DOI:
10.1039/D0FO02274H
(Paper)
Food Funct., 2021,
12, 315-327
Maternal organic selenium supplementation during gestation improves the antioxidant capacity and reduces the inflammation level in the intestine of offspring through the NF-κB and ERK/Beclin-1 pathways†
Received
29th August 2020
, Accepted 21st November 2020
First published on 23rd November 2020
Abstract
Selenium (Se) is postulated to protect against inflammation in the gut by attenuating oxidative stress. This study was conducted to investigate the effects of maternal 2-hydroxy-4-methylselenobutanoic acid (HMSeBA), an organic Se source, on the intestinal antioxidant capacity and inflammation level of the offspring and its possible mechanism. Forty-three sows were randomly assigned to receive one of the following three diets during gestation: control diet, sodium selenite (Na2SeO3) supplemented diet or HMSeBA supplemented diet, respectively. Samples were collected from the offspring at birth and weaning. The results showed that maternal HMSeBA supplementation significantly upregulated ileal GPX2 and SePP1 gene expression compared with the control and Na2SeO3 groups, while suppressed the expression of ileal IL-1β, IL-6 and NF-κB genes in newborn piglets compared with the control group. Moreover, maternal HMSeBA supplementation significantly increased the protein of ileal GPX2 and p-mTOR compared with the control and Na2SeO3 groups, but decreased the ileal p-NF-κB, Beclin-1 and p-ERK proteins in newborn piglets compared with the control group. The weaned piglets of the HMSeBA group had lower serum IL-1β and IL-6 than the piglets of the control group at 2 h of LPS challenge. In addition, after the LPS challenge, the HMSeBA group had a lower relative abundance of ileal p-NF-κB and Beclin-1 proteins than the control and Na2SeO3 groups. In conclusion, maternal HMSeBA supplementation during gestation can improve the offspring's intestinal antioxidant capacity and reduce the inflammation level by suppressing NF-κB and ERK/Beclin-1 signaling.
Introduction
In addition to genetics, the function of intestine can be affected by the intrauterine environment, diet and stress, which will affect lifetime health.1 Our previous studies have shown that the maternal nutritional levels or feed additives could affect offspring's intestinal function and health.2–5 Effective gastrointestinal functionality at early life is crucial to animal health, welfare and performance.6 In addition to being an organ for digestion and absorption of nutrients, intestine is also the largest organ of the immune system.5 Maintenance of redox balance is the cornerstone of effective immunity and health of the gut.6,7 In addition, autophagy plays an important role in the regulation of intestinal inflammation.8 Moreover, autophagy and redox signaling in the gastrointestinal tract have a close link.9 Treatment with antioxidants reduced the formation of autophagosomes and the subsequent degradation of proteins.10 Thus, the regulation of redox signals during pregnancy may not only improve the inflammatory status, but also be of benefit to the function of the intestine of offspring.
Selenium (Se) is an essential trace element and occurs in nature in both inorganic and organic forms.11 The function of Se is mediated in part by its incorporation into selenoproteins.12 Se deficiency was reported to exacerbate colitis induced by dextran sulfate sodium in mice.13 In contrast, Se supplementation may attenuate inflammation and colitis severity in a colitis mouse model.14 As many selenoproteins exhibit antioxidant functions, Se has long been postulated to protect against inflammation in the gut by attenuating oxidative stress.12 In particular, nuclear factor-κB (NF-κB) is a redox-sensitive transcription factor regulated by the Se status and selenoproteins.15 Lipopolysaccharide (LPS) is a component of the cell wall of Gram-negative bacteria and can induce mammalian cell inflammation and can activate the immune systems of host animals through the TLR4-MyD88-NF-κB signaling pathway.5 A previous study showed that NF-κB activation was inhibited by Se supplementation with LPS challenge.16 In addition, Wang et al.17 showed that selenium could protect effectively from chromium-induced autophagy. Se could improve the arsenic-induced apoptotic pathway by inhibiting mTOR/Atk mediated autophagy.18 Moreover, the function of mTOR-dependent autophagy in the pathogenesis of intestinal inflammation was modulated by the TLR4-MyD88-MAPK signal and the NF-κB pathway.19 A stable organic Se source HMSeBA, named Selisseo® (SO), has been shown to be more bioavailable than sodium selenite or selenium yeast.20,21 Maternal HMSeBA supplementation during gestation may reduce the inflammation level and inhibit autophagy by increasing GPX2 and regulating the NF-κB and ERK/mTOR/Beclin-1 pathways. In this study, we have investigated the effects of HMSeBA supplementation during gestation on the intestinal antioxidant capacity and inflammation level of offspring based on our previous results.
Materials and methods
Ethics approval
This study was carried out in accordance with the Guide for the Care and Use of Laboratory Animals prepared by the Institutional Animal Care and Use Committee of Sichuan Agricultural University, and all animal protocols were approved by the Animal Care and Use Committee of Sichuan Agricultural University (approval number: DKYB20131704).
Animal study
Sows were treated as previously mentioned.20 Briefly, forty-three five-parity sows (Landrace × Yorkshire) with 222.11 ± 3.17 kg initial body weight (BW) (initial backfat thickness (BF), 13.76 ± 0.63 mm) were artificially inseminated on the day of estrus. After artificial insemination, sows were randomly assigned to receive one of the following three diets during gestation: control diet (control, basal diet, n = 15), Na2SeO3 supplemented diet (Na2SeO3, basal diet + 0.3 mg Se per kg as Na2SeO3, n = 13) or 2-hydroxy-4-methylselenobutanoic acid (HMSeBA) supplemented diet (HMSeBA, basal diet + 0.3 mg Se per kg as HMSeBA, n = 15). HMSeBA (2-hydroxy-4-methylselenobutanoic acid, Selisseo® 2% Se) was provided by Adisseo France S.A.S. and Na2SeO3 was obtained from Chengdu Shuxing Feed Co. Ltd (1% Se). The experimental diets were formulated to meet the nutrient requirements of gestating sows as recommended by the National Research Council (NRC, 2012) (ESI, Table 1†), except for that of selenium. During gestation, all sows ingested 2.32 kg of diet daily (8:30 and 14:30) from day 0 to day 90 of gestation and 2.76 kg of diet daily (8:30 and 14:30) from day 91 of gestation to parturition. After farrowing, sows received the same standard lactation diet as recommended by the NRC (2012) (ESI, Table 1†). In the first 24 h after farrowing, the litter sizes were adjusted to 12 ± 1 piglets per nest by cross-fostering during the same treatment. Sow's milk was the unique source of food to the suckling piglet during lactation. Throughout the experiment, all animals had free access to water. The ambient temperature for gestation sows was maintained at 20–23 °C. Heating light and pads were provided for suckling piglets and the temperature was maintained at 26–32 °C, which was gradually decreased with the increase in age.
Escherichia coli lipopolysaccharide challenge
After the separation of piglets from sows (day 21 post-birth), twelve healthy male piglets from six sows (two piglets per sow) were selected from each of the three treatment groups. Six piglets in each treatment group were intraperitoneally injected with Escherichia coli lipopolysaccharide (LPS, Escherichia coli L2880, Sigma) at a dose of 50 μg per kg body weight, while the other six piglets were intraperitoneally injected with an equivalent amount of sterile saline. The piglets were separated from sows and separately raised in a separate metabolic cage (1.2 × 0.4 × 0.5 m) 4 h before the injection. The rectal temperature of each piglet was measured at 0 h, 2 h and 4 h after LPS/saline injection. LPS was dissolved in sterile saline to prepare the stock solution (500 mg LPS per liter of saline). The piglets were sacrificed 4 h after LPS injection, as previously described.5
Sample collection
Ten newborn piglets of each group (one piglet with an average litter BW per sow) were selected for intestine collection before the colostrum intake. Meanwhile, fasting weaned piglets were harvested 4 h post LPS or saline injection for blood and intestine collection. All pigs were anesthetized with an intramuscular injection of sodium pentobarbital (50 mg per kg BW) before sample collection, and then they were slaughtered. The middle portion (approximately 3 cm) of the ileum was clipped rapidly and rinsed with saline, and then snap-frozen and stored at −80 °C for further analysis, as previously described.5 In addition, about 1 cm jejunum and ileum tissues were fixed in 4% paraformaldehyde for immunohistochemistry analysis.
Analysis of the oxidant and antioxidant content
Before the analysis, the ileum was rapidly weighed, thawed, and placed in sterile Eppendorf tubes containing ice-cold phosphate buffer saline (PBS). The ratio of the ileal tissue sample (g) to PBS (mL) was 1
:
9. A mixture of the weighed tissue and ice-cold PBS was homogenized using a tissue homogenizer (Bullet Blender, Next Advance, Inc., Averill Park, NY, USA) and then centrifuged at 6000g for 15 min at 4 °C.22 The supernatants were used to measure the oxidant and antioxidant content. The content of malondialdehyde (MDA), activities of anti-superoxide anion free radical (ASAFR), catalase (CAT), superoxide dismutase (SOD), glutathione peroxidase (GPH-Px) and hydrogen peroxide (H2O2) contents and total antioxidant capacity (T-AOC) were analyzed using the respective assay kits (Nanjing Institute of Jiancheng Biological Engineering, Nanjing, China) according to the manufacturers’ instructions. Briefly, MDA was quantified using the thiobarbituric acid reactive substances method. ASAFR was quantified by simulating the reaction system of xanthine and xanthine oxidase in the body. The CAT activity was quantified using the reaction of CAT to decompose H2O2. The SOD activity was quantified using the superoxide anion radical produced by xanthine and the xanthine oxidase reaction system. GSH-Px can promote the reaction of hydrogen peroxide and reduced glutathione to produce water and oxidized glutathione. Thus, the activity of GSH-Px can be reflected by measuring the consumption rate of reduced glutathione in the enzymatic reaction. H2O2 was detected spectrophotometrically at 405 nm in terms of developing chelates with molybdic acid. T-AOC was detected spectrophotometrically at 520 nm in terms of developing stable and colored chelates. The protein content in the tissue lysate was used to calculate the foregoing antioxidant parameter activities.22
Serum TNF-α, IL-1β, IL-10 and IL-6 analyses
Serum tumor necrosis factor-α (TNF-α), interleukin-1β (IL-1β), interleukin-10 (IL-10) and interleukin-6 (IL-6) concentrations were measured using the respective ELISA kits suitable for pigs (Nanjing Institute of Jiancheng Biological Engineering, Nanjing, China) according to the manufacturers’ protocols. The levels of TNFα, IL-1β, IL-10 and IL-6 were calculated from the standard curve and were expressed as nanograms per liter. The intra-assay and inter-assay coefficients of variation were less than 10% and 12%, respectively. The threshold levels of TNFα, IL-1β, IL-10 and IL-6 were 3 ng L−1, 0.5 ng L−1, 5 ng L−1 and 2 ng L−1, respectively.
Assessment of gene expression
The gene expression levels were detected using reverse transcription polymerase chain reaction (RT-PCR), as previously described.23 Briefly, total RNA was extracted from the frozen ileum samples with the TRI reagent (Sigma-Aldrich); approximately 50 mg of the tissue sample was homogenized in 1 mL of TRI reagent. The concentration of RNA was determined using a DU-800 nucleic acid and protein detector (Beckman Coulter Inc., Fullerton, CA) at an optical density (OD) of 260 nm. The ratio of OD260 nm to OD 280 nm ranging between 1.8 and 2.0 was considered to be acceptable. The integrity of RNA was verified by agarose gel (1%) electrophoresis. 1 μg of the isolated total RNA was used to synthesize cDNA using the Prime-Script™ RT reagent kit (Takara, Dalian, China), according to the instructions of the manufacturer. Real-time PCR assay was performed with a 7900HT ABI Prism Sequence Detection System (Applied Biosystems, Foster City, CA, USA) using SYBR green (Takara). The PCR protocol was 1 cycle of 95 °C for 30 s and 40 cycles of 95 °C for 15 s, followed by 60 °C for 1 min. Real-time PCR data were analyzed by the 2-delta CT method with β-actin as the reference gene. Primers for the genes are given in the ESI, Table 2.†
Immunohistochemistry
Immunohistochemistry of the ileal glutathione peroxidase 2 (GPX2) protein was analyzed by LiLai Biotechnology (Chengdu, Chia) as previously described.24 Briefly, the ileum samples were embedded in paraffin wax blocks, and then sectioned. After being deparaffinized, the sections were soaked in 3% H2O2 in methanol for 10 min and then washed 3 times (5 min each time) with PBS. The sections were boiled in 10 mmol L−1 citrate buffer (pH 6.0), washed twice with PBS, and then incubated with 10% goat serum albumin for 20 min at room temperature. Subsequently, the sections were incubated with the primary antibody of rabbit anti-GPX2 (1
:
500, ab137431, Abcam plc, Cambridge, UK) overnight at 4 °C. Rabbit IgG was employed as the negative control. Then the sections were incubated with biotinylated goat anti-rabbit IgG secondary antibodies (Beijing ZhongShan Golden Bridge Biotechnology Co., Ltd, Beijing, China) for 30 min at 37 °C. The sections were washed with PBS and incubated with 3,3-diaminobenzidine (DAB) to visualize the immune complexes. The sections were then counterstained with hematoxylin and mounted with glycerol gelatin. Images were obtained using a BA200 Digital microscope (Motic China Group Co., Ltd).
Western blot analysis
The relative protein abundance of GPX2, extracellular signal regulated kinase (ERK), phospho-extracellular signal regulated kinase (p-ERK), mechanistic target of rapamycin (mTOR), phospho-mechanistic target of rapamycin (p-mTOR), inhibitor of nuclear factor kappa B (IκBα), phospho-nuclear factor kappa B (p-NF-κB) and Beclin-1 were determined by western blot analysis as previously described.25 Briefly, ileum tissues were lysed with radioimmunoprecipitation assay (RIPA) lysis buffer (Beyotime, Songjiang, Shanghai, China). The protein concentration was determined by using a bicinchoninic acid (BCA) protein assay kit (Thermo Fisher Scientific, Waltham, MA, USA). 30 μg of total protein was loaded into each lane and separated on 10% sodium dodecyl sulfate polyacrylamide gel electrophoresis (SDS-PAGE) gels (Absin, Pudong, Shanghai, China). The primary antibodies of ERK1/2 (9102S), phospho-ERK1/2 (9101S), mTOR (2972), phospho-mTOR (2971), IκBα (4814S), phospho-NF-κB p65 (Ser536) (3033S), Beclin-1 (3495S) and β-actin (4967) were obtained from Cell Signaling Technology (Danvers, MA, USA). The antibody of GPX2 (ab137431) was purchased from Abcam (Cambridge, UK). Chemiluminescence detection was performed using the Clarity Western enhanced chemiluminescence (ECL) substrate (Bio-Rad, Hercules, CA, USA). Images were obtained and analyzed using the Image Lab software (Bio-Rad, Hercules, CA, USA).
Statistical analyses
SPSS 21.0 (IBM SPSS Company, Chicago, IL, USA) was used for all statistical analyses. Data of newborn piglets were analyzed using the one-way ANOVA procedure of SPSS statistical software, followed by Tukey's multiple range test. Data of weaned piglets after LPS challenge were analyzed by Duncan's multiple comparison test using the general linear model (GLM) procedure of SPSS statistical software as follows: Yijk = μ + αi + βj + (αβ)ij + εijk, where Y is the analyzed variable; μ is the mean; αi is the effect of diets (i = 1, 2 or 3); βj is the effect of LPS (j = 1 or 2); (αβ)ij refers to the interaction between diets and LPS; and εijk represents the error term. An individual piglet served as the experimental unit. The normality and homogeneity of variances were evaluated by the Shapiro–Wilk W test and Levene's test, respectively. The results are presented as mean values with their standard errors. Differences were considered to be significant when p < 0.05.
Results
Antioxidative and oxidative status indicators in the ileum of newborn piglets
The results showed that the maternal HMSeBA diet significantly decreased the concentration of MDA in the ileum of newborn piglets compared with the control diet (p < 0.05, Fig. 1). Meanwhile, maternal HMSeBA supplementation tended to increase the ileal GSH-Px activity of newborn piglets compared to the control and Na2SeO3 groups (p = 0.063, Fig. 1).
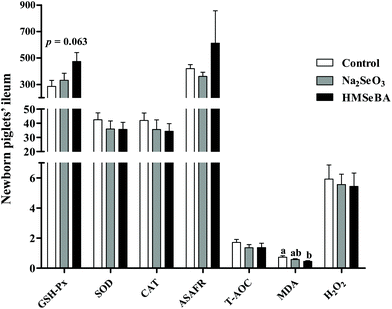 |
| Fig. 1 Effect of maternal Se supplementation during gestation on the ileal oxidative status of newborn piglets. GSH-Px (U mg−1 protein), glutathione peroxidase; SOD (U mg−1 protein), superoxide dismutase; CAT (U mg−1 protein), catalase; ASAFR (U g−1 protein), anti-superoxide anion radical; T-AOC (U mg−1 protein), total antioxidant capacity; MDA (nmol mg−1 protein), malondialdehyde; H2O2 (mmol g−1 protein), hydrogen peroxide. Mean values with standard errors are depicted by vertical bars. n = 10 for each group. Control, basal diet; Na2SeO3, basal diet supplemented with 0.3 mg Se per kg of Na2SeO3; and HMSeBA, basal diet supplemented with 0.3 mg Se per kg of HMSeBA. a,bColumns with different superscript letters indicate significant differences (p < 0.05). | |
Maternal organic Se supplementation changed the expression of selenoproteins and innate immune system related genes in the ileum of newborn piglets
In newborn piglets, maternal HMSeBA supplementation during gestation significantly upregulated the gene expression of GPX2, GPX3 and SePP1 in the ileum compared with the control and Na2SeO3 groups (p < 0.01, Fig. 2A). Meanwhile, the HMSeBA supplemented maternal diet significantly upregulated the expression of ileal SEPW1 compared with the Na2SeO3 group (p < 0.05, Fig. 2A).
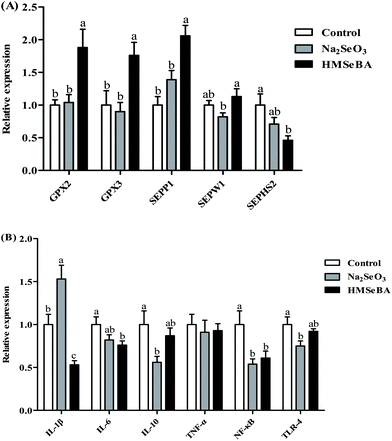 |
| Fig. 2 Effect of maternal Se supplementation during gestation on the expression of selenoprotein genes and innate immune system related genes in newborn piglets’ ileum. (A) Expression of selenoprotein genes. (B) Expression of innate immune system related genes. n = 10 for each group. Control, basal diet; Na2SeO3, basal diet supplemented with 0.3 mg Se per kg of Na2SeO3; and HMSeBA, basal diet supplemented with 0.3 mg Se per kg of HMSeBA. GPX, glutathione peroxidase; SEPP1, selenoprotein P; SEPW1, selenoprotein W; SEPHS2, selenophosphate synthetase 2; IL-1β, interleukin-1β; IL-6, interleukin-6; IL-10, interleukin-10; TNF-α, tumor necrosis factor-α; NF-κB, nuclear factor kappa B; TLR-4, toll-like receptor-4. Mean values with standard errors are depicted by vertical bars. a,b,cValues with different superscript letters for the same gene were significantly different (p < 0.05). | |
In the ileum of newborn piglets, maternal HMSeBA supplementation during gestation significantly downregulated the mRNA expression of IL-1β compared with the control and Na2SeO3 groups (p < 0.01, Fig. 2B). The maternal Na2SeO3 diet significantly downregulated ileal IL-10 and TLR-4 genes compared with the control group (p < 0.05, Fig. 2B). In addition, the HMSeBA group had a lower mRNA relative expression of ileal NF-κB and IL-6 genes than the control group (p < 0.05, Fig. 2B).
The expression of GPX2, NF-κB and ERK/mTOR/Beclin-1 pathway related proteins in the ileum of newborn piglets
Maternal HMSeBA supplementation significantly increased the protein abundance of ileal GPX2 and p-mTOR/mTOR in newborn piglets compared with the control and Na2SeO3 groups (p < 0.05, Fig. 3). Meanwhile, maternal HMSeBA supplementation significantly decreased the protein abundance of Beclin-1 and p-ERK/ERK in the ileum of newborn piglets (p < 0.05, Fig. 3). In addition, the protein abundance of ileal p-NF-κB in the HMSeBA group was lower than that in the control group (p < 0.05, Fig. 3).
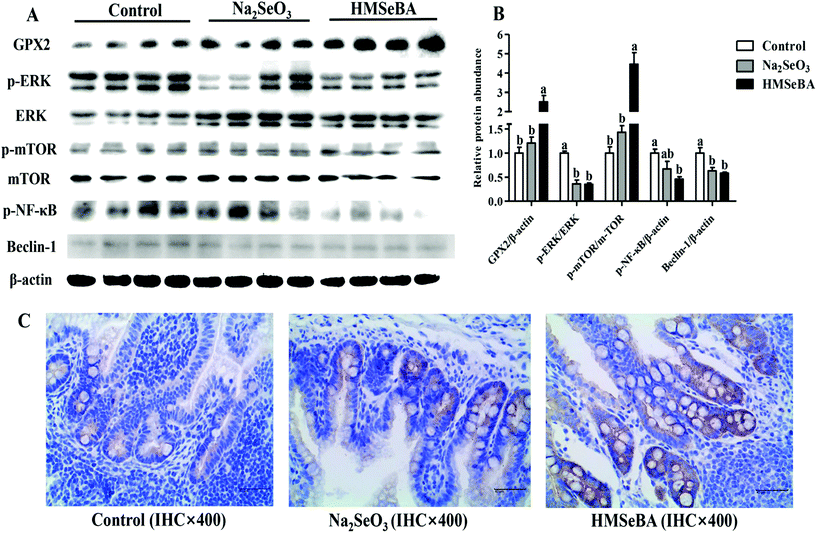 |
| Fig. 3 Effect of maternal Se supplementation during gestation on the GPX2, p-NF-κB and ERK/mTORC1/Beclin-1 signaling related protein levels in newborn piglets’ ileum. (A) and (B) Relative protein abundances of ERK/mTORC1/Beclin-1 signaling, GPX2 and p-NF-κB. (C) Immunohistochemistry of GPX2; the negative cells are represented in blue, the substrate is represented in white, the positive cells are represented in yellow or brown, and the GXP2 positive product is mainly distributed in the cytoplasm. Each set of data was normalized to the control group within an experiment. n = 4 for each group. Control, basal diet; Na2SeO3, basal diet supplemented with 0.3 mg Se per kg of Na2SeO3; and HMSeBA, basal diet supplemented with 0.3 mg Se per kg of HMSeBA. GPX2, glutathione peroxidase 2; ERK, extracellular signal regulated kinase; p-ERK, phosphor-extracellular signal regulated kinase; mTOR, mechanistic target of rapamycin; p-mTOR, phosphor-mechanistic target of rapamycin; p-NF-κB, phosphor-nuclear factor ‘kappa-light-chain-enhancer’ of the activated B-cells. Mean values with standard errors are depicted by vertical bars. a,bValues with different superscript letters for the same protein were significantly different (p < 0.05). | |
Oxidative status indicators in the ileum of weaned piglets after LPS challenge
In the ileum of weaned piglets, LPS challenge significantly increased the concentrations of MDA (p < 0.05) and H2O2 (p < 0.01). The activity of ileal GSH-Px was affected by the interaction of the diet and LPS challenge (p < 0.05) (Fig. 4). After LPS challenge, the piglets of the HMSeBA group showed a higher GSH-Px activity than the piglets of the control and Na2SeO3 groups. The ileal H2O2 concentration of the Na2SeO3 group after LPS challenge was higher than that in the piglets not treated with LPS (Fig. 4).
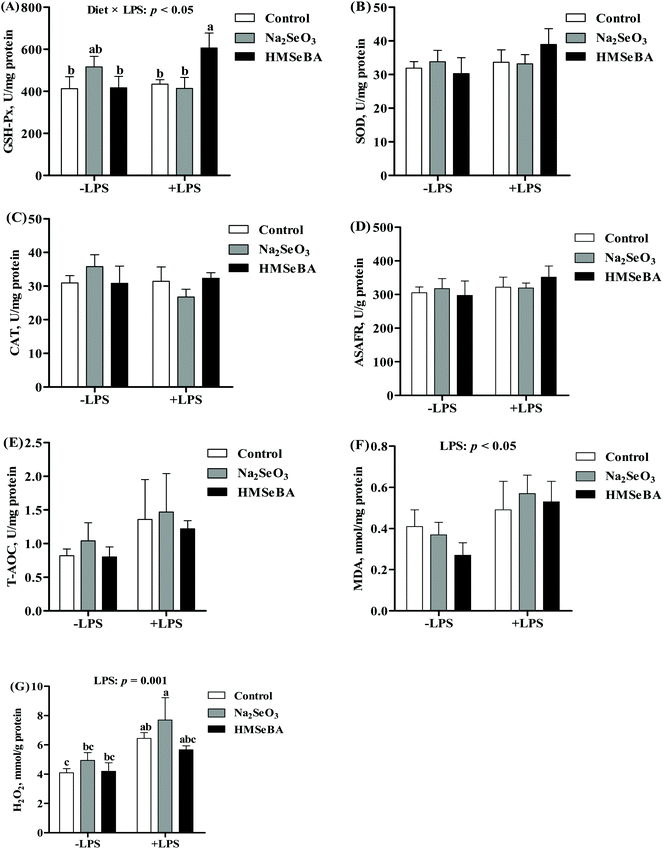 |
| Fig. 4 Effect of maternal Se supplementation during gestation on the ileal oxidative status of weaned piglets. (A) GSH-Px activity, (B) SOD activity, (C) CAT activity, (D) ASAFR activity, (E) T-AOC, (F) MDA, and (G) H2O2 in weaned pigs’ ileum. −LPS, piglets not challenged with LPS; +LPS, piglets challenged with LPS. GSH-Px, glutathione peroxidase; SOD, superoxide dismutase; CAT, catalase; ASAFR, anti-superoxide anion radical; T-AOC, total antioxidant capacity; MDA, malondialdehyde; H2O2, hydrogen peroxide. Mean values with standard errors are depicted by vertical bars, n = 6 for each group. Control, basal diet; Na2SeO3, basal diet supplemented with 0.3 mg Se per kg of Na2SeO3; and HMSeBA, basal diet supplemented with 0.3 mg Se per kg of HMSeBA. a,b,cColumns with different superscript letters indicate significant differences (p < 0.05). | |
The body temperature and serum inflammation factors of weaned piglets after LPS challenge
It has been reported that LPS challenge increased the body temperature and serum inflammation, and so we investigated the body temperature and serum inflammation factors of the piglets. The results showed that LPS challenge increased (p < 0.001) the rectal temperature at 2 h and 4 h post LPS treatment (ESI, Table 3†). However, the diet did not change the body temperature (ESI, Table 3†).
Further studies indicated that LPS challenge significantly affected the serum levels of IL-1β, IL-6 and IL-10 and the ratios serum TNF-α/IL-10 and IL-1β/IL-10 (p < 0.05, Fig. 5), while the maternal diet significantly changed the serum IL-1β and IL-10 concentrations and the ratios serum TNF-α/IL-10 and IL-1β/IL-10 (p < 0.05, Fig. 5) in the piglets. Besides, the interaction effect of diet × LPS on the serum levels of IL-β and IL-6 (p < 0.05, Fig. 5) was observed. LPS challenge increased serum IL-1β of the piglets in the control group; however, maternal HMSeBA supplementation reversed this effect. In addition, serum IL-6 of the piglets in the control group that were treated with LPS for 2 h was higher than that of the LPS untreated piglets in the control, Na2SeO3 or HMSeBA group. Meanwhile, IL-6 and IL-10 of the piglets in the control group that were treated with LPS for 4 h were higher than those of the LPS untreated piglets in the Na2SeO3 group. In the offsprings of control diet group, four-hour LPS challenge significantly decreased the ratio of serum TNF-α:IL-10 compared with the offsprings without LPS challenge. Whereas the ratio of serum TNF-α:IL-10 in the offsprings of Na2SeO3 and HMSeBA groups were not affected by LPS challenge as compared with the offsprings without LPS challenge. Moreover, maternal HMSeBA supplementation decreased the piglets’ serum IL-1β/IL-10 ratio at a 2 h-LPS challenge when compared to the Na2SeO3 group (Fig. 5).
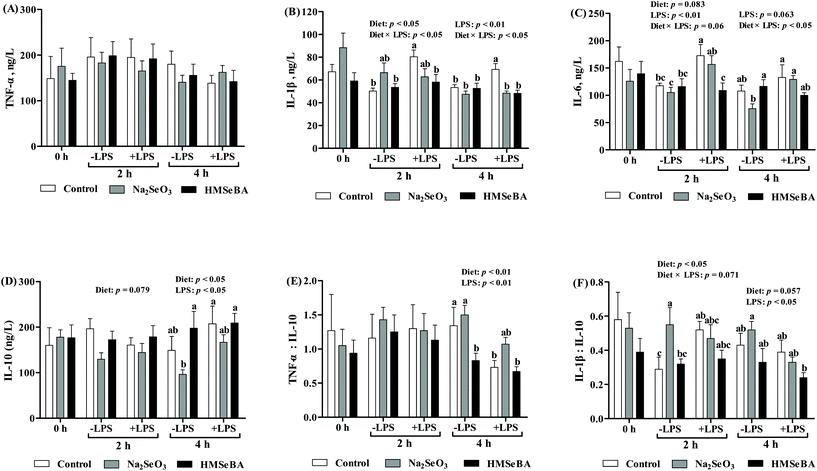 |
| Fig. 5 Effect of maternal Se supplementation during gestation on serum inflammatory cytokines of weaned piglets. −LPS, piglets not challenged with LPS; +LPS, piglets challenged with LPS. (A) TNF-α, (B) IL-1β, (C) IL-6, (D) IL-10, (E) the ratio of TNF-α to IL-10, and (F) the ratio of IL-1β to IL-10 in weaned piglets’ serum. TNF-α, tumor necrosis factor α; IL-1β, interleukin-1β; IL-6, interleukin-6; IL-10, and interleukin-10. Mean values with standard errors are depicted by vertical bars, n = 6 for each group. Control, basal diet; Na2SeO3, basal diet supplemented with 0.3 mg Se per kg of Na2SeO3; and HMSeBA, basal diet supplemented with 0.3 mg Se per kg of HMSeBA. a,b,cColumns with different superscript letters indicate significant differences (p < 0.05). | |
Maternal organic Se supplementation changed the expression of ileal antioxidant activity and innate immune system related genes of weaned piglets after LPS challenge
In weaned piglets, LPS challenge significantly affected the mRNA relative expression of ileal CAT, SOD2 and GPX3 (p < 0.05, p < 0.01 and p < 0.01, respectively, Fig. 6). The ileal SOD2 gene expression in the LPS treated piglets of the Na2SeO3 group was higher than that in the LPS untreated piglets of the control, Na2SeO3 and HMSeBA groups (Fig. 6). The ileal GPX3 gene expression in the LPS treated piglets of the HMSeBA group was higher than that in the LPS untreated piglets of the Na2SeO3 and HMSeBA groups (Fig. 6).
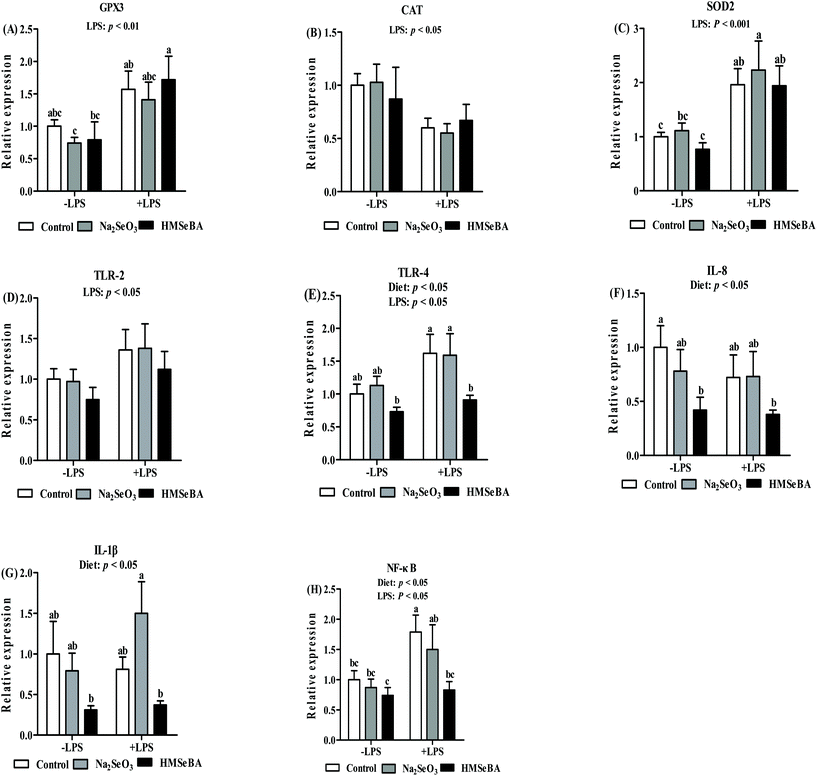 |
| Fig. 6 Effect of maternal Se supplementation during gestation on the expression of selenoprotein genes and innate immune system related genes in weaned piglets’ ileum. −LPS, piglets not challenged with LPS; +LPS, piglets challenged with LPS. (A) GPX3, (B) CAT, (C) SOD2, (D) TLR-2, (E) TLR-4, (F) IL-8, (G) IL-1β, and (H) NF-κB genes in weaned piglets’ ileum. Control, basal diet; Na2SeO3, basal diet supplemented with 0.3 mg Se per kg of Na2SeO3; and HMSeBA, basal diet supplemented with 0.3 mg Se per kg of HMSeBA. GPX3, glutathione peroxidase 3; CAT, catalase; SOD2, superoxide dismutase; TLR-2, toll-like receptor-2; TLR-4, toll-like receptor-4; IL-8, interleukin-8; IL-1β, interleukin-1β; NF-κB, nuclear factor kappa B. Mean values with standard errors are depicted by vertical bars. n = 6 for each group. a,b,cValues with different superscript letters for the same gene were significantly different (p < 0.05). | |
Besides, the maternal diet significantly affected the mRNA level of ileal TLR-4, IL-8 and IL-1β (p < 0.05, Fig. 6). LPS challenge significantly affected the mRNA abundance of TLR-2 and TLR-4 in the ileum (p < 0.05, Fig. 6). The ileal TLR-4 mRNA abundance in the LPS challenged and LPS non-challenged piglets of the HMSeBA group was lower than that in the LPS treated piglets of the control and Na2SeO3 groups. Meanwhile, the ileal IL-8 mRNA abundance in the LPS challenged and LPS non-challenged piglets of the HMSeBA group was lower than that in the piglets of the control group. In addition, the ileal IL-1β mRNA abundance in the LPS challenged and LPS non-challenged HMSeBA groups was lower than that in the LPS treated piglets of the Na2SeO3 group (Fig. 6).
The expression of NF-κB and ERK/Beclin-1 pathway related proteins in the ileum of weaned piglets after LPS challenge
The maternal diet significantly affected ileal p-ERK/ERK (p < 0.05) and p-NF-κB (p < 0.01) in weaned piglets (Fig. 7). LPS challenge significantly affected ileal p-ERK/ERK (p = 0.000) and p-NF-κB (p < 0.05) in weaned piglets (Fig. 7). The interaction between the maternal diet and LPS challenge significantly affected ileal p-ERK/ERK (p = 0.000), Beclin-1 (p = 0.000) and p-NF-κB (p = 0.000) in weaned piglets (Fig. 7). In addition, p-ERK/ERK was lower in the HMSeBA saline group compared with all diets of the LPS challenged pigs. The protein abundance of Beclin-1 and p-NF-κB in the LPS challenged HMSeBA group were lower than that in the HMSeBA saline, LPS challenged control and Na2SeO3 groups (Fig. 7).
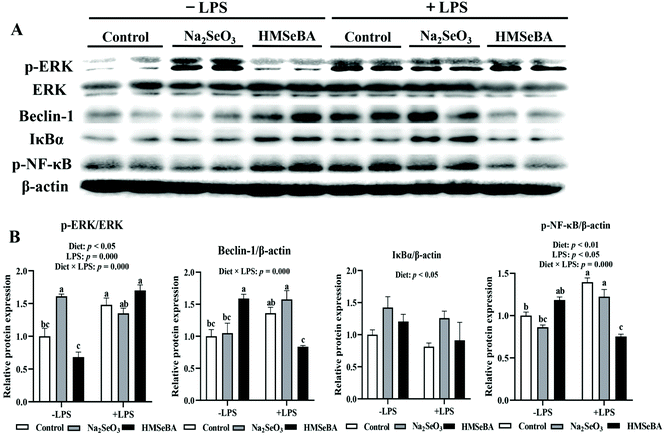 |
| Fig. 7 Effect of maternal Se supplementation during gestation on the expression of autophagy and NF-κB signaling related proteins in weaned piglets’ ileum. (A) Autophagy and NF-κB signaling related proteins determined by western blot. (B) The abundances of Beclin-1, IκBα and p-NF-κB were expressed in relation to that of β-actin; p-ERK/ERK, the ratio of p-ERK to ERK. Each set of data was normalized to the LPS non-challenged control group within an experiment. n = 4 for each group. Control, basal diet; Na2SeO3, basal diet supplemented with 0.3 mg Se per kg of Na2SeO3; and HMSeBA, basal diet supplemented with 0.3 mg Se per kg of HMSeBA. ERK, extracellular signal regulated kinase; IκBα, inhibitor of NF-κB; p-NF-κB, phosphor-nuclear factor kappa B. Mean values with standard errors are depicted by vertical bars. a,b,cValues with different superscript letters for the same gene were significantly different (p < 0.05). | |
Discussion
Our previous study found that maternal HMSeBA supplementation during gestation significantly increased the plasma selenium levels of sows and their offspring.20 Se is postulated to protect against inflammation in the gut by attenuating oxidative stress.12 In this study, maternal HMSeBA supplementation during gestation improved the antioxidant capacity and reduced the inflammation level in the intestine of offspring. This study provides new information about the role of maternal selenium supplementation in intestinal oxidative stress, inflammation and autophagy of the offspring, and suggested that maternal organic selenium supplementation regulates the intestinal health of the offspring.
Superoxide dismutase (SOD), catalase (CAT), and particularly glutathione peroxidases (GPXs) exert tremendous protective effects against intestinal epithelial injury and inflammation.7 In addition, MDA content is a biomarker of lipid peroxidation in the body.26 In the current study, maternal HMSeBA supplementation during gestation tended to increase the ileal and serum GSH-Px activity and significantly decreased the MDA concentration in the ileum of newborn piglets compared to the control group. In addition, the HMSeBA group showed a higher GPX2 gene expression and protein abundance in the ileum of newborn piglets than the control and Na2SeO3 groups. Liu et al.27 found that when the dietary Se and vitamin E levels increased the GPX2 mRNA and GPX activities increased linearly in the gut of pigs. Besides, a previous study also found that both GPX1 and GPX2 activities and the mRNA levels were significantly suppressed in the ileum of Se-deprived mice.28 On the other hand, some studies have revealed that dietary Se supplementation can inhibit an intestinal inflammatory reaction and maintain epithelial integrity in the intestine by suppressing the inflammatory related pathways, such as TNF-α- and NF-κB-dependent pathways.13 In this study, we also found that HMSeBA supplementation inhibited the activation of ileal NF-κB in newborn piglets, and lowered the expression of IL-1β and IL-6 compared with the control group. A previous study has revealed that deficiency of selenoproteins is associated with increased susceptibility to oxidative stress.29 In addition, oxidative stress can lead to the activation of NF-κB during inflammatory bowel diseases.30 Selenoproteins such as GPX2 and SePP1, which can reduce oxidative stress, could also decrease the activation of NF-κB.30 Similarly, a recent study has also shown that GPX2 is able to limit NF-κB signaling both in vivo and in vitro.31 Thus, maternal supplementation of HMSeBA during gestation might increase the intestinal antioxidant capacity of newborn piglets by increasing the expression of antioxidant-related selenoproteins, thereby further reducing the level of intestinal inflammation.
Autophagy plays an important role in regulating intestinal inflammation;8 hampered autophagy of the intestine results in inflammation and contributes to susceptibility to Crohn's disease.32 Besides, autophagy is closely related to antioxidant defence in the gastrointestinal tract.9 To test whether maternal HMSeBA supplementation regulates intestinal inflammation via autophagy, we studied ERK/mTOR/Beclin-1 signaling in neonatal piglets’ ileum. The results showed that maternal HMSeBA supplementation decreased the ratio p-ERK/ERK in newborn piglets’ ileum compared to the control group. A previous study has suggested that the activation of the ERK1/2 pathway resulted in the induction of autophagy.33 Depletion of ERK partially inhibited autophagy.34 Therefore, the inhibition of the ERK1/2 pathway may reduce autophagy. In addition, mTOR promotes anabolic metabolism and inhibits autophagy induction.35 Moreover, the inhibition of the mammalian target of rapamycin complex 1 (mTORC1) by sustained ERK activation caused a significant increase in Beclin-1 leading to cytodestructive autophagy.34 In the present study, we found that maternal HMSeBA supplementation increased the ratio p-mTORC1/mTORC1, while decreased the abundance of Beclin-1 in newborn piglets’ ileum, which ultimately inhibited autophagy. Kuma et al.36 reported that during embryogenesis the level of autophagy remains low, while it is immediately upregulated in various tissues after birth. Thus, the newborn piglets may be susceptible to autophagy, but maternal HMSeBA supplementation may inhibit autophagy by upregulating mTOR and downregulating ERK/Beclin-1. Similarly, Qian et al.37 found that SeMet (at 2, 4 and 6 μM) could block ochratoxin A-induced porcine circovirus type 2 replication promotion by inhibiting autophagy via the AKT/mTOR pathway. Zhang et al.38 showed that SeMet (1 μM) could inhibit the initiation of autophagy by activating the AKT/mTOR/p70S6K signaling pathway. However, Lee et al.39 found that Se (3 mg kg−1 day−1in vivo and ≥500 μM in vitro) inhibited mTORC1 activation. Another study has shown that Se (≥10 μM) promotes autophagic cell death by inhibiting mTOR activation.40 However, during co-treatment with Se (5 μM) and Cd (5 μM) Se protects PC12 cells from Cd-induced apoptosis by inhibiting Cd induced oxidative stress.40 These results indicate that a high level of selenium can inhibit mTOR activation, but appropriate selenium levels can activate mTOR and can inhibit autophagy in this way. Besides, our study showed that maternal HMSeBA supplementation decreased the ratio of p-ERK/ERK in newborn piglets' ileum, while a previous study reported that inhibition of ERK1/2 phosphorylation protected lymphocytes from H2O2 induced cell death.41 In the present study, maternal HMSeBA supplementation improved the antioxidative capacity of offspring. Thus, maternal HMSeBA supplementation could inhibit the initiation of autophagy in newborn piglets’ ileum by decreasing the production of ROS. Taken together, maternal HMSeBA supplementation could reduce ileal inflammation in newborn piglets by regulating mTOR, ERK/Beclin-1 and GPX2.
Early life nutrition has long-term effects on later health.42 Our previous studies have shown that the maternal nutrition levels or the supplementation of nutrients could affect the intestinal health of weaned piglets.4,5 The second objective of this study was to determine the potential effect of maternal HMSeBA on the redox state and immune responses of the LPS-challenged piglets. Consistent with a previous study,5 we found that LPS challenge increased the body temperature and serum inflammatory cytokine concentration in weaned piglets. LPS is a powerful inflammatory inducer and has been used to mimic bacterial infection experimentally in farm animals.43 LPS challenge can activate the immune systems through the TLR-4-MyD88-NF-κB signaling pathway.5 The physiological action of LPS is to increase the secretion of cytokines and to increase the body temperature.43 Cytokines, including proinflammatory cytokines (such as TNF-α and IL-6) and anti-inflammatory cytokines (such as IL-10 and TGF-β), play crucial roles in the modulation of the inflammatory response in the gastrointestinal tract.44 In this study, the LPS treated piglets of the HMSeBA group had lower serum IL-1β and IL-6 compared with the LPS challenged control group. This result was similar to that from previous studies showing that Se addition exhibited more protective effects against inflammatory bowel diseases and experimental colitis in rodents than the inadequate Se supplementation.45 On the other hand, LPS can induce ROS generation through the TLR-4 interaction with NAD(P)H oxidase 4 (NOX4).46 In the present study, LPS challenge significantly increased the expression of the TLR-4 gene and the concentration of H2O2 in weaned piglets’ ileum. Meanwhile, the LPS challenged piglets of the HMSeBA group showed a higher GSH-Px activity in the ileum than the control group. Previous studies found that SOD1, SOD2, glutathione peroxidase (particularly intestinal GPx2), and CAT adaptively increased in the inflamed mucosa of patients with Crohn's disease and ulcerative colitis.47 Our results also showed that LPS challenge increased weaned piglets’ ileal H2O2 concentration and SOD2 expression in the LPS challenged Na2SeO3 group. However, the upregulation of SOD2, SOD1, GPX2, and CAT that occurs in the intestinal mucosa during inflammatory bowel diseases does not cope with O2˙− and H2O2 generated by TNF-α-induced NOX1, together with NOX2 from the activated leukocytes.7 In the current study, LPS challenge increased the ROS and inflammatory cytokine levels in the offspring of the control and Na2SeO3 groups, while the addition of HMSeBA improved the intestinal antioxidant capacity and reduced the level of inflammation in weaned piglets. Thus, maternal HMSeBA supplementation during gestation has protective effects on LPS-induced intestinal inflammation and oxidative stress.
Similar to the NF-κB pathway, the pattern recognition receptors (such as TLRs and NLRs) can activate mitogen-activated protein kinase (MAPK) subfamilies (ERK, p38 and JNK), and in conjunction with the activation of NF-κB, MAPK activation induces the expression of inflammatory response related genes.48 LPS can be used as an inflammatory agent to identify autophagy in intestinal inflammation and oxidative stress.19 In order to further determine the role of HMSeBA in intestinal autophagy and inflammation, we also analyzed the ERK/Beclin-1 pathway after the LPS challenge in weaned piglets. LPS challenge increased the ERK phosphorylation level, whereas maternal HMSeBA supplementation decreased it. On the other hand, after LPS challenge, the HMSeBA group had lower protein expression of Beclin-1. Vunta et al.49 showed that supplementation of Se to macrophages (Se-deficient) leads to a significant decrease in the LPS-induced expression of cyclooxygenase-2 and TNF-α via the inhibition of the MAPK/ERK pathways. However, as described by Xiao et al.,50 the role of MAPKs (ERK, p38 and JNK) is controversial. Some studies have found that oxidative stress contributes to the activation of MAPK/ERK,51 while some studies have reported that the activation of MAPK/ERK could improve oxidative stress.50,52 Activation of MAPK/ERK by oxidative stress may be related to stress levels and autophagy. Besides, maternal HMSeBA supplementation increased the antioxidation ability after LPS challenge in weaned piglets. Therefore, although LPS challenge increased the ERK phosphorylation level, maternal HMSeBA decreased Beclin-1 after LPS challenge. That is to say, maternal HMSeBA supplementation during gestation had a long-term protective effect on intestinal inflammation and autophagy.
In conclusion, maternal HMSeBA supplementation during gestation improves the intestinal antioxidant capacity, while reducing the intestinal inflammation level of piglets by suppressing the NF-κB and ERK/Beclin-1 pathways, and further protects weaned piglets from LPS-induced intestinal inflammation. Therefore, maternal HMSeBA supplementation can improve the intestinal health of offspring. Our study proved that maternal HMSeBA supplementation during pregnancy can improve the intestinal function and health of newborn piglets, and this maternal effect on piglets can last until weaning. In addition, this study also elucidated the mechanism of maternal selenium supplementation regulating the intestinal antioxidant capacity and inflammation of offspring. In future studies, the long-term effect of maternal HMSeBA supplementation during gestation on the intestinal health and growth performance of adult offspring and the exact mechanism of maternal HMSeBA supplementation will be explored.
Funding
This work was supported by the National Key Research and Development Program of China (2017YFD0500503). B. F. was a recipient of the Initial Research Funding from Sichuan Agricultural University and the Thousand Talent Program from Sichuan Province.
Author contributions
The authors’ responsibilities were as follows: B. F., D. M. and D. W. designed the study; D. M., D. D., M. Y., X. J., and L. Z. conducted the research; L. C., Z. F., S. X., Y. L., J. L., C. H., Y. Z., and L. L. analyzed the data; D. M. wrote the manuscript; B. F. and D. W. revised the manuscript; B. F. had the primary responsibility for the final content; and all authors read and approved the final manuscript.
Conflicts of interest
The authors declare no competing financial interest.
Acknowledgements
We thank Adisseo France S.A.S. for kindly providing HMSeBA.
References
- L. A. Drozdowski, T. Clandinin and A. B. R. Thomson, Ontogeny, growth and development-of the small intestine: Understanding pediatric gastroenterology, World J. Gastroenterol., 2010, 16, 787–799 CAS.
- M. Cao, L. Q. Che, J. Wang, M. Yang, G. Q. Su, Z. F. Fang, Y. Lin, S. Y. Xu and D. Wu, Effects of maternal over- and undernutrition on intestinal morphology, enzyme activity, and gene expression of nutrient transporters in newborn and weaned pigs, Nutrition, 2014, 30, 1442–1447 CrossRef CAS.
- P. L. Liu, L. Che, Z. G. Yang, B. Feng, L. Q. Che, S. Y. Xu, Y. Lin, Z. F. Fang, J. Li and D. Wu, A Maternal High-Energy Diet Promotes Intestinal Development and Intrauterine Growth of Offspring, Nutrients, 2016, 8, 258 CrossRef.
- H. Liu, J. Wang, D. L. Mou, L. Q. Che, Z. F. Fang, B. Feng, Y. Lin, S. Y. Xu, J. Li and D. Wu, Maternal Methyl Donor Supplementation during Gestation Counteracts the Bisphenol A-Induced Impairment of Intestinal Morphology, Disaccharidase Activity, and Nutrient Transporters Gene Expression in Newborn and Weaning Pigs, Nutrients, 2017, 9, 423 CrossRef.
- Y. L. Chen, D. L. Mou, L. Hu, J. Zhen, L. Q. Che, Z. F. Fang, S. Y. Xu, Y. Lin, B. Feng, J. Li and D. Wu, Effects of Maternal Low-Energy Diet during Gestation on Intestinal Morphology, Disaccharidase Activity, and Immune Response to Lipopolysaccharide Challenge in Pig Offspring, Nutrients, 2017, 9, 1115 CrossRef.
- C. Pietro, V. Viviane, P. C. Estefania, S. Jerome and K. Anna-Maria, Biomarkers of gastrointestinal functionality in animal nutrition and health, Anim. Feed Sci. Technol., 2019, 250, 9–31 CrossRef.
- S. Perez, R. Talens-Visconti, S. Rius-Perez, I. Finamor and J. Sastre, Redox signaling in the gastrointestinal tract, Free Radical Biol. Med., 2017, 104, 75–103 CrossRef CAS.
- T. Schwerd, S. Pandey, H. T. Yang, K. Bagola, E. Jameson, J. Jung, R. H. Lachmann, N. Shah, S. Y. Patel, C. Booth, H. Runz, G. Duker, R. Bettels, M. Rohrbach, S. Kugathasan, H. Chapel, S. Keshav, A. Elkadri, N. Platt, A. M. Muise, S. Koletzko, R. J. Xavier, T. Marquardt, F. Powrie, J. E. Wraith, M. Gyrd-Hansen, F. M. Platt and H. H. Uhlig, Impaired antibacterial autophagy links granulomatous intestinal inflammation in Niemann-Pick disease type C1 and XIAP deficiency with NOD2 variants in Crohn's disease, Gut, 2017, 66, 1060–1073 CrossRef CAS.
- J. Lee, S. Giordano and J. H. Zhang, Autophagy, mitochondria and oxidative stress: cross-talk and redox signalling, Biochem. J., 2012, 441, 523–540 CrossRef CAS.
- R. Scherz-Shouval, E. Shvets, E. Fass, H. Shorer, L. Gil and Z. Elazar, Reactive oxygen species are essential for autophagy and specifically regulate the activity of Atg4, EMBO J., 2007, 26, 1749–1760 CrossRef CAS.
- L. V. Papp, J. Lu, A. Holmgren and K. K. Khanna, From selenium to selenoproteins: Synthesis, identity, and their role in human health, Antioxid. Redox Signaling, 2007, 9, 775–806 CrossRef CAS.
- S. P. Short, J. M. Pilat and C. S. Williams, Roles for selenium and selenoprotein P in the development, progression, and prevention of intestinal disease, Free Radical Biol. Med., 2018, 127, 26–35 CrossRef CAS.
- C. W. Barrett, K. Singh, A. K. Motley, M. K. Lintel, E. Matafonova, A. M. Bradley, W. Ning, S. V. Poindexter, B. Parang, V. K. Reddy, R. Chaturvedi, B. M. Fingleton, M. K. Washington, K. T. Wilson, S. S. Davies, K. E. Hill, R. F. Burk and C. S. Williams, Dietary Selenium Deficiency Exacerbates DSS-Induced Epithelial Injury and AOM/DSS-Induced Tumorigenesis, PLoS One, 2013, 8, e67845 CrossRef CAS.
- C. Zhu, S. Zhang, C. Song, Y. Zhang, Q. Ling, P. R. Hoffmann, J. Li, T. Chen, W. Zheng and Z. Huang, Selenium nanoparticles decorated with Ulva lactuca polysaccharide potentially attenuate colitis by inhibiting NF-kappaB mediated hyper inflammation, J. Nanobiotechnol., 2017, 15, 20 CrossRef.
- S. K. Nettleford and K. S. Prabhu, Selenium and Selenoproteins in Gut Inflammation-A Review, Antioxidants, 2018, 7, 36 CrossRef.
- H. Vunta, F. Davis, U. D. Palempalli, D. Bhat, R. J. Arner, J. T. Thompson, D. G. Peterson, C. C. Reddy and K. S. Prabhu, The anti-inflammatory effects of selenium are mediated through 15-deoxy-Delta(12,14)-prostaglandin J(2) in macrophages, J. Biol. Chem., 2007, 282, 17964–17973 CrossRef CAS.
- S. Wang, Z. Xu, H. Yin, Y. H. Min and S. Li, Alleviation Mechanisms of Selenium on Cadmium-Spiked in Chicken Ovarian Tissue: Perspectives from Autophagy and Energy Metabolism, Biol. Trace Elem. Res., 2018, 186, 521–528 CrossRef CAS.
- M. M. Rahman, R. A. Uson-Lopez, M. T. Sikder, G. X. Tan, T. Hosokawa, T. Saito and M. Kurasaki, Ameliorative effects of selenium on arsenic-induced cytotoxicity in PC12 cells via modulating autophagy/apoptosis, Chemosphere, 2018, 196, 453–466 CrossRef CAS.
- M. Zhou, W. Xu, J. Wang, J. Yan, Y. Shi, C. Zhang, W. Ge, J. Wu, P. Du and Y. Chen, Boosting mTOR-dependent autophagy via upstream TLR4-MyD88-MAPK signalling and downstream NF-kappaB pathway quenches intestinal inflammation and oxidative stress injury, EBioMedicine, 2018, 35, 345–360 CrossRef.
- D. Mou, D. Ding, H. Yan, B. Qin, Y. Dong, Z. Li, L. Che, Z. Fang, S. Xu, Y. Lin, Y. Zhuo, J. Li, C. Huang, Y. Zou, L. Li, M. Briens, D. Wu and B. Feng, Maternal supplementation of organic selenium during gestation improves sows and offspring antioxidant capacity and inflammatory status and promotes embryo survival, Food Funct., 2020, 11, 7748–7761 RSC.
- D. Mou, D. Ding, S. Li, H. Yan, B. Qin, Z. Li, L. Zhao, L. Che, Z. Fang, S. Xu, L. Yan, Y. Zhuo, J. Li, C. Huang, Y. Zou, L. Li, M. Briens, D. Wu and B. Feng, Effect of maternal organic selenium supplementation during pregnancy on sow reproductive performance and long-term effect on their progeny, J. Anim. Sci., 2020 DOI:10.1093/jas/skaa366.
- D. Mou, J. Wang, H. Liu, Y. Chen, L. Che, Z. Fang, S. Xu, Y. Lin, B. Feng, J. Li and D. Wu, Maternal methyl donor supplementation during gestation counteracts bisphenol A-induced oxidative stress in sows and offspring, Nutrition, 2018, 45, 76–84 CrossRef CAS.
- B. Feng, P. Jiao, Y. Helou, Y. Li, Q. He, M. S. Walters, A. Salomon and H. Xu, Mitogen-activated protein kinase phosphatase 3 (MKP-3)-deficient mice are resistant to diet-induced obesity, Diabetes, 2014, 63, 2924–2934 CrossRef.
- J. Chen, B. Yu, D. Chen, Z. Huang, X. Mao, P. Zheng, J. Yu, J. Luo and J. He, Chlorogenic acid improves intestinal barrier functions by suppressing mucosa inflammation and improving antioxidant capacity in weaned pigs, J. Nutr. Biochem., 2018, 59, 84–92 CrossRef CAS.
- H. J. Zhong, Y. M. Song, P. Wang, B. Feng, X. L. Zhang, L. Q. Che, Y. Lin, S. Y. Xu, J. Li, D. Wu and Z. F. Fang, Mammary Protein Synthesis upon Long-Term Nutritional Restriction Was Attenuated by Oxidative-Stress-Induced Inhibition of Vacuolar H+-Adenosine Triphosphatase/Mechanistic Target of Rapamycin Complex 1 Signaling, J. Agric. Food Chem., 2019, 67, 8950–8957 CrossRef CAS.
- C. S. Liu, N. H. Chen and J. T. Zhang, Protection of PC12 cells from hydrogen peroxide-induced cytotoxicity by salvianolic acid B, a new compound isolated from Radix Salviae miltiorrhizae, Phytomedicine, 2007, 14, 492–497 CrossRef CAS.
- F. Liu, J. J. Cottrell, J. B. Furness, L. R. Rivera, F. W. Kelly, U. Wijesiriwardana, R. V. Pustovit, L. J. Fothergill, D. M. Bravo, P. Celi, B. J. Leury, N. K. Gabler and F. R. Dunshea, Selenium and vitamin E together improve intestinal epithelial barrier function and alleviate oxidative stress in heat-stressed pigs, Exp. Physiol., 2016, 101, 801–810 CrossRef CAS.
- R. S. Esworthy, L. Yang, P. H. Frankel and F. F. Chu, Epithelium-specific glutathione peroxidase, Gpx2, is involved in the prevention of intestinal inflammation in selenium-deficient mice, J. Nutr., 2005, 135, 740–745 CrossRef CAS.
- C. W. Barrett, V. K. Reddy, S. P. Short, A. K. Motley, M. K. Lintel, A. M. Bradley, T. Freeman, J. Vallance, W. Ning, B. Parang, S. V. Poindexter, B. Fingleton, X. Chen, M. K. Washington, K. T. Wilson, N. F. Shroyer, K. E. Hill, R. F. Burk and C. S. Williams, Selenoprotein P influences colitis-induced tumorigenesis by mediating stemness and oxidative damage, J. Clin. Invest., 2015, 125, 2646–2660 CrossRef.
- A. K. Kudva, A. E. Shay and K. S. Prabhu, Selenium and inflammatory bowel disease, Am. J. Physiol.: Gastrointest. Liver Physiol., 2015, 309, G71–G77 CrossRef CAS.
- S. C. Koeberle, A. Gollowitzer, J. Laoukili, O. Kranenburg, O. Werz, A. Koeberle and A. P. Kipp, Distinct and overlapping functions of glutathione peroxidases 1 and 2 in limiting NF-kappa B-driven inflammation through redox-active mechanisms, Redox Biol., 2020, 28, 101388 CrossRef CAS.
- C. Strisciuglio, M. Duijvestein, A. P. Verhaar, A. C. W. Vos, G. R. van den Brink, D. W. Hommes and M. E. Wildenberg, Impaired autophagy leads to abnormal dendritic cell-epithelial cell interactions, J. Crohns Colitis, 2013, 7, 534–541 CrossRef.
- H. Aoki, Y. Takada, S. Kondo, R. Sawaya, B. B. Aggarwal and Y. Kondo, Evidence that curcumin suppresses the growth of malignant gliomas in vitro and in vivo through induction of autophagy: Role of Akt and extracellular signal-regulated kinase signaling pathways, Mol. Pharmacol., 2007, 72, 29–39 CrossRef CAS.
- J. R. Wang, M. W. Whiteman, H. Q. Lian, G. X. Wang, A. Singh, D. Y. Huang and T. Denmark, A Non-canonical MEK/ERK Signaling Pathway Regulates Autophagy via Regulating Beclin 1, J. Biol. Chem., 2009, 284, 21412–21424 CrossRef CAS.
- Y. C. Kim and K. L. Guan, mTOR: a pharmacologic target for autophagy regulation, J. Clin. Invest., 2015, 125, 25–32 CrossRef.
- A. Kuma, M. Hatano, M. Matsui, A. Yamamoto, H. Nakaya, T. Yoshimori, Y. Ohsumi, T. Tokuhisa and N. Mizushima, The role of autophagy during the early neonatal starvation period, Nature, 2004, 432, 1032–1036 CrossRef CAS.
- G. Qian, D. Liu, J. Hu, F. Gan, L. Hou, N. Zhai, X. Chen and K. Huang, SeMet attenuates OTA-induced PCV2 replication promotion by inhibiting autophagy by activating the AKT/mTOR signaling pathway, Vet. Res., 2018, 49, 15 CrossRef.
- Z. H. Zhang, Q. Y. Wu, C. Chen, R. Zheng, Y. Chen, Q. Liu, J. Z. Ni and G. L. Song, Selenomethionine Attenuates the Amyloid-beta Level by Both Inhibiting Amyloid-beta Production and Modulating Autophagy in Neuron-2a/A beta PPswe Cells, J. Alzheimer's Dis., 2017, 59, 591–602 CAS.
- Y. K. Lee, S. Y. Park, Y. M. Kim, D. C. Kim, W. S. Lee, Y. J. Surh and O. J. Park, Suppression of mTOR via Akt-dependent and -independent mechanisms in selenium-treated colon cancer cells: involvement of AMPKalpha1, Carcinogenesis, 2010, 31, 1092–1099 CrossRef CAS.
- K. F. Binte Hossain, M. M. Rahman, M. T. Sikder, T. Saito, T. Hosokawa and M. Kurasaki, Inhibitory effects of selenium on cadmium-induced cytotoxicity in PC12 cells via regulating oxidative stress and apoptosis, Food Chem. Toxicol., 2018, 114, 180–189 CrossRef CAS.
- A. A. Akhiani, O. Werlenius, J. Aurelius, C. Movitz, A. Martner, K. Hellstrand and F. B. Thoren, Role of the ERK pathway for oxidant-induced parthanatos in human lymphocytes, PLoS One, 2014, 9, e89646 CrossRef.
- B. Koletzko, B. Brands, L. Poston, K. Godfrey, H. Demmelmair and E. N. Project, Early nutrition programming of long-term health, Proc. Nutr. Soc., 2012, 71, 371–378 CrossRef.
- D. I. Adewole, I. H. Kim and C. M. Nyachoti, Gut Health of Pigs: Challenge Models and Response Criteria with a Critical Analysis of the Effectiveness of Selected Feed Additives - A Review, Asian-Australas. J. Anim. Sci., 2016, 29, 909–924 CrossRef CAS.
- M. Sun, C. He, Y. Cong and Z. Liu, Regulatory immune cells in regulation of intestinal inflammatory response to microbiota, Mucosal Immunol., 2015, 8, 969–978 CrossRef CAS.
- Q. X. Zhai, Y. Xiao, P. Li, F. W. Tian, J. X. Zhao, H. Zhang and W. Chen, Varied doses and chemical forms of selenium supplementation differentially affect mouse intestinal physiology, Food Funct., 2019, 10, 5398–5412 RSC.
- H. S. Park, H. Y. Jung, E. Y. Park, J. Kim, W. J. Lee and Y. S. Bae, Cutting edge: direct interaction of TLR4 with NAD(P)H oxidase 4 isozyme is essential for lipopolysaccharide-induced production of reactive oxygen species and activation of NF-kappa B, J. Immunol., 2004, 173, 3589–3593 CrossRef CAS.
- L. Kruidenier, I. Kuiper, W. van Duijn, S. L. Marklund, R. A. van Hogezand, C. B. Lamers and H. W. Verspaget, Differential mucosal expression of three superoxide dismutase isoforms in inflammatory bowel disease, J. Pathol., 2003, 201, 7–16 CrossRef CAS.
- J. S. Arthur and S. C. Ley, Mitogen-activated protein kinases in innate immunity, Nat. Rev. Immunol., 2013, 13, 679–692 CrossRef CAS.
- H. Vunta, B. J. Belda, R. J. Arner, C. Channa Reddy, J. P. Vanden Heuvel and K. Sandeep Prabhu, Selenium attenuates pro-inflammatory gene expression in macrophages, Mol. Nutr. Food Res., 2008, 52, 1316–1323 CrossRef CAS.
- X. Xiao, Y. Cheng, D. Song, X. Li, Y. Hu, Z. Lu, F. Wang and Y. Wang, Selenium-enriched Bacillus paralicheniformis SR14 attenuates H2O2-induced oxidative damage in porcine jejunum epithelial cells via the MAPK pathway, Appl. Microbiol. Biotechnol., 2019, 103, 6231–6243 CrossRef CAS.
- W. Rui, L. F. Guan, F. Zhang, W. Zhang and W. J. Ding, PM2.5-induced oxidative stress increases adhesion molecules expression in human endothelial cells through the ERK/AKT/NF-kappa B-dependent pathway, J. Appl. Toxicol., 2016, 36, 48–59 CrossRef CAS.
- C. Borras, J. Gambini, M. C. Gomez-Cabrera, J. Sastre, F. V. Pallardo, G. E. Mann and J. Vina, 17beta-oestradiol up-regulates longevity-related, antioxidant enzyme expression via the ERK1 and ERK2[MAPK]/NFkappaB cascade, Aging Cell, 2005, 4, 113–118 CrossRef CAS.
Footnotes |
† Electronic supplementary information (ESI) available. See DOI: 10.1039/d0fo02274h |
‡
These authors contributed equally to this work.
|
|
This journal is © The Royal Society of Chemistry 2021 |
Click here to see how this site uses Cookies. View our privacy policy here.