DOI:
10.1039/D0FO02605K
(Paper)
Food Funct., 2021,
12, 881-891
Pectin limits epithelial barrier disruption by Citrobacter rodentium through anti-microbial effects
Received
5th October 2020
, Accepted 17th November 2020
First published on 7th January 2021
Abstract
Scope: C. rodentium is the murine equivalent of Enteropathogenic Escherichia. coli (EPEC) and Enterohemorrhagic Escherichia coli (EHEC) which induce damage to the intestinal epithelial barrier that results in diarrhea and intestinal inflammation. Dietary fibre intake can be an effective approach to limit epithelial damage by these enteric pathogens. Therefore, the protective effect of dietary fibre pectin against dysfunction of epithelial barrier integrity upon C. rodentium infection was investigated. Methods and results: Pectins that structurally differed in the degree and distribution of methylesters were tested on barrier protective effects on epithelial cells against C. rodentium by measuring transepithelial electrical resistance and lucifer yellow fluxes. All three pectins protected the epithelial barrier from C. rodentium induced damage in a structure-independent manner. These barrier protective effects were also independent of pectin-induced TLR2 activation. Furthermore, the pectins induced anti-adhesive effects on C. rodentium by interacting with C. rodentium and not with epithelial cells. This may be explained by antimicrobial effects of pectins on C. rodentium and not on other enteric bacteria including Lactobacillus plantarum and E. coli. A competition ELISA for binding of C. rodentium to pectin supported this finding as it showed that pectin interacts strongly with C. rodentium, whereas it interacts weakly or not with L. plantarum or E. coli. Conclusion: These findings demonstrate that pectin protects the epithelial barrier from C. rodentium induced damage by inducing anti-microbial effects.
Introduction
Enteropathogenic Escherichia. coli (EPEC) and Enterohemorrhagic Escherichia coli (EHEC) are enteric pathogens that are an important cause of food poisoning in developing countries which results in watery diarrhea.1Citrobacter rodentium is the murine equivalent of EPEC and EHEC and is used to model human infections with EPEC and EHEC.2 EPEC, EHEC, and C. rodentium disrupt intestinal epithelium by the formation of attaching and effacing (A/E) lesions. An intestinal infection by EPEC, EHEC, or C. rodentium leads to a dysfunctional intestinal barrier which results in diarrhea and intestinal inflammation.3 Therapeutic approaches to preserve the epithelial barrier from damage by EPEC, EHEC, or C. rodentium are required to reduce the development of diarrhea and intestinal inflammation.
Dietary fibre intake can be an effective therapeutic approach to limit EPEC, EHEC, or C. rodentium-induced epithelial damage and intestinal inflammation. Previously it has been demonstrated that fibre-deprived diets enhance the susceptibility to C. rodentium.4 Fibres enriched diets may enhance the growth of commensals that outcompete C. rodentium in the gastrointestinal tract.5 Furthermore, the protective effects of dietary fibres against C. rodentium can also relate to the fermentation of dietary fibres by the intestinal microbiota that results in the production of key secondary metabolites with anti-inflammatory properties, such as short-chain fatty acids (SCFAs).6,7 SCFAs are used as an energy source for intestinal epithelial cells and have a wide range of immune modulatory-functions, including augmentation of regulatory T cell development.8 as well as suppression of phagocytic cell activations,9,10 which results in maintenance of epithelial barrier integrity and alleviation of intestinal inflammation.11 In addition to the microbiota-dependent stimulation of the epithelial barrier, several studies also demonstrated that dietary fibres directly stimulate the epithelial barrier in microbiota-independent ways.12,13 Direct in vitro stimulation of the epithelium by dietary fibres enhanced epithelial integrity and protected against epithelial disrupting agents.13 Thus, direct stimulation of the epithelial barrier by dietary fibres may, therefore, be an effective therapeutic approach to protect the epithelium from EPEC, EHEC or C. rodentium induced damage.
Pectin is a dietary fibre that is known to enhance the epithelial barrier through direct interaction with epithelial cells.12,13 Pectin is a complex polysaccharide that is universally included in the middle lamella in terrestrial plants.14 Natural pectin in fruits and vegetables acts as soluble dietary fibre, and exerts prebiotic effects that modulate host cell physiology.15 Pectin mainly consists of a linear homopolymer of α-1,4-linked-D-galacturonic acid, which forms the backbone of the pectin molecule. The pectin backbone is partly esterified with methyl esters.16 The degree of methyl esterification (DM) of pectin differs between plant species and influences fermentation in the cecum, SCFA production, and anti-inflammatory activity.17,18 Besides, the galacturonic acid residues in the pectin backbone can be covalently linked to the rhamnogalacturonan (RG)-I and RG-II regions, both of which contain side-chain regions in the pectin molecule.19 These specific pectin structures are known to enhance the intestinal immune barrier.20 How specific pectin structures protect against C. rodentium induced epithelial damage through microbiota independent ways is still unknown.
Recently it was shown in vitro that pectin with a specific DM protect the epithelial barrier against damaging agents through direct stimulation of epithelial cells.13 Since pectins are known to stimulate the epithelial barrier through microbiota-independent ways, we hypothesize that pectin exerts protective effects against C. rodentium infection by direct effects on epithelial cells and in a structural dependent manner. In the present study, we investigated in vitro whether specific pectin structures protect against dysfunction of epithelial barrier integrity upon C. rodentium infection, and explored possible mechanisms that regulate protection against the C. rodentium infection. Structurally different pectins that varied in the degree and distribution of methyl esters were used in the current study.
Materials and methods
Pectin samples
Commercial extracted pectins from orange origin (DM32, DM59 and DM64) were obtained from DSM Andre pectin (Delft, The Netherlands). Pectins were extracted from the pulp of ripe oranges that were left over after fruit juice production. Molecular weight was determined by high performance size exclusion chromatography, monosaccharide content was determined by gas–liquid chromatography21 and the DM and DB was determined by gas chromatographic method as previously described.22 Polygalacturonic acid was derived from MP Biomedical (Santa Ana, CA, USA). RG-I pectins were previously characterized.23
Reporter cell assays
To study whether pectins can activate mouse TLR2, an activation assay was performed with pectins using HEK-Blue™ reporter cells expressing mouse TLR2 (Invivogen). The HEK-Blue™ mTLR2 cells were seeded in a 96 well plate in a volume of 180 μl at 2.8 × 105 cells per ml. DMEM medium was replaced after 24 hours of incubation with 180 μl medium containing DM32, DM59, or DM64 pectins in a concentration of 0.5, 1.0 or 2.0 mg ml−1. Culture medium as used as negative control and TLR2 agonist heat-killed Listeria monocytogenes (HKLM) was used as positive control in a concentration of 107 cells per ml. After 24 hours of incubation, TLR activation was quantified by adding 20 μl of cell supernatant to 180 μl of QUANTIBLUE. After 1 hour incubation at 37 °C, NF-κB activation was quantified at 650 nm using a Versa Max ELISA plate reader (Molecular Devices, Sunnyvale, CA, USA). TLR activation was represented as fold-change compared to the negative control. Each experiment was performed at least six times.
Cell culturing
Mouse rectal epithelial CMT93 cells (American Type Tissue Culture, Manassas, USA) were cultured in DMEM (Lonza, Basel, Switzerland) containing 10% de-complemented fetal calf serum (Sigma Aldrich, Zwijnsdrecht, the Netherlands), 50 U ml−1 penicillin (Sigma Aldrich) and 50 μg ml−1 streptomycin (Sigma Aldrich). After two passages and during all experiments, the cells were cultured in infection medium consisting of normal culture medium without penicillin and streptomycin. The cells were cultured at 37 °C in a humidified environment containing 5% CO2/95% O2. The medium was replaced trice a week and the cells were passaged at a 85% confluency after trypsinization. Cells were cultured between passage 10 and 18.
C. rodentium bacterial solution
Citrobacter rodentium DBS-100 (American Type Tissue Culture, Manassas, USA) was cultured overnight in Lysogeny broth (LB; Sigma) at 37 °C in a humidified environment containing 5% CO2/95% O2. The next day, the bacteria were resuspended 1
:
5 in LB and grown to mid-log phase (OD600 = 0.6) at 37 °C. Next, the bacteria were centrifuged at 3000 rpm for 10 minutes and resuspended in 1/5th volume infection medium. This bacterial solution was used for further application.
TEER measurements in transwell experiments
A transwell system was applied to quantify protective effects of pectins against the barrier disrupting C. rodentium. This system allowed us to assess epithelial barrier function by measuring trans-epithelial electrical resistance (TEER) and paracellular fluxes. CMT93 cells were cultured in polycarbonate membrane cell culture inserts (1 cm2, pore size 0.4 μm; Sigma Aldrich) at a density of 100
000 cells per insert in 500 μl infection medium in the apical chamber. The basolateral chamber contained 1000 μl of infection medium. Medium was changed and TEER was measured thrice a week. TEER was measured using an EVOM2 Epithelial vol-ohm-meter (World Precision Instuments, Inc). CMT93 cells were grown for approximately 3 weeks until they reached confluency and a TEER of ∼300 Ω cm2. Confluent CMT93 cells were pretreated for 24 hours with the pectins in a concentration of 0.5, 1, or 2 mg ml−1. After 24 hours of pectin incubation, the cells were stimulated with 50 μl of the bacterial solution to the apical chamber to give a multiplicity of infection (MOI) of approximately 350. TEER was measured before C. rodentium addition (t = 0), 4 hours after C. rodentium addition (t = 4) and 24 hours after C. rodentium addition (t = 24). Cells treated with pectins only were used as control to investigate if pectins enhanced TEER.
Paracellular lucifer yellow (LY) flux
After TEER measurements, the paracellular flux of LY (Sigma Aldrich) over the epithelial cell layer was measured. LY (0.457 kDa) is a molecule that can be transported across the epithelial layer via the paracellular route and it can be used to evaluate epithelial permeability. LY was added (100 μg ml−1) to the apical chamber in the transwell plate and incubated for 3 hours. After 3 hours, 80 μl of the basolateral compartment was collected in a black 96 well plate (ThermoFisher Scientific, Waltham, USA) which protected the fluorescence of LY. A standard curve ranging from 5.12 × 10−7–100 μg ml−1 and a negative control (as blank) was also added to the black plate. Fluorescent intensity was measured using a Varioskan™ LUX multimode reader (ThermoFisher Scientific) at an excitation wavelength of 428 nm and an emission wavelength of 536 nm. The relative fluorescent intensity was determined by calculating the fluorescent intensity relative to the fluorescent intensity of the medium.
Assessment of bacterial adhesion
Bacterial adhesion was measured to evaluate whether pectins prevent the adhesion of C. rodentium to CMT93 epithelial cells (Fig. 1A). CMT93 cells were cultured in a 6-well plate (Corning Life Sciences, Kennebunk, USA) at a cell density of 600.000 cells per well in a volume of 2 ml infection medium. The cells were grown until they reached confluency. Next, the cells were pre-incubated with the pectins in the concentrations 0.5, 1, or 2 mg ml−1 for 24 hours. After 24 hours, 200 μl of the bacterial solution was added to the cells to give a MOI of approximately 150 and incubated for 4 hours. Next, 50 μl of the medium was collected to determine the growth of C. rodentium when in culture with epithelial cells. The remaining medium was removed and the epithelial cells were washed 3 times with PBS. CMT93 cells were disrupted using 1 mL of PBS containing 0.1% Triton X-100. Cells and bacteria were scraped and collected into Eppendorf tubes and the tubes were extensively vortexed before determining CFU. Bacterial growth and bacterial adherence were quantitated by plating for CFU on LB agar medium (Sigma Aldrich). The extensive washing steps required for the accurate adherence measurements excluded analysis of later time points than 4 h due to cell sloughing.
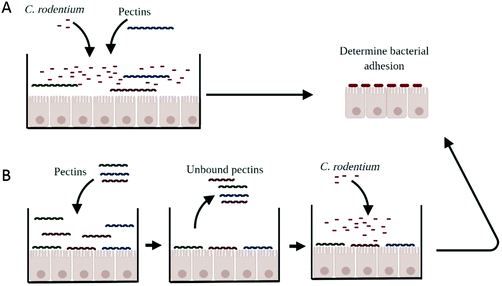 |
| Fig. 1 Schematic representation of different methods to determine the impact of pectins on bacterial adhesion to CMT93 epithelial cells. With the coincubation of pectins with C. rodentium (A), we determined whether pectins prevent adhesion of C. rodentium to CMT93 epithelial cells. With the pretreatment of pectins to epitheliail cells (B), we investigate whether pectins block adhesion sites where C. rodentium could bind to. We first pretreat cells with pectins. Then we wash off the unbound pectins and then we add C. rodentium to the epithelial cells and measure bacterial adhesion to CMT93 epithelial cells. | |
To confirm whether bacterial adhesion was blocked through the interaction of pectins to epithelial cells, epithelial cells were first incubated for 24 hours with the pectins (Fig. 1B). The next day, the solution containing pectins was removed and replaced for the bacterial solution. Cells were not washed, because that may disturb the pectin-epithelial interaction. After four hours of incubation with C. rodentium, bacterial adhesion was determined as described above.
Growth experiments
Bacterial growth was measured to determine whether pectins can inhibit the growth of C. rodentium (ATCC), Lactobacillus plantarum WCFS1 (NIZO strain collection24) or E. coli O119 (ATCC). Bacterial solution of L. plantarum WCFS1 and E. coli O119 were prepared as described above for C. rodentium. For growth experiments, bacteria were not dissolved in DMEM medium but in LB medium. Pectins were dissolved in LB medium at 2 mg ml−1. To 2 ml of pectin solution, 50 μl of bacteria solution was added. OD600 was measured each hour for 4 hours.
Competition ELISA for binding of C. rodentium to pectin
To demonstrate that pectins bind to C. rodentium, a competition ELISA was designed. The ELISA buffer (1 mM CaCl2; 150 mM NaCl; 0.05 M Tris buffer; PH 8.2) was used for washing (400 μl per well) and diluting pectins.18 Antibodies were diluted in blocking buffer (3% milk powder; FrieslandCampina, Amersfoort, The Netherlands) with ELISA buffer in a ratio of 1
:
2. ELISA plates (Corning, Tewksbury, MA, USA) were coated with 100 μl of 50 μg ml−1 of poly-L-lysine for 90 minutes at 37 °C. The plates were washed once with ELISA buffer. Next, DM59 pectin was serially diluted in ELISA buffer at 0.02, 0.2, 2, 20 and 200 μg ml−1 and 50 μl of these pectins solution were incubated for 4 hours at 37 °C. The plate was washed once and blocked overnight with 100 μl blocking buffer at 4 °C. The next day, the bacteria grown to mid-log phase (OD600 = 0.6) was resuspended at 1
:
10, 1
:
100 or 1
:
1000 in ELISA buffer, and the bacterial solutions were added to each well. Four hours after the incubation at 37 °C, the plates were washed thrice, and rat anti-pectin antibody LM20 (1
:
200; Plantprobes, Leeds, UK) were added into the wells as a primary antibody to detect the amount of plate-bound pectin. The primary antibody was incubated for 1 hour at 37 °C. Anti-rat IgM-biotin antibodies (1
:
1000; Abcam) were used as secondary antibodies for pectin binding and incubated for 1 hour at 37 °C. The plates were washed five times and incubated with 100 μl Streptavidin-HRP (1
:
1000; Southern Biotech) for 45 minutes at 37 °C and subsequently washed seven times. As final step, 100 μl 3,3′,5,5′-tetramethylbenzidine (Cell Signaling) was incubated for 30 minutes at 37 °C. The reaction was stopped with 100 μl stop solution (Cell Signaling). The plate readout was performed at 420 nm using Versa Max ELISA plate reader (Molecular Devices). Each pectin standard curve was generated as a 4-parameter sigmoid curve, and the ED50 without competitors (control) was compared to that with possible competitors.
Statistics
The results were analysed using Graphpad Prism program (La Jolla, CA, USA). Normal distribution was confirmed using Kolmogorov–Smirnov test. Values are expressed as mean ± standard error (SEM). Statistical comparisons were performed using one-way repeated-measures ANOVA for analysis of parametrically distributed data and Kruskal–Wallis for analysis of non-parametrically distributed data. Two-way repeated measures ANOVA was performed for grouped analysis of parametrically distributed data. Post-testing was performed with Dunnet post-test (* p < 0.05 was considered as statistically significant; * p < 0.05, ** p < 0.01, *** p < 0.001), **** p < 0.0001).
Results
Chemical composition of pectins
Pectins were characterized for the DM, degree of blockiness (DB), molecular weight, and sugar composition (Table 1). The characteristics of the pectins are given in Table 1. The pectins did not differ in molecular weight or sugar composition but mainly differed in the level and distribution of methyl esters. To investigate DM-dependent effects, one low DM pectin (DM32) and two high DM pectins were used (DM59 and DM64). The two high DM pectins (DM59 and DM64) differed in the DB (17% and 37%, respectively), which allowed us to study DB-dependent effects. The low DM pectin contained a DB of 35%.
Table 1 Structural characteristics of pectins
Pectin |
Origin |
DB (%) |
M
w (kDa) |
Neutral sugar composition (mol%) |
Carbohydrate content (%) |
Rha |
Ara |
Gal |
Glc |
UA |
Degree of methyl-esterification (DM): mol of methanol per 100 mol of the total GalA in the sample. Degree of blockiness (DB): the amount of mono-, di- and triGalA per 100 mol of the non-esterified GalA in the sample. Molecular weight (Mw) as measured by HPSEC. Rha = rhamnose, Ara = arabinose, Gal = Galactose, Glc = Glucose, UA = Uronic Acid (galacturonic acids). |
DM32 |
Orange |
35 |
77 |
1 |
3 |
6 |
1 |
85 |
57 |
DM59 |
Orange |
17 |
86 |
1 |
3 |
8 |
1 |
88 |
87 |
DM64 |
Orange |
37 |
92 |
1 |
7 |
8 |
2 |
82 |
81 |
Pectins protect the epithelial barrier against C. rodentium induced damage in a structure independent manner
Pectins might protect epithelial integrity against damaging agents or pathogens. Previously it was demonstrated that pectins protect the epithelial integrity against barrier disrupting PMA through direct stimulation of epithelial integrity.13 This effect of pectins was induced in a DM-dependent manner.13 It is however unknown whether pectins can protect the epithelium against the barrier disrupting pathogen C. rodentium. Pectins that structurally differed in DM and DB were tested to investigate whether structurally different pectins play a role in the protective effects of pectins on epithelial integrity against C. rodentium.
As shown in Fig. 2, all three pectins reduced the C. rodentium induced decline in TEER of CMT93 epithelial cells at a similar level (Fig. 2A and B). This effect was measured after 4 hours of C. rodentium incubation and even maintained after 24 hours of C. rodentium incubation. The most clear effects of pectins on epithelial integrity were measured with 2 mg ml−1. After 4 hours of C. rodentium incubation, C. rodentium reduced TEER with 25.4% (p < 0.0001 compared to control). However, at 2 mg ml−1 pectins with DM32, DM59 and DM64 reduced TEER with 5.9% (p < 0.001 compared to C. rodentium only), 10.9% (p < 0.001 compared to C. rodentium only) 5.7% (p < 0.001 compared to C. rodentium only), respectively. After 24 hours of C. rodentium incubation, C. rodentium reduced TEER with 62.4% (p < 0.0001 compared to medium only). However, at 2 mg ml−1 pectins with DM32, DM59 and DM64 reduced TEER with 48.6% (p < 0.01 compared to C. rodentium only), 52.4% (p < 0.05 compared to C. rodentium only) and 52.6% (p < 0.05 compared to C. rodentium only), respectively. Pectins without the addition of C. rodentium did not influence the TEER of CMT93 cells (Fig. 2A and B). Furthermore, the protective effects of the pectins were also visible after measuring paracellular fluxes of LY over the epithelial barrier (Fig. 2C). The addition of C. rodentium strongly stimulated the paracellular transport of LY (p < 0.0001 vs. control). However, at 2 mg ml−1 DM32, DM59 and DM64 reduced paracellular transport of LY with 27.8% (p < 0.05), 33.1% (p < 0.01) and 27.3% (p < 0.01). The effects of pectins on paracellular transport of LY after a C. rodentium challenge were independent of the DM and the DB. These results show that the different pectins induce a similar level of protection of the epithelial integrity, independent of the DM and the DB.
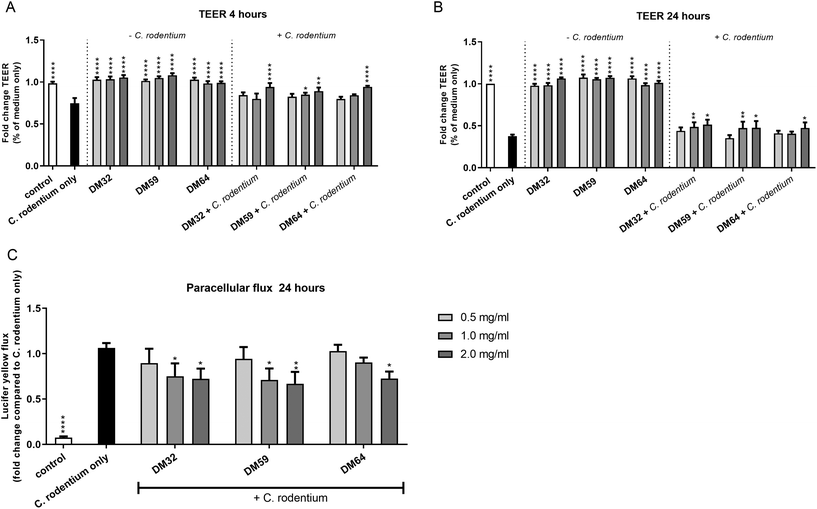 |
| Fig. 2 Protective effects of pectins against C. rodentium induced barrier disruption. TEER measurements of CMT93 cells treated with pectins and C. rodentium. Cells were pretreated for 24 hours with DM32, DM59 and DM64 pectins at 0.5, 1.0 and 2.0 mg ml−1, after which medium (−C. rodentium) or C. rodentium (+C. rodentium) were applied. TEER was measured at 4 hours (A) and 24 hours (B) incubation with C. rodentium. Next, paracellular transport of lucifer yellow over the epithelial layer was measured of pectin + C. rodentium treated wells (C). Significant differences compared to the C. rodentium only were indicated by * (p < 0.05), ** (p < 0.01) or **** (p < 0.0001). | |
We next tested whether the protective effect of pectins on epithelial integrity may be related to the activation of TLR2, as activation of TLR2 plays an important role in tight-junction mediated epithelial barrier function.25 As shown in Fig. 3, all pectins were tested on TLR2 activating properties. Both high DM pectins (DM59 and DM64) strongly activated TLR2, whereas the low DM pectin (DM32) showed low activation of TLR2. There was no difference in TLR2 activation observed between the high DM pectins, showing that the DB of pectins does not affect TLR2 activation. At 2 mg ml−1, DM32 pectin activated TLR2 by 2.2 fold (p < 0.05 vs. control), DM59 pectin activated TLR2 by 7.6 fold (p < 0.0001 vs. control) and DM64 activated TLR2 by 7.6 fold (p < 0.0001 vs. control). Since the pectins show a different pattern in TLR2 activation than in the protection of the epithelium against C. rodentium, these results suggest that the protective effects of pectins on epithelial integrity against C. rodentium were independent of TLR2 activation.
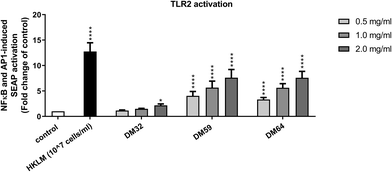 |
| Fig. 3 TLR2 activating properties of structurally different pectins. Mouse TLR2 reporter cells expressing an secreted alkaline phosphatase (SEAP) reporter gene under the control of a promoter inducible by NF-B and AP-1 transcription factors were treated with DM32, DM59 and DM64 pectins at 0.5, 1.0 and 2.0 mg ml−1. TLR2 activation was determined by measuring the SEAP activity secreted into cell culture supernatant. Significant differences compared to control were indicated by * (p < 0.05) or **** (p < 0.0001). | |
Pectins induce anti-adhesive effects of C. rodentium on epithelial cells by interacting with C. rodentium and not with the epithelium
To study how pectins can protect the epithelium against C. rodentium, the adhesion of C. rodentium to CMT93 epithelial cells was determined. Epithelial cells were pretreated with pectins and then incubated for 4 hours with C. rodentium. As shown in Fig. 4A, pectins strongly inhibited the attachment of C. rodentium to CMT93 cells. At 2 mg ml−1, pectin with DM32, DM59 and DM64 induced anti-adhesive effects of 87.6% (p < 0.001), 85.4% (p < 0.01) and 81.6% (p < 0.01), respectively. As with TEER and LY fluxes, the effects of pectins on bacterial attachment were independent of the DM or DB. This indicates that pectins protect the epithelial barrier by reducing the adhesion of C. rodentium to epithelial cells.
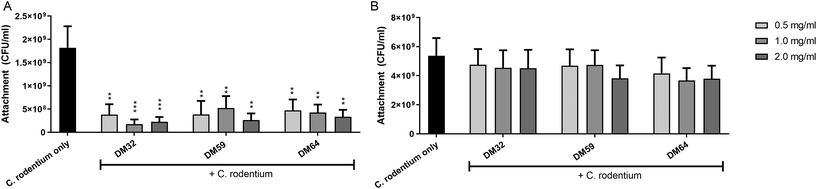 |
| Fig. 4 Protective effects of pectins against the attachment of C. rodentium to CMT93 epithelial cells. CMT93 cells were pre-treated with pectins for 24 hours, and then C. rodentium was inoculated under pectin coexisting conditions (A) or under pectin removal conditions (B) in the culture medium. Significant differences compared to the C. rodentium only were indicated by ** (p < 0.01) or *** (p < 0.001). | |
We next investigated whether pectins interact with epithelial cells directly which may be a possible mechanism explaining how pectins prevent the attachment of C. rodentium to epithelial cells. Pectins were first incubated on epithelial cells for 24 hours. Afterward, the medium containing unbound pectins was removed and replaced for medium containing C. rodentium. After 4 hours, the attachment of C. rodentium was assessed. As shown in Fig. 4B, the pectins did not induce anti-adhesive effects on C. rodentium by having a direct interaction with epithelial cells. This suggests that the anti-adhesive effects of pectins on C. rodentium were derived from a direct interaction of the pectins with C. rodentium.
Pectins inhibit the growth of C. rodentium
We hypothesize that pectins directly interact with C. rodentium. Therefore, we investigated if pectins influence the growth of C. rodentium. As shown in Fig. 5A, C. rodentium exhibited a progressive increase in OD600 value from one to four hours after incubation without pectins (control). However, C. rodentium incubated with the pectins showed little growth for 4 hours. The AUC of pectin-treated C. rodentium was significantly decreased as compared to that of pectin-free C. rodentium (Fig. 5B). Consistent with the results of C. rodentium infection to CMT93 cells, pectins strongly inhibited the growth of C. rodentium, irrespectively of DM. Furthermore, we investigated whether the whole pectin molecule or fractions of the pectin molecule are required for the growth inhibition of C. rodentium. The growth inhibitory effect of RG-I polysaccharides or poly-galacturonic acid (analog to pectin backbone with DM0) was tested. The RG-I, polysaccharides composed of neutral sugars, is known to covalently attach with the pectin backbone.19 The growth of C. rodentium did, however, not change after incubation with either RG-I or poly-galacturonic acid (Fig. 5C and D). This suggests that the whole molecule of pectin is important for the growth inhibitory effect.
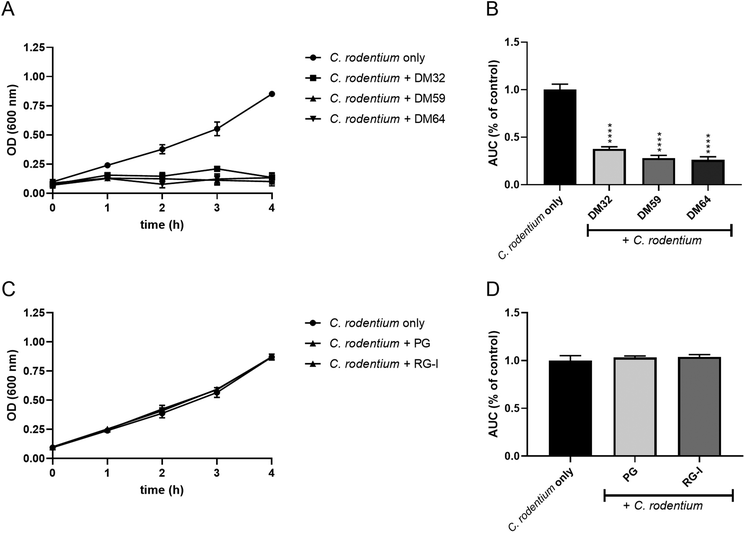 |
| Fig. 5 Growth curves of C. rodentium co-incubated with pectins. In a concentration of 2 mg ml−1, whole pectins (A and B), or with poly-galacturonic acid (PG; DM0 pectin) and RG-I (C and D) were added in the LB medium, and OD600 was measured for 4 hours. Significant differences compared to the C. rodentium only were indicated by **** (p < 0.0001). | |
To determine whether the effect was C. rodentium specific, we also studied the influence of pectins on the growth of other bacteria, L. plantarum WCFS1 and E. coli O119. The growth curves of L. plantarum exhibited similar kinetics with or without pectins (Fig. 6A). However, the AUC of pectin-treated L. plantarum was slightly but significantly decreased as compared to that of pectin-free L. plantarum cultures (Fig. 6B). The growth and AUC of E. coli were similar in cultures with or without pectins (Fig. 6C and D). These results suggest that pectin acts preferentially on C. rodentium to inhibit its growth.
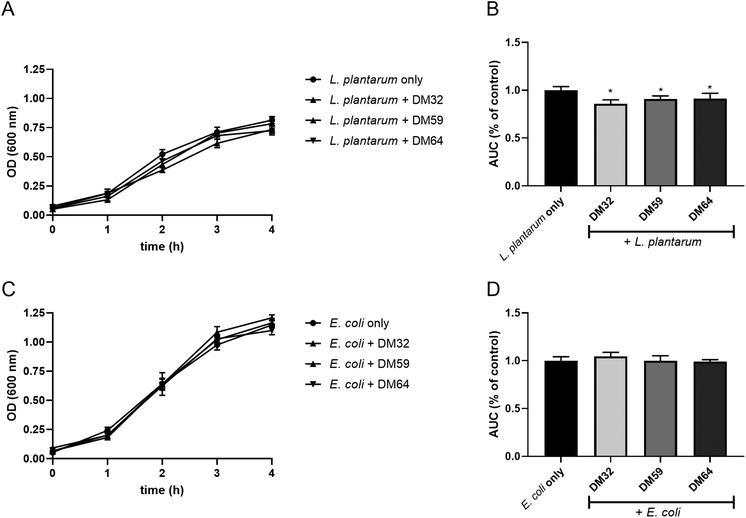 |
| Fig. 6 Growth curves of L. plantarum and E. coli co-incubated with pectins. L. plantarum (A and B) and E. coli (C and D) was cultured in the LB medium containing 2 mg ml−1 of whole pectins, and OD600 was measured for 4 hours. Significant differences compared to the L. plantarum only or E. coli only were indicated by * (p < 0.05). | |
Pectins preferentially interact with C. rodentium
As the pectins markedly inhibited the growth of C. rodentium, we conducted an ELISA-based competition assay to detect a direct interaction between pectin and C. rodentium. We developed an ELISA that detects plate-coated pectins by using an anti-pectin antibody. We examined whether the interaction between anti-pectin antibody and pectin was inhibited by incubating bacteria including C. rodentium, L. plantarum, and E. coli as its potential competitors. As shown in Fig. 7A, the ED50 of the pectin antibody was significantly increased in a bacterial dose dependent manner by co-incubating C. rodentium. Although L. plantarum significantly increased the ED50 of pectin antibody at the highest concentration (L. plantarum 1
:
10, p < 0.05 vs. control; Fig. 7B), this increase was smaller as compared with the C. rodentium competitor. Since E. coli showed only a marginal effect on the ED50 of pectin antibody (Fig. 7C), the competitive activities were correlated with the bacterial growth inhibitions (Fig. 5 and 6). Together, these results suggest that pectin preferentially interacts with C. rodentium, and thereby inhibits its bacterial growth.
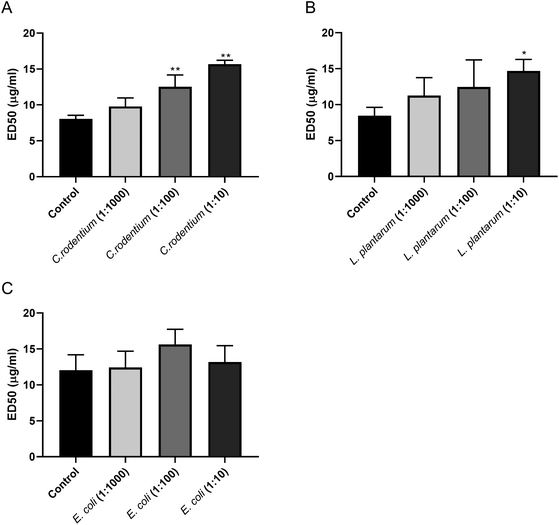 |
| Fig. 7 ED50 of pectin antibody against plate-bound DM59 pectin in ELISA. Serial dilutions of C. rodentium (A), L. plantarum (B), or E. coli (C) suspension were pre-incubated before reacting with anti-pectin antibody in ELISA. Significant differences compared to control were indicated by * (p < 0.05) or ** (p < 0.01). | |
Discussion
To the best of our knowledge, this is the first study demonstrating that the dietary fibre pectin limits C. rodentium induced epithelial barrier disruption through direct interactions with C. rodentium per se. Previous studies demonstrated that dietary fibres exert protective effects against C. rodentium by stimulating the growth of commensals that outcompete the growth of C. rodentium4,5 or by stimulating the production SCFAs,6,7 but the current study shows an additional property of the dietary fibre pectin to protect the epithelial barrier from C. rodentium induced damage by inhibiting the growth of this enteric pathogen.
The present study demonstrates that pectin preserves epithelial integrity from C. rodentium induced disruption (Fig. 2). This is in line with previous studies showing that pectin enhances epithelial barrier function by upregulating tight junction proteins in rats and mice.26,27 Several studies also demonstrated that pectin can bind to TLR213,18 and that stimulation of TLR2 on epithelial cells maintains epithelial integrity mediated by the tight junction protein ZO-1.25,28 Although it is tempting to assume that stimulation of TLR2 by pectin may protect the epithelial barrier against C. rodentium, our data showed that protection of the epithelium is independent of TLR2 activation. Instead, our data suggest that the protective effect of pectin against C. rodentium induced barrier disruption may be, at least partly, due to the inhibition of the bacterial adhesion to epithelial cells. The anti-adhesive effect of pectin was only observed when pectin and C. rodentium coexisted, but not when pectin was removed from supernatant before C. rodentium infection (Fig. 4A and B), suggesting that the protective effect on epithelial integrity is induced by the action of pectins on C. rodentium per se and not on epithelial cells. This anti-adhesive effect of pectin on C. rodentium is in line with the anti-adhesive effects of galacto-oligosaccharides and pectins on C. rodentium and E. coli O157:H7, respectively.29,30 Accordingly, these results indicate that pectin does not target TLR2 or epithelial cells to augment epithelial barrier integrity or block bacterial translocation, but pectins target C. rodentium instead and inhibit their adhesion to epithelial cells.
The anti-adhesive effects of pectin on C. rodentium may be explained by the growth inhibitory effects of pectin on C. rodentium. Pectin markedly inhibited the growth of C. rodentium, but not that of L. plantarum and E. coli (Fig. 5 and 6). Our study is in line with several studies showing that dietary fibres inhibit the growth of pathogens,31,32 but the exact mechanism of how the dietary fibre exerts this specific antimicrobial effects on pathogens is not fully understood. Our data showed that this might be accomplished through the interaction between C. rodentium and pectins. C. rodentium competed and inhibited the interaction between pectin and pectin antibody, whereas L. plantarum showed only weak competition with the pectin antibody and E. coli did not compete with the pectin antibody at all (Fig. 7). These results suggest that pectin preferentially interacts with C. rodentium, presumably via binding to the bacterial surface molecules expressed only in C. rodentium. Chen et al.,12 have proposed that high DM pectin was able to bind with lipopolysaccharide, a major component of the outer membrane of Gram-negative bacteria. Besides, pectins may also act on a periplasmic pectin-binding protein, such as sph1118 that was recently identified in Sphingomonas sp. strain A1 as a binding receptor for pectin molecules.33 However, to date, C. rodentium specific molecules associated with pectin has yet to be identified. Extensive studies revealed that several virulence factors critical for C. rodentium colonization and pathogenicity were identified, including adhesin intimin, the translocated intimin receptor, and the translocator protein EspA,34 and vaccination against intimin as antigens successfully reduced the colonization of C. rodentium.35 Although it remains unclear whether pectin interacts with surface molecules or virulence factors, we found that pectins interact directly with C. rodentium per se which may contribute to the antimicrobial of pectins.
The effects of pectins on C. rodentium may relate to specific structural characteristics of pectins. Our data showed that the whole molecule of the pectins was required for the growth inhibition of C. rodentium, whereas RG-I and polygalacturonic acid structures (DM0 pectin) did not induce these effects on C. rodentium. This is in line with a previous study showing that higher levels of RG-I structures reduced the anti-adhesive properties of pectins against the gastrointestinal pathogen Helicobacter pylori.36 This suggests that homogalacturonan regions of the pectins may be responsible for the antimicrobial and anti-adhesive effects of pectins on C. rodentium. Furthermore, we did not find DM or DB-dependent trends in anti-adhesive and antimicrobial effects of pectins on C. rodentium. Other studies found that, next to the DM of pectins, the lower molecular weight regulates the anti-adhesive properties and anti-microbial properties of pectins.29,32 This may explain the similar anti-adhesive and antimicrobial effects of the DM32, DM59, and DM64 pectins because these pectins have a relatively similar molecular weight. Together, these results indicate that the molecular weight of homogalacturonan pectins plays an important role in regulating anti-adhesive and anti-microbial effects on C. rodentium.
Taken together, our present study demonstrates that pectin prevents C. rodentium-induced damage to intestinal epithelial cells at least in part via the inhibition of C. rodentium attachment and growth. These findings illustrate a novel role of pectin as a functional food ingredient exerting an unconventional effect of dietary fibres that directly inhibit the attachment and growth of certain kinds of pathogenic bacteria. This information may be useful in the design of therapeutic approaches to preserve the epithelial barrier from damage by attaching and effacing bacteria, such as EPEC, EHEC, or C. rodentium.
Abbreviations
A/E | Attaching and effacing |
AUC | Area under the curve |
CFU | Colony forming units; |
DB | Degree of blockiness |
DM | Degree of methylesterification |
ED | Effective dose |
EHEC | Enterohemorrhagic Escherichia coli |
EPEC | Enteropathogenic Escherichia. coli |
HKLM | Heat killed Listeria monocytogenes |
LB | Lysogeny broth |
LY | Lucifer yellow |
RG | Rhamnogalacturonan |
SCFA | Short chain fatty acid |
TEER | Transepithelial electrical resistance |
TLR | Toll-like receptor |
Conflicts of interest
There are no conflicts to declare.
Acknowledgements
Research of Martin Beukema was performed within the public-private partnership ‘CarboKinetics’ coordinated by the Carbohydrate Competence Center (CCC, http://www.cccresearch.nl). CarboKinetics is financed by participating industrial partners Agrifirm Innovation Center B.V., Nutrition Sciences N.V., Cooperatie Avebe U.A., DSM Food Specialties B.V., VanDrie Holding N.V. and Sensus B.V., and allowances of The Netherlands Organisation for Scientific Research (NWO).
Graphical abstract and schematic figures in Fig. 3 were created with BioRender.com.
References
- J. P. Nataro and J. B. Kaper, Diarrheagenic Escherichia coli, Clin. Microbiol. Rev., 1998, 47, 3–30 Search PubMed.
- S. A. Luperchio, J. V. Newman, C. A. Dangler, M. D. Schrenzel, D. J. Brenner and A. G. Steigerwalt,
et al., Citrobacter rodentium, the causative agent of transmissible murine colonic hyperplasia, exhibits clonality: Synonymy of C. rodentium and mouse-pathogenic Escherichia coli, J. Clin. Microbiol., 2000, 38, 4343–4350 CrossRef CAS.
- J. W. Collins, K. M. Keeney and V. F. Crepin,
et al., Citrobacter rodentium: infection, inflammation and the microbiota, Nat. Rev. Microbiol., 2014, 12, 612–623 CrossRef CAS.
- M. S. Desai, A. M. Seekatz, N. M. Koropatkin, N. Kamada, C. A. Hickey and M. Wolter,
et al., A Dietary Fiber-Deprived Gut Microbiota Degrades the Colonic Mucus Barrier and Enhances Pathogen Susceptibility, Cell, 2016, 167, 1339–1353 CrossRef CAS.
- N. Kamada, Y. G. Kim and H. P. Sham,
et al., Regulated virulence controls the ability of a pathogen to compete with the gut microbiota, Science, 2012, 336, 1325–1329 CrossRef CAS.
- J. A. Jiminez, T. C. Uwiera, D. W. Abbott, R. R. E. Uwiera and G. D. Inglis, Butyrate Supplementation at High Concentrations Alters Enteric Bacterial Communities and Reduces Intestinal Inflammation in Mice Infected with Citrobacter rodentium, mSphere, 2017, 2, e00243-17 CrossRef.
- L. Osbelt, S. Thiemann, N. Smit, T. R. Lesker, M. Schröter and E. J. C. Gálvez,
et al., Variations in microbiota composition of laboratory mice influence Citrobacter rodentium infection via variable short-chain fatty acid production, PLoS Pathog., 2020, 16, e1008448 CrossRef CAS.
- Y. Furusawa, Y. Obata and S. Fukuda,
et al., Commensal microbe-derived butyrate induces the differentiation of colonic regulatory T cells, Nature, 2013, 504, 446–450 CrossRef CAS.
- K. M. Maslowski, A. T. Vieira and A. Ng,
et al., Regulation of inflammatory responses by gut microbiota and chemoattractant receptor GPR43, Nature, 2009, 461, 1282–1286 CrossRef CAS.
- C. Sina, O. Gavrilova and M. Förster,
et al., G protein-coupled receptor 43 is essential for neutrophil recruitment during intestinal inflammation, J. Immunol., 2009, 183, 7514–7522 CrossRef CAS.
- P. D. Cani, S. Possemiers and T. Van de Wiele,
et al., Changes in gut microbiota control inflammation in obese mice through a mechanism involving GLP-2-driven improvement of gut permeability, Gut, 2009, 58, 1091–1103 CrossRef CAS.
- C. H. Chen, M. T. Sheu and T. F. Chen,
et al., Suppression of endotoxin-induced proinflammatory responses by citrus pectin through blocking LPS signaling pathways, Biochem. Pharmacol., 2006, 72, 1001–1009 CrossRef CAS.
- L. M. Vogt, N. M. Sahasrabudhe, U. Ramasamy, D. Meyer, G. Pullens and M. M. Faas,
et al., The impact of lemon pectin characteristics on TLR activation and T84 intestinal epithelial cell barrier function, J. Funct. Foods, 2016, 22, 398–407 CrossRef CAS.
- E. G. Maxwell, N. J. Belshaw, K. W. Waldron and V. J. Morris, Pectin – An emerging new bioactive food polysaccharide, Trends Food Sci. Technol., 2012, 24, 64–73 CrossRef CAS.
- B. Min, O. K. Koo, S. H. Park, N. Jarvis, S. C. Ricke, P. G. Crandall and S. O. Lee, Fermentation Patterns of Various Pectin Sources by Human Fecal Microbiota, Food Nutr. Sci., 2015, 6, 1103–1114 CAS.
- D. Mohnen, Pectin structure and biosynthesis, Curr. Opin. Plant Biol., 2008, 11, 266–277 CrossRef CAS.
- L. Tian, J. Scholte and K. Borewicz,
et al., Effects of pectin supplementation on the fermentation patterns of different structural carbohydrates in rats, Mol. Nutr. Food Res., 2016, 60, 2256–2266 CrossRef CAS.
- N. M. Sahasrabudhe, M. Beukema and L. Tian,
et al., Dietary Fibre pectin directly blocks Toll-Like receptor 2–1 and prevents doxorubicin-induced ileitis, Front. Immunol., 2018, 9, 383 CrossRef.
- K. H. Caffall and D. Mohnen, The structure, function, and biosynthesis of plant cell wall pectic polysaccharides, Carbohydr. Res., 2009, 344, 1879–1900 CrossRef CAS.
- M. Beukema, M. M. Faas and P. Vos de, The effects of different dietary fiber pectin structures on the gastrointestinal immune barrier: impact via gut microbiota and direct effects on immune cells, Exp. Mol. Med., 2020, 52, 1364–1376 CrossRef CAS.
- H. N. Englyst and J. H. Cummings, Simplified method for the measurement of total non-starch polysaccharides by gas - liquid chromatography of constituent sugars as alditol acetates, Analyst, 1984, 109, 937–942 RSC.
- M. Beukema, É. Jermendi, M. A. van den Berg, M. M. Faas, H. A. Schols and P. de Vos, The impact of the level and distribution of methyl-esters of pectins on TLR2-1 dependent anti-inflammatory responses, Carbohydr. Polym., 2021, 251, 117093 CrossRef CAS.
- A. A. Torkova, K. V. Lisitskaya, I. S. Filimonov, O. A. Glazunova, G. S. Kachalova and V. N. Golubev,
et al., Physicochemical and functional properties of Cucurbita maxima pumpkin pectin and commercial citrus and apple pectins: A comparative evaluation, PLoS One, 2018, 13, e0204261 CrossRef.
- P. de Vos, Z. Mujagic, B. J. de Haan, R. J. Siezen, P. A. Bron and M. Meijerink,
et al., Lactobacillus plantarum strains can enhance human mucosal and systemic immunity and prevent non-steroidal anti-inflammatory drug induced reduction in T regulatory Cells, Front. Immunol., 2017, 8, 1000 CrossRef.
- E. Cario, G. Gerken and D. K. Podolsky, Toll-like receptor 2 enhances ZO-1-associated intestinal epithelial barrier integrity via protein kinase C, Gastroenterology, 2004, 127, 224–238 CrossRef CAS.
- T. Jiang, X. Gao and C. Wu,
et al., Apple-derived pectin modulates gut microbiota, improves gut barrier function, and attenuates metabolic endotoxemia in rats with diet-induced obesity, Nutrients, 2016, 8, 126 CrossRef.
- Y. Sun, Y. He, F. Wang, H. Zhang, P. de Vos and J. Sun, Low-methoxyl lemon pectin attenuates inflammatory responses and improves intestinal barrier integrity in caerulein-induced experimental acute pancreatitis, Mol. Nutr. Food Res., 2017, 61, 1600885 CrossRef.
- E. Cario, G. Gerken and D. K. Podolsky, Toll-like receptor 2 controls mucosal inflammation by regulating epithelial barrier function, Gastroenterology, 2007, 132, 1359–1374 CrossRef CAS.
- R. Di, M. S. Vakkalanka, C. Onumpai, H. K. Chau, A. White and R. A. Rastall,
et al., Pectic oligosaccharide structure-function relationships: Prebiotics, inhibitors of Escherichia coli O157:H7 adhesion and reduction of Shiga toxin cytotoxicity in HT29 cells, Food Chem., 2017, 227, 245–254 CrossRef CAS.
- H. Kittana, M. I. Quintero-Villegas, L. B. Bindels, J. C. Gomes-Neto, R. J. Schmaltz, R. R. Segura Munoz, L. A. Cody, R. A. Moxley, J. Hostetter, R. W. Hutkins and A. E. Ramer-Tait, Galactooligosaccharide supplementation provides protection against citrobacter rodentium-induced colitis without limiting pathogen burden, Microbiology, 2018, 164, 154–162 CrossRef CAS.
- A. E. Lin, C. A. Autran, A. Szyszka, T. Escajadillo, M. Huang and K. Godula,
et al., Human milk oligosaccharides inhibit growth of group B Streptococcus, J. Biol. Chem., 2017, 292, 11243–11249 CrossRef CAS.
- P. J. Li, J. L. Xia, Z. Y. Nie and Y. Shan, Pectic oligosaccharides hydrolyzed from orange peel by fungal multi-enzyme complexes and their prebiotic and antibacterial potentials, LWT–Food Sci. Technol., 2016, 69, 203–210 CrossRef CAS.
- H. Konishi, M. Hio, M. Kobayashi, R. Takase and W. Hashimoto, Bacterial chemotaxis towards polysaccharide pectin by pectin-binding protein, Sci. Rep., 2020, 10, 3977 CrossRef CAS.
- W. Deng, J. L. Puente and S. Gruenheid,
et al., Dissecting virulence: systematic and functional analyses of a pathogenicity island, Proc. Natl. Acad. Sci. U. S. A., 2004, 101, 3597–3602 CrossRef CAS.
- M. Ghaem-Maghami, C. P. Simmons and S. Daniell,
et al., Intimin-specific immune responses prevent bacterial colonization by the attaching-effacing pathogen Citrobacter rodentium, Infect. Immun., 2001, 69, 5597–5605 CrossRef CAS.
- J. H. Lee, J. S. Shim, J. S. Lee, M. K. Kim, M. S. Chung and K. H. Kim, Pectin-like acidic polysaccharide from Panax ginseng with selective antiadhesive activity against pathogenic bacteria, Carbohydr. Res., 2006, 341, 1154–1163 CrossRef CAS.
|
This journal is © The Royal Society of Chemistry 2021 |