DOI:
10.1039/D0FO02122A
(Review Article)
Food Funct., 2021,
12, 41-56
The effect of black tea supplementation on blood pressure: a systematic review and dose–response meta-analysis of randomized controlled trials†
Received
11th August 2020
, Accepted 3rd November 2020
First published on 16th November 2020
Abstract
The main goal of this work was to clarify the effects of black tea supplementation on blood pressure (BP) by performing a systematic review according to the PRISMA (preferred reporting items for systematic reviews and meta-analyses) guidelines, followed by a dose–response meta-analysis of randomized controlled trials (RCTs). Electronic search was carried out in PubMed, Embase and the Cochrane Library databases published up to March 2020. To be included, RCTs had to report the effect of black tea supplementation on systolic blood pressure (SBP) or diastolic blood pressure (DBP) in adults. A total of 13 trials, including 22 study arms were eligible for inclusion in the final quantitative analysis. It was observed that black tea supplementation significantly reduced SBP (WMD – 1.04 mmHg; 95% CI – 2.05 to −0.03; and P = 0.04) and DBP (WMD – 0.59 mmHg; 95% CI – 1.05 to −0.13; and P = 0.01) compared to the control. However, nonlinear analysis failed to indicate a significant influence of black tea flavonoid supplementation dose or duration on both SBP and DBP. Sensitivity analysis showed that no individual study had a significant impact on our results. In addition, we found no evidence for the presence of small-study effects among studies for both SBP and DBP. Thus, the favorable effect of black tea supplementation emerging from the current meta-analysis suggests the possible use of this tea as an active compound in order to promote cardiovascular health, mostly when used for longer duration (>7 days) and in men. Furthermore RCTs using different doses of black tea and various durations may contribute to confirming our conclusion.
Introduction
The leading causes of death and morbidity worldwide are noncommunicable diseases, in particular, cardiovascular disease (CVD).1 Hypertension is a major risk factor for CVD and is strongly linked to ischemic heart disease, cerebrovascular disease, and chronic kidney disease, with a worldwide prevalence exceeding 1 billion.2,3 Furthermore hypertension is recognized as one of the leading risk factors for the global disease burden.4,5 In 2010, 1.4 billion people globally had hypertension, and it was anticipated that until 2025, hypertension would affect as many as 1.6 billion individuals in the world.6 Studies have shown that a 10 mmHg reduction in systolic blood pressure (SBP) reduced the risk of major cardiovascular disease events by 20%, and even small changes in BP caused by dietary modification might have a significant impact on the onset of CVD and stroke.7,8 Thus, even a relatively small reduction in population blood pressure can be considered a priority to the healthcare system.
Hypertension is affected by changes in a population's diets and lifestyle.9,10 It has been shown that specific nutrients help in lowering blood pressure.11 Tea is produced from the leaves of Camellia sinensis, and is the second most consumed beverage in the world after water.12 Traditionally, there are three types of tea: green tea (unfermented), oolong tea (partially fermented), and black tea (fully fermented),13 among which the main type produced and consumed worldwide is black tea. Black tea is rich in flavanols, making it a major contributor to the intake of flavanol.14 Numerous studies have shown the antihypertensive effects of black tea in different preclinical models of hypertension. In previous reports, it has been indicated that administration of black tea attenuated the blood pressure levels and endothelial dysfunction in rats.15,16 The mechanisms underlying the beneficial effects of black tea are mainly attributed to a class of polyphenols known as flavonoids.17 Moreover, the results of population studies indicate that a higher intake of black tea can protect against hypertension and cardiovascular disease.17,18 All these effects may promote BP reduction and improve BP control in hypertensive patients in a setting of clinical practice.
Today, available studies report conflicting data, probably due to their heterogeneous methods and the fact that they were small-scale RCTs. While some clinical trials revealed some beneficial effects of black tea supplementation on blood pressure,19,20 others failed to find such an influence.21,22 In the present study, we performed a systematic review and a dose–response meta-analysis of randomized-controlled trials to summarize the effects of black tea supplementation on blood pressure in adults.
Methods
This meta-analysis, addressing the effect of black tea supplementation on blood pressure, was performed according to the preferred reporting items for systematic reviews and meta-analyses (PRISMA) guidelines.23
The protocol for this systematic review was registered on INPLASY and is available in full on inplasy.com (https://doi.org/10.37766/inplasy00000000). Besides, the review protocol was also registered in PROSPERO, the International Prospective Register of Systematic Reviews (unique identifier: CRD42020185670).
Search strategy
A literature search was performed using defined databases (PubMed, Embase, and the Cochrane Library) to identify relevant studies published from the index date of each database to March 2020. Titles and abstracts in the medical subject headings (MeSH) and related keywords were searched for the following terms: black tea, flavonoid(s), tea extract(s), tea component(s), tea solid(s), camellia sinensis, blood pressure, hypertension, hypertensive, hypotension, hypotensive, endothelium, endothelial function, and endothelial dysfunction. Language was restricted to English. References in all relevant reviews were also checked for additional studies that might be missed in our search. Only the most informative or most recent publication was used when there were several publications from the same trial.
Inclusion criteria
Studies were included if they: (1) were randomized controlled trials (RCTs) with either parallel or crossover designs; (2) included populations aged ≥18 years; (3) examined the effects of black tea supplementation on SBP or DBP as the primary or secondary outcomes with a control group; (4) reported mean/median and standard deviation (SD) or other available data (95% confidence interval, interquartile range, standard errors, and ranges) on SBP or DBP.
Exclusion criteria
Observational (cohort, case-control, and cross-sectional) studies, reviews, animal studies, in vitro studies, and those that were conducted on children, adolescents and pregnant or lactating women were not included. Studies without randomized allocation, trials that assessed the effect of black tea in combination with other interventions, studies with insufficient data, studies with citations that did not assess SBP or DBP, articles that reported the findings of the same trial, and publications in languages other than English were excluded. In addition, book chapters, editorials, grey literature, interviews, conference abstracts, comments, methodological papers, opinion pieces, and letters were not included in this meta-analysis.
Data extraction
Two reviewers (Chang Ma and Xuehui Zheng) independently extracted the following data from eligible published papers: first author's name, publication year, study design (parallel or cross-over), number of subjects in the black tea and placebo groups, duration of intervention, country/region, mean age, gender and body mass index (BMI) of participants, participant health status, BP medication, ambulatory BP, tea group, control group, results and risk of bias. To minimize possible errors, data were cross-checked and disagreements were resolved by discussion with the corresponding author (Peili Bu). Studies with a longer duration of intervention were chosen for inclusion in the meta-analysis over studies with a short duration on the same population.
Risk of bias
The quality of eligible publications was assessed using the Cochrane risk of bias tool for RCTs (Table 1).24 Two independent investigators (Chang Ma and Xuehui Zheng) completed this checklist for each included paper. Methodological features applied for assessment were: (a) adequate sequence generation, (b) allocation concealment, (c) blinding of participants and personnel, (d) blinding of outcome assessment, (e) incomplete outcome data, (f) selective outcome reporting (reporting bias), and (g) other potential sources of bias. Based on the above-mentioned features, studies were classified in terms of bias into three groups: low risk, unclear risk, and high risk of bias.24
Table 1 The basic characteristics of the studies included in the meta-analysis
References |
Design |
Size (tea/placebo) |
Duration of intervention |
Country |
Mean age (year) |
Gender (male/female) |
BMI |
Participant health status |
BP medication |
Ambulatory BP |
Tea group |
Placebo group |
Results |
Risk of bias |
Ahmad (2018)21 |
Randomized, placebo-controlled, open-label, crossover design |
17/17 |
4 weeks |
Australia |
22.4 |
7/10 |
24.1 |
Healthy |
No |
No |
600 ml tea powder, 486 mg total polyphenols, 405 mg flavanols |
200 ml placebo, no matched caffeine |
No significant change |
High risk of bias |
Ashigai (2016)22 |
Randomized, placebo-controlled, double-blind, crossover design |
25/25 |
10 days |
Japan |
40.4 |
13/12 |
22.2 |
Healthy |
No |
No |
1050 ml tea powder, 165 mg total polyphenols, 24 mg flavanols |
1050 ml placebo, matched caffeine |
No significant change |
Low risk of bias |
Bingham (1997)33 |
Randomized, placebo-controlled, single-blind, cross-over design |
65/65 |
4 weeks |
UK |
40.0 |
31/34 |
24.2 |
Healthy |
No |
No |
1800 ml leaf tea, 1494 mg of flavanols |
1800 ml placebo, matched caffeine |
No significant change |
High risk of bias |
Duffy (2001)35 (2 hours) |
Randomized, placebo-controlled, cross-over design |
50/50 |
2 hours |
USA |
55.0 |
39/11 |
29.7 |
CAD |
Yes |
No |
450 ml leaf tea, 733.5 mg tea polyphenols, 477 mg flavanols |
450 ml placebo, no matched caffeine |
No significant change |
High risk of bias |
Duffy (2001)35 (4 weeks) |
Randomized, placebo-controlled, cross-over design |
50/50 |
4 weeks |
USA |
55.0 |
39/11 |
29.7 |
CAD |
Yes |
No |
900 ml extract powder, 1350 mg tea polyphenols, 873 mg flavanols |
900 ml placebo, no matched caffeine |
No significant change |
High risk of bias |
Grassia (2009)19 (100 mg flavanols) |
Randomized, placebo-controlled, double-blind, cross-over design |
19/19 |
1 week |
Italy |
32.9 |
19/0 |
23.9 |
Healthy |
No |
No |
300 ml tea powder, 100 mg flavanols |
300 ml placebo, matched caffeine |
No significant change |
Low risk of bias |
Grassi (2009) (200 mg flavanols) |
Randomized, placebo-controlled, double-blind, cross-over design |
19/19 |
1 week |
Italy |
32.9 |
19/0 |
23.9 |
Healthy |
No |
No |
300 ml tea powder, 200 mg flavanols |
300 ml placebo, matched caffeine |
No significant change |
Low risk of bias |
Grassi (2009) (400 mg flavanols) |
Randomized, placebo-controlled, double-blind, cross-over design |
19/19 |
1 week |
Italy |
32.9 |
19/0 |
23.9 |
Healthy |
No |
No |
300 ml tea powder, 400 mg flavanols |
300 ml placebo, matched caffeine |
Significant reduction in SBP and DBP |
Low risk of bias |
Grassi (2009) (800 mg flavanols) |
Randomized, placebo-controlled, double-blind, cross-over design |
19/19 |
1 week |
Italy |
32.9 |
19/0 |
23.9 |
Healthy |
No |
No |
300 ml tea powder, 800 mg flavanols |
300 ml placebo, matched caffeine |
Significant reduction in SBP and DBP |
Low risk of bias |
Grassi (2009) (AMBP, 100 mg flavanols) |
Randomized, placebo-controlled, double-blind, cross-over design |
19/19 |
1 week |
Italy |
32.9 |
19/0 |
23.9 |
Healthy |
No |
Yes |
300 ml tea powder, 100 mg flavanols |
300 ml placebo, matched caffeine |
No significant change |
Low risk of bias |
Grassi (2009) (AMBP, 200 mg flavanols) |
Randomized, placebo-controlled, double-blind, cross-over design |
19/19 |
1 week |
Italy |
32.9 |
19/0 |
23.9 |
Healthy |
No |
Yes |
300 ml tea powder, 200 mg flavanols |
300 ml placebo, matched caffeine |
No significant change |
Low risk of bias |
Grassi (2009) (AMBP, 400 mg flavanols) |
Randomized, placebo-controlled, double-blind, cross-over design |
19/19 |
1 week |
Italy |
32.9 |
19/0 |
23.9 |
Healthy |
No |
Yes |
300 ml tea powder, 400 mg flavanols, |
300 ml placebo, matched caffeine |
No significant change |
Low risk of bias |
Grassi (2009) (AMBP, 800 mg flavanols) |
Randomized, placebo-controlled, double-blind, cross-over design |
19/19 |
1 week |
Italy |
32.9 |
19/0 |
23.9 |
Healthy |
No |
Yes |
300 ml tea powder, 800 mg flavanols, |
300 ml placebo, matched caffeine |
No significant change |
Low risk of bias |
Grassi (2015)31 |
Randomized, placebo-controlled, double-blind, cross-over design |
19/19 |
8 days |
Italy |
51.5 |
5/14 |
27.1 |
Essential hypertensive (EH) |
No |
No |
300 ml tea powder, 300 mg tea polyphenols, 258 mg flavanols |
300 ml placebo, matched caffeine |
Significant reduction in SBP and DBP |
Low risk of bias |
Hodgson (1999)32 (1 hour) |
Randomized, placebo-controlled, cross-over design |
20/20 |
1 hour |
Australia |
56.2 |
20/0 |
25.6 |
Healthy |
No |
No |
400 ml leaf tea, 740 mg polyphenols, 594 mg flavanols |
400 ml placebo, matched caffeine |
No significant change |
Unclear risk of bias |
Hodgson (1999)32 (7 days) |
Randomized, placebo-controlled, cross-over design |
13/13 |
7 days |
Australia |
59.8 |
10/3 |
27.0 |
High-normal systolic BP and mild systolic hypertension |
No |
Yes |
1000 ml leaf tea, 1029 mg polyphenols, 830 mg flavanols |
1000 ml placebo, matched caffeine |
No significant change |
Unclear risk of bias |
Hodgson (2002)38 |
Randomized, placebo-controlled, open-label, parallel design |
10/11 |
4 weeks |
Australia |
59.1 |
16/5 |
27.5 |
MHC |
No |
No |
1250 ml leaf tea, 1037.5 mg flavanols |
1250 ml placebo, no matched caffeine |
No significant change |
High risk of bias |
Hodgson (2003)39 |
Randomized, placebo-controlled, cross-over design |
22/22 |
4 weeks |
Australia |
59.0 |
16/6 |
27.0 |
Mildly elevated serum total cholesterol concentrations |
No |
No |
1250 ml tea leaf, 1037.5 mg flavanols |
1250 ml placebo, no matched caffeine |
No significant change |
High risk of bias |
Hodgson (2005)36 |
Randomized, placebo-controlled, open-label, cross-over design |
20/20 |
4 hours |
Australia |
62.1 |
— |
28.1 |
CAD |
Yes |
No |
750 ml tea leaf, 900 mg of polyphenols |
750 ml placebo, no matched caffeine |
Significant increase in SBP |
High risk of bias |
Hodgson (2012)20 |
Randomized, placebo-controlled, double-blind, parallel design |
46/49 |
6 months |
Australia |
56.6 |
33/62 |
25.1 |
Healthy |
No |
Yes |
600 ml tea powder, 429 mg of polyphenols, 360.4 mg flavanols |
600 ml placebo, no matched caffeine |
Significant reduction in SBP and DBP |
Low risk of bias |
Hodgson (2013)34 |
Randomized, placebo-controlled, double-blind, parallel design |
41/43 |
1 day |
Australia |
56.2 |
31/53 |
25.1 |
Normal to mildly elevated systolic blood pressure |
Yes |
Yes |
600 ml tea leaf, 429 mg total polyphenols |
600 ml placebo, matched caffeine |
No significant change |
High risk of bias |
Mukamal (2007)37 |
Randomized, placebo-controlled, parallel design |
14/14 |
6 months |
USA |
65.8 |
10/18 |
29.1 |
High CVD risk |
Yes |
No |
600 ml tea powder, 318 mg of flavanols |
600 ml placebo, no matched caffeine |
No significant change |
High risk of bias |
Data synthesis and statistical analysis
The effect sizes were reported as weighted mean difference (WMD) between the black tea groups and the control groups for SBP or DBP and their standard deviations (SD). The weighted mean differences (WMD) and 95% confidence intervals (CI) were determined using a random-effects model following the DerSimonian and Laird method.25 Net changes in SBP and DBP were calculated by subtracting the value at the baseline from that after intervention in the tea groups and in the control groups. SDs of the mean differences were calculated as follows: SD = square root [(SD baseline)2 + (SD final)2 − (2R × SD baseline × SD final)], assuming a correlation coefficient (R) of D 0.5 for both parallel and crossover study designs.26 If the required values were reported in mean and variation range or mean and inter-quartile range, SDs were calculated as described by Hozo et al.27 If only standard error of the mean (SEM) was reported, then the SD was estimated as follows: SD = SEM × √n, where “n” is the number of subjects.28 Furthermore, 95% CIs were also converted to SDs using the formula: SD = sqrt(n) × (upper CI limit (UL) − lower CI limit (LL))/3.92.
When results were presented at multiple time points, only data relating to the longest duration of treatment were considered. Furthermore, considering that the confounding effects from different black tea doses could influence the results, we included them separately. The results of the selected studies were combined using the generic inverse variance method and a fixed- or random-effects model, depending respectively on the presence of low-to-moderate (<50%) or high (>50%) heterogeneity, which was quantitatively assessed using Higgins index (I2). Effect sizes were expressed as WMD and 95% confidence interval (CI). Subgroup analyses were performed based on prespecified factors, including duration of intervention, dose of black tea flavanol supplements, type of intervention, caffeine, gender, mean age, BMI, study country, study design, ambulatory BP, participant health status and risk of bias.
Finally, to explore the influence of each study on the overall effect size, sensitivity analyses were performed. A leave-one-out method was used (i.e. one study was removed at a time and the analysis was repeated).29 All statistical analyses were performed using STATA (Version 12.0, Stata Corp, College Station, TX). P < 0.05 was regarded as statistically significant.
Meta-regression
Univariate and multivariate meta-regression analyses were performed to estimate the impact of various research characteristics on the effect size. The selection of covariates for meta-regression analysis mainly depended on the results of subgroup analysis and biological knowledge. Given the type I errors of the multivariate meta-regression, we also applied the Monte Carlo permutation test.
Dose–response analysis
For all study arms, fractional polynomial models (polynomials) were used to explore the nonlinear associations between the black tea flavonoid supplementation dosage (mg day−1) and the duration of intervention (days).
Publication bias
Potential publication biases were explored using visual inspection of funnel plot asymmetry. Duval and Tweedie's “trim and fill” method was used to adjust for the effects of publication bias in the analysis.30
Results
Study selection
After database searches according to the inclusion and exclusion criteria, 920 published studies were identified and the abstracts were reviewed. Of these, 93 were non-original articles and so they were excluded; another 175 studies were eliminated because they did not meet the inclusion criteria. Thus, 29 full text articles were carefully assessed and reviewed. After assessment, 12 studies were excluded due to the following reasons: the study selection process is shown in Fig. 1.The primary search identified a total of 920 articles; of these, 343 duplicates were removed. A flowchart of the detailed steps of the selection procedure is shown in Fig. 1. After screening by title/abstract, 539 publications were excluded and 38 records remained for further examination. Of these articles, 25 were excluded for the following reasons: no blood pressure outcome reported (n = 15), insufficient data reported for the outcomes (n = 5), full text unavailable except the abstract (n = 2), lack of a control group (n = 1), and the intervention was performed in combination with other components (n = 2). Therefore, a total of 13 trials, including 22 study arms, were eligible for inclusion in the final quantitative analysis.
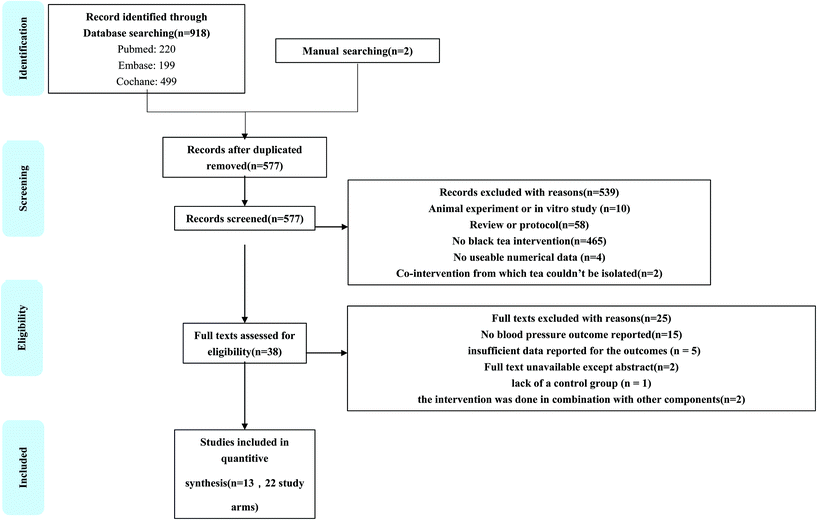 |
| Fig. 1 Flow diagram of the number of literature searches and selection strategy. | |
Study characteristics
The characteristics of studies including 22 study arms are described in Table 1. Selected qualified trials enrolled 1115 participants aged from 20 to 75 years. All study arms included both male and female participants except nine arms that had exclusively recruited men.31,32 The number of subjects in the intervention and control groups was 556 and 559 in total, respectively. Participants were healthy individuals,19–22,32,33 patients with hypertension,31,32,34 coronary artery diseases (CAD),20,35,36 high CVD risk,37 MHC,38 and mildly elevated serum total cholesterol concentrations.39 Their baseline BMI varied from 22.2 to 29.7 kg m−2. These trials were published from 1997 to 2018 and were performed in Australia,20,21,32,34,36,38,39 Japan,22 UK,33 USA,35,37 and Italy.19,31 All selected studies were cross-over RCTs except for four arms with a parallel design.20,34,37,38 The dose of black tea prescribed in these trials varied from 300 ml to 1800 ml, and the duration of black tea supplementation ranged from 1 day to 6 months.
In terms of findings, four study arms indicated that black tea supplementation had a significant reducing effect on SBP19,20,31 while other study arms failed to find such a significant reducing effect. With regard to DBP, six study arms revealed a significant reduction,19,20,31 and others failed to find any favourable effect on DBP after black tea supplementation.
Risk of bias assessment
We used the bias assessment tool recommended by the Cochrane Collaboration to evaluate the quality of the included studies (Fig. 2). Nine study arms were judged to be at high risk of bias, two arms were at an unclear risk of bias, and 11 study arms were at low risk of bias. Details of the quality of bias assessment are reported in Fig. 2.
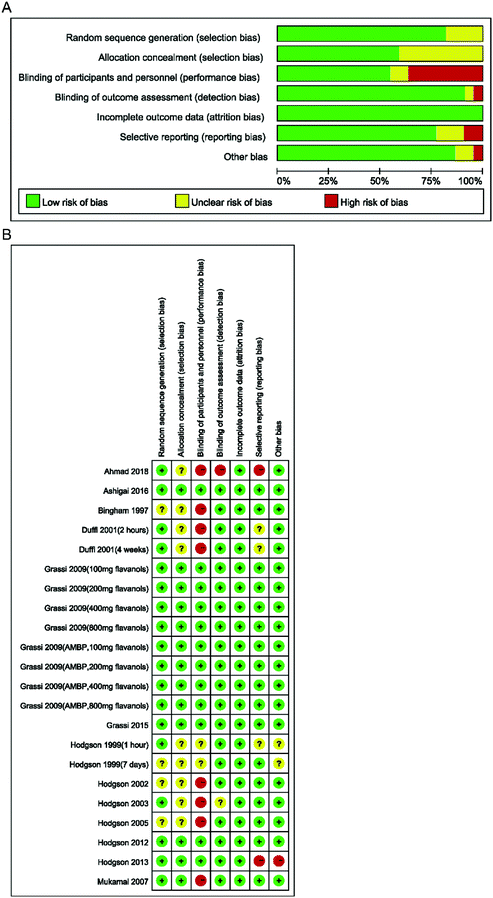 |
| Fig. 2 Risk of bias. A risk of bias graph; B risk of bias summary. | |
The pooled effect of black tea supplementation on SBP
The effect of black tea supplementation on SBP was examined in 22 study arms, including a total of 1115 participants. Our analysis based on the random-effects model revealed that black tea supplementation resulted in a significant reduction in SBP (WMD – 1.04 mmHg; 95% CI – 2.05 to −0.03; and P = 0.04). However, between-study heterogeneity was high (I2 = 71%, P < 0.00001) (Fig. 3A). These results were robust in the leave-one-out sensitivity analysis.
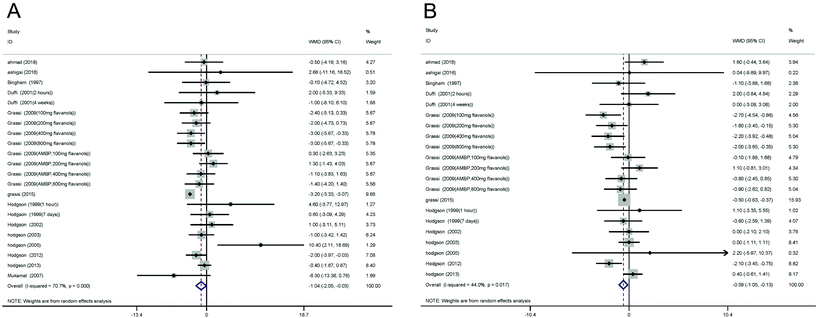 |
| Fig. 3 A and B Forest plot presenting weighted mean difference and 95% confidence intervals (CIs) for the effect of black tea supplementation on SBP and DBP. | |
In our subgroup analyses, a significant reduction in SBP was observed in trials with a duration of ≥7 days (WMD – 1.49 mmHg; 95% CI – 2.41, −0.56; and P = 0.002), but not in studies with a duration of less than 7 days (WMD 3.06 mmHg; 95% CI – 1.73, 7.86; and P = 0.05). SBP was also decreased in trials that administered black tea flavanol supplements at dosages between 200 mg day−1 and 800 mg day−1 (WMD – 1.52 mmHg; 95% CI – 2.83, −0.22; and P < 0.0001), in studies that used the black tea powder (WMD – 1.86 mmHg; 95% CI – 2.82, −0.91; and P = 0.02), in those that were performed on matched caffeine (WMD – 1.36 mmHg; 95% CI – 2.43, −0.28; and P < 0.0001), in studies that included a larger proportion of males (WMD – 1.24 mmHg; 95% CI – 2.33, −0.15; and P = 0.0009), in trials that were performed in subjects with a mean age of <50 years (WMD – 1.33 mmHg; 95% CI – 2.25, −0.41; and P = 0.50), in those that were performed in people with BMI ≤25 kg m−2 (WMD – 1.33 mmHg; 95% CI – 2.55, −0.41; and P = 0.50), in trials that were carried out in European countries (WMD – 1.80 mmHg; 95% CI – 2.94, −0.66; and P = 0.01), and in trials that were at low risk of bias (WMD – 1.86 mmHg; 95% CI – 2.87 −0.84; and P = 0.01) (Table 2).
Table 2 Meta-analysis displaying the effect of black tea supplementation on blood pressure based on several subgroups
Subgroup |
Trials (n) |
Change in SBP |
Trials (n) |
Change in DBP |
Net change mmHg (95% CI) |
P
|
I
2
|
Net change mmHg (95% CI) |
P
|
I
2
|
Duration
|
<7 days |
4 |
3.06 (−1.73, 7.86) |
0.05 |
62% |
4 |
0.62 (−0.30, 1.54) |
0.73 |
0 |
≥7 days |
18 |
−1.49 (−2.41, −0.56) |
0.002 |
56% |
17 |
−0.79 (−1.29, −0.30) |
0.03 |
44% |
Flavonols
|
>200, ≤800 |
12 |
−1.52 (−2.83, −0.22) |
<0.001 |
74% |
10 |
−0.79 (−1.47, −0.11) |
0.01 |
58% |
>800 |
5 |
−0.25 (−1.89, 1.39) |
0.91 |
0 |
5 |
−0.03 (−0.83, 0.78) |
0.58 |
0 |
≤200 |
5 |
−0.68 (−2.29, 0.93) |
0.28 |
21% |
5 |
−0.88 (−2.39, 0.64) |
0.04 |
59% |
Type of intervention
|
Black tea powder |
14 |
−1.86 (−2.82, −0.91) |
0.02 |
50% |
13 |
−0.94 (−1.58, −0.31) |
0.007 |
56% |
Black tea leaf |
8 |
0.25 (−1.14, 1.73) |
0.26 |
21% |
8 |
0.17 (−0.45, 0.79) |
0.82 |
0 |
Caffeine
|
Matched caffeine |
14 |
−1.36 (−2.43, −0.28) |
<0.001 |
73% |
14 |
−0.90 (−1.45, −0.36) |
0.01 |
52% |
No matched caffeine |
8 |
0.08 (−2.21, 2.36) |
0.16 |
33% |
7 |
0.43 (−0.37, 1.24) |
0.75 |
0 |
Gender (male/female)
|
>50% |
16 |
−1.24 (−2.33, −0.15) |
<0.001 |
60% |
16 |
−0.56 (−1.07, −0.05) |
0.04 |
41% |
<50% |
5 |
−1.02 (−2.23, 0.19) |
0.35 |
9% |
4 |
−0.83 (−2.53, 0.88) |
0.03 |
65% |
Mean age
|
<50 |
11 |
−1.33 (−2.25, −0.41) |
0.50 |
0 |
11 |
−0.93 (−1.76, −0.11) |
0.03 |
49% |
≥50 |
11 |
−0.67 (−2.33, 0.99) |
<0.001 |
79% |
10 |
−0.31 (−0.84, 0.23) |
0.15 |
33% |
BMI
|
>25 |
11 |
−0.67 (−2.33, 0.99) |
<0.001 |
79% |
10 |
−0.31 (−0.84, 0.23) |
0.15 |
33% |
≤25 |
11 |
−1.33 (−2.25, −0.41) |
0.50 |
0 |
11 |
−0.93 (−1.76, −0.11) |
0.03 |
49% |
Country
|
Europe |
10 |
−1.80 (−2.94,−0.66) |
0.01 |
58% |
10 |
−1.03 (−1.67, −0.39) |
0.04 |
50% |
Not Europe |
12 |
−0.40 (−1.62, 0.81) |
0.20 |
25% |
11 |
0.01 (−0.75, 0.77) |
0.13 |
33% |
Study design
|
Parallel |
4 |
−1.06 (−2.68, 1.57) |
0.18 |
38% |
3 |
−0.58 (−2.29, 1.13) |
0.01 |
77% |
Crossover |
18 |
−1.01 (−2.14, 0.12) |
<0.001 |
65% |
18 |
−0.51 (−0.63, −0.39) |
0.06 |
37% |
Ambulatory BP
|
Yes |
7 |
−0.57 (−1.40, 0.26) |
0.53 |
0 |
7 |
−0.45 (−1.27, 0.38) |
0.07 |
48% |
No |
15 |
−1.56 (−2.78, −0.34) |
0.009 |
52% |
14 |
−0.88 (−1.56, −0.20) |
0.007 |
55% |
Health status of subjects
|
High-normal or hypertension |
3 |
−1.34 (−3.82, 1.14) |
<0.001 |
91% |
3 |
−0.49 (−0.61, −0.36) |
0.22 |
34% |
Normotension |
19 |
−1.08 (−1.98, −0.17) |
0.18 |
23% |
18 |
−0.69 (−1.35, −0.03) |
0.02 |
45% |
Risk of bias
|
Low |
11 |
−1.86 (−2.87, −0.84) |
0.01 |
56% |
11 |
−1.15 (−1.81, −0.50) |
0.01 |
56% |
Unclear |
2 |
1.25 (−2.13, 4.63) |
0.39 |
0 |
2 |
−0.32 (−2.13, 1.50) |
0.49 |
0 |
High |
9 |
−0.24 (−1.64, 1.16) |
0.24 |
23% |
8 |
−0.35 (−0.27, 0.96) |
0.72 |
0 |
The pooled effect of black tea supplementation on DBP
In twenty-one eligible trials, including a total of 1087 subjects, the effect of black tea supplementation on DBP was examined. Our analysis based on the random-effects model revealed that black tea supplementation resulted in a significant reduction in DBP (WMD – 0.59 mmHg; 95% CI – 1.05 to −0.13; and P = 0.01). However, there was significant heterogeneity between studies (I2 = 71%, P < 0.00001) (Fig. 3B). These effects were robust in the leave-one-out sensitivity analysis.
Subgroup analyses based on study duration [I2 = 44%, P = 0.03], dose of black tea flavanol supplements [I2 = 58%, P = 0.01], type of black tea supplements [I2 = 56%, P = 0.007], male sex [I2 = 41%, P = 0.04], and health status of subjects [I2 = 5%, P = 0.39] explained this heterogeneity. We found a significant reduction in DBP with a black tea intervention duration of ≥7 days (WMD – 0.79 mmHg; 95% CI – 1.29, −0.30; and P = 0.03). In addition, a significant effect on DBP was observed in trials that administered black tea flavanol supplements at dosages between 200 mg day−1 and 800 mg day−1 (WMD – 0.79 mmHg; 95% CI – 1.47, −0.11; and P = 0.01), in those that used the black tea powder (WMD – 0.94 mmHg; 95% CI – 1.58, −0.31; P = 0.007), in those that were performed in population that included greater number of men (WMD – 0.56 mmHg; 95% CI – 1.07, −0.05; P = 0.04), in trials that were performed in European countries (WMD – 1.03 mmHg; 95% CI – 1.67, −0.39; and P = 0.04), and in trials that were at low risk of bias (WMD – 1.15 mmHg; 95% CI – 1.81, −0.50; and P = 0.001) (Table 2).
Meta-regression analysis
The results of meta-regression analysis of the effects of black tea supplementation on BP (expressed as WMD) are shown in Table S1, Fig. S1 and S2.† Whether caffeine was matched or ambulatory BP (AMBP) was used did not affect the BP-lowering effect of black tea supplementation in univariate analysis. Both whether caffeine was matched and AMBP was used affected the SBP-lowering effect of black tea supplementation in multivariate analysis.
The dose–response effect of black tea on blood pressure
Using the dose–response assessment, we found a significant non-linear association between the duration of black tea supplementation and SBP (P nonlinearity = 0.0018), and a greater reduction was observed when the prescribed durations were longer than 7 days (Fig. 4A). A significant non-linear association was also found for DBP (P nonlinearity = 0.02) (Fig. 4B). In addition, the flavanol dose was not significantly associated with SBP (P nonlinearity = 0.42) (Fig. 5A) and DBP (P nonlinearity = 0.50) (Fig. 5B).
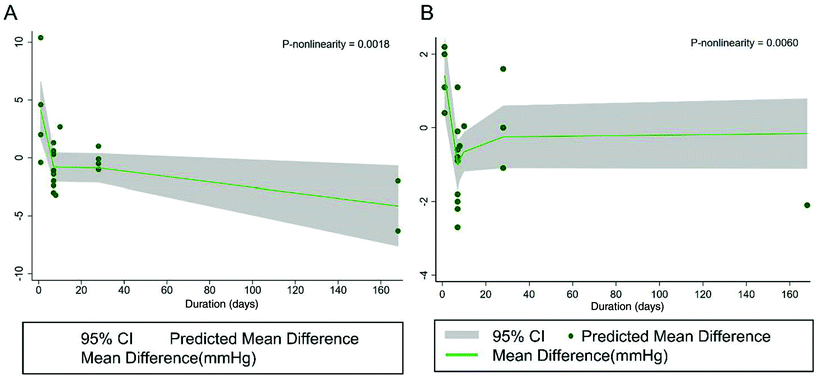 |
| Fig. 4 Non-linear dose–response relationship between the duration of treatment (days) and unstandardized mean difference in A SBP and B DBP. The 95% CI is shown in the shaded regions. | |
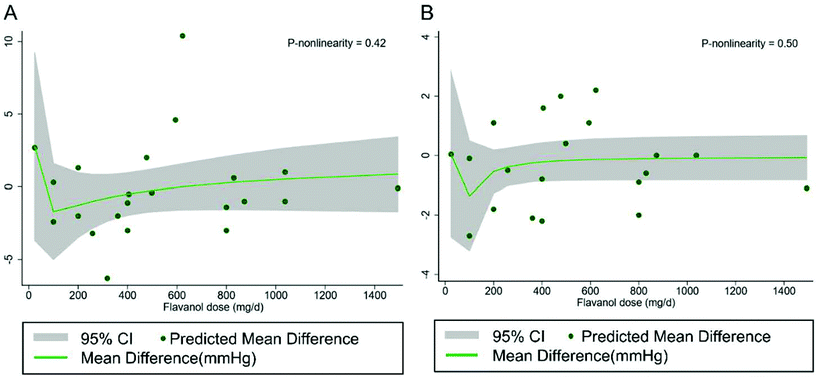 |
| Fig. 5 Non-linear dose–response relationship between flavanol dosage (mg day−1) and unstandardized mean difference in A SBP and B DBP. The 95% CI is shown in the shaded regions. | |
Publication biases
The funnel plots of standard error by WMD were asymmetric (Fig. 6), suggesting potential publication biases in the meta-analysis of the black tea effect on BP. The conclusions were not changed after adjustment for publication bias by the asymmetries using Duval and Tweedie's “trim and fill” method. Correction of the asymmetries yielded potentially missing studies for SBP [10 missing studies of the funnel plot, adjusted effect size = −2.69 (95% CI: −3.65, −1.73) mmHg] and DBP [2 missing studies of the funnel plot, adjusted effect size = −0.65 (95% CI: −1.12, −0.19) mmHg] (Fig. 7).
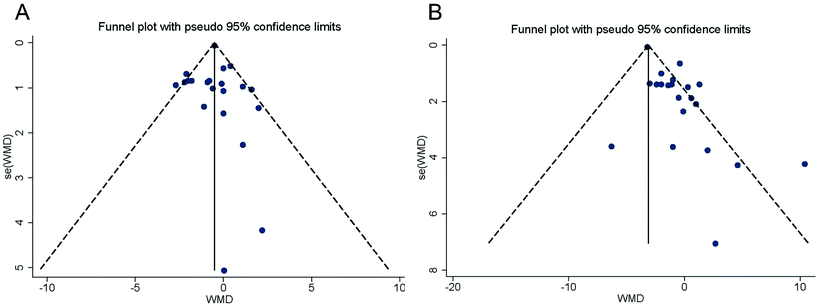 |
| Fig. 6 Funnel plots of the RCTs on the effect of black tea on A SBP and B DBP. | |
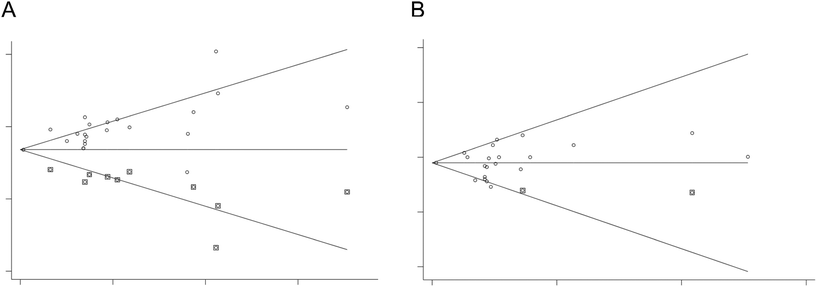 |
| Fig. 7 Adjustment for publication bias by the asymmetries using Duval and Tweedie's “trim and fill” method in A SBP and B DBP. | |
Discussion
This systematic review and meta-analysis of RCTs found an inverse association between black tea supplementation and BP. Although some observational studies were included in our initial search, we restricted the meta-analysis to RCTs only, because evidence provided by these studies is of relatively higher quality. The association could suggest a potential beneficial role of black tea in the prevention of hypertension. In addition, the relationship seems to be more evident in men and in European populations. Conversely, a non-significant association between black tea supplementation and BP was observed, though it failed to indicate a significant influence of black tea flavonoid supplementation dose or duration on either SBP or DBP.
Previously, prospective observational studies were performed to determine the effect of black tea on CVD; however, the results were contradictory. A large population-based meta-analysis involving 856
206 individuals revealed that the consumption of three cups of tea per day was associated with a reduced risk of CVD and death from CVDs.40 Some studies have revealed that black tea supplements significantly lowered BP.19,31 In contrast, several other studies have reported that there were no positive correlations between black tea supplementation and BP reduction.21,22 To clarify the precise effect of black tea on BP control, the current meta-analysis of published RCTs was performed.
The present meta-analysis is the first dose–response meta-analysis of RCTs performed to evaluate the effect of black tea supplementation on BP. Data were extracted from 13 trials, including 22 study arms, retrieving a total of 1115 patients from different geographical settings (Australia, Japan, UK, USA, and Italy). Our results showed that black tea supplementation significantly decreased SBP (WMD – 1.04 mmHg; 95% CI – 2.05 to −0.03; and P = 0.04) and DBP (WMD – 0.59 mmHg; 95% CI – 1.05 to −0.13; and P = 0.01). In addition, the subgroup analyses based on gender indicated that the BP-lowering effect of black tea was more significant in men than in women. This result was in congruence with a prospective cohort study of 0.5 million Chinese adults consuming black tea, which demonstrated that black tea lowered the risk of stroke in men, but not in women.41 Furthermore, the subgroup analyses indicated that black tea showed a significant BP-lowering effect in European populations. The fact that countries with the highest per capita consumption of black tea in the world are in Europe42 may account for the result, because European populations might have consumed black tea supplements for a longer duration. Moreover, higher-quality studies have indicated that black tea exhibits a significant BP-lowering effect.
Most oral supplements have a relatively nonlinear dose–response pattern and, as a consequence, an increased dosage is not directly associated with a higher response.43,44 In our analysis, we observed a non-linear dose–response relationship between the duration of black tea supplementation and SBP and DBP. In addition, the dose of black tea supplements was not significantly associated with SBP and DBP in a nonlinear fashion. Nevertheless, as the blood pressure levels are affected by multiple variables such as neuro-endocrine regulation and the renal-endocrine system,45 the lack of significant non-linear association is not unexpected.
Consistent with this study, previous in vivo studies have revealed that blood pressures were normalized and endothelial functions were improved by black tea consumption in rat models.16,46 Although the exact mechanism underlying the effect of black tea supplementation on blood pressure remains to be fully elucidated, increasing evidence suggest that it may provide antihypertensive benefits, such as decreased peripheral vascular tone and wave reflections, suggesting vasodilation of peripheral arteries and a decrease in vascular resistance, rendering a putative mechanism for BP reduction;31 the mechanism could probably be related to increased nitric oxide bioavailability, as the regulation of arterial mechanics in peripheral conduit arteries is principally mediated by nitric oxide.47,48 Tea flavonoids, as antioxidants, cause a reduction in oxidative stress resulting in enhanced synthesis and release, as well as reduced breakdown of endothelium-derived nitric oxide.49,50 Moreover, in addition to flavonoids, tea contains other potentially relevant active substances that positively affect the vascular function. In this regard, Siamwala et al.51 showed that L-theanine administration in vitro enhanced NO production and thereby vasodilation in the arteries, suggesting that L-theanine might mediate the vascular health benefits of tea.
At the population level, the observed differences in BP are strongly associated with the death rate from stroke, and vascular causes or ischemic heart disease in people between 40 and 69 years of age.8 This relationship has been shown to start from 115/75 mmHg.8 Our data revealed the blood pressure-relieving benefits of black tea supplementation, substantiating the potential of black tea to be developed as beneficial dietary supplementation for hypertensive patients. Further investigations into the effects of black tea and their underlying mechanisms may provide an avenue for many dietary-based approaches to lowering BP.
The present study is the first dose–response meta-analysis of RCTs performed to evaluate the impact of black tea supplementation on blood pressure in adults. Sole inclusion of RCTs in this study leads to robust causal inference of findings. Furthermore, subgroup analyses performed in this study allowed the examination of the effects across different subgroups and detection of possible sources of heterogeneity.
Potential limitations
Limitations of this study are as follows: firstly, our study included the relatively restricted number of randomized-controlled trials. Second, we had some sort of between-study heterogeneity in some of our analyses. Thus, there is a clear need for researchers to conduct high-quality randomized-controlled trials, at different doses, while also analysing any adverse effects following black tea supplementation. In addition, the effect of black tea supplementation on blood pressure may be dependent on the pre-intervention black tea status and change in circulating black tea concentrations from the baseline. In addition, language and publication bias limited our study. In future, there is a need for a greater diversity of research to be included in the analysis, such as multi-center studies, more rigorous clinical reports, and prospective studies.
Conclusions
In conclusion, the favourable effect of black tea emerging from the current meta-analysis suggests the possible use of black tea as an active compound in order to promote cardiovascular health, particularly when used for longer durations and in men. Our findings may provide evidence for physicians and medical researchers on the efficacy of alternative treatments for patients with hypertension.
Conflicts of interest
There are no conflicts to declare.
Acknowledgements
This work was supported by the State Key Program of National Natural Science Foundation of China 81530014; the National Key R&D Plan of China 2017YFC1700502; and the National Natural Science Foundation for Young Scientists of China 81700366.
References
- E. J. Benjamin, M. J. Blaha, S. E. Chiuve, M. Cushman, S. R. Das, R. Deo, S. D. De Ferranti, J. Floyd, M. Fornage, C. Gillespie, C. R. Isasi, M. C. Jim'nez, L. C. Jordan, S. E. Judd, D. Lackland, J. H. Lichtman, L. Lisabeth, S. Liu, C. T. Longenecker, R. H. MacKey, K. Matsushita, D. Mozaffarian, M. E. Mussolino, K. Nasir, R. W. Neumar, L. Palaniappan, D. K. Pandey, R. R. Thiagarajan, M. J. Reeves, M. Ritchey, C. J. Rodriguez, G. A. Roth, W. D. Rosamond, C. Sasson, A. Towfghi, C. W. Tsao, M. B. Turner, S. S. Virani, J. H. Voeks, J. Z. Willey, J. T. Wilkins, J. H. Y. Wu, H. M. Alger, S. S. Wong and P. Muntner, Circulation, 2017, 135, e146–e603 CrossRef.
- Y. N. V. Reddy and B. A. Borlaug, Eur. J. Heart Failure, 2020, 22, 469–471 CrossRef.
- M. H. Forouzanfar, P. Liu, G. A. Roth, M. Ng, S. Biryukov, L. Marczak, L. Alexander, K. Estep, K. H. Abate, T. F. Akinyemiju, R. Ali, N. Alvis-Guzman, P. Azzopardi, A. Banerjee, T. Bärnighausen, A. Basu, T. Bekele, D. A. Bennett, S. Biadgilign, F. Catalá-López, V. L. Feigin, J. C. Fernandes, F. Fischer, A. A. Gebru, P. Gona, R. Gupta, G. J. Hankey, J. B. Jonas, S. E. Judd, Y. H. Khang, A. Khosravi, Y. J. Kim, R. W. Kimokoti, Y. Kokubo, D. Kolte, A. Lopez, P. A. Lotufo, R. Malekzadeh, Y. A. Melaku, G. A. Mensah, A. Misganaw, A. H. Mokdad, A. E. Moran, H. Nawaz, B. Neal, F. N. Ngalesoni, T. Ohkubo, F. Pourmalek, A. Rafay, R. K. Rai, D. Rojas-Rueda, U. K. Sampson, I. S. Santos, M. Sawhney, A. E. Schutte, S. G. Sepanlou, G. T. Shifa, I. Shiue, B. A. Tedla, A. G. Thrift, M. Tonelli, T. Truelsen, N. Tsilimparis, K. N. Ukwaja, O. A. Uthman, T. Vasankari, N. Venketasubramanian, V. V. Vlassov, T. Vos, R. Westerman, L. L. Yan, Y. Yano, N. Yonemoto, M. El Sayed Zaki and C. J. L. Murray, JAMA, J. Am. Med. Assoc., 2017, 317, 165–182 CrossRef.
- M. Naghavi, A. A. Abajobir, C. Abbafati, K. M. Abbas, F. Abd-Allah, S. F. Abera, V. Aboyans, O. Adetokunboh, J. Ärnlöv, A. Afshin, A. Agrawal, A. A. Kiadaliri, A. Ahmadi, M. B. Ahmed, A. N. Aichour, I. Aichour, M. T. E. Aichour, S. Aiyar, A. Al-Eyadhy, F. Alahdab, Z. Al-Aly, K. Alam, N. Alam, T. Alam, K. A. Alene, S. D. Ali, R. Alizadeh-Navaei, J. M. Alkaabi, A. Alkerwi, F. Alla, P. Allebeck, C. Allen, R. Al-Raddadi, U. Alsharif, K. A. Altirkawi, N. Alvis-Guzman, A. T. Amare, E. Amini, W. Ammar, Y. A. Amoako, N. Anber, H. H. Andersen, C. L. Andrei, S. Androudi, H. Ansari, C. A. T. Antonio, P. Anwari, M. Arora, A. Artaman, K. K. Aryal, H. Asayesh, S. W. Asgedom, T. M. Atey, L. Avila-Burgos, E. F. G. A. Avokpaho, A. Awasthi, B. P. A. Quintanilla, Y. Béjot, T. K. Babalola, U. Bacha, K. Balakrishnan, A. Barac, M. A. Barboza, S. L. Barker-Collo, S. Barquera, L. Barregard, L. H. Barrero, B. T. Baune, N. Bedi, E. Beghi, B. B. Bekele, M. L. Bell, J. R. Bennett, I. M. Bensenor, A. Berhane, E. Bernabé, B. D. Betsu, M. Beuran, S. Bhatt, S. Biadgilign, K. Bienhof, B. Bikbov, D. Bisanzio, R. R. A. Bourne, N. J. K. Breitborde, L. N. B. Bulto, B. R. Bumgarner, Z. A. Butt, R. Cárdenas, L. Cahuana-Hurtado, E. Cameron, J. C. Campuzano, J. Car, J. J. Carrero, A. Carter, D. C. Casey, C. A. Castañeda-Orjuela, F. Catalá-López, F. J. Charlson, C. E. Chibueze, O. Chimed-Ochir, V. H. Chisumpa, A. A. Chitheer, D. J. Christopher, L. G. Ciobanu, M. Cirillo, A. J. Cohen, D. Colombara, C. Cooper, B. C. Cowie, M. H. Criqui, L. Dandona, R. Dandona, P. I. Dargan, J. Das Neves, D. V. Davitoiu, K. Davletov, B. De Courten, L. Degenhardt, S. Deiparine, K. Deribe, A. Deribew, S. Dey, D. Dicker, E. L. Ding, S. Djalalinia, H. P. Do, D. T. Doku, D. Douwes-Schultz, T. R. Driscoll, M. Dubey, B. B. Duncan, M. Echko, Z. Z. El-Khatib, C. L. Ellingsen, A. Enayati, H. E. Erskine, S. Eskandarieh, A. Esteghamati, S. P. Ermakov, K. Estep, C. S. Sa Farinha, A. Faro, F. Farzadfar, V. L. Feigin, S. M. Fereshtehnejad, J. C. Fernandes, A. J. Ferrari, T. R. Feyissa, I. Filip, S. Finegold, F. Fischer, C. Fitzmaurice, A. D. Flaxman, N. Foigt, T. Frank, M. Fraser, N. Fullman, T. Fürst, J. M. Furtado, E. Gakidou, A. L. Garcia-Basteiro, T. Gebre, G. B. Gebregergs, T. T. Gebrehiwot, D. Y. Gebremichael, J. M. Geleijnse, R. Genova-Maleras, H. A. Gesesew, P. W. Gething, R. F. Gillum, I. A. M. Ginawi, A. Z. Giref, M. Giroud, G. Giussani, W. W. Godwin, A. L. Gold, E. M. Goldberg, P. N. Gona, S. V. Gopalani, H. N. Gouda, A. C. Goulart, M. Griswold, P. C. Gupta, R. Gupta, T. Gupta, V. Gupta, J. A. Haagsma, N. Hafezi-Nejad, A. D. Hailu, G. B. Hailu, R. R. Hamadeh, M. T. Hambisa, S. Hamidi, M. Hammami, J. Hancock, A. J. Handal, G. J. Hankey, Y. Hao, H. L. Harb, H. A. Hareri, M. S. Hassanvand, R. Havmoeller, S. I. Hay, F. He, M. T. Hedayati, N. J. Henry, I. B. Heredia-Pi, C. Herteliu, H. W. Hoek, M. Horino, N. Horita, H. D. Hosgood, S. Hostiuc, P. J. Hotez, D. G. Hoy, C. Huynh, K. M. Iburg, C. Ikeda, B. V. Ileanu, A. A. Irenso, C. M. S. Irvine, M. Jürisson, K. H. Jacobsen, N. Jahanmehr, M. B. Jakovljevic, M. Javanbakht, S. P. Jayaraman, P. Jeemon, V. Jha, D. John, C. O. Johnson, S. C. Johnson, J. B. Jonas, Z. Kabir, R. Kadel, A. Kahsay, R. Kamal, A. Karch, S. M. Karimi, C. Karimkhani, A. Kasaeian, N. A. Kassaw, N. J. Kassebaum, S. V. Katikireddi, N. Kawakami, P. N. Keiyoro, L. Kemmer, C. N. Kesavachandran, Y. S. Khader, E. A. Khan, Y. H. Khang, A. T. A. Khoja, A. Khosravi, M. H. Khosravi, J. Khubchandani, C. Kieling, D. Kievlan, D. Kim, Y. J. Kim, R. W. Kimokoti, Y. Kinfu, N. Kissoon, M. Kivimaki, A. K. Knudsen, J. A. Kopec, S. Kosen, P. A. Koul, A. Koyanagi, B. K. Defo, X. R. Kulikof, G. A. Kumar, P. Kumar, M. Kutz, H. H. Kyu, D. K. Lal, R. Lalloo, T. L. N. Lambert, Q. Lan, V. C. Lansingh, A. Larsson, P. H. Lee, J. Leigh, J. Leung, M. Levi, Y. Li, D. L. Kappe, X. Liang, M. L. Liben, S. S. Lim, A. Liu, P. Y. Liu, Y. Liu, R. Lodha, G. Logroscino, S. Lorkowski, P. A. Lotufo, R. Lozano, T. C. D. Lucas, S. Ma, E. R. K. Macarayan, E. R. Maddison, M. M. Abd El Razek, M. Majdan, R. Majdzadeh, A. Majeed, R. Malekzadeh, R. Malhotra, D. C. Malta, H. Manguerra, T. Manyazewal, C. C. Mapoma, L. B. Marczak, D. Markos, J. Martinez-Raga, F. R. Martins-Melo, I. Martopullo, C. McAlinden, M. McGaughey, J. J. McGrath, S. Mehata, T. Meier, K. G. Meles, P. Memiah, Z. A. Memish, M. M. Mengesha, D. T. Mengistu, B. G. Menota, G. A. Mensah, A. Meretoja, T. J. Meretoja, A. Millear, T. R. Miller, S. Minnig, M. Mirarefn, E. M. Mirrakhimov, A. Misganaw, S. R. Mishra, K. A. Mohammad, A. Mohammadi, S. Mohammed, A. H. Mokdad, G. L. D. Mola, S. K. Mollenkopf, M. Molokhia, L. Monasta, J. C. M. Hernandez, M. Montico, M. D. Mooney, M. Moradi-Lakeh, P. Moraga, L. Morawska, S. D. Morrison, C. Morozof, C. Mountjoy-Venning, K. B. Mruts, K. Muller, G. V. S. Murthy, K. I. Musa, J. B. Nachega, A. Naheed, L. Naldi, V. Nangia, B. R. Nascimento, J. T. Nasher, G. Natarajan, I. Negoi, J. W. Ngunjiri, C. T. Nguyen, G. Nguyen, M. Nguyen, Q. Le Nguyen, T. H. Nguyen, E. Nichols, D. N. A. Ningrum, V. M. Nong, J. J. N. Noubiap, F. A. Ogbo, I. H. Oh, A. Okoro, A. T. Olagunju, H. E. Olsen, B. O. Olusanya, J. O. Olusanya, K. Ong, J. N. Opio, E. Oren, A. Ortiz, M. Osman, E. Ota, P. A. Mahesh, R. E. Pacella, S. Pakhale, A. Pana, B. K. Panda, S. Jonas, C. Papachristou, E. K. Park, S. B. Patten, G. C. Patton, D. Paudel, K. Paulson, D. M. Pereira, F. Perez-Ruiz, N. Perico, A. Pervaiz, M. Petzold, M. R. Phillips, D. M. Pigott, C. Pinho, D. Plass, M. A. Pletcher, S. Polinder, M. J. Postma, F. Pourmalek, C. Purcell, M. Qorbani, A. Radfar, A. Rafay, V. Rahimi-Movaghar, M. Rahman, M. H. Ur Rahman, R. K. Rai, C. L. Ranabhat, Z. Rankin, P. C. Rao, G. K. Rath, S. Rawaf, S. E. Ray, J. Rehm, R. C. Reiner, M. B. Reitsma, G. Remuzzi, S. Rezaei, M. S. Rezai, M. B. Rokni, L. Ronfani, G. Roshandel, G. A. Roth, D. Rothenbacher, G. M. Ruhago, S. A. Rizwan, S. Saadat, P. S. Sachdev, N. Sadat, M. Safdarian, S. Saf, S. Safiri, R. Sagar, R. Sahathevan, J. Salama, P. Salamati, J. A. Salomon, A. M. Samy, J. R. Sanabria, M. D. Sanchez-Niño, D. Santomauro, I. S. Santos, M. M. S. Milicevic, B. Sartorius, M. Satpathy, S. Shahraz, M. I. Schmidt, I. J. C. Schneider, S. Schulhofer-Wohl, A. E. Schutte, D. C. Schwebel, F. Schwendicke, S. G. Sepanlou, E. E. Servan-Mori, K. A. Shackelford, M. A. Shaikh, M. Shamsipour, M. Shamsizadeh, S. M. S. Islam, J. Sharma, R. Sharma, J. She, S. Sheikhbahaei, M. Shey, P. Shi, C. Shields, M. Shigematsu, R. Shiri, S. Shirude, I. Shiue, H. Shoman, M. G. Shrime, I. D. Sigfusdottir, N. Silpakit, J. P. Silva, A. Singh, J. A. Singh, E. Skiadaresi, A. Sligar, A. Smith, D. L. Smith, M. Smith, B. H. A. Sobaih, S. Soneji, R. J. D. Sorensen, J. B. Soriano, C. T. Sreeramareddy, V. Srinivasan, J. D. Stanaway, V. Stathopoulou, N. Steel, D. J. Stein, C. Steiner, S. Steinke, M. A. Stokes, M. Strong, B. Strub, M. Subart, M. B. Sufyan, B. F. Sunguya, P. J. Sur, S. Swaminathan, B. L. Sykes, R. Tabarés-Seisdedos, S. K. Tadakamadla, K. Takahashi, J. S. Takala, R. T. Talongwa, M. R. Tarawneh, M. Tavakkoli, N. Taveira, T. K. Tegegne, A. Tehrani-Banihashemi, M. H. Temsah, A. S. Terkawi, J. S. Thakur, O. Thamsuwan, K. R. Thankappan, K. E. Thomas, A. H. Thompson, A. J. Thomson, A. G. Thrift, R. Tobe-Gai, R. Topor-Madry, A. Torre, M. Tortajada, J. A. Towbin, B. X. Tran, C. Troeger, T. Truelsen, D. Tsoi, E. M. Tuzcu, S. Tyrovolas, K. N. Ukwaja, E. A. Undurraga, R. Updike, O. A. Uthman, B. S. C. Uzochukwu, J. F. M. Van Boven, T. Vasankari, N. Venketasubramanian, F. S. Violante, V. V. Vlassov, S. E. Vollset, T. Vos, T. Wakayo, M. T. Wallin, Y. P. Wang, E. Weiderpass, R. G. Weintraub, D. J. Weiss, A. Werdecker, R. Westerman, B. Whetter, H. A. Whiteford, T. Wijeratne, C. S. Wiysonge, B. G. Woldeyes, C. D. A. Wolfe, R. Woodbrook, A. Workicho, D. Xavier, Q. Xiao, G. Xu, M. Yaghoubi, B. Yakob, Y. Yano, M. Yaseri, H. H. Yimam, N. Yonemoto, S. J. Yoon, M. Yotebieng, M. Z. Younis, Z. Zaidi, M. El Sayed Zaki, E. A. Zegeye, Z. M. Zenebe, T. A. Zerfu, A. L. Zhang, X. Zhang, B. Zipkin, S. Zodpey, A. D. Lopez and C. J. L. Murray, Lancet, 2017, 390, 1151–1210 CrossRef.
- S. S. Lim, T. Vos, A. D. Flaxman, G. Danaei, K. Shibuya, H. Adair-Rohani, M. Amann, H. R. Anderson, K. G. Andrews, M. Aryee, C. Atkinson, L. J. Bacchus, A. N. Bahalim, K. Balakrishnan, J. Balmes, S. Barker-Collo, A. Baxter, M. L. Bell, J. D. Blore, F. Blyth, C. Bonner, G. Borges, R. Bourne, M. Boussinesq, M. Brauer, P. Brooks, N. G. Bruce, B. Brunekreef, C. Bryan-Hancock, C. Bucello, R. Buchbinder, F. Bull, R. T. Burnett, T. E. Byers, B. Calabria, J. Carapetis, E. Carnahan, Z. Chafe, F. Charlson, H. Chen, J. S. Chen, A. T. A. Cheng, J. C. Child, A. Cohen, K. E. Colson, B. C. Cowie, S. Darby, S. Darling, A. Davis, L. Degenhardt, F. Dentener, D. C. Des Jarlais, K. Devries, M. Dherani, E. L. Ding, E. R. Dorsey, T. Driscoll, K. Edmond, S. E. Ali, R. E. Engell, P. J. Erwin, S. Fahimi, G. Falder, F. Farzadfar, A. Ferrari, M. M. Finucane, S. Flaxman, F. G. R. Fowkes, G. Freedman, M. K. Freeman, E. Gakidou, S. Ghosh, E. Giovannucci, G. Gmel, K. Graham, R. Grainger, B. Grant, D. Gunnell, H. R. Gutierrez, W. Hall, H. W. Hoek, A. Hogan, H. D. Hosgood, D. Hoy, H. Hu, B. J. Hubbell, S. J. Hutchings, S. E. Ibeanusi, G. L. Jacklyn, R. Jasrasaria, J. B. Jonas, H. Kan, J. A. Kanis, N. Kassebaum, N. Kawakami, Y. H. Khang, S. Khatibzadeh, J. P. Khoo, C. Kok, F. Laden, R. Lalloo, Q. Lan, T. Lathlean, J. L. Leasher, J. Leigh, Y. Li, J. K. Lin, S. E. Lipshultz, S. London, R. Lozano, Y. Lu, J. Mak, R. Malekzadeh, L. Mallinger, W. Marcenes, L. March, R. Marks, R. Martin, P. McGale, J. McGrath, S. Mehta, G. A. Mensah, T. R. Merriman, R. Micha, C. Michaud, V. Mishra, K. M. Hanafiah, A. A. Mokdad, L. Morawska, D. Mozaffarian, T. Murphy, M. Naghavi, B. Neal, P. K. Nelson, J. M. Nolla, R. Norman, C. Olives, S. B. Omer, J. Orchard, R. Osborne, B. Ostro, A. Page, K. D. Pandey, C. D. H. Parry, E. Passmore, J. Patra, N. Pearce, P. M. Pelizzari, M. Petzold, M. R. Phillips, D. Pope, C. A. Pope, J. Powles, M. Rao, H. Razavi, E. A. Rehfuess, J. T. Rehm, B. Ritz, F. P. Rivara, T. Roberts, C. Robinson, J. A. Rodriguez-Portales, I. Romieu, R. Room, L. C. Rosenfeld, A. Roy, L. Rushton, J. A. Salomon, U. Sampson, L. Sanchez-Riera, E. Sanman, A. Sapkota, S. Seedat, P. Shi, K. Shield, R. Shivakoti, G. M. Singh, D. A. Sleet, E. Smith, K. R. Smith, N. J. C. Stapelberg, K. Steenland, H. Stöckl, L. J. Stovner, K. Straif, L. Straney, G. D. Thurston, J. H. Tran, R. Van Dingenen, A. Van Donkelaar, J. L. Veerman, L. Vijayakumar, R. Weintraub, M. M. Weissman, R. A. White, H. Whiteford, S. T. Wiersma, J. D. Wilkinson, H. C. Williams, W. Williams, N. Wilson, A. D. Woolf, P. Yip, J. M. Zielinski, A. D. Lopez, C. J. L. Murray and M. Ezzati, Lancet, 2012, 380, 2224–2260 CrossRef.
- B. M. Egan, S. E. Kjeldsen, G. Grassi, M. Esler and G. Mancia, J. Hypertens., 2019, 37, 1148–1153 CrossRef CAS.
- D. Ettehad, C. A. Emdin, A. Kiran, S. G. Anderson, T. Callender, J. Emberson, J. Chalmers, A. Rodgers and K. Rahimi, Lancet, 2016, 387, 957–967 CrossRef.
- S. Lewington, R. Clarke, N. Qizilbash, R. Peto and R. Collins, Lancet, 2002, 360, 1903–1913 CrossRef.
- B. Williams, G. Mancia, W. Spiering, E. A. Rosei, M. Azizi, M. Burnier, D. L. Clement, A. Coca, G. De Simone, A. Dominiczak, T. Kahan, F. Mahfoud, J. Redon, L. Ruilope, A. Zanchetti, M. Kerins, S. E. Kjeldsen, R. Kreutz, S. Laurent, G. Y. H. Lip, R. Mcmanus, K. Narkiewicz, F. Ruschitzka, R. E. Schmieder, E. Shlyakhto, C. Tsioufis, V. Aboyans and I. Desormais, J. Hypertens., 2018, 36, 1956–2041 Search PubMed.
- C. Borghi, K. Tsioufis, E. Agabiti-Rosei, M. Burnier, A. F. G. Cicero, D. Clement, A. Coca, G. Desideri, G. Grassi, D. Lovic, E. Lurbe, T. Kahan, R. Kreutz, B. Jelakovic, J. Polonia, J. Redon, P. Van De Borne and G. Mancia, J. Hypertens., 2020, 38, 799–812 CrossRef CAS.
- C. Borghi and A. F. G. Cicero, Br. J. Clin. Pharmacol., 2017, 83, 163–171 CrossRef CAS.
- Q. P. Dou, Nutrients, 2019, 11(4), 929 CrossRef CAS.
- J. Shi, D. Xie, D. Qi, Q. Peng, Z. Chen, M. Schreiner, Z. Lin and S. Baldermann, Front. Plant Sci., 2019, 10, 781 CrossRef.
- K. Kent, K. Charlton, T. O'Sullivan and W. H. Oddy, Eur. J. Nutr., 2020, 1–16 Search PubMed.
- Z. Yan, Y. Zhong, Y. Duan, Q. Chen and F. Li, Anim. Nutr., 2020, 6, 115–123 CrossRef.
- W. S. Cheang, C. Y. Ngai, Y. Y. Tam, X. Y. Tian and W. T. Wong, Sci. Rep., 2015, 5, 1–10 Search PubMed.
- D. Li, R. Wang, J. Huang, Q. Cai, C. S. Yang, X. Wan and Z. Xie, Nutrients, 2019, 11, 1115 CrossRef CAS.
- J. Fang, A. Sureda, A. S. Silva, F. Khan, S. Xu and S. M. Nabavi, Trends Food Sci. Technol., 2019, 88, 385–396 CrossRef CAS.
- D. Grassia, T. P. J. Mulder, R. Draijer, G. Desideri, H. O. F. Molhuizen and C. Ferri, J. Hypertens., 2009, 27, 774–781 CrossRef.
- J. M. Hodgson, I. B. Puddey, R. J. Woodman, T. P. J. Mulder, D. Fuchs, K. Scott and K. D. Croft, Arch. Intern. Med., 2012, 172(2), 186–188 CrossRef.
- A. F. Ahmad, L. Rich, H. Koch, K. D. Croft, M. G. Ferruzzi, C. D. Kay, J. M. Hodgson and N. C. Ward, Food Funct., 2018, 9, 6307–6314 RSC.
- H. Ashigai, Y. Taniguchi, M. Suzuki, E. Ikeshima, T. Kanaya, K. Zembutsu, S. Tomita, M. Miyake and I. Fukuharae, Biol. Pharm. Bull., 2016, 39, 699–704 CrossRef CAS.
- D. Moher, A. Liberati, J. Tetzlaff, D. G. Altman and P. Grp, Phys. Ther., 2009, 89, 873–880 CrossRef.
- J. P. T. Higgins, D. G. Altman, P. C. Gøtzsche, P. Jüni, D. Moher, A. D. Oxman, J. Savović, K. F. Schulz, L. Weeks and J. A. C. Sterne, Br. Med. J., 2011, 343, 1–9 CrossRef.
- R. DerSimonian and N. Laird, Controlled Clin. Trials, 1986, 7, 177–188 CrossRef CAS.
- C. Applications and N. Heart, J. Clin. Epidemiol., 1992, 45(7), 769–773 CrossRef.
- S. P. Hozo, B. Djulbegovic and I. Hozo, BMC Med. Res. Methodol., 2005, 5, 1–10 CrossRef.
- A. Sahebkar, Ž. Reiner, L. E. Simental-Mendía, G. Ferretti and A. F. G. Cicero, Metabolism, 2016, 65, 1664–1678 CrossRef CAS.
- F. Fogacci, A. F. G. Cicero, G. Derosa, M. Rizzo, M. Veronesi and C. Borghi, Crit. Rev. Food Sci. Nutr., 2019, 59, 328–335 CrossRef CAS.
- S. Duval and R. Tweedie, Biometrics, 2000, 56, 455–463 CrossRef CAS.
- D. Grassi, R. Draijer, G. Desideri, T. Mulder and C. Ferri, Nutrients, 2015, 7, 1037–1051 CrossRef CAS.
- J. M. Hodgson, I. B. Puddey, V. Burke, L. J. Beilin and N. Jordan, J. Hypertens., 1999, 17, 457–463 CrossRef CAS.
- S. A. Bingham, H. Vorster, J. C. Jerling, E. Magee, A. Mulligan, S. A. Runswick and J. H. Cummings, Br. J. Nutr., 1997, 78, 41–55 CrossRef CAS.
- J. M. Hodgson, R. J. Woodman, I. B. Puddey, T. Mulder, D. Fuchs and K. D. Croft, Food Funct., 2013, 4, 111–115 RSC.
- S. J. Duffy, J. F. Keaney, M. Holbrook, N. Gokce, P. L. Swerdloff, B. Frei and J. A. Vita, Asia Pac. J. Clin. Nutr, 2001, 104(2), 151–156 CAS.
- J. M. Hodgson, V. Burke and I. B. Puddey, J. Hypertens., 2005, 23, 47–54 CrossRef CAS.
- K. J. Mukamal, K. MacDermott, J. A. Vinson, N. Oyama, W. J. Manning and M. A. Mittleman, Am. Heart J., 2007, 154, 724.e1–724.e6 CrossRef.
- J. M. Hodgson, I. B. Puddey, V. Burke, G. F. Watts and L. J. Beilin, Clin. Sci., 2002, 102, 195–201 CrossRef.
- J. M. Hodgson, V. Burke, L. J. Beilin, K. D. Croft and I. B. Puddey, Am. J. Clin. Nutr., 2003, 77, 907–911 CrossRef CAS.
- C. Zhang, Y. Y. Qin, X. Wei, F. F. Yu, Y. H. Zhou and J. He, Eur. J. Epidemiol., 2015, 30, 103–113 CrossRef CAS.
- T. Tian, J. Lv, G. Jin, C. Yu, Y. Guo, Z. Bian, L. Yang, Y. Chen, H. Shen, Z. Chen, Z. Hu and L. Li, Am. J. Clin. Nutr., 2019, 1–10 Search PubMed.
-
D. T. Waugh, W. Potter, H. Limeback and M. Godfrey, mdpi.com, DOI: DOI:10.3390/ijerph13030259.
- T. Filippini, F. Violi, R. D'Amico and M. Vinceti, Int. J. Cardiol., 2017, 230, 127–135 CrossRef.
- F. Ding, B. Ma, A. Nazary-Vannani, H. Kord-Varkaneh, S. Fatahi, M. Papageorgiou, J. Rahmani, F. Poursoleiman, I. Borges do Nascimento Júnior, H. Li, D. Han and D. Wang, Nutr. Metab. Cardiovasc. Dis., 2020, 30, 1–10 CrossRef CAS.
- T. M. Seccia, B. Caroccia, M. L. Muiesan and G. P. Rossi, Int. J. Cardiol., 2016, 206, 71–76 CrossRef.
- F. P. Leung, L. M. Yung, C. Y. Ngai, W. S. Cheang, X. Y. Tian, C. W. Lau, Y. Zhang, J. Liu, Z. Y. Chen, Z. X. Bian, X. Yao and Y. Huang, Eur. J. Nutr., 2016, 55, 1963–1972 CrossRef.
- I. B. Wilkinson, A. Qasem, C. M. McEniery, D. J. Webb, A. P. Avolio and J. R. Cockcroft, Circulation, 2002, 105, 213–217 CrossRef CAS.
- G. Y. Tang, X. Meng, R. Y. Gan, C. N. Zhao, Q. Liu, Y. Bin Feng, S. Li, X. L. Wei, A. G. Atanasov, H. Corke and H. Bin Li, Int. J. Mol. Sci., 2019, 20(24), 6196 CrossRef CAS.
- J. M. May, Free Radicals Biol. Med., 2000, 28, 1421–1429 CrossRef CAS.
- C. Forni, F. Facchiano, M. Bartoli, S. Pieretti, A. Facchiano, D. D'Arcangelo, S. Norelli, G. Valle, R. Nisini, S. Beninati, C. Tabolacci and R. N. Jadeja, BioMed Res. Int., 2019, 2019, 8748253 Search PubMed.
- J. H. Siamwala, P. M. Dias, S. Majumder, M. K. Joshi, V. P. Sinkar, G. Banerjee and S. Chatterjee, J. Nutr. Biochem., 2013, 24, 595–605 CrossRef CAS.
Footnote |
† Electronic supplementary information (ESI) available. See DOI: 10.1039/d0fo02122a |
|
This journal is © The Royal Society of Chemistry 2021 |
Click here to see how this site uses Cookies. View our privacy policy here.