficacy and safety of the topical use of intranasal cinnamon bark extract in seasonal allergic rhinitis patients: A double-blind placebo-controlled pilot study, J. Herb. Med., 2014, 4, 37–47 CrossRef.
- P. Libby, J. E. Buring, L. Badimon, G. K. Hansson, J. Deanfield, M. S. Bittencourt, L. Tokgozoglu and E. F. Lewis, Atherosclerosis, Nat. Rev. Dis. Primers, 2019, 5, 56 CrossRef PubMed.
- H. Lu and A. Daugherty, Atherosclerosis, Arterioscler., Thromb., Vasc. Biol., 2015, 35, 485–491 CrossRef CAS PubMed.
- J. Davignon and P. Ganz, Role of endothelial dysfunction in atherosclerosis, Circulation, 2004, 109, III27–III32 Search PubMed.
- D. A. Chistiakov, V. V. Revin, I. A. Sobenin, A. N. Orekhov and Y. V. Bobryshev, Vascular endothelium: functioning in norm, changes in atherosclerosis and current dietary approaches to improve endothelial function, Mini-Rev. Med. Chem., 2015, 15, 338–350 CrossRef CAS PubMed.
- W. K. Alderton, C. E. Cooper and R. G. Knowles, Nitric oxide synthases: structure, function and inhibition, Biochem. J., 2001, 357, 593–615 CrossRef CAS.
- M. Lind, A. Hayes, M. Caprnda, D. Petrovic, L. Rodrigo, P. Kruzliak and A. Zulli, Inducible nitric oxide synthase: Good or bad?, Biomed. Pharmacother., 2017, 93, 370–375 CrossRef CAS PubMed.
- P. Nyadjeu, A. Dongmo, T. B. Nguelefack and A. Kamanyi, Antihypertensive and vasorelaxant effects of Cinnamomum zeylanicum stem bark aqueous extract in rats, J. Complementary Integr. Med., 2011, 8, 2 Search PubMed.
- M. M. Tarkhan, K. S. Balamsh and H. M. El-Bassossy, Cinnamaldehyde protects from methylglyoxal-induced vascular damage: Effect on nitric oxide and advanced glycation end products, J. Food Biochem., 2019, 43, e12907 CrossRef PubMed.
- A. Yanaga, H. Goto, T. Nakagawa, H. Hikiami, N. Shibahara and Y. Shimada, Cinnamaldehyde induces endothelium-dependent and -independent vasorelaxant action on isolated rat aorta, Biol. Pharm. Bull., 2006, 29, 2415–2418 CrossRef CAS PubMed.
- H. C. Ou, F. P. Chou, T. M. Lin, C. H. Yang and W. H. Sheu, Protective effects of eugenol against oxidized LDL-induced cytotoxicity and adhesion molecule expression in endothelial cells, Food Chem. Toxicol., 2006, 44, 1485–1495 CrossRef CAS PubMed.
- F. Wang, C. Pu, P. Zhou, P. Wang, D. Liang, Q. Wang, Y. Hu, B. Li and X. Hao, Cinnamaldehyde prevents endothelial dysfunction induced by high glucose by activating Nrf2, Cell. Physiol. Biochem., 2015, 36, 315–324 CrossRef CAS PubMed.
- D. J. Rader and A. Daugherty, Translating molecular discoveries into new therapies for atherosclerosis, Nature, 2008, 451, 904–913 CrossRef CAS PubMed.
- K. Skalen, M. Gustafsson, E. K. Rydberg, L. M. Hulten, O. Wiklund, T. L. Innerarity and J. Boren, Subendothelial retention of atherogenic lipoproteins in early atherosclerosis, Nature, 2002, 417, 750–754 CrossRef CAS PubMed.
- J. R. Burnett, A. J. Hooper and R. A. Hegele, Lipids and cardiovascular disease, Pathology, 2019, 51, 129–130 CrossRef PubMed.
- E. M. Maguire, S. W. A. Pearce and Q. Xiao, Foam cell formation: A new target for fighting atherosclerosis and cardiovascular disease, Vasc. Pharmacol., 2019, 112, 54–71 CrossRef CAS PubMed.
- J. E. Feig, B. Hewing, J. D. Smith, S. L. Hazen and E. A. Fisher, High-density lipoprotein and atherosclerosis regression: evidence from preclinical and clinical studies, Circ. Res., 2014, 114, 205–213 CrossRef CAS PubMed.
- B. G. Nordestgaard and A. Varbo, Triglycerides and cardiovascular disease, Lancet, 2014, 384, 626–635 CrossRef CAS.
- B. G. Nordestgaard, Triglyceride-Rich Lipoproteins and Atherosclerotic Cardiovascular Disease: New Insights From Epidemiology, Genetics, and Biology, Circ. Res., 2016, 118, 547–563 CrossRef CAS PubMed.
- P. Azimi, R. Ghiasvand, A. Feizi, M. Hariri and B. Abbasi, Effects of Cinnamon, Cardamom, Saffron, and Ginger Consumption on Markers of Glycemic Control, Lipid Profile, Oxidative Stress, and Inflammation in Type 2 Diabetes Patients, Rev. Diabet. Stud., 2014, 11, 258–266 CrossRef PubMed.
- S. G. Jain, S. Puri, A. Misra, S. Gulati and K. Mani, Effect of oral cinnamon intervention on metabolic profile and body composition of Asian Indians with metabolic syndrome: a randomized double -blind control trial, Lipids Health Dis., 2017, 16, 113 CrossRef PubMed.
- F. Askari, B. Rashidkhani and A. Hekmatdoost, Cinnamon may have therapeutic benefits on lipid profile, liver enzymes, insulin resistance, and high-sensitivity C-reactive protein in nonalcoholic fatty liver disease patients, Nutr. Res., 2014, 34, 143–148 CrossRef CAS PubMed.
- O. Markey, C. M. McClean, P. Medlow, G. W. Davison, T. R. Trinick, E. Duly and A. Shafat, Effect of cinnamon on gastric emptying, arterial stiffness, postprandial lipemia, glycemia, and appetite responses to high-fat breakfast, Cardiovasc. Diabetol., 2011, 10, 78 CrossRef CAS PubMed.
- S. M. Blevins, M. J. Leyva, J. Brown, J. Wright, R. H. Scofield and C. E. Aston, Effect of cinnamon on glucose and lipid levels in non insulin-dependent type 2 diabetes, Diabetes Care, 2007, 30, 2236–2237 CrossRef PubMed.
- K. Mnafgui, A. Derbali, S. Sayadi, N. Gharsallah, A. Elfeki and N. Allouche, Anti-obesity and cardioprotective effects of cinnamic acid in high fat diet- induced obese rats, J. Food Sci. Technol., 2015, 52, 4369–4377 CrossRef CAS PubMed.
- I. N. Nayak, R. Chinta and R. Jetti, Anti-Atherosclerotic Potential of Aqueous Extract of Cinnamomum Zeylanicum Bark against Glucocorticoid Induced Atherosclerosis in Wistar Rats, J. Clin. Diagnostic Res., 2017, 11, FC19–FC23 CAS.
- B. H. Cheng, L. Y. Sheen and S. T. Chang, Hypolipidemic effects of S-(+)-linalool and essential oil from Cinnamomum osmophloeum ct. linalool leaves in mice, J. Tradit. Complement. Med., 2018, 8, 46–52 CrossRef PubMed.
- B. P. Lopes, T. G. Gaique, L. L. Souza, G. S. Paula, G. E. Kluck, G. C. Atella, A. C. Gomes, N. K. Simas, R. M. Kuster, T. M. Ortiga-Carvalho, C. C. Pazos-Moura and K. J. Oliveira, Cinnamon extract improves the body composition and attenuates lipogenic processes in the liver and adipose tissue of rats, Food Funct., 2015, 6, 3257–3265 RSC.
- N. H. Kopp, S. Mukherjee and J. W. Yun, Trans-Cinnamic Acid Stimulates White Fat Browning and Activates Brown Adipocytes, Nutrients, 2019, 11, 577 CrossRef PubMed.
- C. Kopp, S. P. Singh, P. Regenhard, U. Muller, H. Sauerwein and M. Mielenz, Trans-cinnamic acid increases adiponectin and the phosphorylation of AMP-activated protein kinase through G-protein-coupled receptor signaling in 3 T3-L1 adipocytes, Int. J. Mol. Sci., 2014, 15, 2906–2915 CrossRef PubMed.
- S. H. Kim and S. Y. Choung, Antihyperglycemic and antihyperlipidemic action of Cinnamomi Cassiae (Cinnamon bark) extract in C57BL/Ks db/db mice, Arch. Pharmacal Res., 2010, 33, 325–333 CrossRef PubMed.
- P. Khare, S. Jagtap, Y. Jain, R. K. Baboota, P. Mangal, R. K. Boparai, K. K. Bhutani, S. S. Sharma, L. S. Premkumar, K. K. Kondepudi, K. Chopra and M. Bishnoi, Cinnamaldehyde supplementation prevents fasting-induced hyperphagia, lipid accumulation, and inflammation in high-fat diet-fed mice, Biofactors, 2016, 42, 201–211 CrossRef CAS PubMed.
- B. Qin, M. M. Polansky and R. A. Anderson, Cinnamon extract regulates plasma levels of adipose-derived factors and expression of multiple genes related to carbohydrate metabolism and lipogenesis in adipose tissue of fructose-fed rats, Horm. Metab. Res., 2010, 42, 187–193 CrossRef CAS PubMed.
- A. Handberg, K. Levin, K. Hojlund and H. Beck-Nielsen, Identification of the oxidized low-density lipoprotein scavenger receptor CD36 in plasma: a novel marker of insulin resistance, Circulation, 2006, 114, 1169–1176 CrossRef CAS PubMed.
- M. M. Hussain, J. Shi and P. Dreizen, Microsomal triglyceride transfer protein and its role in apoB-lipoprotein assembly, J. Lipid Res., 2003, 44, 22–32 CrossRef CAS PubMed.
- B. Qin, M. M. Polansky, Y. Sato, K. Adeli and R. A. Anderson, Cinnamon extract inhibits the postprandial overproduction of apolipoprotein B48-containing lipoproteins in fructose-fed animals, J. Nutr. Biochem., 2009, 20, 901–908 CrossRef CAS PubMed.
- B. Qin, H. D. Dawson, N. W. Schoene, M. M. Polansky and R. A. Anderson, Cinnamon polyphenols regulate multiple metabolic pathways involved in insulin signaling and intestinal lipoprotein metabolism of small intestinal enterocytes, Nutrition, 2012, 28, 1172–1179 CrossRef CAS PubMed.
- N. Kaur, H. Chugh, V. Tomar, M. K. Sakharkar, S. K. Dass and R. Chandra, Cinnamon attenuates adiposity and affects the expression of metabolic genes in Diet-Induced obesity model of zebrafish, Artif. Cells, Nanomed., Biotechnol., 2019, 47, 2930–2939 CrossRef PubMed.
- A. R. Tall, Plasma cholesteryl ester transfer protein, J. Lipid Res., 1993, 34, 1255–1274 CrossRef CAS.
- S. Lee, J. M. Han, H. Kim, E. Kim, T. S. Jeong, W. S. Lee and K. H. Cho, Synthesis of cinnamic acid derivatives and their inhibitory effects on LDL-oxidation, acyl-CoA:cholesterol acyltransferase-1 and -2 activity, and decrease of HDL-particle size, Bioorg. Med. Chem. Lett., 2004, 14, 4677–4681 CrossRef CAS PubMed.
- Z. Tuzcu, C. Orhan, N. Sahin, V. Juturu and K. Sahin, Cinnamon Polyphenol Extract Inhibits Hyperlipidemia and Inflammation by Modulation of Transcription Factors in High-Fat Diet-Fed Rats, Oxid. Med. Cell. Longevity, 2017, 2017, 1583098 Search PubMed.
- C. Gorrini, I. S. Harris and T. W. Mak, Modulation of oxidative stress as an anticancer strategy, Nat. Rev. Drug Discovery, 2013, 12, 931–947 CrossRef CAS PubMed.
- U. Forstermann, N. Xia and H. Li, Roles of Vascular Oxidative Stress and Nitric Oxide in the Pathogenesis of Atherosclerosis, Circ. Res., 2017, 120, 713–735 CrossRef PubMed.
- J. S. Lee, S. M. Jeon, E. M. Park, T. L. Huh, O. S. Kwon, M. K. Lee and M. S. Choi, Cinnamate supplementation enhances hepatic lipid metabolism and antioxidant defense systems in high cholesterol-fed rats, J. Med. Food, 2003, 6, 183–191 CrossRef CAS PubMed.
- H. Zhao, M. Zhang, F. Zhou, W. Cao, L. Bi, Y. Xie, Q. Yang and S. Wang, Cinnamaldehyde ameliorates LPS-induced cardiac dysfunction via TLR4-NOX4 pathway: The regulation of autophagy and ROS production, J. Mol. Cell. Cardiol., 2016, 101, 11–24 CrossRef CAS PubMed.
- P. Wang, Y. Yang, D. Wang, Q. Yang, J. Wan, S. Liu, P. Zhou and Y. Yang, Cinnamaldehyde Ameliorates Vascular Dysfunction in Diabetic Mice by Activating Nrf2, Am. J. Hypertens., 2020, 33, 610–619 CrossRef CAS PubMed.
- J. Maher and M. Yamamoto, The rise of antioxidant signaling–the evolution and hormetic actions of Nrf2, Toxicol. Appl. Pharmacol., 2010, 244, 4–15 CrossRef CAS PubMed.
- M. C. Flynn, G. Pernes, M. K. S. Lee, P. R. Nagareddy and A. J. Murphy, Monocytes, Macrophages, and Metabolic Disease in Atherosclerosis, Front. Pharmacol., 2019, 10, 666 CrossRef CAS PubMed.
- D. Wang, Y. Yang, Y. Lei, N. T. Tzvetkov, X. Liu, A. W. K. Yeung, S. Xu and A. G. Atanasov, Targeting Foam Cell Formation in Atherosclerosis: Therapeutic Potential of Natural Products, Pharmacol. Rev., 2019, 71, 596–670 CrossRef CAS PubMed.
- V. V. Kunjathoor, M. Febbraio, E. A. Podrez, K. J. Moore, L. Andersson, S. Koehn, J. S. Rhee, R. Silverstein, H. F. Hoff and M. W. Freeman, Scavenger receptors class A-I/II and CD36 are the principal receptors responsible for the uptake of modified low density lipoprotein leading to lipid loading in macrophages, J. Biol. Chem., 2002, 277, 49982–49988 CrossRef CAS PubMed.
- H. Kang, S. H. Park, J. M. Yun, T. G. Nam, Y. E. Kim, D. O. Kim and Y. J. Kim, Effect of cinnamon water extract on monocyte-to-macrophage differentiation and scavenger receptor activity, BMC Complementary Altern. Med., 2014, 14, 90 CrossRef PubMed.
- B. H. Kim, Y. G. Lee, J. Lee, J. Y. Lee and J. Y. Cho, Regulatory effect of cinnamaldehyde on monocyte/macrophage-mediated inflammatory responses, Mediators Inflammation, 2010, 2010, 529359 Search PubMed.
- G. R. Geovanini and P. Libby, Atherosclerosis and inflammation: overview and updates, Clin. Sci., 2018, 132, 1243–1252 CrossRef CAS PubMed.
- A. Solanki, L. K. Bhatt and T. P. Johnston, Evolving targets for the treatment of atherosclerosis, Pharmacol. Ther., 2018, 187, 1–12 CrossRef CAS PubMed.
- D. Tousoulis, E. Oikonomou, E. K. Economou, F. Crea and J. C. Kaski, Inflammatory cytokines in atherosclerosis: current therapeutic approaches, Eur. Heart J., 2016, 37, 1723–1732 CrossRef CAS PubMed.
- F. Shishehbor, M. R. Safar, E. Rajaei and M. H. Haghighizadeh, Cinnamon Consumption Improves Clinical Symptoms and Inflammatory Markers in Women With Rheumatoid Arthritis, J. Am. Coll. Nutr., 2018, 1–6, DOI:10.1080/07315724.2018.1460733.
- M. Davari, R. Hashemi, P. Mirmiran, M. Hedayati, S. Sahranavard, S. Bahreini, R. Tavakoly and B. Talaei, Effects of cinnamon supplementation on expression of systemic inflammation factors, NF-kB and Sirtuin-1 (SIRT1) in type 2 diabetes: a randomized, double blind, and controlled clinical trial, Nutr. J., 2020, 19, 1 CrossRef CAS PubMed.
- P. Mirmiran, M. Davari, R. Hashemi, M. Hedayati, S. Sahranavard, S. Bahreini, R. Tavakoly and B. Talaei, A randomized controlled trial to determining the effect of cinnamon on the plasma levels of soluble forms of vascular adhesion molecules in type 2 diabetes mellitus, Eur. J. Clin. Nutr., 2019, 73, 1605–1612 CrossRef CAS PubMed.
- B. C. Liao, C. W. Hsieh, Y. C. Liu, T. T. Tzeng, Y. W. Sun and B. S. Wung, Cinnamaldehyde inhibits the tumor necrosis factor-alpha-induced expression of cell adhesion molecules in endothelial cells by suppressing NF-kappaB activation: effects upon IkappaB and Nrf2, Toxicol. Appl. Pharmacol., 2008, 229, 161–171 CrossRef CAS PubMed.
- S. C. Lee, S. Y. Wang, C. C. Li and C. T. Liu, Anti-inflammatory effect of cinnamaldehyde and linalool from the leaf essential oil of Cinnamomum osmophloeum Kanehira in endotoxin-induced mice, J. Food Drug Anal., 2018, 26, 211–220 CrossRef CAS PubMed.
- A. Schink, K. Naumoska, Z. Kitanovski, C. J. Kampf, J. Frohlich-Nowoisky, E. Thines, U. Poschl, D. Schuppan and K. Lucas, Anti-inflammatory effects of cinnamon extract and identification of active compounds influencing the TLR2 and TLR4 signaling pathways, Food Funct., 2018, 9, 5950–5964 RSC.
- C. Pannee, I. Chandhanee and L. Wacharee, Antiinflammatory effects of essential oil from the leaves of Cinnamomum cassia and cinnamaldehyde on lipopolysaccharide-stimulated J774A.1 cells, J. Adv. Pharm. Technol. Res., 2014, 5, 164–170 CrossRef PubMed.
- H. Cao, J. F. Urban Jr. and R. A. Anderson, Cinnamon polyphenol extract affects immune responses by regulating anti- and proinflammatory and glucose transporter gene expression in mouse macrophages, J. Nutr., 2008, 138, 833–840 CrossRef CAS PubMed.
- J. A. DiDonato, F. Mercurio and M. Karin, NF-kappaB and the link between inflammation and cancer, Immunol. Rev., 2012, 246, 379–400 CrossRef PubMed.
- S. H. Lee, S. Y. Lee, D. J. Son, H. Lee, H. S. Yoo, S. Song, K. W. Oh, D. C. Han, B. M. Kwon and J. T. Hong, Inhibitory effect of 2′-hydroxycinnamaldehyde on nitric oxide production through inhibition of NF-kappa B activation in RAW 264.7 cells, Biochem. Pharmacol., 2005, 69, 791–799 CrossRef CAS PubMed.
- J. C. Liao, J. S. Deng, C. S. Chiu, W. C. Hou, S. S. Huang, P. H. Shie and G. J. Huang, Anti-Inflammatory Activities of Cinnamomum cassia Constituents In Vitro and In Vivo, Evidence-Based Complementary Altern. Med., 2012, 2012, 429320 Search PubMed.
- W. Li, W. Zhi, J. Zhao, W. Li, L. Zang, F. Liu and X. Niu, Cinnamaldehyde attenuates atherosclerosis via targeting the IkappaB/NF-kappaB
signaling pathway in high fat diet-induced ApoE(-/-) mice, Food Funct., 2019, 10, 4001–4009 RSC.
- B. C. Liao, C. W. Hsieh, Y. C. Lin and B. S. Wung, The glutaredoxin/glutathione system modulates NF-kappaB activity by glutathionylation of p65 in cinnamaldehyde-treated endothelial cells, Toxicol. Sci., 2010, 116, 151–163 CrossRef CAS PubMed.
- J. W. Hong, G. E. Yang, Y. B. Kim, S. H. Eom, J. H. Lew and H. Kang, Anti-inflammatory activity of cinnamon water extract in vivo and in vitro LPS-induced models, BMC Complementary Altern. Med., 2012, 12, 237 CrossRef PubMed.
- M. R. Bennett, S. Sinha and G. K. Owens, Vascular Smooth Muscle Cells in Atherosclerosis, Circ. Res., 2016, 118, 692–702 CrossRef CAS PubMed.
- N. E. Buglak, W. Jiang and E. S. M. Bahnson, Cinnamic aldehyde inhibits vascular smooth muscle cell proliferation and neointimal hyperplasia in Zucker Diabetic Fatty rats, Redox Biol., 2018, 19, 166–178 CrossRef CAS PubMed.
- W. Li, W. Zhi, J. Zhao, Q. Yao, F. Liu and X. Niu, Cinnamaldehyde protects VSMCs against ox-LDL-induced proliferation and migration through S arrest and inhibition of p38, JNK/MAPKs and NF-kappaB, Vasc. Pharmacol., 2018, 108, 57–66 CrossRef CAS PubMed.
- L. Ouyang, K. Zhang, J. Chen, J. Wang and H. Huang, Roles of platelet-derived growth factor in vascular calcification, J. Cell. Physiol., 2018, 233, 2804–2814 CrossRef CAS PubMed.
- H. Kwon, J. J. Lee, J. H. Lee, W. K. Cho, M. J. Gu, K. J. Lee and J. Y. Ma, Cinnamon and its Components Suppress Vascular Smooth Muscle Cell Proliferation by Up-Regulating Cyclin-Dependent Kinase Inhibitors, Am. J. Chin. Med., 2015, 43, 621–636 CrossRef CAS PubMed.
- A. Sachinidis, R. Locher, W. Vetter, D. Tatje and J. Hoppe, Different effects of platelet-derived growth factor isoforms on rat vascular smooth muscle cells, J. Biol. Chem., 1990, 265, 10238–10243 CrossRef CAS.
- T. J. Kim and Y. P. Yun, Antiproliferative activity of NQ304, a synthetic 1,4-naphthoquinone, is mediated via the suppressions of the PI3 K/Akt and ERK1/2 signaling pathways in PDGF-BB-stimulated vascular smooth muscle cells, Vasc. Pharmacol., 2007, 46, 43–51 CrossRef CAS PubMed.
- Y. H. Jin and S. A. Kim, 2-Methoxycinnamaldehyde inhibits the TNF-alpha-induced proliferation and migration of human aortic smooth muscle cells, Int. J. Mol. Med., 2017, 39, 191–198 CrossRef CAS PubMed.
- J. Yeung, W. Li and M. Holinstat, Platelet Signaling and Disease: Targeted Therapy for Thrombosis and Other Related Diseases, Pharmacol. Rev., 2018, 70, 526–548 CrossRef CAS PubMed.
- J. Huang, S. Wang, X. Luo, Y. Xie and X. Shi, Cinnamaldehyde reduction of platelet aggregation and thrombosis in rodents, Thromb. Res., 2007, 119, 337–342 CrossRef CAS PubMed.
- H. Matsuda, R. Matsuda, S. Fukuda, H. Shiomoto and M. Kubo, Anti-thrombic actions of 70% methanolic extract and cinnamic aldehyde from cinnamomi cortex, Chem. Pharm. Bull., 1987, 35, 1275–1280 CrossRef CAS PubMed.
- S. Y. Kim, Y. K. Koo, J. Y. Koo, T. M. Ngoc, S. S. Kang, K. Bae, Y. S. Kim and H. S. Yun-Choi, Platelet anti-aggregation activities of compounds from Cinnamomum cassia, J. Med. Food, 2010, 13, 1069–1074 CrossRef CAS PubMed.
- S. M. Yu, T. S. Wu and C. M. Teng, Pharmacological characterization of cinnamophilin, a novel dual inhibitor of thromboxane synthase and thromboxane A2 receptor, Br. J. Pharmacol., 1994, 111, 906–912 CrossRef CAS PubMed.
- T. Sinnathamby, J. Yun, M. E. Clavet-Lanthier, C. Cheong and M. G. Sirois, VEGF and angiopoietins promote inflammatory cell recruitment and mature blood vessel formation in murine sponge/Matrigel model, J. Cell. Biochem., 2015, 116, 45–57 CrossRef CAS PubMed.
- H. L. Lin, L. Zhang, C. X. Liu, X. S. Xu, M. X. Tang, H. X. Lv, C. J. Li, H. W. Sun, M. Zhang, J. Hong and Y. Zhang, Haemin-enhanced expression of haem oxygenase-1 stabilizes erythrocyte-induced vulnerable atherosclerotic plaques, Br. J. Pharmacol., 2010, 160, 1484–1495 CrossRef PubMed.
- E. C. Kim, H. J. Kim and T. J. Kim, Water extract of Cinnamomum cassia suppresses angiogenesis through inhibition of VEGF receptor 2 phosphorylation, Biosci., Biotechnol., Biochem., 2015, 79, 617–624 CrossRef CAS PubMed.
- R. R. Bansode, T. Leung, P. Randolph, L. L. Williams and M. Ahmedna, Cinnamon extract inhibits angiogenesis in zebrafish and human endothelial cells by suppressing VEGFR1, VEGFR2, and PKC-mediated MAP kinase, Food Sci. Nutr., 2013, 1, 74–82 CrossRef CAS PubMed.
- X. Yuan, L. Han, P. Fu, H. Zeng, C. Lv, W. Chang, R. S. Runyon, M. Ishii, L. Han, K. Liu, T. Fan, W. Zhang and R. Liu, Cinnamaldehyde accelerates wound healing by promoting angiogenesis via up-regulation of PI3 K and MAPK signaling pathways, Lab. Invest., 2018, 98, 783–798 CrossRef CAS PubMed.
- R. Akilen, Z. Pimlott, A. Tsiami and N. Robinson, Effect of short-term administration of cinnamon on blood pressure in patients with prediabetes and type 2 diabetes, Nutrition, 2013, 29, 1192–1196 CrossRef CAS PubMed.
- T. N. Ziegenfuss, J. E. Hofheins, R. W. Mendel, J. Landis and R. A. Anderson, Effects of a water-soluble cinnamon extract on body composition and features of the metabolic syndrome in pre-diabetic men and women, J. Int. Soc. Sports Nutr., 2006, 3, 45–53 CrossRef PubMed.
- R. Akilen, A. Tsiami, D. Devendra and N. Robinson, Glycated haemoglobin and blood pressure-lowering effect of cinnamon in multi-ethnic Type 2 diabetic patients in the UK: a randomized, placebo-controlled, double-blind clinical trial, Diabet. Med., 2010, 27, 1159–1167 CrossRef CAS PubMed.
- J. Wainstein, N. Stern, S. Heller and M. Boaz, Dietary cinnamon supplementation and changes in systolic blood pressure in subjects with type 2 diabetes, J. Med. Food, 2011, 14, 1505–1510 CrossRef CAS PubMed.
- S. M. Mousavi, E. Karimi, M. Hajishafiee, A. Milajerdi, M. R. Amini and A. Esmaillzadeh, Anti-hypertensive effects of cinnamon supplementation in adults: A systematic review and dose-response Meta-analysis of randomized controlled trials, Crit. Rev. Food Sci. Nutr., 2020, 60, 3144–3154 CrossRef PubMed.
- H. G. Preuss, B. Echard, M. M. Polansky and R. Anderson, Whole cinnamon and aqueous extracts ameliorate sucrose-induced blood pressure elevations in spontaneously hypertensive rats, J. Am. Coll. Nutr., 2006, 25, 144–150 CrossRef CAS PubMed.
- P. Nyadjeu, E. P. Nguelefack-Mbuyo, A. D. Atsamo, T. B. Nguelefack, A. B. Dongmo and A. Kamanyi, Acute and chronic antihypertensive effects of Cinnamomum zeylanicum stem bark methanol extract in L-NAME-induced hypertensive rats, BMC Complementary Altern. Med., 2013, 13, 27 CrossRef PubMed.
- G. Raffai, B. Kim, S. Park, G. Khang, D. Lee and P. M. Vanhoutte, Cinnamaldehyde and cinnamaldehyde-containing micelles induce relaxation of isolated porcine coronary arteries: role of nitric oxide and calcium, Int. J. Nanomed., 2014, 9, 2557–2566 CrossRef PubMed.
- L. Zhang, L. Wu, Y. Pan, L. Qin, T. Liu, X. Liu, H. Yoshitomi, K. Ikeda, H. Negishi, M. Gao, J. Li and T. Xu, Trans-cinnamaldehyde promotes nitric oxide release via the protein kinase-B /v-Akt murine thymoma viral oncogene -endothelial nitric oxide synthase pathway to alleviate hypertension in SHR.Cg-Leprcp/NDmcr rats, J. Tradit. Chin. Med., 2018, 38, 548–555 CrossRef PubMed.
- K. Mnafgui, A. Derbali, S. Sayadi, N. Gharsallah, A. Elfeki and N. Allouche, Anti-obesity and cardioprotective effects of cinnamic acid in high fat diet- induced obese rats, J. Food Sci. Technol., 2015, 52, 4369–4377 CrossRef CAS PubMed.
- N. R. Tykocki, E. M. Boerman and W. F. Jackson, Smooth Muscle Ion Channels and Regulation
of Vascular Tone in Resistance Arteries and Arterioles, Compr. Physiol., 2017, 7, 485–581 Search PubMed.
- J. Alvarez-Collazo, L. Alonso-Carbajo, A. I. Lopez-Medina, Y. A. Alpizar, S. Tajada, B. Nilius, T. Voets, J. R. Lopez-Lopez, K. Talavera, M. T. Perez-Garcia and J. L. Alvarez, Cinnamaldehyde inhibits L-type calcium channels in mouse ventricular cardiomyocytes and vascular smooth muscle cells, Pflugers Arch., 2014, 466, 2089–2099 CrossRef CAS PubMed.
- Z. Wang, D. Ye, J. Ye, M. Wang, J. Liu, H. Jiang, Y. Xu, J. Zhang, J. Chen and J. Wan, The TRPA1 Channel in the Cardiovascular System: Promising Features and Challenges, Front. Pharmacol., 2019, 10, 1253 CrossRef CAS PubMed.
- G. Pozsgai, J. V. Bodkin, R. Graepel, S. Bevan, D. A. Andersson and S. D. Brain, Evidence for the pathophysiological relevance of TRPA1 receptors in the cardiovascular system in vivo, Cardiovasc. Res., 2010, 87, 760–768 CrossRef CAS PubMed.
- T. J. Guzik and R. M. Touyz, Oxidative Stress, Inflammation, and Vascular Aging in Hypertension, Hypertension, 2017, 70, 660–667 CrossRef CAS PubMed.
- A. Singh, S. A. Khan, R. Choudhary and S. H. Bodakhe, Cinnamaldehyde Attenuates Cataractogenesis via Restoration of Hypertension and Oxidative Stress in Fructose-Fed Hypertensive rats, J. Pharmacopuncture, 2016, 19, 137–144 CrossRef PubMed.
- G. W. Reed, J. E. Rossi and C. P. Cannon, Acute myocardial infarction, Lancet, 2017, 389, 197–210 CrossRef.
- D. J. Hausenloy and D. M. Yellon, Myocardial ischemia-reperfusion injury: a neglected therapeutic target, J. Clin. Invest., 2013, 123, 92–100 CrossRef CAS PubMed.
- M. Sedighi, A. Nazari, M. Faghihi, M. Rafieian-Kopaei, A. Karimi, M. Moghimian, S. A. Mozaffarpur, M. Rashidipour, M. Namdari, M. Cheraghi and B. Rasoulian, Protective effects of cinnamon bark extract against ischemia-reperfusion injury and arrhythmias in rat, Phytother. Res., 2018, 32, 1983–1991 CrossRef CAS PubMed.
- J. S. Hwa, Y. C. Jin, Y. S. Lee, Y. S. Ko, Y. M. Kim, L. Y. Shi, H. J. Kim, J. H. Lee, T. M. Ngoc, K. H. Bae, Y. S. Kim and K. C. Chang, 2-methoxycinnamaldehyde from Cinnamomum cassia reduces rat myocardial ischemia and reperfusion injury in vivo due to HO-1 induction, J. Ethnopharmacol., 2012, 139, 605–615 CrossRef CAS PubMed.
- S. Li, X. Sun, H. Wu, P. Yu, X. Wang, Z. Jiang, E. Gao, J. Chen, D. Li, C. Qiu, B. Song, K. Chen, K. He, D. Yang and Y. Yang, TRPA1 Promotes Cardiac Myofibroblast Transdifferentiation after Myocardial Infarction Injury via the Calcineurin-NFAT-DYRK1A Signaling Pathway, Oxid. Med. Cell. Longevity, 2019, 2019, 6408352 Search PubMed.
- A. Pollack, A. R. Kontorovich, V. Fuster and G. W. Dec, Viral myocarditis–diagnosis, treatment options, and current controversies, Nat. Rev. Cardiol., 2015, 12, 670–680 CrossRef PubMed.
- Y. Zhang, W. Cao, Y. H. Xie, Q. Yang, X. Q. Li, X. X. Liu and S. W. Wang, The comparison of alpha-bromo-4-chlorocinnamaldehyde and cinnamaldehyde on coxsackie virus B3-induced myocarditis and their mechanisms, Int. Immunopharmacol., 2012, 14, 107–113 CrossRef CAS PubMed.
- M. Gyongyosi, J. Winkler, I. Ramos, Q. T. Do, H. Firat, K. McDonald, A. Gonzalez, T. Thum, J. Diez, F. Jaisser, A. Pizard and F. Zannad, Myocardial fibrosis: biomedical research from bench to bedside, Eur. J. Heart Fail., 2017, 19, 177–191 CrossRef PubMed.
- L. L. Kang, D. M. Zhang, C. H. Ma, J. H. Zhang, K. K. Jia, J. H. Liu, R. Wang and L. D. Kong, Cinnamaldehyde and allopurinol reduce fructose-induced cardiac inflammation and fibrosis by attenuating CD36-mediated TLR4/6-IRAK4/1 signaling to suppress NLRP3 inflammasome activation, Sci. Rep., 2016, 6, 27460 CrossRef CAS PubMed.
- B. M. Leon and T. M. Maddox, Diabetes and cardiovascular disease: Epidemiology, biological mechanisms, treatment recommendations and future research, World J. Diabetes, 2015, 6, 1246–1258 CrossRef PubMed.
- K. E. Bornfeldt, Uncomplicating the Macrovascular Complications of Diabetes: The 2014 Edwin Bierman Award Lecture, Diabetes, 2015, 64, 2689–2697 CrossRef CAS PubMed.
- A. American Diabetes, Diagnosis and classification of diabetes mellitus, Diabetes Care, 2013, 36(Suppl 1), S67–S74 CrossRef PubMed.
- N. H. Cho, J. E. Shaw, S. Karuranga, Y. Huang, J. D. da Rocha Fernandes, A. W. Ohlrogge and B. Malanda, IDF Diabetes Atlas: Global estimates of diabetes prevalence for 2017 and projections for 2045, Diabetes Res. Clin. Pract., 2018, 138, 271–281 CrossRef CAS PubMed.
- N. Kleefstra, S. J. Logtenberg, K. H. Groenier and H. J. Bilo, Cinnamon in glycaemic control: systematic review and meta analysis, Clin. Nutr., 2012, 31, 776 CrossRef PubMed , author reply 777.
- Q. Jia, X. Liu, X. Wu, R. Wang, X. Hu, Y. Li and C. Huang, Hypoglycemic activity of a polyphenolic oligomer-rich extract of Cinnamomum parthenoxylon bark in normal and streptozotocin-induced diabetic rats, Phytomedicine, 2009, 16, 744–750 CrossRef CAS PubMed.
- R. W. Allen, E. Schwartzman, W. L. Baker, C. I. Coleman and O. J. Phung, Cinnamon use in type 2 diabetes: an updated systematic review and meta-analysis, Ann. Fam. Med., 2013, 11, 452–459 CrossRef PubMed.
- S. Gupta, S. B. Sharma, S. K. Bansal and K. M. Prabhu, Antihyperglycemic and hypolipidemic activity of aqueous extract of Cassia auriculata L. leaves in experimental diabetes, J. Ethnopharmacol., 2009, 123, 499–503 CrossRef PubMed.
- K. Vijayakumar, B. Prasanna, R. L. Rengarajan, A. Rathinam, S. Velayuthaprabhu and A. V. Anand, Anti-diabetic and hypolipidemic effects of Cinnamon cassia bark extracts: an in vitro, in vivo, and in silico approach, Arch. Physiol. Biochem., 2020, 1–11, DOI:10.1080/13813455.2020.1822415.
- S. Kirkham, R. Akilen, S. Sharma and A. Tsiami, The potential of cinnamon to reduce blood glucose levels in patients with type 2 diabetes and insulin resistance, Diabetes Obes. Metab., 2009, 11, 1100–1113 CrossRef CAS PubMed.
- K. Im, A. Issac, J. Nm, E. Ninan, B. Maliakel and R. Kuttan, Effects of the polyphenol content on the anti-diabetic activity of Cinnamomum zeylanicum extracts, Food Funct., 2014, 5, 2208–2220 RSC.
- J. Jitomir and D. S. Willoughby, Cassia cinnamon for the attenuation of glucose intolerance and insulin resistance resulting from sleep loss, J. Med. Food, 2009, 12, 467–472 CrossRef CAS PubMed.
- E. J. Verspohl, K. Bauer and E. Neddermann, Antidiabetic effect of Cinnamomum cassia and Cinnamomum zeylanicum in vivo and in vitro, Phytother. Res., 2005, 19, 203–206 CrossRef PubMed.
- K. Couturier, C. Batandier, M. Awada, I. Hininger-Favier, F. Canini, R. A. Anderson, X. Leverve and A. M. Roussel, Cinnamon improves insulin sensitivity and alters the body composition in an animal model of the metabolic syndrome, Arch. Biochem. Biophys., 2010, 501, 158–161 CrossRef CAS PubMed.
- R. I. Holt, H. L. Simpson and P. H. Sonksen, The role of the growth hormone-insulin-like growth factor axis in glucose homeostasis, Diabet. Med., 2003, 20, 3–15 CrossRef CAS PubMed.
- A. R. Saltiel and C. R. Kahn, Insulin signalling and the regulation of glucose and lipid metabolism, Nature, 2001, 414, 799–806 CrossRef CAS PubMed.
- K. A. A. Mohammed, H. M. S. Ahmed, H. A. Sharaf, A. A. El-Nekeety, S. H. Abdel-Aziem, F. M. Mehaya and M. A. Abdel-Wahhab, Encapsulation of cinnamon oil in whey protein counteracts the disturbances in biochemical parameters, gene expression, and histological picture of the liver and pancreas of diabetic rats, Environ. Sci. Pollut. Res. Int., 2020, 27, 2829–2843 CrossRef CAS PubMed.
- H. Cao, M. M. Polansky and R. A. Anderson, Cinnamon extract and polyphenols affect the expression of tristetraprolin, insulin receptor, and glucose transporter 4 in mouse 3 T3-L1 adipocytes, Arch. Biochem. Biophys., 2007, 459, 214–222 CrossRef CAS PubMed.
- C. Cardillo, S. S. Nambi, C. M. Kilcoyne, W. K. Choucair, A. Katz, M. J. Quon and J. A. Panza, Insulin stimulates both endothelin and nitric oxide activity in the human forearm, Circulation, 1999, 100, 820–825 CrossRef CAS PubMed.
- J. R. Petrie, S. Ueda, D. J. Webb, H. L. Elliott and J. M. Connell, Endothelial nitric oxide production and insulin sensitivity. A physiological link with implications for pathogenesis of cardiovascular disease, Circulation, 1996, 93, 1331–1333 CrossRef CAS PubMed.
- M. Montagnani, L. V. Ravichandran, H. Chen, D. L. Esposito and M. J. Quon, Insulin receptor substrate-1 and phosphoinositide-dependent kinase-1 are required for insulin-stimulated production of nitric oxide in endothelial cells, Mol. Endocrinol., 2002, 16, 1931–1942 CrossRef CAS PubMed.
- G. Zeng, F. H. Nystrom, L. V. Ravichandran, L. N. Cong, M. Kirby, H. Mostowski and M. J. Quon, Roles for insulin receptor, PI3-kinase, and Akt in insulin-signaling pathways related to production of nitric oxide in human vascular endothelial cells, Circulation, 2000, 101, 1539–1545 CrossRef CAS PubMed.
- B. Qin, M. Nagasaki, M. Ren, G. Bajotto, Y. Oshida and Y. Sato, Cinnamon extract prevents the insulin resistance induced by a high-fructose diet, Horm. Metab. Res., 2004, 36, 119–125 CrossRef CAS PubMed.
- L. Sylow, M. Kleinert, C. Pehmoller, C. Prats, T. T. Chiu, A. Klip, E. A. Richter and T. E. Jensen, Akt and Rac1 signaling are jointly required for insulin-stimulated glucose uptake in skeletal muscle and downregulated in insulin resistance, Cell. Signal., 2014, 26, 323–331 CrossRef CAS PubMed.
- M. E. Abdelmageed, G. S. Shehatou, R. A. Abdelsalam, G. M. Suddek and H. A. Salem, Cinnamaldehyde ameliorates STZ-induced rat diabetes through modulation of IRS1/PI3 K/AKT2 pathway and AGEs/RAGE interaction, Naunyn Schmiedebergs Arch. Pharmacol., 2019, 392, 243–258 CrossRef CAS PubMed.
- J. E. Li, K. Futawaka, H. Yamamoto, M. Kasahara, T. Tagami, T. H. Liu and K. Moriyama, Cinnamaldehyde Contributes to Insulin Sensitivity by Activating PPARdelta, PPARgamma, and RXR, Am. J. Chin. Med., 2015, 43, 879–892 CrossRef CAS PubMed.
- R. M. Hafizur, A. Hameed, M. Shukrana, S. A. Raza, S. Chishti, N. Kabir and R. A. Siddiqui, Cinnamic acid exerts anti-diabetic activity by improving glucose tolerance in vivo and by stimulating insulin secretion in vitro, Phytomedicine, 2015, 22, 297–300 CrossRef CAS PubMed.
- H. Cao, D. J. Graves and R. A. Anderson, Cinnamon extract regulates glucose transporter and insulin-signaling gene expression in mouse adipocytes, Phytomedicine, 2010, 17, 1027–1032 CrossRef CAS PubMed.
- C. Kopp, S. P. Singh, P. Regenhard, U. Muller, H. Sauerwein and M. Mielenz, Trans-cinnamic acid increases adiponectin and the phosphorylation of AMP-activated protein kinase through G-protein-coupled receptor signaling in 3 T3-L1 adipocytes, Int. J. Mol. Sci., 2014, 15, 2906–2915 CrossRef PubMed.
- S. Camacho, S. Michlig, C. de Senarclens-Bezencon, J. Meylan, J. Meystre, M. Pezzoli, H. Markram and J. le Coutre, Anti-obesity and anti-hyperglycemic effects of cinnamaldehyde via altered ghrelin secretion and functional impact on food intake and gastric emptying, Sci. Rep., 2015, 5, 7919 CrossRef CAS PubMed.
- D. Liu, H. Gao, W. Tang and S. Nie, Plant non-starch polysaccharides that inhibit key enzymes linked to type 2 diabetes mellitus, Ann. N. Y. Acad. Sci., 2017, 1401, 28–36 CrossRef CAS PubMed.
- S. H. Kim, S. H. Hyun and S. Y. Choung, Anti-diabetic effect of cinnamon extract on blood glucose in db/db mice, J. Ethnopharmacol., 2006, 104, 119–123 CrossRef PubMed.
- H. M. S. Shihabudeen, D. H. Priscilla and K. Thirumurugan, Cinnamon extract inhibits alpha-glucosidase activity and dampens postprandial glucose excursion in diabetic rats, Nutr. Metab., 2011, 8, 46 CrossRef PubMed.
- S. Adisakwattana, O. Lerdsuwankij, U. Poputtachai, A. Minipun and C. Suparpprom, Inhibitory activity of cinnamon bark species and their combination effect with acarbose against intestinal alpha-glucosidase and pancreatic alpha-amylase, Plant Foods Hum. Nutr., 2011, 66, 143–148 CrossRef CAS PubMed.
- P. Ercan and S. N. El, Inhibitory effects of bioaccessible anthocyanins and procyanidins from apple, red grape, cinnamon on alpha-amylase, alpha-glucosidase and lipase, Int. J. Vitam. Nutr. Res., 2021, 91, 16–24 CrossRef CAS PubMed.
- D. M. Cheng, P. Kuhn, A. Poulev, L. E. Rojo, M. A. Lila and I. Raskin, In vivo and in vitro antidiabetic effects of aqueous cinnamon extract and cinnamon polyphenol-enhanced food matrix, Food Chem., 2012, 135, 2994–3002 CrossRef CAS PubMed.
- T. Sartorius, A. Peter, N. Schulz, A. Drescher, I. Bergheim, J. Machann, F. Schick, D. Siegel-Axel, A. Schurmann, C. Weigert, H. U. Haring and A. M. Hennige, Cinnamon extract improves insulin sensitivity in the brain and lowers liver fat in mouse models of obesity, PLoS One, 2014, 9, e92358 CrossRef PubMed.
- G. W. Gould, H. M. Thomas, T. J. Jess and G. I. Bell, Expression of human glucose transporters in Xenopus oocytes: kinetic characterization and substrate specificities of the erythrocyte, liver, and brain isoforms, Biochemistry, 1991, 30, 5139–5145 CrossRef CAS PubMed.
- K. Tobe, R. Suzuki, M. Aoyama, T. Yamauchi, J. Kamon, N. Kubota, Y. Terauchi, J. Matsui, Y. Akanuma, S. Kimura, J. Tanaka, M. Abe, J. Ohsumi, R. Nagai and T. Kadowaki, Increased expression of the sterol regulatory element-binding protein-1 gene in insulin receptor substrate-2(-/-) mouse liver, J. Biol. Chem., 2001, 276, 38337–38340 CrossRef CAS PubMed.
- X. Xia, J. Yan, Y. Shen, K. Tang, J. Yin, Y. Zhang, D. Yang, H. Liang, J. Ye and J. Weng, Berberine improves glucose metabolism in diabetic rats by inhibition of hepatic gluconeogenesis, PLoS One, 2011, 6, e16556 CrossRef CAS PubMed.
- M. Legan, S. Tevzic, A. Tolar, B. Luzar and V. F. Marolt, Glucose transporter-1 (GLUT-1) immunoreactivity in benign, premalignant and malignant lesions of the gallbladder, Pathol. Oncol. Res., 2011, 17, 61–66 CrossRef CAS PubMed.
- P. Anand, K. Y. Murali, V. Tandon, P. S. Murthy and R. Chandra, Insulinotropic effect of cinnamaldehyde on transcriptional regulation of pyruvate kinase, phosphoenolpyruvate carboxykinase, and GLUT4 translocation in experimental diabetic rats, Chem. Biol. Interact., 2010, 186, 72–81 CrossRef CAS PubMed.
- Y. Shen, M. Fukushima, Y. Ito, E. Muraki, T. Hosono, T. Seki and T. Ariga, Verification of the antidiabetic effects of cinnamon (Cinnamomum zeylanicum) using insulin-uncontrolled type 1 diabetic rats and cultured adipocytes, Biosci., Biotechnol., Biochem., 2010, 74, 2418–2425 CrossRef CAS PubMed.
- Q. Yang, T. E. Graham, N. Mody, F. Preitner, O. D. Peroni, J. M. Zabolotny, K. Kotani, L. Quadro and B. B. Kahn, Serum retinol binding protein 4 contributes to insulin resistance in obesity and type 2 diabetes, Nature, 2005, 436, 356–362 CrossRef CAS PubMed.
- T. E. Graham, Q. Yang, M. Bluher, A. Hammarstedt, T. P. Ciaraldi, R. R. Henry, C. J. Wason, A. Oberbach, P. A. Jansson, U. Smith and B. B. Kahn, Retinol-binding protein 4 and insulin resistance in lean, obese, and diabetic subjects, N. Engl. J. Med., 2006, 354, 2552–2563 CrossRef CAS PubMed.
- W. Zhang, Y. C. Xu, F. J. Guo, Y. Meng and M. L. Li, Anti-diabetic effects of cinnamaldehyde and berberine and their impacts on retinol-binding protein 4 expression in rats with type 2 diabetes mellitus, Chin. Med. J., 2008, 121, 2124–2128 CrossRef CAS PubMed.
- B. Roffey, A. Atwal and S. Kubow, Cinnamon water extracts increase glucose uptake but inhibit adiponectin secretion in 3 T3-L1 adipose cells, Mol. Nutr. Food Res., 2006, 50, 739–745 CrossRef CAS PubMed.
- J. Li, T. Liu, L. Wang, X. Guo, T. Xu, L. Wu, L. Qin and W. Sun, Antihyperglycemic and antihyperlipidemic action of cinnamaldehyde in C57BLKS/J db/db mice, J. Tradit. Chin. Med., 2012, 32, 446–452 CrossRef PubMed.
- Y. Shen, N. Honma, K. Kobayashi, L. N. Jia, T. Hosono, K. Shindo, T. Ariga and T. Seki, Cinnamon extract enhances glucose uptake in 3 T3-L1 adipocytes and C2C12 myocytes by inducing LKB1-AMP-activated protein kinase signaling, PLoS One, 2014, 9, e87894 CrossRef PubMed.
- S. Qusti, H. A. El Rabey and S. A. Balashram, The Hypoglycemic and Antioxidant Activity of Cress Seed and Cinnamon on Streptozotocin Induced Diabetes in Male Rats, Evidence-Based Complementary Altern. Med., 2016, 2016, 5614564 CrossRef PubMed.
- R. P. Robertson and J. S. Harmon, Pancreatic islet beta-cell and oxidative stress: the importance of glutathione peroxidase, FEBS Lett., 2007, 581, 3743–3748 CrossRef CAS PubMed.
- F. Hu, F. Guo, Y. Zhu, Q. Zhou, T. Li, H. Xiang and D. Shang, IL-17 in pancreatic disease: pathogenesis and pharmacotherapy, Am. J. Cancer Res., 2020, 10, 3551–3564 CAS.
- P. Drikvandi, S. Bahramikia and M. Alirezaei, Modulation of the antioxidant defense system in liver, kidney, and pancreas tissues of alloxan-induced diabetic rats by camphor, J. Food Biochem., 2020, 44, e13527 CrossRef CAS PubMed.
- S. Kumar, N. Vasudeva and S. Sharma, GC-MS analysis and screening of antidiabetic, antioxidant and hypolipidemic potential of Cinnamomum tamala oil in streptozotocin induced diabetes mellitus in rats, Cardiovasc. Diabetol., 2012, 11, 95 CrossRef CAS PubMed.
- P. Sun, T. Wang, L. Chen, B. W. Yu, Q. Jia, K. X. Chen, H. M. Fan, Y. M. Li and H. Y. Wang, Trimer procyanidin oligomers contribute to the protective effects of cinnamon extracts on pancreatic beta-cells in vitro, Acta Pharmacol. Sin., 2016, 37, 1083–1090 CrossRef CAS PubMed.
- A. A. Hosni, A. A. Abdel-Moneim, E. S. Abdel-Reheim, S. M. Mohamed and H. Helmy, Cinnamaldehyde potentially attenuates gestational hyperglycemia in rats through modulation of PPARgamma, proinflammatory cytokines and oxidative stress, Biomed. Pharmacother., 2017, 88, 52–60 CrossRef CAS PubMed.
- S. C. Lee, W. X. Xu, L. Y. Lin, J. J. Yang and C. T. Liu, Chemical composition and hypoglycemic and pancreas-protective effect of leaf essential oil from indigenous cinnamon (Cinnamomum osmophloeum Kanehira), J. Agric. Food Chem., 2013, 61, 4905–4913 CrossRef CAS PubMed.
- J. A. Hebda and A. D. Miranker, The interplay of catalysis and toxicity by amyloid intermediates on lipid bilayers: insights from type II diabetes, Annu. Rev. Biophys., 2009, 38, 125–152 CrossRef CAS PubMed.
- R. A. Ritzel and P. C. Butler, Replication increases beta-cell vulnerability to human islet amyloid polypeptide-induced apoptosis, Diabetes, 2003, 52, 1701–1708 CrossRef CAS PubMed.
- L. Jiao, X. Zhang, L. Huang, H. Gong, B. Cheng, Y. Sun, Y. Li, Q. Liu, L. Zheng and K. Huang, Proanthocyanidins
are the major anti-diabetic components of cinnamon water extract, Food Chem. Toxicol., 2013, 56, 398–405 CrossRef CAS PubMed.
- H. Ping, G. Zhang and G. Ren, Antidiabetic effects of cinnamon oil in diabetic KK-Ay mice, Food Chem. Toxicol., 2010, 48, 2344–2349 CrossRef CAS PubMed.
- M. Van Hul, L. Geurts, H. Plovier, C. Druart, A. Everard, M. Stahlman, M. Rhimi, K. Chira, P. L. Teissedre, N. M. Delzenne, E. Maguin, A. Guilbot, A. Brochot, P. Gerard, F. Backhed and P. D. Cani, Reduced obesity, diabetes, and steatosis upon cinnamon and grape pomace are associated with changes in gut microbiota and markers of gut barrier, Am. J. Physiol. Endocrinol. Metab., 2018, 314, E334–E352 CrossRef CAS PubMed.
- H. Zhao, H. Wu, M. Duan, R. Liu, Q. Zhu, K. Zhang and L. Wang, Cinnamaldehyde Improves Metabolic Functions in Streptozotocin-Induced Diabetic Mice by Regulating Gut Microbiota, Drug Des. Devel. Ther., 2021, 15, 2339–2355 CrossRef PubMed.
- R. Singh, A. Barden, T. Mori and L. Beilin, Advanced glycation end-products: a review, Diabetologia, 2001, 44, 129–146 CrossRef CAS PubMed.
- N. J. Hayward, G. J. McDougall, S. Farag, J. W. Allwood, C. Austin, F. Campbell, G. Horgan and V. Ranawana, Cinnamon Shows Antidiabetic Properties that Are Species-Specific: Effects on Enzyme Activity Inhibition and Starch Digestion, Plant Foods Hum. Nutr., 2019, 74, 544–552 CrossRef CAS PubMed.
- P. Muthenna, G. Raghu, P. A. Kumar, M. V. Surekha and G. B. Reddy, Effect of cinnamon and its procyanidin-B2 enriched fraction on diabetic nephropathy in rats, Chem. Biol. Interact., 2014, 222, 68–76 CrossRef CAS PubMed.
- P. Muthenna, G. Raghu, C. Akileshwari, S. N. Sinha, P. Suryanarayana and G. B. Reddy, Inhibition of protein glycation by procyanidin-B2 enriched fraction of cinnamon: delay of diabetic cataract in rats, IUBMB Life, 2013, 65, 941–950 CrossRef CAS PubMed.
- H. Zheng, S. A. Whitman, W. Wu, G. T. Wondrak, P. K. Wong, D. Fang and D. D. Zhang, Therapeutic potential of Nrf2 activators in streptozotocin-induced diabetic nephropathy, Diabetes, 2011, 60, 3055–3066 CrossRef CAS PubMed.
- Y. M. Yan, P. Fang, M. T. Yang, N. Li, Q. Lu and Y. X. Cheng, Anti-diabetic nephropathy compounds from Cinnamomum cassia, J. Ethnopharmacol., 2015, 165, 141–147 CrossRef CAS PubMed.
- A. Mishra, R. Bhatti, A. Singh and M. P. S. Ishar, Ameliorative effect of the cinnamon oil from Cinnamomum zeylanicum upon early stage diabetic nephropathy, Planta Med., 2010, 76, 412–417 CrossRef CAS PubMed.
- H. M. El-Bassossy, A. Fahmy and D. Badawy, Cinnamaldehyde protects from the hypertension associated with diabetes, Food Chem. Toxicol., 2011, 49, 3007–3012 CrossRef CAS PubMed.
- A. Jawale, A. K. Datusalia, M. Bishnoi and S. S. Sharma, Reversal of diabetes-induced behavioral and neurochemical deficits by cinnamaldehyde, Phytomedicine, 2016, 23, 923–930 CrossRef CAS PubMed.
- A. A. Hemmati, S. Alboghobeish and A. Ahangarpour, Effects of cinnamic acid on memory deficits and brain oxidative stress in streptozotocin-induced diabetic mice, Korean J. Physiol. Pharmacol., 2018, 22, 257–267 CrossRef CAS PubMed.
- C. Sampath, J. C. Sprouse, M. L. Freeman and P. R. Gangula, Activation of Nrf2 attenuates delayed gastric emptying in obesity induced diabetic (T2DM) female mice, Free Radicals Biol. Med., 2019, 135, 132–143 CrossRef CAS PubMed.
- A. Daemi, M. Lotfi, M. R. Farahpour, A. Oryan, S. J. Ghayour and A. Sonboli, Topical application of Cinnamomum hydroethanolic extract improves wound healing by enhancing re-epithelialization and keratin biosynthesis in streptozotocin-induced diabetic mice, Pharm. Biol., 2019, 57, 799–806 CrossRef CAS PubMed.
- R. Zare, A. Nadjarzadeh, M. M. Zarshenas, M. Shams and M. Heydari, Efficacy of cinnamon in patients with type II diabetes mellitus: A randomized controlled clinical trial, Clin. Nutr., 2019, 38, 549–556 CrossRef CAS PubMed.
- B. Mang, M. Wolters, B. Schmitt, K. Kelb, R. Lichtinghagen, D. O. Stichtenoth and A. Hahn, Effects of a cinnamon extract on plasma glucose, HbA, and serum lipids in diabetes mellitus type 2, Eur. J. Clin. Invest., 2006, 36, 340–344 CrossRef CAS PubMed.
- T. Lu, H. Sheng, J. Wu, Y. Cheng, J. Zhu and Y. Chen, Cinnamon extract improves fasting blood glucose and glycosylated hemoglobin level in Chinese patients with type 2 diabetes, Nutr. Res., 2012, 32, 408–412 CrossRef CAS PubMed.
- J. C. G. L. Neto, M. M. C. Damasceno, M. A. Ciol, R. de Freitas, M. F. M. de Araujo, C. R. S. Teixeira, G. C. N. Carvalho, K. Lisboa, R. L. L. Marques, A. Alencar and M. L. Zanetti, Efficacy of Cinnamon as an Adjuvant in Reducing the Glycemic Biomarkers of Type 2 Diabetes Mellitus: A Three-Month, Randomized, Triple-Blind, Placebo-Controlled Clinical Trial, J. Am. Coll. Nutr., 2021, 1–9, DOI:10.1080/07315724.2021.1878967.
- M. J. Leach and S. Kumar, Cinnamon for diabetes mellitus, Cochrane Database Syst. Rev., 2012,(9), CD007170 Search PubMed.
- J. A. Altschuler, S. J. Casella, T. A. MacKenzie and K. M. Curtis, The effect of cinnamon on A1C among adolescents with type 1 diabetes, Diabetes Care, 2007, 30, 813–816 CrossRef CAS PubMed.
- B. Talaei, A. Amouzegar, S. Sahranavard, M. Hedayati, P. Mirmiran and F. Azizi, Effects of Cinnamon Consumption on Glycemic Indicators, Advanced Glycation End Products, and Antioxidant Status in Type 2 Diabetic Patients, Nutrients, 2017, 9, 991 CrossRef PubMed.
- M. Dorri, S. Hashemitabar and H. Hosseinzadeh, Cinnamon (Cinnamomum zeylanicum) as an antidote or a protective agent against natural or chemical toxicities: a review, Drug Chem. Toxicol., 2018, 41, 338–351 CrossRef CAS PubMed.
Footnotes |
† Electronic supplementary information (ESI) available. See DOI: 10.1039/d1fo01935j |
‡ First author. |
§ Co-first author. |
|
This journal is © The Royal Society of Chemistry 2021 |